- Department of Molecular, Cellular and Biomedical Sciences, Center for Molecular and Comparative Endocrinology, University of New Hampshire, Durham, NH, USA
The hypothalamic-pituitary system is considered to be a vertebrate innovation and seminal event that emerged prior to or during the differentiation of the ancestral agnathans. Lampreys are the earliest evolved vertebrates for which there is a demonstrated neuroendocrine system. Lampreys have three hypothalamic gonadotropin-releasing hormones (GnRHs; lGnRH-I, -II, and -III) and two and possibly three pituitary GnRH receptors involved in mediating reproductive processes. Estradiol is considered to be a major reproductive steroid in both male and female lampreys. The purpose of this study was to investigate estrogen receptor (ER) expression in the lamprey brain in adult sea lampreys. Expression of ER mRNA was confirmed in the adult lamprey brain using RT-PCR. Using digoxigenin (DIG)-labeled probes, ER expression was shown to yield moderate, but distinct reaction products in specific neuronal nuclei of the lamprey brain, including the olfactory lobe, hypothalamus, habenular area, and hindbrain. Expression of ER in the hypothalamic area of the brain provides evidence of potential interaction between estradiol and GnRH(s), and is consistent with previous evidence showing estrogen feedback on GnRH in adult lamprey brain. Earlier studies have reported that there is a close distribution of glutamic acid decarboxylase (GAD; GABA-synthesizing enzyme) and lamprey GnRH in the preoptic region in adult lampreys. The establishment of a direct estradiol–kisspeptin–GABA–GnRH interaction in lamprey has yet to be determined and will require future functional and co-localization studies. The phylogenetic position of lampreys as a basal vertebrate allows lampreys to be a basis for understanding the molecular evolution of the neuroendocrine system that arose in the vertebrates.
Introduction
Modern vertebrates are classified into two major groups, the gnathostomes (jawed vertebrates) and the agnathans (jawless vertebrates). The agnathans are classified into two groups, myxinoids (hagfish) and petromyzonids (lampreys), while the gnathostomes constitute all other living vertebrates including the bony and cartilaginous fishes and the tetrapods. The hypothalamic-pituitary system is considered to be a vertebrate innovation and seminal event that emerged prior to or during the differentiation of the ancestral agnathans (Sower et al., 2009). Lampreys are the earliest evolved vertebrates for which there is a demonstrated neuroendocrine system. Lampreys have three hypothalamic gonadotropin-releasing hormone (GnRHs; lGnRH-I, -II, and -III) and two and possibly three pituitary GnRH receptors involved in mediating reproductive processes (Sower et al., 2009; Hall et al., 2011).
In lampreys, there is a general pattern of GnRH distribution in the anterior-preoptic-neurohypophysial tract to the neurohypophysis of adult lampreys as determined by several immunohistochemical (IHC) studies using specific GnRH antisera to each of the lamprey GnRHs and also by in situ hybridization studies using specific GnRH probes (Crim et al., 1979a,b; Nozaki and Kobayashi, 1979; King et al., 1988; Nozaki et al., 1994; Reed et al., 2002; Kavanaugh et al., 2008). Data showing GnRH immunoreactive (ir) neurons in adult lampreys using IHC showed cells restricted to a single bilateral dense arc along the third ventricle in the rostral hypothalamus and preoptic area. Of all vertebrates, only the agnathan and teleosts lack a portal vascular system (median eminence) for transferring neurohormones from the hypothalamus to the adenohypophysis (Gorbman, 1965). Studies were done to experimentally examine the functional anatomical relationship between the hypothalamus and adenohypophysis in sea lamprey (Nozaki et al., 1994). It was shown that neurosecretory peptides like GnRH diffuse from the brain (neurohypophysis) to the adenohypophysis, and thus regulate its secretory activity in lampreys. Thus, there is evidence of normal occurrence of GnRH in a part of the lamprey brain homologous with that brain region in later evolved vertebrates in which GnRH localization forms part of a neuroendocrine mechanism for gonadotropin secretion. In other words, neurosecretory peptides like GnRH are able to diffuse from the brain to the pituitary to regulate its secretory activity. In addition, Crim (1981) and King et al. (1988) showed that GnRH neurons project into the third ventricle. These authors proposed an additional route of GnRH via secretion into the third ventricle and transported by tanycytes to the adenohypophysis (King et al., 1988).
There are several important brain neurohormones/factors that have been shown to stimulate/modulate GnRH and/or gonadotropin synthesis and/or release in vertebrates. In some teleosts, those neurohormones/factors include dopamine, neuropeptide Y, gamma-aminobutyric acid (GABA), and more recently gonadotropin-inhibitory hormone (GnIH) and kisspeptin (KiSS; Kah and Dufour, 2010). In lampreys, GABA and neuropeptide Y have been shown to be involved with brain GnRH and reproduction (Conlon et al., 1994; Root et al., 2004, 2005). Glutamic acid decarboxylase (GAD; GABA-synthesizing enzyme) mRNA was expressed in four distinct cell populations in the lamprey brain, ranging from the telencephalon and diencephalon of the forebrain to the mesencephalon and rhombencephalon of the midbrain and hindbrain in adult lamprey (Root et al., 2005). The close distribution of GAD and lamprey GnRH in the preoptic region supports the hypothesis that GABA might act on the reproductive axis through the feedback on GnRH neurons (Reed et al., 2002; Root et al., 2005).
