- 1Beijing Earthquake Agency, Beijing, China
- 2Beijing Municipal Commission of Planning and Natural Resources, Beijing, China
- 3The Bureau of seismology of Yanqing District of Beijing, Beijing, China
- 4The Bureau of seismology of Haidian District of Beijing, Beijing, China
The Northern Margin Fault of the Yanqing Basin (NMYB Fault) is an important active fault at the intersection of the Zhangjiakou–Bohai (Zhang-Bo) Belt and the Shanxi Belt in North China. The Yanqing Basin, controlled by the NMYB Fault, is rich in escaping gas from hot springs, and previous investigations have indicated that the Yanqing Basin is located in the peak area of upwelling deep fluids from the mantle source material within the Zhang-Bo Belt. Hence, the site is suitable for geochemical gas precursor observations; to facilitate this, five new fault soil gas continuous stations were built on different segments of the NMYB Fault to carry out observations of fault gas (H2 and CO2) concentrations. The five new stations were approximately 50–60 m deep in the bedrock to monitor the release of gas from the depths of the fault. This was the first time that such geochemical station arrays were deployed in the same fault zone at a high density and depth. The results of the deep-hole observations of fault gas within the Yanqing Fault zone show that the time series of the hydrogen (H2) escape gas concentration has a close relationship with recent seismic activities, reflecting different physical processes of YFBF fault activity. The H2 concentration at the observatory was more sensitive to the stress-loading response of the NMYB Fault system.
1 Introduction
Earthquake precursor mechanisms are difficult to understand, which has hindered the development of earthquake prediction (Donald, 1988; Cicerone et al., 2009; Gherardi et al., 2017). The high uncertainty and low predictability of the earthquake forecasting process also make earthquakes one of the most serious natural hazards, often leading to instantaneous loss of life and property (Gupta, 2001; Wen et al., 2008). In recent years, especially in China, Japan, Italy, and the United States, anomalously high concentrations of hydrogen, carbon dioxide, helium, radon, and methane (H2, CO2, He, Rn, and CH4, respectively) in fault and hot spring gases have been extensively studied in seismically active faults in search of a probable earthquake precursor (King, 1986; Sugisaki and Sugiura, 1986; Nagamine, 1994; Cicerone et al., 2009; Babuska et al., 2016; Weinlich et al., 2016; Fischer et al., 2017; Huang et al., 2017; Yang et al., 2022). Temporal anomalous changes in gas concentrations, which could last from a few hours to a few days, have been observed at some monitoring stations before and after some large earthquakes, the epicenters of which were hundreds of kilometers away. The anomalous mechanisms are usually associated with chemical and physical changes occurring in active faults before and after the earthquakes, such as enhanced/decreased water–rock interactions, crustal stresses/strains, and permeability changes (Sugisaki et al., 1983; Sugisaki et al., 1996; Cicerone et al., 2009; Umeda et al., 2013; Weinlich et al., 2016; Chen and Liu, 2023).
The Yanqing Basin is rich in escaping gas from hot springs, making it an ideal site for fault gas monitoring. Previous investigations and studies of the samples of escaping gas from geothermal hot spring wells in the Yanqing North Fault of Beijing [Northern Margin Fault of the Yanqing Basin (NMYB Fault)] had been carried out. The results show that (Table 1) the hot spring escaping gas in the Yanqing Basin controlled by the Yanqing North Fault was rich in helium, and helium isotope ratios were richer, varying from 0.65 to 2.93 Ra. The mantle-sourced He averages 21.95% (maximum: 35.4%), indicating that the Yanqing Basin is located in the area of the upwelling deep fluids. The very good correspondence between the release of mantle-sourced gases and regional seismic activities indicates that the Yanqing Basin is a sensitive area for seismic geochemical observations and also an important active fault at the intersection of the Zhangjiakou–Bohai (Zhang-Bo) Seismic Belt and the Shanxi Seismic Belt of North China.
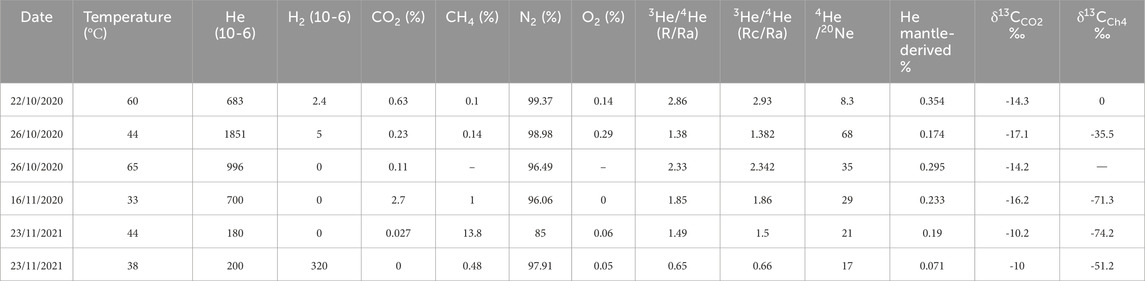
Table 1. Chemical composition and helium and carbon isotope data of hot spring gas in geothermal wells along the Zhangjiakou–Bohai Fault Zone (cited by Yang et al., 2022).
Therefore, in this paper, six new gas geochemical stations at high density along the NMYB Fault distribution were constructed (Figure 1) as pilots for applied research on gas geochemistry on active faults for an earthquake precursor. The research objectives of the paper include 1) monitoring the H2 concentration changes in the NMYB Fault before and after the earthquake; 2) analyzing hydrogen concentration characterization of short-term anomalies; and 3) investigating the mechanism of the gas precursor of earthquakes at the NMYB Fault.
