- 1Key Laboratory of Intraplate Volcanoes and Earthquakes (China University of Geosciences, Beijing), Ministry of Education, Beijing, China
- 2School of Geophysics and Information Technology, China University of Geosciences (Beijing), Beijing, China
- 3State Key Laboratory of Geological Processes and Mineral Resources, China University of Geosciences, Beijing, China
Underground space in Xuzhou City, North China, has become a favorable site for continuous geomagnetic observation to reveal geomagnetic-field variations and to monitor seismic activity due to its distinct advantage of an “ultra-quiet” and “ultra-clean” environment with low electromagnetic interference. In order to evaluate the underground electromagnetic environment at the Longdong coal mine area (LCMA) in Xuzhou City and establish an underground geomagnetic observatory, we conducted a pilot experiment of continuous geomagnetic observations synchronously underground and on the ground during May 2023. In this experiment, two close-space fluxgate magnetometers were deployed in the underground roadway of the LCMA to observe the variations of geomagnetic three components simultaneously, and a proton magnetometer was installed on its ground to observe the variation of geomagnetic total field. Furthermore, we analyzed the features of the wavelet spectrum of these observed data, variation curves of the geomagnetic field, and coupling of the geomagnetic-field variations between underground and ground. In addition, we investigated the characteristics of different frequency bands and horizontal gradient of the underground geomagnetic variation. Our achievements are as follows: 1) the underground geomagnetic environment has the advantage of being stable and clean with a low level of background noise and thus is suitable for high-precision, long-term, and continuous observation of the geomagnetic field; 2) the variation of the geomagnetic field underground is well coupled with that on the ground, with minor differences primarily attributed to an induced magnetic field generated in the surrounding rocks by the external magnetic-field variation; 3) underground geomagnetic observation can clearly record rich time-varying information of the geomagnetic field including calm variation, magnetic storms, geomagnetic pulsations and so on. Moreover, the geomagnetic gradient field contains higher-resolution time-varying information than the conventional geomagnetic field. Our pilot experiment suggests favorable underground sites with low background noise at the LCMA and provides valuable references for understanding the spatiotemporal variation characteristics of geomagnetic field. Based on the findings of our pilot experiment, an underground geomagnetic observatory and calibration station will be established at the LCMA, enabling high-precision, continuous geomagnetic and electromagnetic observation experiments in the future. These efforts will provide crucial constraints for the study of the variation of the geomagnetic-field variation, as well as seismic activity monitoring and disaster early warning related to the Tan-Lu fault zone.
1 Introduction
The geomagnetic field represents one fundamental physical field for the habitable Earth, which consists of the Earth’s intrinsic magnetic field, lithospheric magnetic anomaly, and variability of the magnetic field. The geomagnetic field varies in both space and time, and its spatiotemporal distribution serves as crucial support for understanding the origin and variability of the geomagnetic field, exploring resources and energy, monitoring earthquakes and global environmental changes, as well as conducting geomagnetic navigation and other applications. The temporal variation of the geomagnetic field primarily originates from external sources and is characterized by quiet and disturbed variations. However, its magnitude is typically minimal, far less than that of spatial variation. Therefore, revealing the temporal variation of the geomagnetic field necessitates not only high-precision magnetometers for continuous observation but also a low-electromagnetic-disturbance environment to ensure the accuracy of such continuous observation (Marfaing et al., 2009).
Earthquakes, which result from the rapid release of energy in the Earth’s crust, cause serious damage to human daily life and safety. During the processes of earthquake preparation and occurrence, minor rupture and alteration of the lithospheric structure and tectonics induce effects such as piezomagnetic effect and thermomagnetic effect (Johnston et al., 2006), which change the magnetism of the underground medium, resulting in temporal variations of the lithosphere magnetic field around the epicentral area. It is of great scientific significance to identify the characteristics and patterns of pre- and post-seismic geomagnetic field variations and then to guide the detection of geomagnetic precursor anomalies for short- and medium-term earthquake forecasting. Geomagnetic precursor anomalies, essentially non-random deviations from the normal evolution of the geomagnetic field, are usually weak signals that require long-term and continuous observation with a high-precision magnetometer in a low-noise electromagnetic environment (Gao et al., 2020).
However, the electromagnetic environment on the ground has deteriorated dramatically due to economic and social development, resulting in a decline in the quality and reliability of geomagnetic temporal observation data, which can hardly meet the demands of relevant scientific research and earthquake monitoring for continuous geomagnetic-field observation. Compared with the ground environment, the underground spaces such as caves, tunnels, and deep wells offer “ultra-clean” conditions with minimal electromagnetic interference, making them ideal for geomagnetic observations (Wang et al., 2022). Indeed, the use of underground laboratories for multi-physical field observations and research began as early as the 1950s and has rapidly progressed since the 1990s in countries such as the United States, France, Germany, Japan, China, and others. For instance, the San Andreas Fault Observatory at Depth (SAFOD) in the United States (Chavarria et al., 2003; Hickman et al., 2004), the Laboratoire Souterrain à Bas Bruit (LSBB) in France (Waysand et al., 2009), the Kontinentales Tiefborh program der Bundesrepublik Deutschland (KTB) in Germany (Wang et al., 1999), the Izu-Oshima Deep Borehole Observatory in Japan (Yamauchi et al., 2005), along with the Donghai Deep Borehole Observatory in China (Xu and Zhao, 2009) have confirmed that deep underground environment presents very low background noise (Waysand et al., 2009; Rosat et al., 2018). Notably, the Superconducting QUantum Interference Device (SQUID) magnetometer at the LSBB in France has clearly observed the Schumann resonance phenomenon and detected electromagnetic signals generated by changes in groundwater flow induced by seismic wave propagation (Gaffet et al., 2003; Henry et al., 2014; Henry et al., 2016). From 2020 to 2022, we carried out a comprehensive geophysical observation experiment (including methods such as seismology, gravity, geomagnetism, and electromagnetics) in one underground laboratory of Huainan City in the southern margin of North China, which also confirmed the stable and clean advantages of the underground electromagnetic environment (Wang et al., 2023; Guo et al., 2024; Xie et al., 2024).