Data supporting estrogen feedback on GnRH has been shown in lamprey. Sower (1997) observed that adult female lamprey injected with microencapsulated E2 experienced an elevation in GnRH concentrations, and activity of E2 in the hypothalamic region is supported in vitro autoradiography studies demonstrating binding of E2 in that area (Kim et al., 1980, 1981). However, it is unclear if the E2–GnRH interaction in lamprey occurs through a direct or an indirect mechanism. Within the preoptic anterior hypothalamus, co-localization studies of GABA and GnRH in lamprey have indicated GABA and GnRH-containing cells are closely associated (Reed et al., 2002; Root et al., 2005), supporting the possibility of a GABA-mediated (indirect) mechanism of E2–GnRH interaction in lamprey.
With the cloning of an estrogen receptor (ER), it is now possible to examine the expression of the receptor in the adult lamprey brain (Thornton, 2001). In contrast to teleost fish that have three types of ER (Hawkins et al., 2000; Menuet et al., 2002), lampreys apparently only have one ER (Thornton, 2001). Earlier studies in mammals suggested that the mechanism of E2 action on GnRH is largely indirect, and neurons of the anteroventral periventricular brain region that express the neurotransmitter, gamma amino butyric acid (GABA), are believed to be responsible for facilitating interaction between E2 and GnRH (Petersen et al., 2003). The discovery of kisspeptin and subsequent studies now suggest that steroid feedback may also be occurring on GnRH neurons via kisspeptin and its receptor (Roa et al., 2009).
The proximity of GnRH to GABAergic neurons and the effects of GABA/muscimol on GnRH support a role for GABA in the regulation of lamprey GnRH. However, such studies do not necessarily imply E2 involvement in GABA–GnRH interaction. Until recently, it was not clear that a classical ER existed in lamprey. Thornton’s (2001) discovery and cloning of a lamprey provides an opportunity to enhance our understanding of the relationships between GABA, GnRH, and E2 in lamprey. Therefore, in the present study, we examined the expression patterns of ER in the adult sea lamprey, Petromyzon marinus, brain in relation to GnRH and GABA.
Materials and Methods
Collection and Sampling
Adult female sea lampreys were collected from the fish ladder on the Cocheco River in Dover, New Hampshire. Collection of lamprey occurred in May and June during two successive seasons during the lamprey’s upstream spawning migration from the ocean to coastal rivers. The lampreys were transported to the freshwater fish hatchery at the University of New Hampshire and maintained in an artificial spawning channel supplied with flow-through water from a nearby stream-fed reservoir at an ambient temperature range of 13–20°C, under natural photoperiod following the University of New Hampshire Institutional Animal Care and Use guidelines.
RT-PCR
Expression of ER was examined in the lamprey brain using RT-PCR, both for the purpose of verifying expression and to generate cDNA template for the synthesis of in situ hybridization probes. Expression was also assessed in ovary, testis, and muscle tissue for the purpose of comparison with expression in the brain. One adult, female lamprey was killed by decapitation. Tissues for were snap frozen in liquid nitrogen and stored at −80°C. Total RNA was isolated from ∼100 mg of each tissue using Tri-Reagent (Molecular Research Center, Inc., Cincinnati, OH, USA). Gene specific primers were selected against the partial cDNA sequence of ER cloned from the sea lamprey by Thornton (2001), Accession #AY028456, using Primer31 and were obtained from Integrated DNA technology (Coralville, IA, USA).
Estrogen receptor left primer (first set): 5′-CCTCGTGCACAGAGTTCT-3′
Estrogen receptor right primer (first set): 5′-GTAGCGATCCGGAGCTGA-3′
Estrogen receptor left primer (second set: 5′-GACATGTTCGACATGCTGCT-3′
Estrogen receptor right primer (second set): 5′-AGCGGGATCACATTCTTACG-3′
RT-PCR reactions were prepared (16.75 μl H20, 2.5 μl buffer, 1.5 μl MgCl2, 0.5 μl dNTPs, 0.25 μl Taq Polymerase, 500 nM primer concentration, and 1 μl template). RT-PCR reactions were performed using an Eppendorf Thermal Cycler under the following conditions: 48°C/1 min; (95°C/15 s; 60.1°C/1 min; 72°C/1 min) × 35 cycles; 72°C/5 min; 10°C/hold. The reaction products were analyzed on 1% agarose gels electrophoresed at 90 V for 1 h and stained with ethidium bromide for purposes of visualization.
For the purpose of verifying the sequence identity of candidate ER-bands, RT-PCR products were first gel purified as described in the QIAEX II Gel Purification Kit© (QUIGEN, Valencia, CA, USA). Purified products were then inserted into the pGEM-T-Easy vector (Promega, Madison, WI, USA) and subsequently transformed into E. coli JM109 cells (Promega). Ligations and transformations were performed following the protocol described in the pGEM-T-Easy Vector System (Promega). Overnight cultures were used for plasmid preparation with the Wizard Plus Miniprep system (Promega), following the manufacturer’s protocol. Purified plasmid was then sent for sequencing at the University of Utah’s Health Science Center2.
RNA Probe Synthesis
Templates for probe synthesis were prepared in plasmids as described above. Purified plasmid containing the ER insert was then digested overnight at 37°C with either SalI or NcoI [2 μg BSA and 1× Buffer D (Promega)] restriction enzymes producing singly digested linearized plasmid. Digestions were analyzed by 1% agarose gel electrophoresis to confirm presence of expected clones. Riboprobes were synthesized using the SP6 Riboprobe Synthesis Kit (Promega), and either 35S- or Digoxigenin (DIG)-labeled UTP as previously described (Rubin et al., 1997). This protocol was slightly modified in that the transcription reactions were allowed to continue overnight instead of 2 h.