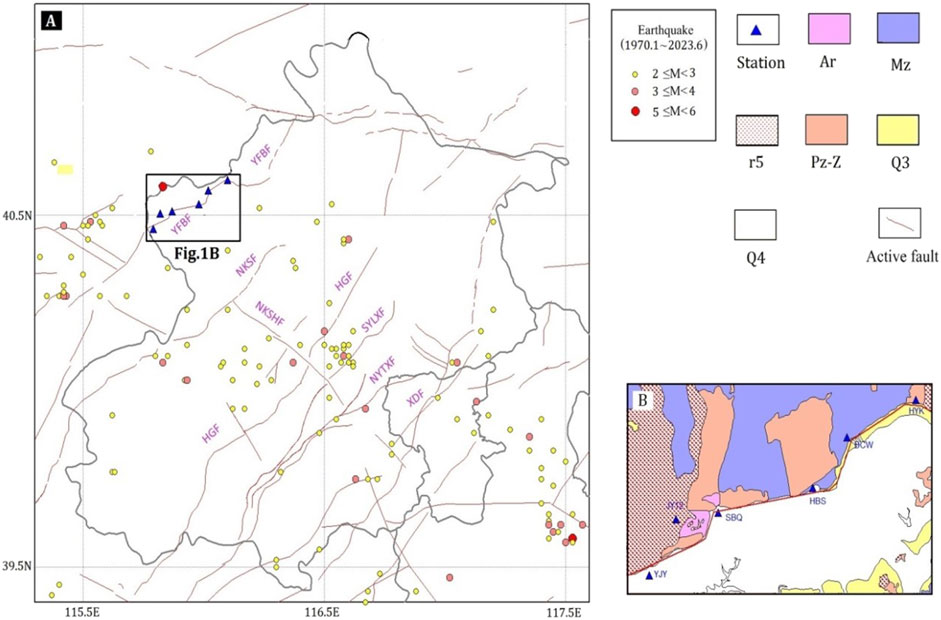
Figure 1. Distribution of gas geochemical stations at the Northern Margin Fault of the Yanqing Basin (NMYB Fault). (A) Locations of gas stations in the historic earthquake distribution map of Beijing. (B) Locations of gas stations in the geological map of the study area.
2 Geological background
Beijing is the capital of China with a large population, and it is located at the intersection of the North China Plain and the Zhang-Bo seismic belt. North China has experienced frequent strong earthquakes over the last few decades (Chen and Liu, 2023; Luo et al., 2024), such as the Xingtai M7.2 earthquake in 1966, Bohai M7.4 earthquake in 1969, Haicheng M7.3 earthquake in 1975, Tangshan M7.8 earthquake in 1976, and Zhangbei M6.2 earthquake in 1998. The Zhang-Bo seismic belt is a group of NW-W orderly active fault zones, starting from the northern margin of the Taihang Mountains in the west and entering the Bohai Sea in the east. It is an important NW seismic activity zone with frequent seismic activity in North China (Yang et al., 2022). Hence, there is a potential risk of damaging earthquakes in Beijing in the following years.
The Yanqing–Huailai (Yan-Huai) sediment basin is in the northwest region of Beijing, where the junction area of the Zhang-Bo seismic belt and Yanshan Mountains is located (Figure 1). Geologically, the basin boundary is mainly controlled by the pre-Cenozoic NNE-oriented positive-tilted and sliding fault groups. The faults continued to be active until the Late Quaternary (Fang et al., 1993). In terms of the overall structure of the basin, the NMYB Fault is the main active fault that controlled the formation and development of this shovel-type fault basin (Yu and Xu, 2004).
Bedrock is exposed in the study area from old to new, based on the stratigraphy of the area (Figure 1), including Archean Gneiss, Meso-Proterozoic carbonate, Mesozoic igneous rock, and Quaternary sediment. Most of the bedrock exposed in the fault zone consists mainly of Cretaceous and Jurassic coarse–facies felsic breccia tuffs, hornblende coarse andesite, gray–green andesite breccia mafic rocks, and volcanic rocks such as granite, which are in angular unconformable contact with the underlying tuffaceous dolomites of the Wumishan Formation in the Jixian System (Calymmian).
The existing geochemical methods from hot springs and gas stations in the Yanqing Basin have many typical earthquake precursor cases. For example, a large number of hot springs and gas anomalies were observed at several underground fluid-monitoring sites in the Yanqing Basin (Che et al., 1999) before the Datong earthquake (19 October 1989), Baotou West earthquake (3 May 1996), and Zhangbei earthquake (10 January 1998), which also suggested that the Yanqing Basin is a typical sensitive area for seismic gas precursors.
3 Methods
3.1 Gas sampling in the study area
Gas samples were collected from the hot springs and five deep soil holes from the NMYB Fault on 23 December 2022 (see Table 2). The gas samples were immediately sent to the Key Laboratory of the Institute of Earthquake Forecasting, China Earthquake Administration. Measurements of the samples were finished within 30 days. The compositions of the gas samples were analyzed using an Agilent 490 Gas Chromatograph, which measured H2, He, CO2, N2, O2, and CH4. The measurement accuracy was estimated as follows: the relative standard deviation was <0.5% when the content was 1%–100%, and the relative standard deviation was <1% when the content was 0.01%–1% (Zhou et al., 2015; Zhou et al., 2020). Helium and neon isotopes were measured using the Noblesse noble gas isotope mass spectrometer. When the R-value in helium isotope measurement was above 1 × 10−7, the test error was ±10%, and the measurement data error was at 1 × 10−8–1 × 10−7 were ±15% (Cao et al., 2018). The results were normalized to standard atmospheric values. The carbon isotope ratio was analyzed using the DeltaPlusXL mass spectrometer (Thermo Finnigan, United States), consisting of an HP 6890 Gas Chromatograph, a combustion/conversion furnace, and an interface connecting to the DeltaPlusXP mass spectrometer. The stable carbon isotope composition was expressed by δ13C; the accuracy of 13C/12C was 0.6‰ (Li et al., 2014).
3.2 Gas concentrations at gas geochemical stations
Based on the above concentration characteristics of the soil gas measurement, five new continuous gas monitoring stations were built along the NMYB Fault (Figure 1); these stations aim to monitor the continuous changes in fault gases (H2 and CO2), analyze the spatial differences of the gas characteristics in different fault segments, and observe their changes over time. Furthermore, the five gas stations can monitor the stress adjustments and seismicity of the Beijing area. The five geochemical monitoring sites along the Northern Margin Fault form high-density gas geochemical networks for automatic and continuous gas sampling and observation (Figure 1 shows the distribution of the stations).
In the past, traditional soil/fault gas observations were subject to many disturbing factors, mainly the shallow depth of gas extraction and its susceptibility to biogenic gases and atmospheric disturbances. In order to minimize the influence of these surface factors, the quality of monitoring was improved, the influence of gases produced by biogenic layers and humus in the soil was avoided as much as possible, and the seasonal interference of the ground temperature was avoided. Fault gas fixed monitoring sites were constructed this time, reaching approximately 50–60 m deep into the bedrock. The depth was well beyond the variable temperate zone of the region (the region’s variable temperature zone is generally in the range of 20 m).