The Tan-Lu fault zone (TLF), a large NNE-oriented intra-plate fault zone in Eastern China (Figure 1), extends northward from the southern margin of North China to Northeastern China. It formed during the Indosinian orogeny by the collision of the North China and South China blocks and has experienced strike-slip, extensional, compressional, and other tectonic deformation, as well as magmatic activity since the Mesozoic (Deng et al., 2003). However, the TLF is also a significant seismically active region where several strong earthquakes exceeding magnitude 6 have occurred historically, such as the 1,668 Tancheng M8.5 earthquake, the 1969 Bohai Sea M7.4 earthquake, the 1975 Haicheng M7.3 earthquake, and the 1976 Tangshan M7.8 earthquake. These events have caused the loss of human life and property along the TLF, highlighting the urgent requirement for enhancing regional earthquake monitoring. Xuzhou City, located on the western margin of the central section of the TLF, has a complex geological structure. Besides the TLF on the east, major faults include in this area the NNE-oriented Liaokao fault zone on the west, the NWW-oriented Fengpei fault zone on the north, the NWW-oriented Fuliji fault zone on the south, and the central NWW-oriented Feihuanghe fault. The rock formations in this area mainly consist of the upper Quaternary loose sedimentary layer, Paleozoic carbonate rocks, and the lower Upper Proterozoic basement, etc. Xuzhou City is a key industrial city in central North China, with numerous underground coal mine areas scattered throughout the city. However, some coal mine areas, such as the LCMA in the north of Xuzhou City, have ceased all underground mining activities due to resource depletion and other reasons. In view of the severe electromagnetic interference caused by the ground industry in Xuzhou City, it is essential to fully utilize the underground space of the now-closed coal mine areas to identify the favorable sites with a low-noise environment, thereby establishing an underground geophysical laboratory. Then, comprehensive geophysical observations including the geomagnetic field can be carried out in the laboratory, which holds scientific importance for monitoring seismic activity and assessing seismic hazards around the TLF.
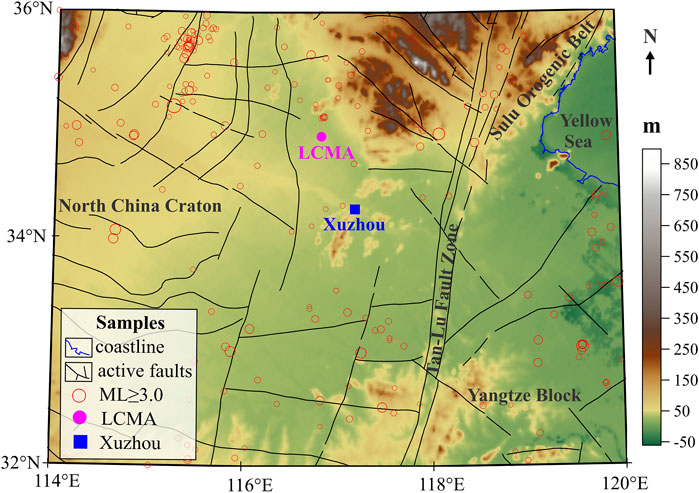
Figure 1. Tectonic map of the study area. LCMA denotes the Longdong coal mine area, and the hollow circles represent the distribution of epicenters since 1970.
We conducted a pilot experiment of continuous geomagnetic-field observation in the underground roadway and on the ground at the LCMA of Xuzhou City, using advanced fluxgate and proton magnetometers from 25 May 2023 to 29 May 2023. In this paper, we evaluated the electromagnetic environment in the underground roadway of the LCMA based on the pilot experiment data through wavelet spectral analysis and comparison of the geomagnetic variation curves. We further identified the favorable low-noise sites for establishing high-quality underground geomagnetic observation laboratories and calibration stations, as well as for conducting high-precision, continuous geomagnetic and electromagnetic observations in the future. Additionally, we analyzed the coupling of geomagnetic-field variations between underground and ground, as well as the characteristics of different frequency bands and horizontal gradient of the underground geomagnetic variation. These results will provide insights into the spatiotemporal variation characteristics of the geomagnetic field, advancing our understanding of Earth sciences and our rational utilization of Earth resources, and promoting earthquake monitoring and disaster early warning.
