- 1Research Institute of Petroleum Exploration and Development, Beijing, China
- 2State Key Laboratory for Enhanced Oil Recovery, Beijing, China
The sedimentary system of the delta front is complex. The hydrodynamic conditions, changes in sediment supply, and mineral composition are crucial factors that influence the quality of reservoirs. Reservoir quality varies among different sedimentary units. In this field of research, there is a general tendency to mainly use core data and logging curves for reservoir characterization and prediction, while neglecting the combination with the microscopic scale of the reservoir. This article proposes a new method to characterize reservoir heterogeneity by studying the sedimentary characteristics of reservoirs. This method uses casting thin-section data to aid in core and logging research. This study consists of five parts: core analysis, casting thin-section study, logging facies study, geological analysis, and single-well prediction. Combining macroscopic and microscopic methods helps clarify the controlling effect of sedimentary characteristics on reservoir heterogeneity. The research results indicate that this research method effectively solves the challenge of characterizing reservoir heterogeneity in the middle and later stages of development of low-permeability reservoirs. The research outputs serve as valuable references for the advancement of analytical matching fields.
1 Introduction
In recent years, the development strategy of old oilfields has been focusing on “water flooding decline, benefit construction, and enhanced oil recovery” in order to fulfill the goal of steady development of these oilfields in the Ordos Basin, China (Jiang, 2020). Reservoir heterogeneity is a major difficulty in the middle and later stages of development of old oilfields (Ma et al., 2019; Doorwar et al., 2020). The understanding of reservoir heterogeneity often guides further development plan design and development measure adjustment for oilfields (Chen et al., 2014; Ehrenberg, 2019). Reservoir heterogeneity commonly refers to vertical and lateral variations in porosity, permeability, and capillarity (Schmid et al., 2004; Medici et al., 2019; Shehata et al., 2021). Reservoir quality is a quantitative measure of reservoir heterogeneity. Heterogeneity and reservoir quality of sandstone reservoirs often exist in different degrees and scales (Halisch et al., 2009; Dutton and Loucks, 2010; Makeen et al., 2016). Heterogeneity of reservoirs is directly controlled by the sedimentary environment, lithofacies types, and mineral composition of river-controlled deltas (Nichols, 2009; Morad et al., 2010; Umar et al., 2011). The type of particles, type of pores, and the degree of pore development are important factors that impact the overall reservoir quality (Gong et al., 2019; Nabawy et al., 2023). In low-permeability sandstone reservoirs (reservoir permeability ranges from 1 to 10 mD), these sedimentary features at different scales control the reservoir heterogeneity and reservoir quality. The reservoir quality varies among different sedimentary units, depending on their sedimentary hydrodynamic conditions and sedimentation period, even though they were deposited in similar environments (Munawar et al., 2018; Fang et al., 2022). Therefore, reservoir quality controlled by sedimentary heterogeneity has always been an important basic question for exploring potential oil and gas reserves and developing oil and gas reservoirs (Becker et al., 2019; Aro et al., 2023). However, little is known about the complexity of sedimentary characteristics (including lithofacies analysis, microfacies analysis, microfacies distribution, and sedimentary environment) that need to be studied at the development scale in low-permeability reservoirs, and further research, description, and characterization are needed. Previous studies on the sedimentary characteristics of oil and gas reservoirs within the designated study area often roughly divided the reservoirs into underwater distributary channels or mouth bars (Zhao et al., 2012; Hao et al., 2014). Combining detailed facies analysis, sedimentary environments, and research on mineral composition will help effectively describe the characteristics of reservoirs in the Wuliwan area (Turner et al., 2024).
This paper comprehensively analyzes the sedimentary characteristics and reservoir heterogeneity by using core, cable logging, and casting thin-section data, and the research scale of sedimentary characteristics is further accurate to lithofacies. We subdivided a variety of sedimentary microfacies, clarified the reservoir quality differences caused by sedimentary heterogeneity during development, and clearly solved the complex interaction and genetic relationship between the two. Understanding the sedimentary characteristics of low-permeability reservoirs and the control of sedimentary characteristics on reservoir heterogeneity can provide new ideas for oil and gas field development and effectively guide the adjustment of further development policies.