Hot spring well stations and faulted soil gas stations make the monitoring of additional tectonic activity possible. The monitoring environment is very stable. There is no industrial or mining activity around the monitoring stations. Instead, the fault gas is introduced at a depth of 50–60 m below ground level using sieve-perforated gas collection cylinders. Both hot spring and fault gases are accumulated (Figure 2) and introduced using polytetrafluoroethylene (PTFE) tubes (length: 33–55 m, outer diameter: 3 cm, and inner diameter: 2 cm). Hydrogen concentrations were monitored every 2 h using an ATG-6118H autoanalyzer in the range of 0.01–1,000 ppm with an accuracy of ±5%, and CO2 concentrations were monitored every 2 h using an ATG-C600 CO2 continuous monitor in the measurement range of 0%–60% with a resolution of 0.001%. Both instruments were calibrated and maintained by the instrument manufacturing company (Wen et al., 2018; NOA Certification, 2019). All gas piping was well sealed from the effects of weather, air, and temperature, as well as external humidity and rainfall. The data obtained from this system were transmitted in real time via 5G.
The gas background values of the new deep-bore fault gas wells in the NMYB Fault correlate with the historical earthquakes. According to the curves of the background H2 observation values of the stations when the station was being constructed (Figure 3), it could be seen that the background values of the hydrogen concentration in the area between Yaojiaying (YJY) and Huangbaisi (HBS), where more historical earthquakes had occurred, were higher. In addition, the historical earthquakes of Baicaowa (BCW) and Huangyukou (HYK), located in the northeast of the rupture, were also fewer, and their H2 concentration background values were low at the same time (Sugisaki and Sugiura, 1986; Cheng et al., 1995).
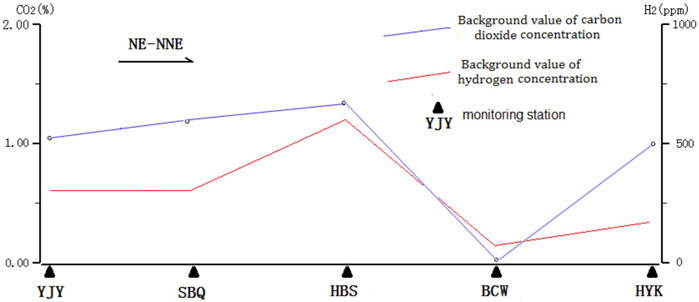
Figure 3. Monitoring values of the five H2 and CO2 concentrations were sent to a laboratory in Beijing through cellphone signals.
4 Results
4.1 Gas chemical composition in stations
Gases (isotopic ratios of CO2, He, Ne, and other gas compositions) collected from Yanqing soil gas wells from 1 January 2023 to 31 January 2024 are shown in Table 2. The N2 concentration of the gas samples was ≥79 vol%. Helium and H2 concentrations in the gases were relatively low (≤500 ppm). O2 concentrations in most fugitive gas samples were less than 20%. Methane concentrations were below 3%. Most CO2 concentrations were below 5%.
4.2 Concentration of H2 in stations
4.2.1 Time-series variation in H2 concentration at the Huangbaisi (HBS) station
From 1 January 2023 to 31 January 2024, there were 4,752 datasets on H2 concentrations (Table 3), which ranged from 0.01 to 501 ppm, with an average of 97 ppm. The anomaly threshold is 170 ppm (Table 3; Figure 4A) according to the Q–Q plot, which is a method of determining the relationship between background and anomalous geochemical data based on the cumulative probability plot delineation of the data threshold (Sinclair, 1991; Zhou et al., 2021). In order to analyze the effect of humidity on the observed values, the correlation coefficient between hydrogen and humidity was calculated to be −0.127 for the 1 January 2023 value of 31 January 2024 at HBS, indicating a very low correlation (see Figure 4B).
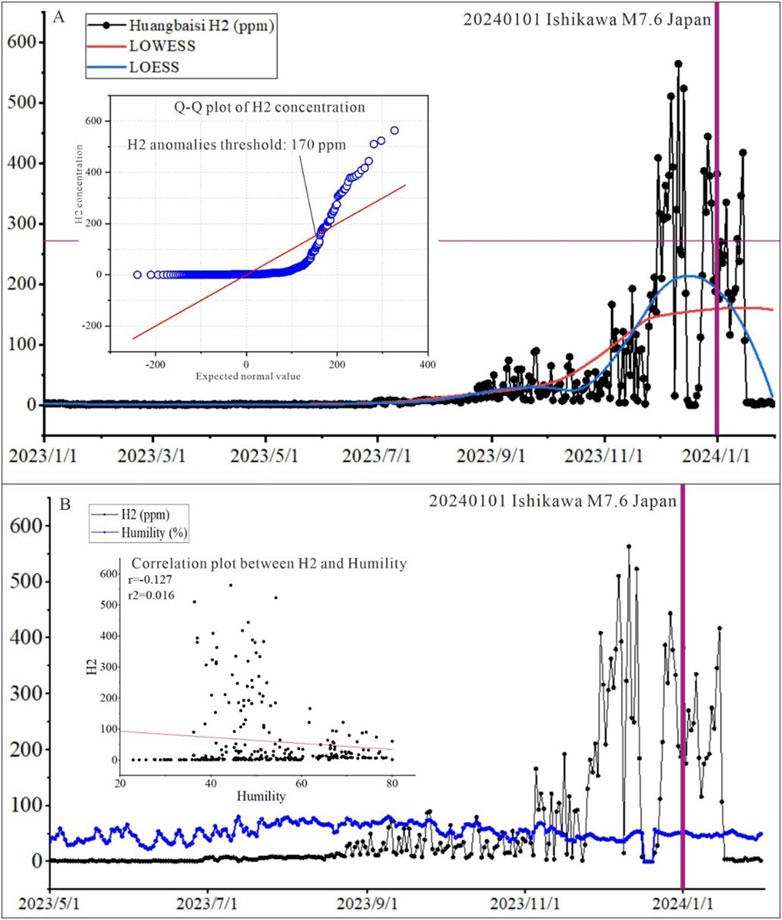
Figure 4. (A) Q–Q plot of H2 concentration at HBS. (B) Correlation between H2 concentration and humidity.