2 Continuous observations of the geomagnetic field in the LCMA
2.1 Instruments for continuous geomagnetic-field observation
This pilot experiment of continuous geomagnetic observation employed two Fluxgate Magnetometer 3-Dimensional (FGM3D) to observe the geomagnetic three-component variations and a GSM-19T proton-precession magnetometer to measure the geomagnetic total-field variation. The FGM3D, a three-axis fluxgate magnetometer, precisely measures the magnitude and variations of the three geomagnetic components, with a sensitivity of 0.1 V/μT and a resolution of up to 150 pT. The GSM-19T proton-precession magnetometer accurately measures the magnitude and variations of the geomagnetic total field, with a sensitivity of less than 0.1nT, a resolution of up to 0.01nT, and an absolute accuracy of ±0.2 nT. Both magnetometers feature low offset errors, low internal noise, and high stability, making them suitable for the long-term, continuous observation of geomagnetic-field variation.
2.2 Layout for continuous geomagnetic-field observation underground and on the ground
Observation stations A and B were set up in the underground roadway (at an elevation of −256 m) of the LCMA in Xuzhou City. An FGM3D fluxgate magnetometer was deployed at each station to continuously observe the geomagnetic field, recording the X-component (north), Y-component (east) and Z-component (vertical) of the geomagnetic field, with a sampling rate of 62.5 Hz. The subsurface geomagnetic signals were collected from 25 May 2023 to 29 May 2023. Stations A and B are located 50 m apart in a straight line. Simultaneously, a GSM-19T proton-precession magnetometer with a sampling rate of 0.2 Hz was installed on the ground of the LCMA with an elevation of +32 m, designated as ground station C, to observe the geomagnetic total field. Station C was selected for comparative analysis of geomagnetic field data between the underground and ground stations. To ensure consistency with the geomagnetic data recorded at ground station C, the geomagnetic three-component data collected at underground stations A and B were resampled at 0.2 Hz. It should be noted that the dates and time mentioned in this paper are reported in Beijing time.
3 Results and discussions
3.1 The superiority of the underground electromagnetic environment
A superior geomagnetic environment is essential for continuous geomagnetic observation, enabling the detection of weak signals such as the geomagnetic precursor anomalies. Time-frequency analysis is one fundamental tool for geomagnetic field signal analysis, which benefits in analyzing signal components such as magnetic storms, geomagnetic pulsations, precursor anomalies, and background noise. In order to evaluate the underground geomagnetic environment, we conducted wavelet spectrum analysis on our geomagnetic observation data of the pilot experiment during the 72 h from 26 May 2023 to 28 May 2023. All our data analyses in the following sections will be based on this time frame. Figure 2 shows the wavelet spectrum maps of the geomagnetic three-component (X, Y, Z) data and their derived total-field data from underground stations A and B, as well as the geomagnetic total-field data from ground station C.
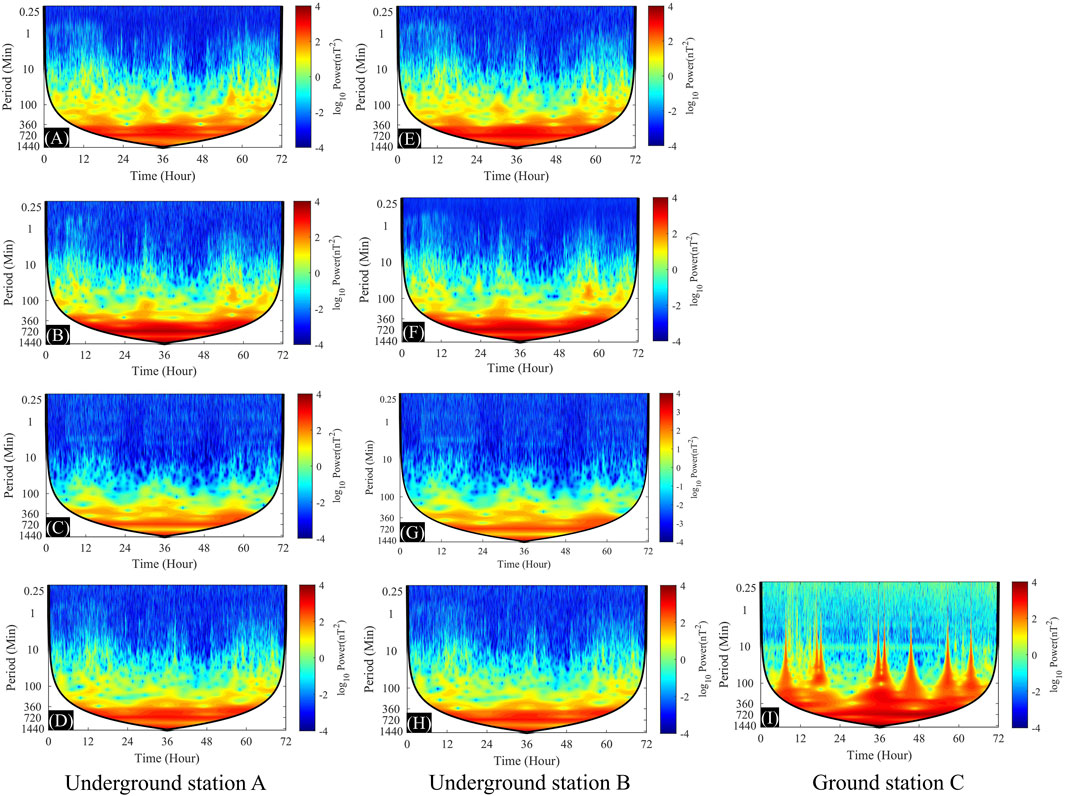
Figure 2. Wavelet spectra maps of the geomagnetic three-component data and their derived total-field data at underground station A (A–D) and underground station B (E–H), as well as that of the geomagnetic total-field data from ground station C (I).