Evaluation of RNA Probe Yield
The yield of DIG-labeled probes was evaluated by spectrometric analysis of total RNA and a modified DIG Northern blot protocol (Allen et al., 2000). For the Northern blot, in the place of total RNA, full length probe template cDNA used for each riboprobe synthesis was cross-linked to a nylon membrane and hybridized with the different anti-sense and sense riboprobes. Briefly, template cDNA was denatured for 2 min at 100°C, spotted onto nylon membrane and UV cross-linked. Hybridization solution (50% dextran sulfate, 4 × SSC, 1 × Denhardt’s, 1 mg/ml yeast tRNA, 10 mM DTT) was prepared and mixed with 0.5 mg/ml DIG-labeled RNA diluted in DEPC water. Probes were applied to each template cDNA and allowed to hybridize overnight at 55°C. Following hybridization, membranes were washed twice in 2 × SSC for 5 min each at RT, 1 × SSC for 15 min at 55°C, and Maleate Buffer (0.1 M maleic acid, 0.3% Tween, 0.15 M NaCl; pH 7.5) for 2 min at RT. Membranes were blocked in Blocking Buffer for 30 min at RT and then incubated in anti-DIG-alkaline phosphatase (AP; Roche) antibody (1:5000) for 30 min at RT. Membranes were washed twice in Maleate Buffer for 15 min each at RT, and then incubated in nitro blue tetrazolium/5-bromo-4-chloro-3-indolyl phosphate [NBT/BCIP (Roche) 1:50 diluted in 100 mM Tris–HCl, 100 mM NaCl, 50 mM Mg2Cl2; pH 9.5)] in darkness for 3 h at RT.
For 35S-labeled probes, radioactivity in the waste products from the LiCl precipitation and washing of probes was measured and compared against the activity of the purified probes. Based on this relationship, the % incorporation of 35S-UTP was calculated. And based on the % of label incorporated, product yield from the synthesis reactions was calculated.
In situ Hybridization
Forty-seven adult, female lamprey were killed by decapitation. Brains were dissected, immersed in O.C.T. Compound (Miles Inc. Elkhart, IN), and then frozen on dry ice. Sagittal tissue sections of 16–18 μm were cut the day of dissection on a cryostat (Reichert-Jung, Leica Instruments, Heidelberg, Germany) at −12°C. Sections were then mounted onto Vectabond (Vector Laboratories, Burlingame, CA, USA) coated slides and immediately moved into tissue preparation steps of day 1 of the in situ hybridization protocol. Tissue sections were incubated with either anti-sense (odd numbered slides) or sense (even numbered slides) riboprobes for lamprey ER. The application and signal development of DIG-labeled probes followed the protocol previously described by Rubin et al. (1997), and characterized for use in lamprey by Root et al. (2005).
The application and signal development of 35S-labeled probes followed the protocol characterized by Root et al. (2005), with the exception of signal development, which followed the protocol described by Hrabovszky et al. (2004). The concentration of probe in the hybridization solution, the concentration of antibody applied, and the stringency of the washes were all varied to optimize the results. Following signal development, both 35S and DIG-labeled tissue sections were viewed under light microscopy using an Olympus BH2 microscope, and digital photographs were captured with a Nikon Coolpix 5000 camera. A detailed visual assessment was made of signal deposition for each slide. In addition, images were also collected at Tufts Medical School using a Zeiss Axioscope and Spot RT color camera (Diagnostic Instruments, Inc.), and submitted for analysis by Optimus™ analysis software, which quantitatively compared the signal intensity between sense and anti-sense slides.
Results
RT-PCR
The first set of primers for lamprey ER yielded specific transcripts in ovarian tissue, but not in brain, testis, or muscle tissue (results not shown). In contrast, the second set primers for lamprey ER yielded specific transcripts in all tissues examined, with strongest expression in ovarian and muscle tissues (Figure 1). Notably, the expression of ER in the target tissue (brain) was exceptionally weak. Megalign™ analysis software was used to compare sequenced PCR products with the published sequence for lamprey ER. Specifically, the transcript cloned from the first set of primers (ER1 = 407 bp total length) had 100% identity with the published sequence for lamprey ER (Thornton, 2001). In contrast, the transcript cloned from second set of primers (ER2 = 341 bp total length) contained a single deletion at position 285, relative to the published sequence. The sequence confirms the analyzed RT-PCR product is from the ER gene; as to the single base-pair difference noted – while presumably changing the reading frame – it is most likely an artifact. A pseudogene would be expected to be much more different over the 341-bps sequenced, thus was not pursued further.
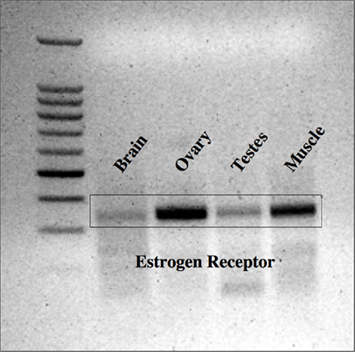
Figure 1. Expression of ER in lamprey tissues. Lamprey ER was most strongly expressed in the ovarian and muscle tissues, but was also expressed in the testis and brain. 100 kb ladder from Promega (Madison, WI, USA).