2 Geological setting
2.1 Tectonic setting
The Ordos Basin, with an area of approximately 26 × 104 km2, located in northern-central China, is the second largest sedimentary basin in China. The Helan, Liupan, Qinling, Luliang, and Yinshan mountain ranges form the boundaries of the basin in four different directions, respectively. In addition, the overall structural features are rectangular in the north–south direction (Johnson et al., 1989; Yuan et al., 2007; Zhang et al., 2018). The Ordos Basin consists of six first-rank tectonic units: the Yimeng High in the north, the Weibei High in the south, the Jinxi Folded Belt in the East, the Yishan Slope in the center, and the Tianhuan Depression and the Western Thrust Belt in the West (Luo et al., 2007). Wuliwan Oilfield in the research area is located in Jingbian County and Zhidan County, Shaanxi Province (Figure 1).
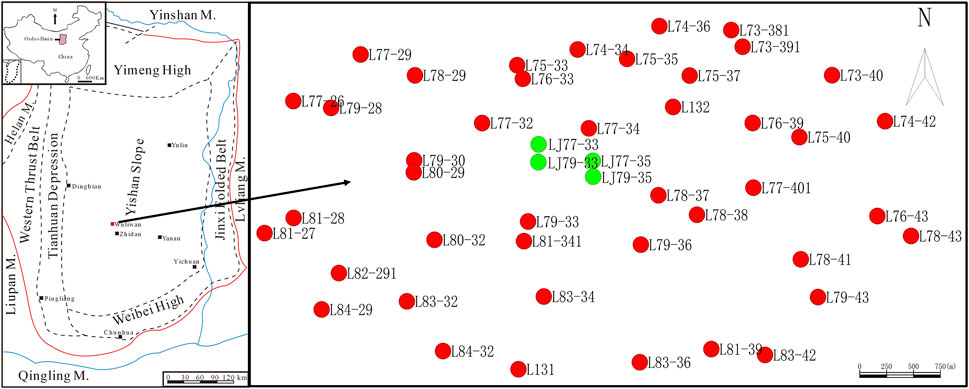
Figure 1. Present locations of the Ordos Basin: the red dot represents Wuliwan (modified from Xiao et al., 2019), and the green dot represents the coring wells.
2.2 Stratigraphic framework
The climate is and humid during the Middle and Late Triassic periods; the Ordos Basin gradually evolved into an inland freshwater lake basin (Ji et al., 2006; Ji et al., 2008). The Upper Triassic Yanchang Formation, which was deposited in the delta–lacustrine sedimentary system, can be divided into 10 intervals from top to bottom (Chang 1–10) determined by the evolution of lake deposits in the Late Triassic (Yao et al., 2009). Sections Chang 9 and 10 were deposited during the expanding stage of the lake basin. When Chang 7 section was deposited, the lake expanding evolution reached its peak stage. Subsequently, delta sedimentation gradually extended toward the center of the basin, and the lake basin significantly shrank during the deposition period of sections Chang 4, 5, and 6. Toward the end of the Late Triassic, when sections Chang 1, 2, and 3 were deposited, the base of the basin rose and the lake disappeared (Li et al., 2009; Lin et al., 2008; Guo et al., 2014). The target formation for the study is the Chang 6 member of the Wuliwan Formation (Figure 2). The reservoir is controlled by the provenance in the northeast and the delta front subfacies. The primary sand belt is distributed in a northeast to southwest direction, with the porosity range of 10%–12% and an air permeability range of 0.6–2.0 mD.