In the high-frequency band with periods less than 60 min, the wavelet spectra of the geomagnetic three-component and total-field data at underground station A (Figures 2A–D) exhibit nearly identical characteristics with those at underground station B (Figures 2E–H). They demonstrate low high-frequency background noise and rich wavelet spectral details, which are conducive to capturing subtle variations in the geomagnetic field. In contrast, the wavelet spectrum of the geomagnetic total field at ground station C (Figure 2I) shows relatively high levels of background noise and distinct stripe-like interference during specific time segments, such as 7–18 h, 35–45 h, and 56–65 h. The 10-min frequency band in Figure 2I also reflects that the geomagnetic field record on the ground is severely affected by electromagnetic interference from industrial activities, making it difficult to reveal the detailed variation of the geomagnetic field.
In the mid- and low-frequency bands with periods greater than 60 min, the wavelet spectra of the geomagnetic three-component and total-field data from the two underground stations are almost the same and rich in features, indicating relatively low background noise levels within this frequency range underground. This favorable condition allows for a detailed depiction of the time-varying characteristics of the geomagnetic field across these frequency bands. Nevertheless, the ground station still suffers from severe interference in the mid- and low-frequency bands, hindering the objective representation of geomagnetic variation characteristics. Therefore, wavelet spectral analysis demonstrates that the underground geomagnetic environment at the LCMA effectively filters electromagnetic interference from industrial activities on the ground. It offers low background noise, stability, and superior conditions, which is suitable for long-term, continuous, and high-precision geomagnetic observation. In contrast, the ground observation environment is subject to electromagnetic interference from industrial activities, which renders it unsuitable for long-term and continuous geomagnetic observation.
Furthermore, we analyzed the geomagnetic environment underground from the geomagnetic variation curves recorded at both underground and ground stations. Figure 3 presents the variation curves of the geomagnetic three components and the total field at each station, with the mean value removed (this kind of demeaning was done in all the following data analyses). It is evident that the geomagnetic total field at ground station C is severely disturbed by electromagnetic interference from industrial activities such as high-voltage electricity, characterized by multiple step-like jumps of varying amplitudes and durations, along with persistent high-frequency noise. Previous studies (Shen et al., 2005; Chen et al., 2017) have similarly identified interference features of both step-like and spiked disturbances with an amplitude of nearly 30 nT in geomagnetic variation caused by high-voltage electricity. In contrast, the geomagnetic three components and total field at underground stations A and B show no step-like jumps and only weak high-frequency noise. The slight differences observed in geomagnetic three-component variations between the two underground stations are primarily due to the tiny bias of orientation for the two magnetometers in the underground roadway. However, the variations of the geomagnetic total field at the two underground stations are consistent with each other and are unaffected by the tiny orientation bias. The underground geomagnetic results reflect that the underground environment effectively filters the electromagnetic interference from industrial activities on the ground. The two underground stations reveal rich time-varying information of the geomagnetic field, with highly consistent observations that exhibit quieter nighttime variation and obvious daytime variation.
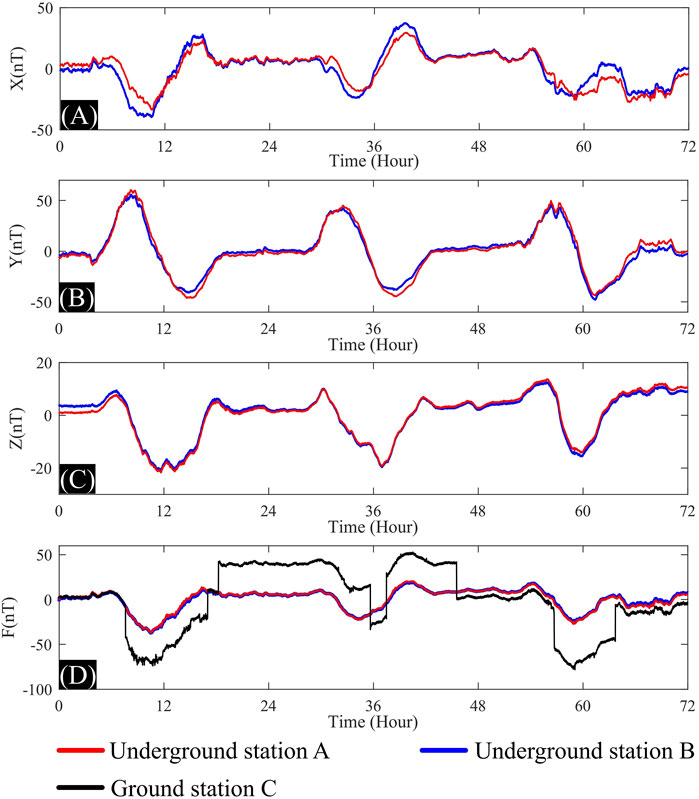
Figure 3. The variation of the geomagnetic X component (A), Y component (B), Z component (C), and geomagnetic total field (D) at different stations.