Evaluation of RNA Probe Yield
The fidelity of DIG-labeled probes was supported through Dot Blot analysis that revealed binding of ER1, ER2, and LIII (GnRH-III) to respective cDNAs (Figure 2). In addition, probes yield was determined to high, as determined by absorption at 260 nm. For 35S-labeled probes, a high amount of label was determined to have been incorporated into all probes, generating a high yield of product that was also consistent between probes (data not shown).
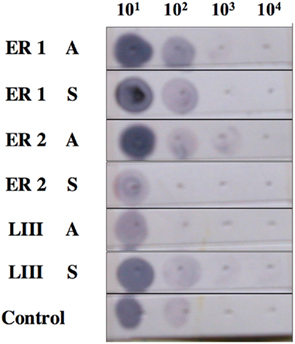
Figure 2. Fidelity of estrogen receptor and GnRH-III (DIG-labeled) probes. Probe fidelity was supported through Dot Blot analysis that revealed binding of ER1, ER2, and LIII (GnRH-III) to respective cDNAs.
In situ Hybridization
A total of 44 lamprey brains were used for DIG-labeled in situ hybridization experiments. DIG-labeled, anti-sense ER probes demonstrated moderate, yet distinct reaction product in specific neuronal nuclei across many regions of the lamprey brain, including the olfactory lobe, hypothalamus, habenular area, and hindbrain. Major brain regions were scored for signal intensity on every slide. Patterns of staining were consistent between all brains examined. Slides were subjected to image analysis performed at Tufts University, and in most cases, indicated that staining on sense slides was significantly less robust than that on anti-sense slides. In addition, similar results were observed in both sagittal (Figure 3) and transverse tissue sections (Figure 4).
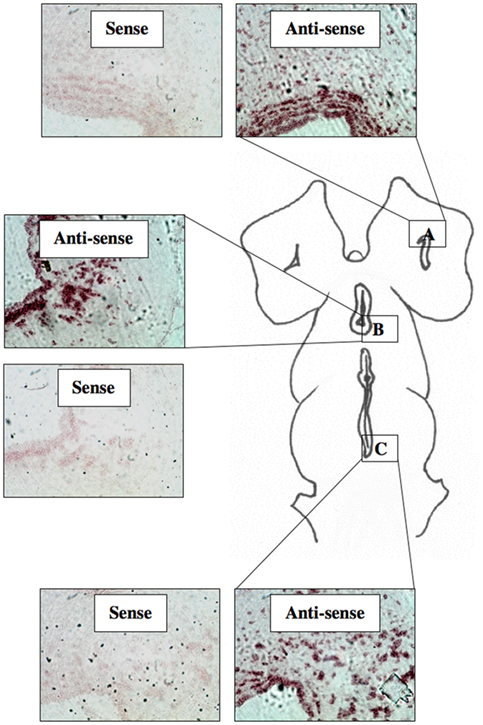
Figure 3. DIG-labeled In situ hybridization for lamprey ER. Light micrographs of sagittal tissue sections showing reaction product using probe ER2 within the olfactory bulb (A), the hypothalamus (B), and the midbrain (C). (200× mag).
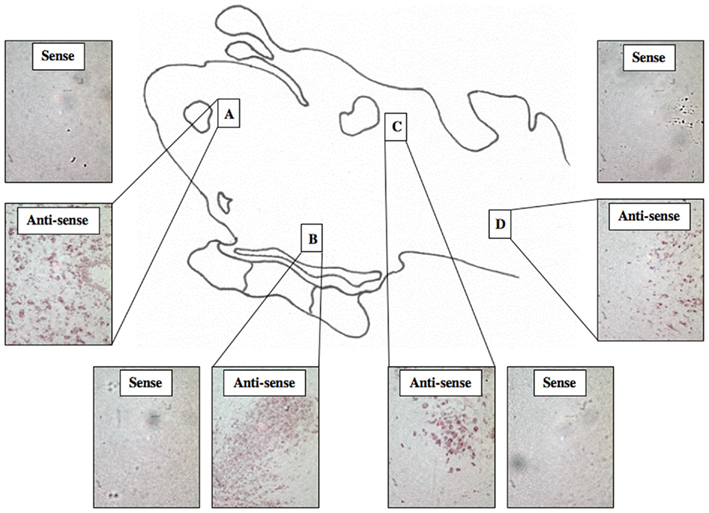
Figure 4. DIG-Labeled In situ hybridization for lamprey ER. Light micrographs of transverse tissue sections showing reaction product using probe ER2 within the olfactory bulb (A), the hypothalamus (B), the midbrain (C), and the hindbrain (D). (200× mag).
For 35S-labeled tissue sections, a total of three lamprey brains were used, and for samples in which the high stringency wash was omitted, results were similar to those seem using DIG-labeled probes. However, while anti-sense slides tended to be more heavily stained than sense slides, a high degree of background staining prevented a detailed analysis (Figure 5). In contrast, for 35S-labeled tissue sections exposed to 10 min of a high stringency wash, no signal was observed (results not shown). The limited range of stringencies attempted (0 and 10 min) using 35S-labeled probes limits the derivative power of these results.