4.2.2 Time-series variation in H2 at the Shangbanquan (SBQ) station
From 1 January 2023 to 31 January 2024, there were 4,752 datasets on H2 concentrations (Table 3), which ranged from 0.01 to 40 ppm, with an average of 10 ppm. The anomaly threshold was 15 ppm (Table 3; Figure 5A) according to the Q–Q plot. In order to analyze the effect of humidity on the observed values, from 1 January 2023 to 31 January 2024, the correlation coefficient between hydrogen and humidity was calculated to be 0.069 at SBQ, which is also a low correlation (see Figure 5B).
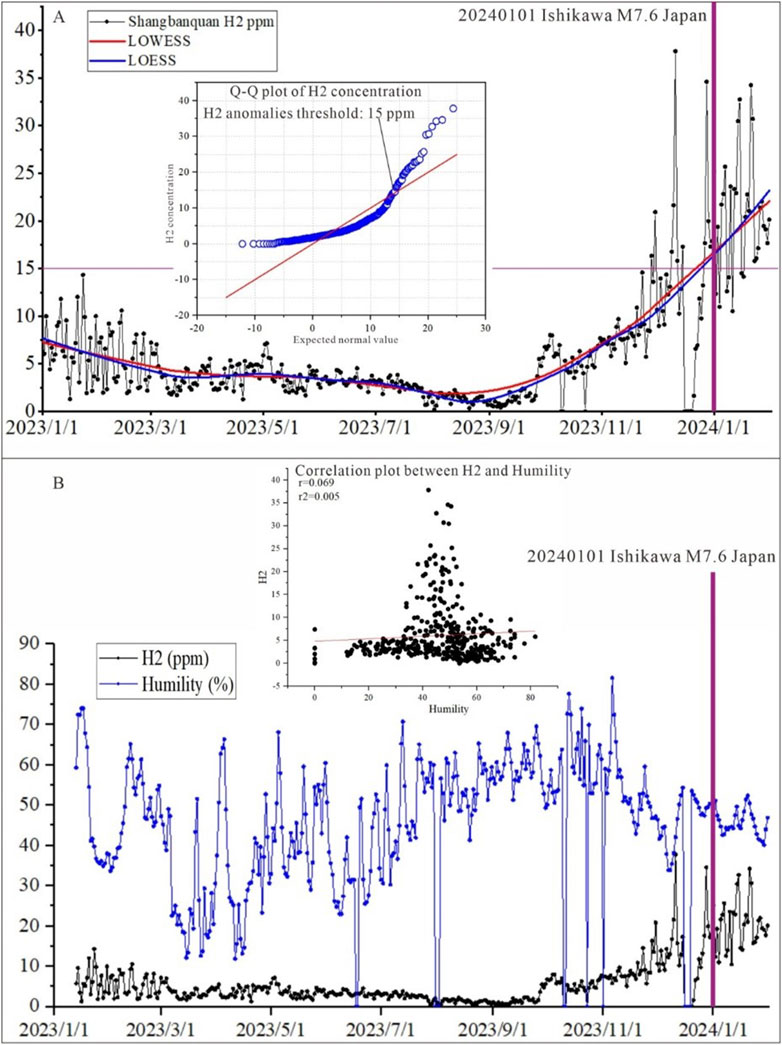
Figure 5. (A) Q–Q plot of H2 concentration at SBQ. (B) Correlation between H2 concentration and humidity.
4.2.3 Time-series variation in H2 at the Huangyukou (HYK) station
From 1 January 2023 to 31 January 2024, there were 4,752 datasets on H2 concentrations (Table 3), which ranged from 0.01 to 40 ppm, with an average of 9.7 ppm. The anomaly threshold of 16 ppm (Table 3; Figure 6A) was determined by a Q–Q plot. In order to analyze the effect of humidity on the observed values, the correlation coefficient between hydrogen and humidity was calculated to be 0.44 for the 1 January 2023 value of 31 January 2024 at SBQ, which is also a low correlation (see Figure 6B).
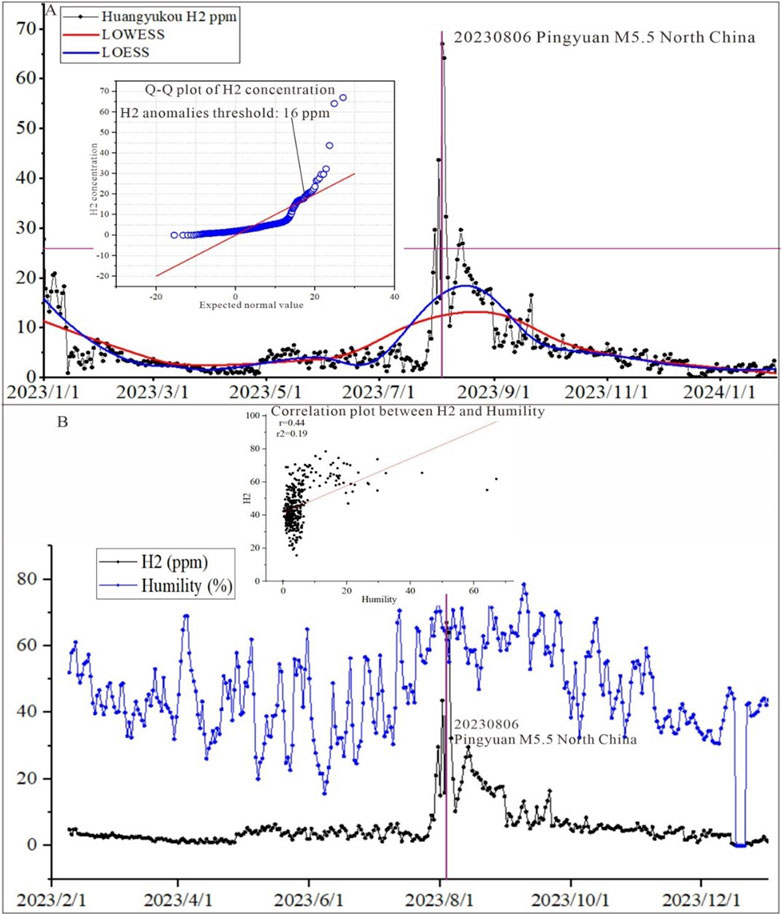
Figure 6. (A) Q–Q plot of H2 concentration at HYK. (B) Correlation between H2 concentration and humidity.