The discrepancy between the geomagnetic total-field variation curves of underground stations A and B is minimal. Their horizontal gradient (Fh) of the geomagnetic total field was calculated based on the deviation in the total field variations and the distance between the two underground stations as illustrated in Figure 4. It displays gradual variation with modest amplitudes, ranging within ±0.05 nT/m. Therefore, the geomagnetic environment at underground stations A and B is stable and clean, exhibiting minimal fluctuations in the gradient field. These attributes render this site highly suitable for establishing the underground geomagnetic observation laboratory and calibration station. Such facilities offer an advantageous platform for high-precision, long-term, and continuous observation of geomagnetic and electromagnetic fields, as well as for monitoring seismic activity in the future.
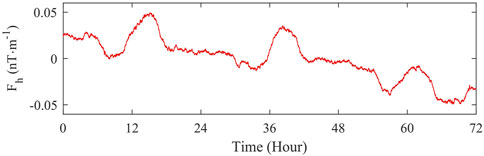
Figure 4. The horizontal gradient variation of the geomagnetic total field at underground station A.
3.2 The coupling of geomagnetic field variations between the underground and ground sites
The geomagnetic total-field data from underground stations A and B ground station C at the LCMA were selected to evaluate the coupling of geomagnetic-field variations between underground and ground. We migrated all step-like jump values in the geomagnetic total-field data observed at ground station C to the correct levels, making its geomagnetic total field more continuous. Figure 5 presents the geomagnetic total-field variation curves (A) and their smoothed deviation curves (B) between underground stations A and B and ground station C (after migration). It is evident that the variation characteristics and trend of the geomagnetic total field at both underground stations A and B are quite similar to those of ground station C, with small deviation values and amplitudes not exceeding ±3 nT. The primary differences between underground and ground stations lie in the high-frequency noise interference observed in the geomagnetic total-field data from the ground station. This suggests that the underground geomagnetic variation is coupled well with that of the ground, which is consistent with the spatial distribution of the geomagnetic variation.
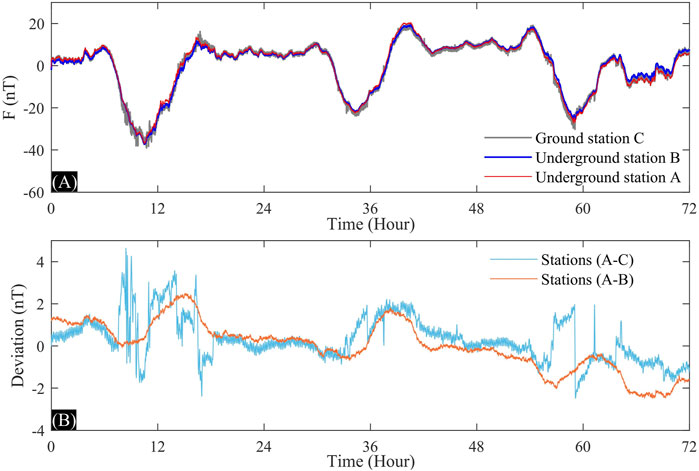
Figure 5. The geomagnetic total-field variation (A) at underground stations A and B and the migrated geomagnetic total-field variation (A) at ground station C along with their smoothed deviations (B).
As shown in Figure 5B, the smoothed deviation of geomagnetic total-field variations between underground stations A and B is small and smooth and is positively correlated to the geomagnetic total-field variations (Figure 5A), implying its close relationship to the Earth’s external magnetic field along with weak noise and interference. In contrast, the smoothed deviation of geomagnetic total-field variations between underground station A and ground station C presents more noise and undulations due to the industrial electromagnetic interference on the ground. However, it is still correlated to the geomagnetic total-field variations to some degree, indicating its relationship to the Earth’s external magnetic field. Gong et al. (2016), Chen et al. (2017), and Guo et al. (2024) presented similar findings in their observations and inferred that these deviations are caused by magnetic field variation induced by the surrounding rocks of the station due to the variation of the Earth’s external magnetic field.
3.3 The richness of time-varying characteristic of the underground geomagnetic field
We analyzed the time-varying characteristics of the geomagnetic total field at underground station A by low-pass, band-pass and high-pass filtering, as shown in Figures 6A–C. In the low-frequency band with a period of greater than 360 min (Figure 6A), the geomagnetic total field at station A exhibits a gentle variation with amplitudes not exceeding ±30 nT, displaying an approximate daily periodicity. Since no significant magnetic storms occurred during the observed period, the low-frequency variation of the geomagnetic field at station A primarily reflects diurnal and semidiurnal variations. In the mid-frequency band with a period ranging from 60 to 360 min (Figure 6B), the geomagnetic total field at station A features rich variation with amplitudes not exceeding ±5 nT, yet shows non-periodic behavior. In particular, there are frequent fluctuations from 0 to 24 h and from 48 to 72 h, while variation from 24 to 48 h is relatively smooth and of a smaller amplitude. Upon inquiry with the International Geomagnetic Observatory Network, the variation of the external magnetic field on 27 May 2023 (24–48 h) was significantly weaker than that on May 26 (0–24 h) and May 28 (48–72 h). Thus, it is inferred that the mid-frequency variation of the geomagnetic field at station A mainly reflects magnetic field disturbances caused by the external magnetic field of medium periods. In the high-frequency band with a period of less than 60 min (Figure 6C), the geomagnetic total field at station A shows the richest variation with amplitudes not exceeding ±2 nT. Sharp fluctuations are observed during certain time segments, including 0–24 h and 48–72 h, while variation between 24 and 48 h is relatively modest. These features suggest that the high-frequency variation of the geomagnetic field at station A is primarily attributed to magnetic disturbances and high-frequency geomagnetic pulses induced by the variation of the external magnetic field in a short period. Notably, the attenuation of high-frequency magnetic activity on May 27 (24–48 h) was attributed to a weakening of the short-period variation of the external magnetic field.