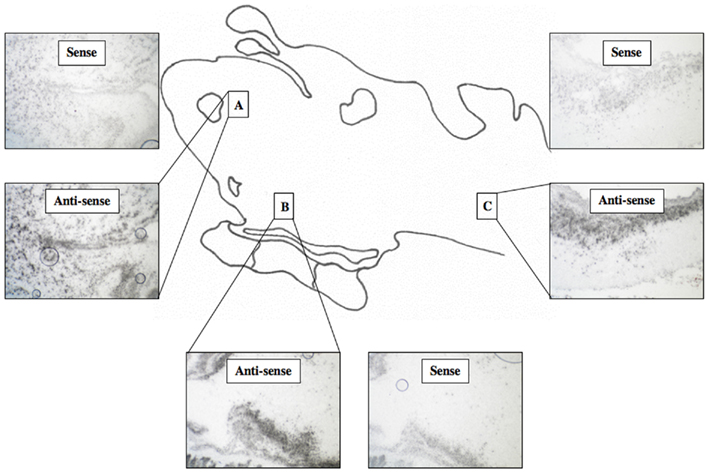
Figure 5. 35 S-labeled In situ hybridization for lamprey ER. Light micrographs of sagittal tissue sections showing reaction product within the olfactory bulb (A), the hypothalamus (B), and the hindbrain (C). (200× mag).
Discussion
In this study, ER was shown to be expressed in the lamprey brain by RT-PCR and DIG-labeled in situ hybridization. Specifically, in situ hybridization indicated that ER is expressed in the olfactory bulb, hypothalamus, habenular area, midbrain, and hindbrain of the lamprey. As stated earlier, in lampreys, there is a general pattern of GnRH distribution in the anterior-preoptic-neurohypophysial tract to the neurohypophysis of adult lampreys (Crim et al., 1979a,b; Nozaki and Kobayashi, 1979; Nozaki and Gorbman, 1984; King et al., 1988; Reed et al., 2002; Kavanaugh et al., 2008). Expression of ER in the hypothalamic area of the brain supports the possibility of interaction between E2 and GnRH, and is consistent with previous evidence that E2 feedback is occurring indirectly or directly via GnRH neurons (Sower, 1987).
The current study revealed much broader expression of ER than that of previous studies in lamprey. Autoradiographic studies using 3H-labeled E2 previously documented binding only within the hypothalamic region and regions immediately adjacent the hypothalamus (Kim et al., 1980, 1981). In the current study, staining was observed in and around the hypothalamus, but also within the olfactory lobe, habenular area, and hindbrain. In vertebrates other than lamprey, expression of ER occurs widely in the brain (Shima et al., 2003), and it may be that the distribution of ER in the lamprey brain more closely parallels that of other vertebrates than was previously thought. The discrepancy between autoradiographic and in situ hybridization studies may have occurred due to the presence of another as yet unknown ER isoform in lamprey or due to non-specific binding. There is increasing evidence suggesting that ERs are capable of activation by means other than E2 (Blaustein, 2004), therefore in situ hybridization may reveal regions of ER expression that function independently of E2, and are otherwise undetectable by autoradiographic methods using 3H-labeled E2.
The pattern of ER expression observed in this study is similar to that of ER expression previously observed in the brains of teleost fish (Menuet et al., 2003; Pellegrini et al., 2005). Interaction between E2 and GnRH has been demonstrated in teleosts (Trudeau et al., 1993), and the absence of ER expression in the GnRH neurons of teleosts suggests that E2–GnRH interaction in this vertebrate class occurs indirectly (Navas et al., 1995). While lamprey have a single identified ER (Thornton, 2001), teleost fish have three types of ER (Hawkins et al., 2000; Menuet et al., 2002). These ERs are differentially expressed in the brain (Hawkins et al., 2000; Pellegrini et al., 2005), and also appear to have distinct functions in the neuroendocrine control of reproduction and behavior (Hawkins et al., 2005). In comparison to teleosts, the possession of only a single ER by lamprey may suggest alternative signaling pathways for E2 in the lamprey brain.
The current study identified ER expression within the same hypothalamic region where GnRH expression occurs, but did not offer any insight into how E2 may interact with GnRH in lamprey. A key question remains as to whether E2 acts directly on GnRH-expressing neurons, or indirectly through a mechanism utilizing GABA or kisspeptin. Root et al. (2005) identified GABA-expressing neurons in close proximity to neurons expressing lamprey GnRH, supporting the possibility of an indirect action of E2 on GnRH. However, the critical evidence needed in order to establish the direct and/or indirect nature of E2–GnRH interaction in lamprey lies in the determination of cellular co-localization between GABA, GnRH, kisspeptin, and ER. Kisspeptin and its receptor is another system that may also be involved in feedback studies. The Kiss1/GpR54 system was discovered and shown to be the central gatekeeper in the regulation of GnRH and puberty in mammals (Seminara et al., 2003; Seminara and Crowley, 2008). In mammals, the kisspeptin system acts in regulating many aspects of reproduction functions including the mediation of steroid feedback (Roa et al., 2009). The identification and function of kisspeptin(s) and respective receptors in lampreys has not yet been elucidated. Future studies will be required to examine the interrelationship between the kisspeptin, GnRH, GABA, and steroid feedback systems and to determine whether there is another ER in lampreys.