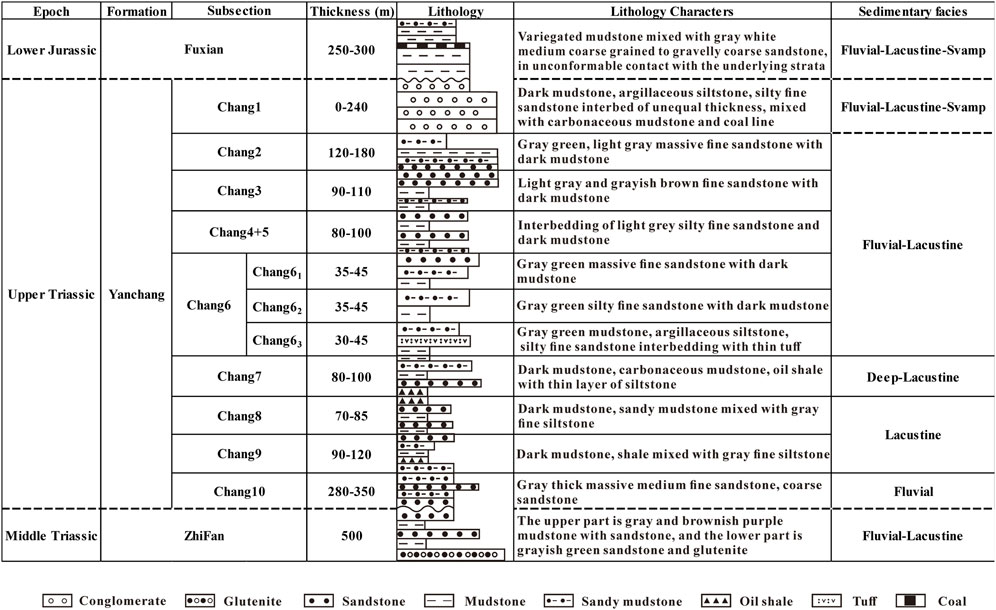
Figure 2. Upper Triassic and Lower Jurassic stratigraphy and depositional environment of the Ordos Basin (modified after Guo et al., 2014).
3 Samples and methods
Research was carried out and existing data, including core, wireline logging, and cast thin sections, from PetroChina Changqing Oilfield Company, were collected. The core comes from four coring wells located in the Chang 6 member (Figure 3). Cable logging consists of several conventional logging methods, including gamma ray (GR), surface potential (SP), acoustic travel time (AC), and density (DEN). The data of casting thin sections are mainly based on the impregnated red dyed epoxy thin sections obtained from the LJ75-61 well. However, this article mainly uses GR curves obtained from 86 wells, with a sampling rate of 0.125 m. After considering the influence of the environment and equipment, the GR curves in the study area were calibrated and normalized.
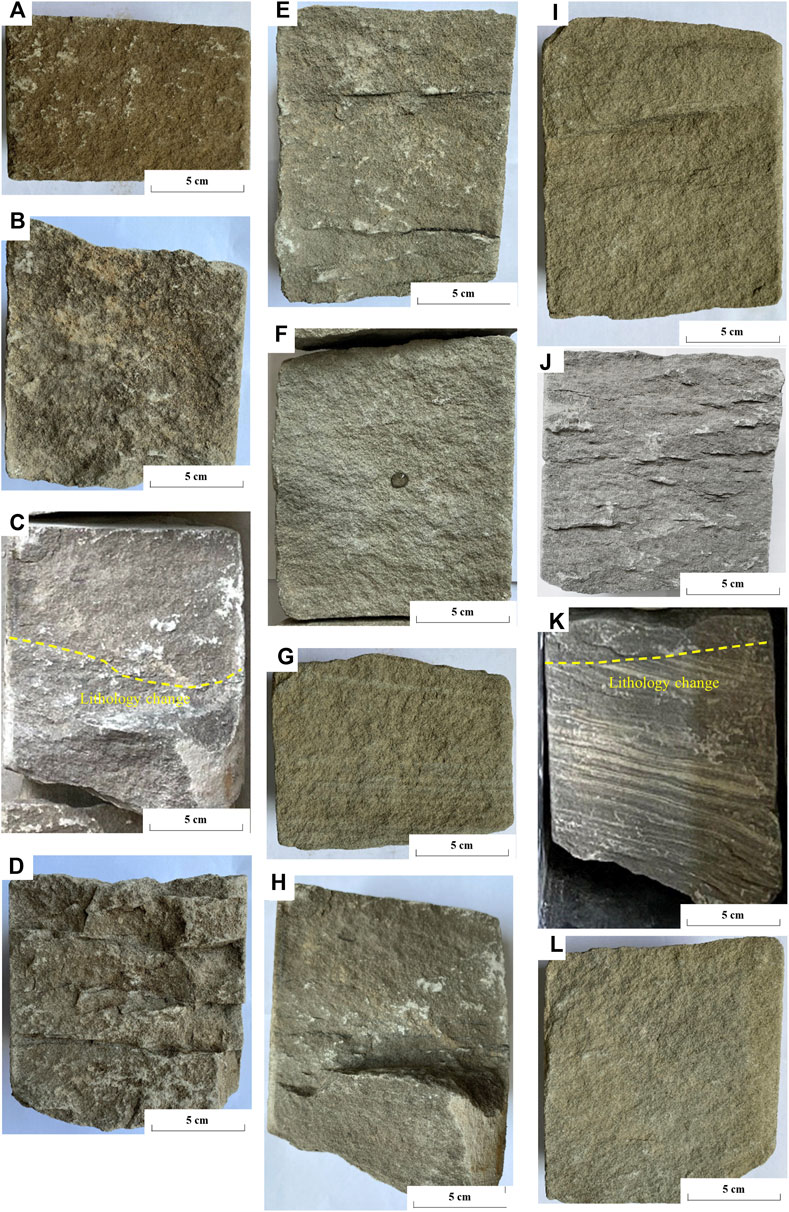
Figure 3. Cores showing sedimentary structures or lithofacies identified as the coarse-grained sandstone facies group in the Chang 6 member: (A) massive bedding fine sandstone, (B) tuffaceous sandstone, (C) interdistributary bay mudstone and underwater distributary channel sandstone lithology change interface, (D) massive bedding fine sandstone, (E) positive rhythmic bedding fine sandstone, (F) calcareous cementation phenomenon, (G) parallel bedding fine sandstone, (H) reverse rhythmic bedding fine sandstone (I) trough cross-bedding fine sandstone, (J) climbing bedding siltstone, (K) corrugated bedding siltstone, and (L) massive bedding siltstone.
The research method is based on specific data obtained from core and wireline logging, using knowledge of the structural background and sedimentary environment to analyze the facies distribution of the target interval and its impact on relative reservoir heterogeneity. The macroscopic characteristics include color, structure, and lithology exhibited by the core through core analysis, and the sedimentary environment and process of different reservoirs were analyzed. Then, combined with the microscopic characteristics of the cast thin section, a facies analysis of the reservoir is carried out to clarify the sedimentary structure and physical characteristics of different sedimentary microfacies. The sedimentological interpretation of cores (mainly lithology associations, sedimentary textures, and structures) and wireline logs (mainly stacking patterns) assists in identifying sedimentary facies and facies associations and predicting facies distributions (Xia et al., 2015). Based on the changes of lateral and vertical facies, the corresponding sedimentary model is established and the distribution of sedimentary facies is described. Finally, by interpreting single-well facies and reservoir dynamics, the potential for development of the Chang 6 reservoir was analyzed.