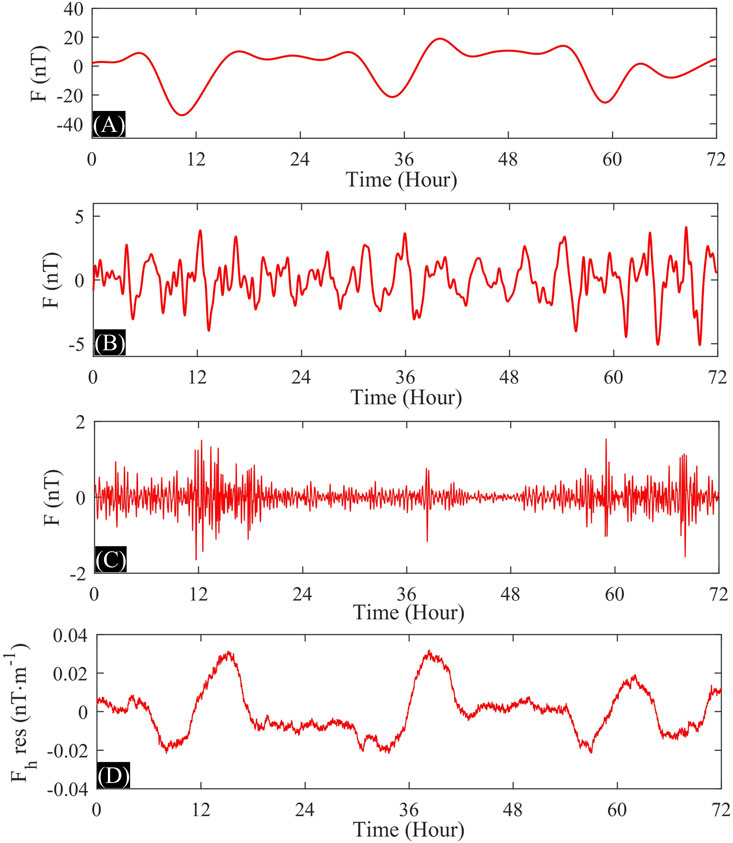
Figure 6. Low-pass filtering (A), band-pass filtering (B), and high-pass filtering (C) for the geomagnetic total field at underground station A. (D) displays the residual field separated from the geomagnetic total-field horizontal-gradient variation.
We further applied the second-order polynomial fitting method to separate the horizontal gradient variation of the geomagnetic total field at underground station A (Figure 4) to extract the residual field of the gradient variation, as shown in Figure 6D. It’s clear that the residual field of the horizontal-gradient variation presents more detailed information than the original geomagnetic total-field variation (Figure 5A). The residual field appears an approximately periodic and rich variation, with an amplitude not exceeding ±0.03 nT/m. It shows relatively large variation during the daytime and calmness at night, indicating that the variation of the geomagnetic field is primarily driven by the variation of the external magnetic field. Additionally, no seismic-related geomagnetic precursor anomaly is observed in the residual field of Figure 6D, as no earthquakes occurred in a broad area where Xuzhou City is located from May 26 to May 28.
In summary, the continuous geomagnetic observation at the underground station can clearly record the effective and rich variation of the geomagnetic field from low to high frequencies, providing support for studying the spatiotemporal variation of the geomagnetic field and monitoring seismic activity and environmental variation. Since the conventional fluxgate and proton magnetometers used in this pilot experiment have limited frequency bands with an effective sampling rate of less than 1 s or even 5 s, the high-frequency geomagnetic signals above 1 Hz failed to be revealed. Thus, considering the superior electromagnetic environment of the underground space, future experiments by higher-resolution, higher-precision, and broader-bandwidth instruments such as SQUID superconducting magnetometers or magnetotelluric instruments are strongly recommended (Nishijima et al., 2013).