Estradiol is considered to be a major reproductive hormone in both male and female lampreys (Sower and Kawauchi, 2010). The role of estradiol in reproduction is supported by the cloning of an estrogen-like receptor in sea lamprey (Thornton, 2001). In sea and Japanese river (Lethenteron camtschaticum) lampreys, estradiol concentrations increased during spermiation (Fukayama and Takahashi, 1985; Sower et al., 1985; Fahien and Sower, 1990) and decreased during ovulation (Sower et al., 1985; Bolduc and Sower, 1992). In the first reported study examining sex steroid profiles in the Pacific lamprey (Entosphenus tridentatus) during overwintering and sexual maturation, estradiol levels were usually higher in males than in females and increases coincided with the development of secondary sex characteristics (Mesa et al., 2010). In another study, there were higher plasma concentrations of estradiol in females compared to males and in both sexes, plasma estradiol significantly increased as the season progressed correlating with a temperature increase that is in general agreement with these earlier studies (Sower and Kawauchi, 2010). In males, higher estradiol concentrations corresponded to males that have mature sperm as shown in maturing lampreys (Fukayama and Takahashi, 1985; Sower et al., 1985; Linville et al., 1987) and are consistent with the presence of an ER in the male testes (Ho et al., 1987). While estradiol is considered to be a major steroid involved in reproductive processes, the precise function(s) of estradiol and its corresponding receptor(s) in both male and female lampreys need to be elucidated. There are still many questions remaining as to the type of steroids that are synthesized and their respective functions (reviewed in Bryan et al., 2008). For example, there is growing evidence that all lampreys produce gonadal steroids that are different from those of other vertebrates by possessing an additional hydroxyl group at the C15 position (Bryan et al., 2006, 2008).
In conclusion, the current study identifies for the first time expression of ER in the lamprey brain using both RT-PCR and in situ hybridization. A broad expression of ER was observed using in situ hybridization, including expression in the olfactory lobe, hypothalamus, habenular area, and hindbrain. Expression of ER in the hypothalamic region supports the possibility of an E2 feedback mechanism the GnRH system, similar to that observed in other vertebrate groups. By demonstrating ER expression in the lamprey brain using in situ hybridization, the current study provides a means for further investigation of possible mechanisms of interaction between E2 and GnRH in lamprey and other critical neurohormone systems.
Conflict of Interest Statement
The authors declare that the research was conducted in the absence of any commercial or financial relationships that could be construed as a potential conflict of interest.
Acknowledgments
This research was supported by NSF IBN-0421923, IOS-0849569 and DBI-0618719. This is scientific contribution number 2459 from the New Hampshire Agriculture Experiment Station. We would like to thank Dr. Beverly Rubin, Tufts Medical School for her advice and use of her Olympus microscope system. We also would like to thank Nathaniel Nucci and Adam Root for their input and assistance.
Footnotes
References
Allen, M. P., Xu, M., Zeng, C., Tobet, S. A., and Wierman, M. E. (2000). Myocyte enhancer factors-2B and -2C are required for adhesion related kinase repression of neuronal gonadotropin releasing hormone gene expression. J. Biol. Chem. 275, 39662–39670.
Blaustein, J. D. (2004). Minireview: neuronal steroid hormone receptors: they’re not just for hormones anymore. Endocrinology 145, 1075–1081.
Bolduc, T. G., and Sower, S. A. (1992). Changes in brain gonadotropin-releasing hormone, plasma estradiol 17- beta, and progesterone during the final reproductive cycle of the female sea lamprey, Petromyzon marinus. J. Exp. Zool. 264, 55–63.
Bryan, M. B., Scott, A. P., and Li, W. (2008). Sex steroids and their receptors in lampreys. Steroids 73, 1–12.
Bryan, M. B., Young, B. A., Close, D. A., Semeyn, J., Robinson, T. C., Bayer, J., and Li, W. (2006). Comparison of synthesis of 15 alpha-hydroxylated steroids in males of four North American lamprey species. Gen. Comp. Endocrinol. 146, 149–156.
Conlon, J. M., Balasubramaniam, A., and Sower, S. A. (1994). Purification of a neuropeptide Y-related peptide from the brain of the sea lamprey and its effect on steroidogenesis. Regul. Pept. 50, 167–175.
Crim, J. W. (1981). “Immunoreactive luteinizing hormone releasing hormone and cerebral spinal fluid-contacting neurons in the preoptic nucleus of lamprey,” in Neurosecretion: Molecules, Cells and Systems, eds. D. S. Farner, and K. Lederis (London: Plenum Publishing Corporation), 441.
Crim, J. W., Urano, A., and Gorbman, A. (1979a). Immunocytochemical studies of luteinizing hormone-releasing hormone in brains of agnathan fishes. I. Comparisons of adult Pacific lamprey (Entosphenus tridentata) and the Pacific hagfish (Eptatretus stouti). Gen. Comp. Endocrinol. 37, 294–305.
Crim, J. W., Urano, A., and Gorbman, A. (1979b). Immunocytochemical studies of luteinizing hormone-releasing hormone in brains of agnathan fishes. II. Patterns of immunoreactivity in larval and maturing Western brook lamprey (Lampetra richardsoni). Gen. Comp. Endocrinol. 38, 290–299.
Fahien, C. M., and Sower, S. A. (1990). Relationship between brain gonadotropin-releasing hormone and final reproductive period of the adult male sea lamprey, Petromyzon marinus. Gen. Comp. Endocrinol. 80, 427–437.
Fukayama, S., and Takahashi, H. (1985). Changes in serum levels of estradiol-17β and testosterone in the Japanes river lamprey, Lampetra japonica, in the course of sexual maturation. Bull. Fac. Fish. Hokkaido Univ. 36, 163–169.
Gorbman, A. (1965). Vascular relations between the neurohypophysis and adenohypophysis of cyclostomes and the problem of evolution of hypothalamic neuroendrocrine control. Arch. Anat. Microsc. Morphol. Exp. 54, 163–194.