5 Discussion
5.1 Sources of H2
The source of H2 is most likely attributable to (A) H2 produced by water–rock reactions. Abiotic H2 is produced under near-surface conditions when water reacts with ultramafic rocks or serpentinization and migrates from deeper reservoirs (Lollar et al., 2014; Parnell and Blamey, 2017; Irfan et al., 2019; Wang et al., 2019; Wang et al., 2020); (B) water interaction with the newly exposed rock surface (Hirose et al., 2011); and (C) mixing with large amounts of microbial H2 produced by bioactivity and organic matter decomposition (Prinzhofer et al., 2019; Myagkiy et al., 2020).
Laboratory experimental data showed that tectonic stress caused by the reaction of broken minerals and groundwater can produce H2. The observed significant increase in H2 in the high concentration of anomalies before and after many large earthquakes suggests that there is a close relationship between the changes in the concentration of H2 and the earthquakes (Sugiyaki et al., 1983; Zhou et al., 2021). A background investigation on soil was carried out by Li et al. (2014) and Chen et al. (2022) over a larger area including the study area. The results show that there are low-velocity bodies in the upper crust of the eastern part of the Yanqing–Huailai Basin (including the Yanqing Basin), with strong tectonic activity. The investigated gases, including Hg and He, mainly originate from the deeper part of the fault zones, contributing more to the soil gas. H2 also originates from the deeper part but was influenced by the redox environment in the soil deposits, which results in a wide range of variations in the H2 content (Li et al., 2014).
5.2 Time-series variation characteristics and anomalies of H2 before earthquakes
Prior to earthquakes from 1 January 2023 to 1 January 2024, there were significant short-term (120–720 h) seismic precursor anomalies in the H2 concentration of fault escape gases at HYK, HBS, and SBQ (Figure 7). Earthquakes occur when stress increases to a state of sub-instability stress on faults (Ma, 2016). The hydrogen concentration in the escaping gas is very sensitive to the increase in stress, which may enhance the openness of microfractures in the fractures along the Northern Margin of the Yanqing Basin.
The anomaly information was reported to the Beijing Earthquake Agency prior to the 5 August 2023 Shandong Pingyuan M5.5 and 1 January 2024 Japan M7.3 earthquakes.
Prior to these two earthquakes, there were significant and persistent precursor anomalies in the H2 concentrations of soil gas in each of the HYK, HBS, and SBQ stations. When the stress increases to a state of sub-instable stress on the faults, the faults misalign to produce earthquakes (Ma, 2016), so the mechanism of precursor anomalies may have been caused by the sustained stress increase prior to the natural seismic events.
The hydrological response to earthquakes depends on the earthquake’s magnitude and epicenter (Wang and Manga, 2010; Cox et al., 2015). Therefore, systematic identification of short-term seismic precursor anomalies based on multiple earthquakes using certain criteria is difficult. By drawing on and analyzing the collection of many natural earthquake cases in the same region, the equation of geochemical gas precursor anomalies in relation to magnitude and distance from the epicenter can be summarized, and some criteria can be provided.
5.2.1 H2 concentration at HYK
Before the 5 August 2023 Pingyuan County M5.5 earthquake in Dezhou city of Shandong Province of China, the H2 concentration anomaly increased at Huangyukou (Figure 7), which appeared 5 days before the earthquake and ended before the earthquake. Details were as follows: it began to rise abruptly on July 30, reaching a first peak of 65.65 ppm on the July 31, a second peak of 91.56 ppm at 11:40 on August 2, and a third peak of 174.6 ppm at 00:40 on August 5, and then, it returned to a low point of 19.568 ppm at 18:40 on August 5. The M5.5 earthquake in Shandong Pingyuan occurred at 02:33 on August 6 and returned to normal at 18:40 on August 7 (Figure 7; Table 4).
5.2.2 H2 concentration at HBS and SBQ
Before the 1 January 2024 M7.6 earthquake in Ishikawa, Japan, there were significant short-term anomalies in the hydrogen concentration in HBS and SBQ (occurring 30 days and 20 days before the earthquake, respectively, and the anomalies decreased significantly after the earthquake), and the parameters of the precursor anomalies for the earthquakes are shown in Figure 7; Table 4.
Analyzing the H2 anomaly magnitude, it was found that the H2 anomaly magnitude at HYK before Shandong Pingyuan M5.5 was larger than that at SBQ and HBS before the Japan M7.6 earthquake. The anomaly amplitude before the Shandong Pingyuan M5.5 earthquake was as high as seven times, while the anomaly amplitudes before the Japan M7.6 earthquake were four and five times, respectively (Figure 7). From another point of view, it indicates that the loading effect of the stress field of the earthquakes with far epicentral distance has a significantly weaker relation with the earthquakes within the North China region.
5.3 Simulation of gas concentration migration in faults
Chen et al. (2022) investigated the relationship between the gas release characteristics of the major active fault zones within the North China Craton and the regional tectonic framework. The results show that in the Zhang-Bo tectonic belt, the release of soil gas Rn and CO2 and the contribution of crustal or mantle sources in the escaping gas from hot springs are significantly higher than in other tectonic zones in the region. A comprehensive analysis combining the results of the shear strain rate field, GPS horizontal velocity field, and S-wave velocity imaging reveals that the region was characterized by strong tectonic activities, and the development of nascent fractures may be widespread in the subsurface, and mantle-source fluids may be present in the subsurface of the Zhang-Bo tectonic belt for 20–40 km. It was also found that the peak region of helium from the mantle source material in the Zhang-Bo belt in the Yanqing Basin region showed increased deep fluid upwelling.
The NMYB Fault is located in the northern part of the central orogenic belt of the North China Craton, which consists of two east–west grade blocks of the North China Craton collided and spliced in the Paleoproterozoic (Liu and Zhao, 2012). After experiencing many geologic effects, a large number of faults were developed under the stresses of Cenozoic tensile-slip, and basin–mountain coupling tectonics were formed in the adjacent basins blocked by the mountain system (Xu et al., 1998), and the Yanqing Basin is one of them (Yang et al., 2022).