4 Results and interpretations
4.1 Subsurface facies analysis from conventional cores
Core analysis is the most authentic reflection of reservoir sedimentary heterogeneity (Worden et al., 2018; Soltani et al., 2019). Through the description and interpretation of conventional cores, the lithofacies combination of the work area was analyzed. Core description and interpretation reveal the lithofacies characteristics of the Chang 6 member section in the Wuliwan area. According to the difference in lithology and vertical distribution, the lithofacies can be divided into eight lithofacies: massive fine sandstone facies, positive rhythm fine sandstone facies, parallel bedding fine sandstone facies, reverse rhythm fine sandstone facies, trough cross-bedding fine sandstone facies, massive bedding siltstone facies, wavy cross-bedding siltstone facies, and climbing bedding siltstone facies. The characteristics of lithofacies are analyzed in detail based on lithological characteristics, hydrodynamic conditions, sedimentary environment, and sedimentary process.
4.1.1 Massive fine sandstone
4.1.1.1 Description
The massive fine sandstone facies in Wuliwan are generally brown, gray, or grayish white, with less mud. The sandstone is fine-grained and well-sorted (Figure 3A). In general, it is a homogeneous rhythmic cycle, usually formed through the superposition of several cycles. The sedimentary thickness is large, ranging from 0.8 to 4 m, and the bottom of a single cycle is often characterized by scouring on the underlying basement. The thickness of this lithofacies is different. It is usually shown as unstructural layered sandstone and occasionally tuffaceous sandstone (Figure 3B).
4.1.1.2 Interpretation
Due to the high quartz content of the parent rock, the debris particles produced by mechanical weathering are transported to the delta front deposition, and the gray and grayish white inherited colors of the massive sandstone facies on the core are obtained. Another part of the brown and brown massive sandstone facies reflects that the reservoir has a strong oxidizing environment during deposition, indicating that the water body in the delta front sedimentary environment of the study area is shallow. The fine sandstone belongs to the coarsest sandstone facies in the study area, reflecting the most intense and stable hydrodynamic characteristics of the delta front. At the bottom of the sandstone layer, the objects carried by the water flow repeatedly collide with the floor to form a stable scour basement (Figure 3C). Therefore, the existence of massive fine-grained sandstone can be interpreted as a sandy pure underwater distributary channel can be