Hall, J., Aquilina-Beck, A., Joseph, N., Macdonald, C., Decatur, W. A., Kavanaugh, S., Freamat, M., and Sower, S. A. (2011). “Expression and functional studies of two novel type III Gnrh receptors (2 and 3) in the sea lamprey, a basal vertebrate,” in Frontiers in Endocrinology Conference Abstract: NASCE 2011: The inaugural meeting of the North American Society for Comparative Endocrinology, Ann Arbor, MI. doi:10.3389/conf.fendo.2011.04.00138
Hawkins, M. B., Godwin, J., Crews, D., and Thomas, P. (2005). The distributions of the duplicate oestrogen receptors ER-beta a and ER-beta b in the forebrain of the Atlantic croaker (Micropogonias undulatus): evidence for subfunctionalization after gene duplication. Proc. Biol. Sci. 272, 633–641.
Hawkins, M. B., Thornton, J. W., Crews, D., Skipper, J. K., Dotte, A., and Thomas, P. (2000). Identification of a third distinct estrogen receptor and reclassification of estrogen receptors in teleosts. Proc. Natl. Acad. Sci. U.S.A. 97, 10751–10756.
Ho, S. M., Press, D., Liang, L. C., and Sower, S. (1987). Identification of an estrogen receptor in the testis of the sea lamprey, Petromyzon marinus. Gen. Comp. Endocrinol. 67, 119–125.
Hrabovszky, E., Kallo, I., Steinhauser, A., Merchenthaler, I., Coen, C. W., Petersen, S. L., and Liposits, Z. (2004). Estrogen receptor-beta in oxytocin and vasopressin neurons of the rat and human hypothalamus: immunocytochemical and in situ hybridization studies. J. Comp. Neurol. 473, 315–333.
Kah, O., and Dufour, S. (2010). “Conserved and divergent features of reproductive neuroendocrinology in teleost fishes,” in Hormones and Reproduction of Vertebrates Fishes, eds D. O. Norris, and K. H. Lopez (Boston: Academic Press), 15–42.
Kavanaugh, S. I., Nozaki, M., and Sower, S. A. (2008). Origins of Gonadotropin-Releasing Hormone (GnRH) in vertebrates: identification of a novel GnRH in a basal vertebrate, the sea lamprey. Endocrinology 149, 3860–3869.
Kim, Y. S., Stumpf, W. E., Reid, F. A., Sar, M., and Selzer, M. E. (1980). Estrogen target cells in the forebrain of river lamprey, Ichthyomyzon unicuspis. J. Comp. Neurol. 191, 607–613.
Kim, Y. S., Stumpf, W. E., Sar, M., Reid, F. A., Selzer, M. E., and Epple, A. W. (1981). Autoradiographic studies of estrogen target cells in the forebrain of larval lamprey, Petromyzon marinus. Brain Res. 210, 53–60.
King, J. C., Sower, S. A., and Anthony, E. L. (1988). Neuronal systems immunoreactive with antiserum to lamprey gonadotropin- releasing hormone in the brain of Petromyzon marinus. Cell Tissue Res. 253, 1–8.
Linville, J. E., Hanson, L. H., and Sower, S. A. (1987). Endocrine events associated with spawning behavior in the sea lamprey (Petromyzon marinus). Horm. Behav. 21, 105–107.
Menuet, A., Anglade, I., Le Guevel, R., Pellegrini, E., Pakdel, F., and Kah, O. (2003). Distribution of aromatase mRNA and protein in the brain and pituitary of female rainbow trout: comparison with estrogen receptor alpha. J. Comp. Neurol. 462, 180–193.
Menuet, A., Pellegrini, E., Anglade, I., Blaise, O., Laudet, V., Kah, O., and Pakdel, F. (2002). Molecular characterization of three estrogen receptor forms in zebrafish: binding characteristics, transactivation properties, and tissue distributions. Biol. Reprod. 66, 1881–1892.
Mesa, M., Bayer, J., Bryan, M., and Sower, S. (2010). Annual sex steroid and other physiological profiles of Pacific lampreys (Entosphenus tridentatus). Comp. Biochem. Physiol. A Mol. Integr. Physiol. 155, 56–63.
Navas, J. M., Anglade, I., Bailhache, T., Pakdel, F., Breton, B., Jego, P., and Kah, O. (1995). Do gonadotrophin-releasing hormone neurons express estrogen receptors in the rainbow trout? A double immunohistochemical study. J. Comp. Neurol. 363, 461–474.
Nozaki, M., and Gorbman, A. (1984). Distribution of immunoreactive sites for several components of pro- opiocortin in the pituitary and brain of adult lampreys, Petromyzon marinus and Entosphenus tridentatus. Gen. Comp. Endocrinol. 53, 335–352.
Nozaki, M., Gorbman, A., and Sower, S. A. (1994). Diffusion between the neurohypophysis and the adenohypophysis of lampreys, Petromyzon marinus. Gen. Comp. Endocrinol. 96, 385–391.
Nozaki, M., and Kobayashi, H. (1979). Distribution of LHRH-like substance in the vertebrate brain as revealed by immunohistochemistry. Arch. Histol. Jpn. 42, 201–219.
Pellegrini, E., Menuet, A., Lethimonier, C., Adrio, F., Gueguen, M. M., Tascon, C., Anglade, I., Pakdel, F., and Kah, O. (2005). Relationships between aromatase and estrogen receptors in the brain of teleost fish. Gen. Comp. Endocrinol. 142, 60–66.
Petersen, S. L., Ottem, E. N., and Carpenter, C. D. (2003). Direct and indirect regulation of gonadotropin-releasing hormone neurons by estradiol. Biol. Reprod. 69, 1771–1778.