Subduction of the paleo-Pacific plate sheet is the geodynamic mechanism leading to the thinning and destruction of the lithospheric mantle of the North China Craton (Zhu et al., 2017). The destruction of the North China Craton occurred mainly in the area east of the Taihang Mountains in North China, resulting in the intrusion of magma, earthquakes, and volcanism in the area, including the Zhang-Bo belt, which are closely related to the geologic activities. The Yanhuai Basin, located in the Zhang-Bo Belt, is one of the areas with the potential danger of strong earthquakes in the eastern part of mainland China. The mechanism of earthquake occurrence in the Yanhuai Basin and basin–mountain interaction, the monitoring and prediction of future strong earthquakes, and other scientific and applied issues have also been attracting the attention of earthquake scientists.
Hence, in the broader context of tectonically active and fracture development in the Zhang-Bo belt region, combined with gas observation anomalies in this region, a model was designed to explain the anomalies. The model assumes that the NMYB Fault is a gas source system capable of responding to external stress loading and that during the anomalous time period, there was stress loading from the periphery of the fault, and anomalous gas rises, along with increases in the H2 concentration, were observed at the observatory stations (see Figure 8).
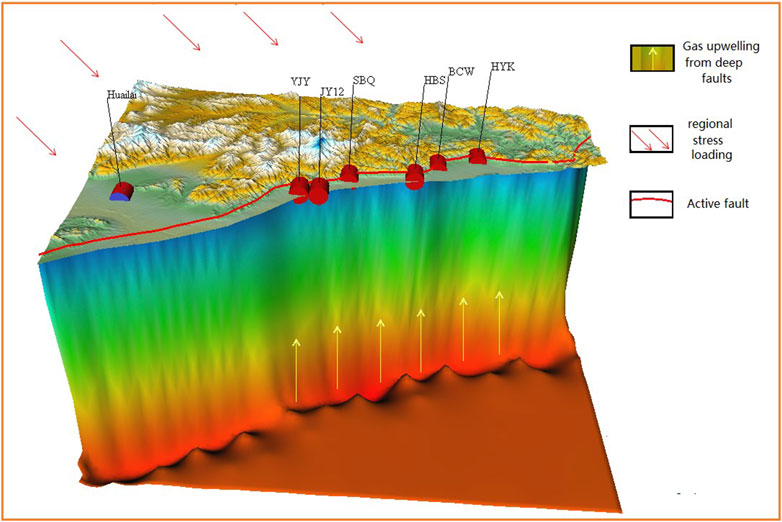
Figure 8. Modeling of the stress loading and gas transportation process in the fracture at the Northern Margin of the Yanqing Basin.
This can be explained by the fact that in the fracture system, the hydrogen concentration in the escaping gas is very sensitive to the increase in stress, which has the potential to enhance the openness of the microfracture under the Yanqing Fracture, leading to an increase in the concentration of the gas originating from the depth.
At the same time, during stress loading, hydrogen released from rock fragmentation and hydrogen generated by water–rock chemistry at the active fault site make hydrogen more concentrated near the active fault, so some hydrogen anomalies can be observed at the location of the active fault at the surface.
6 Conclusion
1. The high-density geochemical observatory stations near the fractures at the Northern Margin of the Yanqing Basin are a preferable site for pilot research on gas geochemistry in active faults.
2. The time series of H2 concentration observations exhibit complex temporal patterns, reflecting a wide range of different physical processes. Observatories located along the rupture showed significant short-term hydrogen concentration anomalies (5–30 days) prior to the earthquake, which may be related to regional stress loading.
3. The use of high-precision automatic continuous monitoring stations to observe hydrogen concentration and other concentrations (CO2, He, radon, water temperature, and ion concentrations) at active rupture locations can be analyzed to determine the physical process of rupture zone activity; the next step is to combine and synthesize the time series and spatial characteristics of hydrogen concentrations and multiple fault gases from observation stations in the same rupture zone. This approach could be a valuable tool for further unraveling the mechanism of earthquakes and predicting earthquakes.
Data availability statement
The original contributions presented in the study are included in the article/supplementary material; further inquiries can be directed to the corresponding authors.
Author contributions
MY: conceptualization, funding acquisition, investigation, methodology, writing–original draft, and writing–review and editing. GL: conceptualization, funding acquisition, supervision, and writing–review and editing. YC: methodology, visualization, and writing–review and editing. PH: investigation and writing–review and editing. LH: writing–review and editing. ZW: writing–review and editing. SW: investigation and writing–review and editing. XS: writing–review and editing. YoZ: writing–review and editing. HZ: methodology, investigation, writing–review and editing. GF: methodology, investigation, writing–review and editing. XG: methodology, investigation, writing–review and editing. YZ: formal analysis, writing–review and editing.
Funding
The author(s) declare that financial support was received for the research, authorship, and/or publication of this article. This work was supported by the surface technology project of the Beijing Earthquake Agency (No. BJMS-2024005).
Acknowledgments
The authors thank the editors and reviewers for valuable constructive comments on this manuscript that greatly enhanced the clarity and improved this work.
Conflict of interest
The authors declare that the research was conducted in the absence of any commercial or financial relationships that could be construed as a potential conflict of interest.
Publisher’s note
All claims expressed in this article are solely those of the authors and do not necessarily represent those of their affiliated organizations, or those of the publisher, the editors, and the reviewers. Any product that may be evaluated in this article, or claim that may be made by its manufacturer, is not guaranteed or endorsed by the publisher.
References
Babuska, V., Ruzek, B., and Dolejs, D. (2016). Origin of earthquakes warms in the western Bohemian Massif:Is the mantle CO2 degassing, followed by the Cheb Basin subsidence, an essential driving force? Tectonophysics 668–669, 42–51. doi:10.1016/j.tecto.2015.12.008
Cao, C. H., Zhang, M. J., Tang, Q. Y., Yang, Y., Lv, Z. G., Zhang, T. W., et al. (2018). Noble gas isotopic variations and geological implication of Longmaxi shale gas in Sichuan Basin, China. China. Mar. Petrol. Geol. 89, 38–46. doi:10.1016/j.marpetgeo.2017.01.022
Che, Y. T., Yu, J. Z., Liu, W. Z., and Yi, L. X. (1999). Analysis of groundwater anomaly field and its causes in the Zhangbei-Shangyi earthquake (in Chinese). Acta Seismol. Sin. 1999 (3), 194–201.