4 Conclusion
Based on the pilot experiment of continuous geomagnetic-field variation synchronously in the underground roadway and on the ground of the LCMA in Xuzhou city, along with the subsequent data analyses, we obtained the following key achievements:
First, the electromagnetic environment on the ground of the LCMA is severely disturbed by industrial electromagnetic activities, whereas the underground environment effectively filters such interference. Hence, the underground space exhibits the great advantages of low background noise, stability, and superiority, and is suitable for high-precision, long-term, and continuous geomagnetic observation. Second, the variation of the geomagnetic field underground is well coupled with the denoised geomagnetic-field variation on the ground. The deviation between the two is positively correlated with the variation of the external magnetic field, suggesting that the deviation primarily originates from the induced magnetic field in the surrounding rocks caused by the external magnetic variation. Third, continuous observation of the underground geomagnetic field can effectively record rich time-varying information of the geomagnetic field from low to high frequencies. The geomagnetic horizontal-gradient variation underground exhibits quasi-periodic variation daily and other significant time-varying details. Therefore, our pilot experiment confirms the advantage of the low-noise environment in the underground space of the LCMA for geomagnetic observation and suggests the favorable site for establishing an underground geomagnetic observatory and conducting long-term, high-precision geomagnetic observation in the future. Our pilot experiment and achievements provide references for studying the spatiotemporal evolution of the geomagnetic field, monitoring seismic activity, and assessing environmental changes around the TLF.
Data availability statement
The raw data supporting the conclusions of this article will be made available by the authors, without undue reservation.
Author contributions
MH: Conceptualization, Investigation, Methodology, Software, Visualization, Writing–original draft, Writing–review and editing. LG: Conceptualization, Methodology, Software, Writing–review and editing. YW: Conceptualization, Writing–review and editing. XW: Data curation, Writing–review and editing.
Funding
The author(s) declare that financial support was received for the research, authorship, and/or publication of this article. This research was supported by the National Science and Technology Major Project of China (Grant number 2024ZD1002700) and the National Natural Science Foundation of China (Grant numbers 41974101 and 62127815).
Acknowledgments
We would like to express our sincere gratitude to Xuzhou Seismic Station and Datun Coal and Electricity (Group) Co., Ltd. for providing convenient support for security and equipment maintenance during the observation period, and to the Beijing Orangelamp Geophysical Exploration Co., Ltd. for supplying parts of the fluxgate instruments used in the observation experiments. Additionally, we thank the Institute of Geophysics, China Earthquake Administration for providing the observational data from the Mengcheng geomagnetic station. We are also grateful to all the workers and graduate students who participated in the continuous geomagnetic observations in Xuzhou City, China. Finally, we sincerely thank the Editor Prof. Alex Hay-Man Ng, and the two reviewers, Prof. F. Javier Pavón-Carrasco and Prof. Henglei Zhang, for their valuable comments and suggestions.
Conflict of interest
The authors declare that the research was conducted in the absence of any commercial or financial relationships that could be construed as a potential conflict of interest.
The reviewer HZ declared a shared affiliation with the authors to the handling editor at time of review.
Generative AI statement
The authors declare that no Generative AI was used in the creation of this manuscript.
Publisher’s note
All claims expressed in this article are solely those of the authors and do not necessarily represent those of their affiliated organizations, or those of the publisher, the editors and the reviewers. Any product that may be evaluated in this article, or claim that may be made by its manufacturer, is not guaranteed or endorsed by the publisher.
References
Chavarria, J. A., Malin, P., Catchings, R. D., and Shalev, E. (2003). A look inside the san Andreas Fault at parkfield through vertical seismic profiling. Science 302, 1746–1748. doi:10.1126/science.1090711
Chen, X. L., Xu, X., Cai, J. Y., Liao, K. X., and Huang, H. (2017). Research and data analysis of the deep borehole geomagnetic observation system in yangjiang city, Guangdong province. South China J. Seismol. 37, 81–89. doi:10.13512/j.hndz.2017.03.012
Deng, Q., Zhang, P., Ran, Y., Yang, X., Min, W., and Chu, Q. (2003). Basic characteristics of active tectonics of China. Sci. China Ser. D Earth Sci. 46, 356–372. doi:10.1360/03yd9032
Gaffet, S., Guglielmi, Y., Virieux, J., Waysand, G., Chwala, A., Stolz, R., et al. (2003). Simultaneous seismic and magnetic measurements in the low-noise underground laboratory (LSBB) of rustrel, France, during the 2001 january 26 Indian earthquake. Geophys. J. Int. 155, 981–990. doi:10.1111/j.1365-246X.2003.02095.x