Reed, K. L., Macintyre, J. K., Tobet, S. A., Trudeau, V. L., Maceachern, L., Rubin, B. S., and Sower, S. A. (2002). The spatial relationship of gamma-aminobutyric acid (GABA) neurons and gonadotropin-releasing hormone (GnRH) neurons in larval and adult sea lamprey, Petromyzon marinus. Brain Behav. Evol. 60, 1–12.
Roa, J., Castellano, J. M., Navarro, V. M., Handelsman, D. J., Pinilla, L., and Tena-Sempere, M. (2009). Kisspeptins and the control of gonadotropin secretion in male and female rodents. Peptides 30, 57–66.
Root, A. R., Nucci, N. V., Sanford, J. D., Rubin, B. S., Trudeau, V. L., and Sower, S. A. (2005). In situ characterization of gonadotropin-releasing hormone-I, -III, and glutamic acid decarboxylase expression in the brain of the sea lamprey, Petromyzon marinus. Brain Behav. Evol. 65, 60–70.
Root, A. R., Sanford, J. D., Kavanaugh, S. I., and Sower, S. A. (2004). In vitro and in vivo effects of GABA, muscimol, and bicuculline on lamprey GnRH concentration in the brain of the sea lamprey (Petromyzon marinus). Comp. Biochem. Physiol. A Mol. Integr. Physiol. 138, 493–501.
Rubin, B. S., Lee, C. E., Ohtomo, M., and King, J. C. (1997). Luteinizing hormone-releasing hormone gene expression differs in young and middle-aged females on the day of a steroid-induced LH surge. Brain Res. 770, 267–276.
Seminara, S., and Crowley, W. F. Jr. (2008). Kisspeptin and GPR54: discovery of a novel pathway in reproduction. J. Neuroendocrinol. 20, 727–731.
Seminara, S., Messager, S., Chatzidaki, E., Thresher, R., Acierno, J. Jr., Shagoury, J., Bo-Abbas, Y., Kuohung, W., Schwinof, K., Hendrick, A., Zahn, D., Dixon, J., Kaiser, U., Slaugenhaupt, S., Gusella, J., O’rahilly, S., Carlton, M., Crowley, W. Jr., Aparicio, S., and Colledge, W. (2003). The GPR54 gene as a regulator of puberty. N. Engl. J. Med. 349, 16114–16127.
Shima, N., Yamaguchi, Y., and Yuri, K. (2003). Distribution of estrogen receptor beta mRNA-containing cells in ovariectomized and estrogen-treated female rat brain. Anat. Sci. Int. 78, 85–97.
Sower, S. A. (1987). “Biological action of lamprey gonadotropin-releasing hormone in lampreys,” in Proceedings of the Third International Symposium on Reproduction and Physiology of Fish, St. John’s Newfoundland, NL, 40–41.
Sower, S. A., and Kawauchi, H. (2010). “Reproduction in agnathan fishes: lampreys and hagfishes,” in Hormones and Reproduction of Vertebrates, Vol. 1, Chapt.10, eds D. O. Norris, and K. H. Lopez (Academic Press).
Sower, S. A. (1997). ”Evolution of GnRH in fish of ancient origins,” in GnRH Neurons: Gene to Behavior, ed. I. S. P. A. Y. Sakuma (Tokya: Brian Shuppan Publishers), 486.
Sower, S. A., Freamat, M., and Kavanaugh, S. (2009). “Insight from lamprey genomics: brain and pituitary reproductive hormones of lampreys,” in Transactions of the American Fisheries Society -Biology, Management, and Conservation of Lampreys in North America, eds L. Brown, S. Chase, M. Mesa, R. Beamish, and P. Moyle (Bethesda, MD: American Fisheries Society), 57–70.
Sower, S. A., Plisetskaya, E., and Gorbman, A. (1985). Changes in plasma steroid and thyroid hormones and insulin during final maturation and spawning of the sea lamprey, Petromyzon marinus. Gen. Comp. Endocrinol. 58, 259–269.
Thornton, J. W. (2001). Evolution of vertebrate steroid receptors from an ancestral estrogen receptor by ligand exploitation and serial genome expansions. Proc. Natl. Acad. Sci. U.S.A. 98, 5671–5676.
Keywords: estrogen receptor, lamprey, GnRH, in situ hybridization, lamprey hypothalamus, estradiol, basal vertebrate, agnathan
Citation: Sower SA and Baron MP (2011) The interrelationship of estrogen receptor and GnRH in a basal vertebrate, the sea lamprey. Front. Endocrin. 2:58. doi: 10.3389/fendo.2011.00058
Received: 29 August 2011; Paper pending published: 21 September 2011;
Accepted: 04 October 2011; Published online: 28 October 2011.
Edited by:
Henryk Urbanski, Oregon National Primate Research Center, USAReviewed by:
Karine Rizzoti, National Institute for Medical Research, UKFrank Siewerdt, University of Maryland at College Park, USA
Copyright: © 2011 Sower and Baron. This is an open-access article subject to a non-exclusive license between the authors and Frontiers Media SA, which permits use, distribution and reproduction in other forums, provided the original authors and source are credited and other Frontiers conditions are complied with.
*Correspondence: Stacia A. Sower, Department of Molecular, Cellular and Biomedical Sciences, Center for Molecular and Comparative Endocrinology, University of New Hampshire, 46 College Road, Durham, NH 03824-3544, USA. e-mail: sasower@unh.edu