Chen, Y. X., and Liu, J. B. (2023). Groundwater trace element changes were probably induced by the ML3.3 earthquake in Chaoyang district, Beijing. Front. Earth Sci. 11. doi:10.3389/feart.2023.1260559
Chen, Z., Ying, Li, Liu, Z., He, H., Martinelli, G., Lu, C., et al. (2022). Geochemical and geophysical effects of tectonic activity in faulted areas of the North China Craton. Chem. Geol. 609, 121048. doi:10.1016/j.chemgeo.2022.121048
Cheng, S. P., Fang, Z. J., Yang, G. Z., and Yang, Z. (1995). On segmentation of the fault zone along the North Margin of Yanqing Basin and earthquake prediction. Seismol. Geol. 17 (3), 231–240. doi:10.1007/978-3-642-31445-2_7
Cicerone, R. D., Ebel, J. E., and Britton, J. (2009). A systematic compilation ofearthquake precursors. Tectonophysics 476 (3–4), 371–396. doi:10.1016/j.tecto.2009.06.008
Cox, S. C., Menzies, C. D., Sutherland, R., Denys, P. H., Chamberlain, C., and Teagle, D. A. H. (2015). Changes in hot spring temperature and hydrogeology of the Alpine Fault hanging wall, New Zealand, induced by distal South Island earthquakes. Geofluids 15, 216–239. doi:10.1111/gfl.12093
Donald, T. (1988). Geochemical precursors to seismic activity. Pure Appl. Geophys126 126 (2-4), 241–266. doi:10.1007/bf00878998
Fang, Z. J., Cheng, S. P., and Ran, Y. K. (1993). Yan-Huai basin-ridge structure and some features of late quanternary fracture movement (in Chinese). Prog. Geophys. (04), 265–266.
Fischer, T., Matyska, C., and Heinicke, J. (2017). Earthquake-enhanced permeability-evidence from carbon dioxide release following the ML 3.5 earthquake in West Bohemia. Earth Planet Sci. Lett. 460, 60–67. doi:10.1016/j.epsl.2016.12.001
Gherardi, F., Pierotti, L., and Vista, A. D. (2017). Water-rock interactions in a siteinvestigated for geochemical precursors of earthquakes: the pieve foscianaspring (Italy). Earth Planet Sci. Lett. 17, 360–363. doi:10.1016/j.proeps.2016.12.091
Gupta, H. K. (2001). Short-term earthquake forecasting may be feasible at Koyna, India. Tectonophysics 338 (3–4), 353–357. doi:10.1016/s0040-1951(01)00083-x
Hirose, T., Kawagucci, S., and Suzuki, K. (2011). Mechanoradical H2 generation during simulated faulting: Implications for an earthquake-driven subsurface biosphere. Geophys. Res. Lett. 38, L17303. doi:10.1029/2011.gl048850
Huang, F., Li, M., Ma, Y., Han, Y., Tian, L., Yan, W., et al. (2017). Studies on earthquake precursors in China: A review for recent 50 years. Geod. Geodyn. 8 (1), 1–12. doi:10.1016/j.geog.2016.12.002
Irfan, M., Zhou, L., Bai, Y., Yuan, S., Liang, T. T., Liu, Y. F., et al. (2019). Insights into the hydrogen generation from water-iron rock reactions at low temperature and the key limiting factors in the process. Int. J. Hydrogen Energy 44 (33), 18007–18018. doi:10.1016/j.ijhydene.2019.05.086
King, C. Y. (1986). Gas geochemistry applied to earthquake prediction: anoverview. J. Geophys. Res. 91, 12269–12281. doi:10.1029/jb091ib12p12269
Li, Z. P., Wang, X. B., Li, L. W., Zhang, M. J., Tao, M. X., Xing, L. T., et al. (2014). Development of new method of δ13C measurement for trace hydrocarbons in natural gas using solid phase micro-extraction coupled to gas chromatography isotope ratio mass spectrometry. J. Chromatogr. A 1372, 228–235. doi:10.1016/j.chroma.2014.10.089
Liu, C. H., Liu, F. L., and Zhao, G. C. (2012). The Paleoproterozoic basin evolution in the Trans-North China Orogen, North China Craton. Acta Petrol. Sin. 28 (9), 2770–2784.
Lollar, B. S., Onstott, T. C., Lacrampe-Couloume, G., and Ballentine, C. J. (2014). The contribution of the Precambrian continental lithosphere to global H2 production. Nature 516, 379–382. doi:10.1038/nature14017
Luo, Z., Yang, M., Zhou, X., Liu, G., Liang, J., Liu, Z., et al. (2024). Evaluation of Various Forms of Geothermal Energy Release in the Beijing Region, China. Water 16, 622. doi:10.3390/w16040622
Ma, J. (2016). On whether earthquake precursors help for prediction do exist. Chin. Sci. Bull. 61, 409–414. doi:10.1360/n972015-01239
Myagkiy, A., Brunet, F., Popov, C., Krüger, R., Guimarães, H., Sousa, R. S., et al. (2020). H2 dynamics in the soil of a H2-emitting zone (São Francisco Basin, Brazil): Microbial uptake quantification and reactive transport modelling. Appl. Geochem. 112, 104474. doi:10.1016/j.apgeochem.2019.104474
Nagamine, K. (1994). Origin and coseismic behavior of mineral spring gas atByakko, Japan, studied by automated gas chromatographic analyses. Chem. Geol. 114 (1–2), 3–17. doi:10.1016/0009-2541(94)90038-8
NOA certification (2019). Validity of the certificate:2019/7/25∼2020/7/25. Available at: http://cx.cnca.cn/CertECloud/result/skipResultList.