Gao, Y. X., Zhao, G. Z., Chong, J. J., Klemperer, S. L., Han, B., Jiang, F., et al. (2020). Coseismic electric and magnetic signals observed during 2017 Jiuzhaigou Mw 6.5 earthquake and explained by electrokinetics and magnetometer rotation. Geophys. J. Int. 223, 1130–1143. doi:10.1093/gji/ggaa374
Gong, Y., Zhu, P. Y., Zhao, J. X., Shi, J. J., Du, L., and Wei, W. (2016). Preliminary analysis of geomagnetic record data quality of deep well station in earthquake administration of Shanghai municipality. Earthq. Res. Shanxi 2, 11–13. doi:10.3969/j.issn.1000-6265.2016.02.003
Guo, L. H., Wang, B., Song, X. L., Wang, Y., Jin, C. Y., Yao, S., et al. (2024). Continuous observation of geomagnetic total-field at the underground laboratory in Huainan City, China and its time-varying characteristics. Chin. J. Geophys. 67, 820–828. doi:10.6038/cjg2023Q0911
Henry, S., Borgo, E. P. D., Danquigny, C., and Abi, B. (2016). Simultaneous geomagnetic monitoring with multiple SQUIDs and fluxgate sensors across underground laboratories. E3S Web Conf 12, 02003. doi:10.1051/e3sconf/20161202003
Henry, S., Pozzo Di Borgo, E., Danquigny, C., Cavaillou, A., Cottle, A., Gaffet, S., et al. (2014). Monitoring geomagnetic signals of groundwater movement using multiple underground SQUID magnetometers. E3S Web Conf. 4, 02004. doi:10.1051/e3sconf/20140402004
Hickman, S., Zoback, M., and Ellsworth, W. (2004). Introduction to special section: preparing for the san Andreas Fault Observatory at depth. Geophys. Res. Lett. 31, 2004GL020688. doi:10.1029/2004GL020688
Johnston, M. J. S., Sasai, Y., Egbert, G. D., and Mueller, R. J. (2006). Seismomagnetic effects from the long-awaited 28 September 2004 M 6.0 Parkfield earthquake. Bull. Seismol. Soc. Am. 96, S206–S220. doi:10.1785/0120050810
Marfaing, J., Yedlin, M., Barroy, P., Auguste, M., Boyer, D., Cavaillou, A., et al. (2009). About the world-wide magnetic-background noise in the millihertz frequency range. Europhys. Lett. 88, 19002. doi:10.1209/0295-5075/88/19002
Nishijima, S., Eckroad, S., Marian, A., Choi, K., Kim, W. S., Terai, M., et al. (2013). Superconductivity and the environment: a roadmap. Supercond. Sci. Technol. 26, 113001. doi:10.1088/0953-2048/26/11/113001
Rosat, S., Hinderer, J., Boy, J. P., Littel, F., Bernard, J. D., Boyer, D., et al. (2018). A two-year analysis of the iOSG-24 superconducting gravimeter at the low noise underground laboratory (LSBB URL) of Rustrel, France: environmental noise estimate. J. Geodyn. 119, 1–8. doi:10.1016/j.jog.2018.05.009
Shen, H. H., Zhou, J. X., Zhang, X. X., Feng, Z. S., and Huangfu, D. Y. (2005). The influence analysis of direct current transmit electricity to geomagnetic observation. Seismol. Geomagnetic Observation Res. 26, 65–70. doi:10.3969/j.issn.1003-3246.2005.05.010
Wang, Y., Jian, Y., He, Y., Miao, Q., Teng, J., Wang, Z., et al. (2022). Underground laboratories and deep underground geophysical observations. Chin. J. Geophys. 65, 4527–4542. doi:10.6038/cjg2022Q0404
Wang, Y., Yang, Y. X., Xie, C. L., Zhang, Q. S., Cui, X. M., Chen, C., et al. (2023). Observation and research of deep underground multi-physical fields—Huainan -848 m deep experiment. Sci. Sin. (Terrae) 53, 55–71. doi:10.1360/SSTe-2022-0092
Waysand, G., Barroy, P., Blancon, R., Gaffet, S., Guilpin, C., Marfaing, J., et al. (2009). Seismo-ionosphere detection by underground SQUID in low-noise environment in LSBB-rustrel, France. Eur. Phys. J. Appl. Phys. 47, 12705. doi:10.1051/epjap:2008186
Xie, C., Chen, C., Liu, C., Wan, W., Jin, S., Ye, G., et al. (2024). Insights from underground laboratory observations: attenuation-induced suppression of electromagnetic noise. Eur. Phys. J. Plus 139, 218. doi:10.1140/epjp/s13360-024-05033-1
Xu, J. R., and Zhao, Z. X. (2009). Recent advance of borehole geophysical observation and Chinese continental scientific drilling long-term observatory at depth. Prog. Geophys. 24, 1176–1182. doi:10.3969/j.issn.1004-2903.2009.04.003
Yamauchi, T., Ishii, H., Asai, Y., Okubo, M., Matsumoto, S., and Azuma, S. (2005). Development of deep borehole instruments for both multi-component observation and in situ stress measurement, and some interesting results obtained. J. Seismol. Soc. Jpn. 2nd Ser 58, 1–14. doi:10.4294/zisin1948.58.1_1
Keywords: continuous geomagnetic observation, three components, underground, time-varying, North China
Citation: Han M, Guo L, Wang Y and Wang X (2025) Continuous underground observation of the geomagnetic field in Xuzhou City, North China, and its time-varying characteristics. Front. Earth Sci. 12:1505187. doi: 10.3389/feart.2024.1505187
Received: 02 October 2024; Accepted: 23 December 2024;
Published: 08 January 2025.
Edited by:
Alex Hay-Man Ng, Guangdong University of Technology, ChinaReviewed by:
F. Javier Pavón-Carrasco, Complutense University of Madrid, SpainHenglei Zhang, China University of Geosciences, China
Copyright © 2025 Han, Guo, Wang and Wang. This is an open-access article distributed under the terms of the Creative Commons Attribution License (CC BY). The use, distribution or reproduction in other forums is permitted, provided the original author(s) and the copyright owner(s) are credited and that the original publication in this journal is cited, in accordance with accepted academic practice. No use, distribution or reproduction is permitted which does not comply with these terms.
*Correspondence: Lianghui Guo, Z3VvbGhAY3VnYi5lZHUuY24=