Parnell, J., and Blamey, N. (2017). Global hydrogen reservoirs in basement and basins. Geochem. Trans. 18, 2. doi:10.1186/s12932-017-0041-4
Prinzhofer, A., Moretti, I., Françolin, J., Pacheco, C., D’Agostino, A., Werly, J., et al. (2019). Natural hydrogen continuous emission from sedimentary basins: The example of a Brazilian H2-emitting structure. Int. J. Hydrogen Energy 44 (12), 5676–5685. doi:10.1016/j.ijhydene.2019.01.119
Sinclair, A. J. (1991). A fundamental approach to threshold estimation in exploration geochemistry: Probability plots revisited. J. Geochem. Explor. 41 (1-2), 1–22. doi:10.1016/0375-6742(91)90071-2
Sugisaki, R., Ido, M., Takeda, H., Isobe, Y., Hayashi, Y., Nakamura, N., et al. (1983). Origin of hydrogen and carbon dioxide in fault gases and its relation to fault activity. J. Geol. 91 (3), 239–258. doi:10.1086/628769
Sugisaki, R., Ito, T., Nagamine, K., and Kawabe, I. (1996). Gas geochemical changes at mineral springs associated with the 1995 southern Hyogo earthquake (M = 7.2), Japan. Earth Planet Sci. Lett. 139 (1-2), 239–249. doi:10.1016/0012-821x(96)00007-6
Sugisaki, R., and Sugiura, T. (1986). Gas anomalies at three mineral springs and afumarole before an inland earthquake, central Japan. J. Geophys. Res. 91 (B12), 12296–12304. doi:10.1029/jb091ib12p12296
Umeda, K., Asamori, K., and Kusano, T. (2013). Release of mantle and crustalhelium from a fault following an inland earthquake. Appl. Geochem. 37, 134–141. doi:10.1016/j.apgeochem.2013.07.018
Wang, C. Y., and Manga, M. (2010). Hydrologic responses to earthquakes and a general metric. Geofluids 10, 206–216. doi:10.1111/j.1468-8123.2009.00270.x
Wang, J., Watanabe, N., Okamoto, A., Nakamura, K., and Komai, T. (2019). Acceleration of hydrogen production during water-olivine-CO2 reactions via high-temperature-facilitated Fe(II) release. Int. J. Hydrogen Energy 44 (23), 11514–11524. doi:10.1016/j.ijhydene.2019.03.119
Wang, J., Watanabe, N., Okamoto, A., Nakamura, K., and Komai, T. (2020). Characteristics of hydrogen production with carbon storage by CO2-rich hydrothermal alteration of olivine in the presence of Mg–Al spinel. Int.J. Hydrogen Energy 45 (24), 13163–13175. doi:10.1016/j.ijhydene.2020.03.032
Weinlich, F. H., Gazdová, R., Teschner, M., and Poggenburg, J. (2016). The ˇOctober 2008 Nový Kostel earthquake swarm and its gas geochemicalprecursor. Geofluids 16 (5), 826–840. doi:10.1111/gfl.12187
Wen, L., He, L., Feng, E., Lian, K., Chen, Q., Zhang, L., et al. (2018). Calibration,Performance Testing and Their Results of the Automatic Trace Hydrogen Analyzer ATG-6118H. Earthq. Res. China 34 (3), 571–579.
Wen, X., Ma, S., Xu, X., and He, Y. (2008). Historical pattern and behavior ofearthquake ruptures along the eastern boundary of the Sichuan-Yunnanfaulted-block, southwestern China. Phys. Earth Planet 168 (1-2), 16–36. doi:10.1016/j.pepi.2008.04.013
Xu, J., Song, C., and Chu, Q. (1998). Preliminary study on the seismotectonic characteristics of the Zhangjiakou Penglai fault zone. Seismol. Geol. 20 (2), 146–154. doi:10.1088/0256-307X/16/9/027
Yang, M. B., Liu, G. P., Liu, Z., Ma, J. C., Li, L. W., Wang, Z. G., et al. (2022). Geochemical characteristics of geothermal and hot spring gases in Beijing and Zhangjiakou Bohai fault zone. Front. Earth Sci. 10, 933066. doi:10.3389/feart.2022.933066
Yu, G. H., and Xu, X. W. (2004). Study on relationship between deep and shallow Structures along north boundary fault of yanqing-fanshan basin (in Chinese). Acta Seismol. Sin. 26 (1), 68–76.
Zhou, X., Wang, W., Chen, Z., Yi, L., Liu, L., Xie, C., et al. (2015). Hot spring gasgeochemistry in western Sichuan Province, China after the Wenchuan Ms 8.0earthquake. Terr. Atmos. Ocean. Sci. 26 (4), 361–373. doi:10.3319/tao.2015.01.05.01(tt
Zhou, X., Wang, W., Li, L., Hou, J., Xing, L., Li, Z., et al. (2020). Geochemicalfeatures of hot spring gases in the Jinshajiang-Red River fault zone,Southeast Tibetan Plateau. Acta Petrol. Sin. 36 (7), 2197–2214. doi:10.18654/1000-0569/2020.07.18
Zhou, X., Yan, Y., Fang, W., Wang, W., and Shi Hand Li, P. (2021). Short-Term Seismic Precursor Anomalies of Hydrogen Concentration in Luojishan Hot SpringBubbling Gas, Eastern Tibetan Plateau. Front. Earth Sci. 8, 586279. doi:10.3389/feart.2020.586279
Keywords: Zhangjiakou–Bohai tectonic zone, gas geochemistry, helium isotope, Yanqing–Huailai basin, earthquake prediction
Citation: Yang M, Liu G, Chen Y, Hua P, Hu L, Wang Z, Wang S, Sun X, Zhou Y, Zhang H, Feng G, Gao X and Zhang Y (2025) Short-term seismic precursor anomalies in hydrogen concentration at fault gas stations along the Northern Margin Fault of the Yanqing Basin of Beijing, China. Front. Earth Sci. 12:1474630. doi: 10.3389/feart.2024.1474630
Received: 02 August 2024; Accepted: 24 October 2024;
Published: 06 January 2025.
Edited by:
Giovanni Martinelli, National Institute of Geophysics and Volcanology, Section of Palermo, ItalyReviewed by:
Jiao Tian, China Earthquake Administration, ChinaXiaocheng Zhou, China Earthquake Administration, China
Copyright © 2025 Yang, Liu, Chen, Hua, Hu, Wang, Wang, Sun, Zhou, Zhang, Feng, Gao and Zhang. This is an open-access article distributed under the terms of the Creative Commons Attribution License (CC BY). The use, distribution or reproduction in other forums is permitted, provided the original author(s) and the copyright owner(s) are credited and that the original publication in this journal is cited, in accordance with accepted academic practice. No use, distribution or reproduction is permitted which does not comply with these terms.
*Correspondence: Mingbo Yang, eWFuZ21iMjAwOEAxNjMuY29t; Guiping Liu, bGl1Z3VpcGluZ0BianNlaXMuZ292LmNu; Yuxuan Chen, Y3l4NjMwQDE2My5jb20=