supported (Figure 3D). Occasionally, tuffaceous sandstones are observed in the massive fine sandstone facies, which reflects the magmatic activity in the Chang 6 member saline water. The tuffaceous sandstones are formed by condensation within the blocky, fine-grained sandstones (Table 1). Tuffaceous sandstone may contain delicate diagenetic clay, which can further degrade reservoir permeability in the subsurface, particularly in fine-grained sandstones, but the phenomenon of tuffaceous material in fine sandstone is extremely rare.
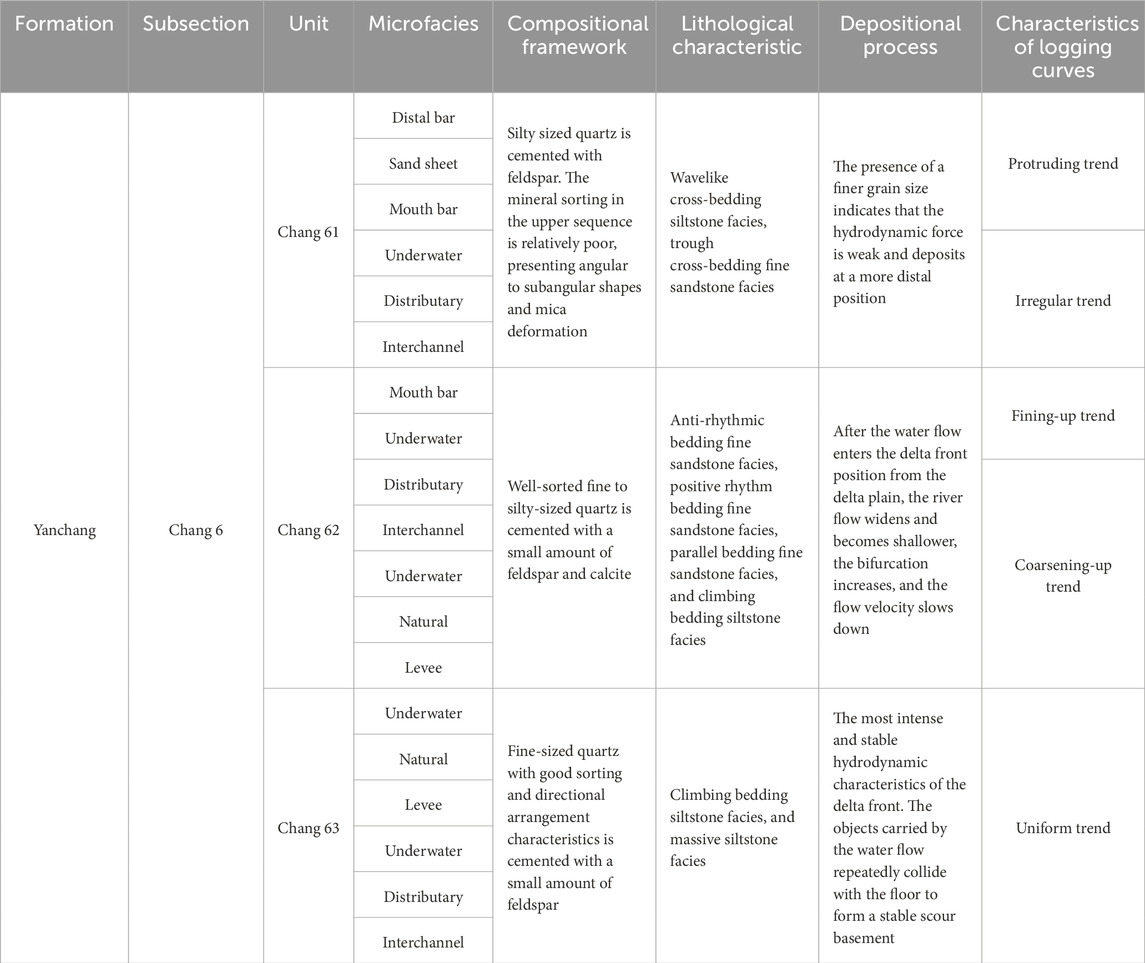
Table 1. Summarized description of the litho and microfacies and the depositional processes and complex of Chang 6 formation.
4.1.2 Positive rhythm bedding fine sandstone facies
4.1.2.1 Description
The fine sandstone facies of positive rhythm bedding are mostly brown. The erosion base can be seen at the bottom, and the sedimentary grain size is slightly finer toward the top, which is shown as positive rhythm layered sandstone (Figure 3E). The thickness of the positive rhythm bedding fine sandstone facies on the core is large, ranging from 0.6 to 4 m. There is often calcareous cementation at the bottom of the sandstone layer, and conglomerate deposits are rare.
4.1.2.2 Interpretation
The observed positive rhythm layered sandstone with upward thinning of deposition is the result of the gradual decrease of water flow velocity or intensity. After the water flow enters the delta front position from the delta plain, the river flow widens and becomes shallower, the bifurcation increases, and the flow velocity decreases. The grain size of the positive rhythm sediment becomes finer upward, and the clastic particles deposited in the lower part are thicker, which leads to the flow of calcium-rich pore water along the reservoir with fresh water flow in the later diagenetic process. Coarse grain size and high physical properties facilitate easy flow to the lower part, contact with high salinity formation water, precipitate, and generate calcareous cement at the bottom (Figure 3F). The sedimentary characteristics of coarser sediment grain size and positive rhythm bedding can be explained as underwater distributary channel microfacies. The phenomenon of rare conglomerate is due to the fact that after entering the sedimentary environment of the delta plain, the water flow rate decreases, and most of the conglomerates formed through the weathering of the parent rock have been deposited in the delta plain owing to a strong hydrodynamic force. The water flow within the underwater distributary channel can carry less gravel debris (Table 1). Therefore, compared with the deposition in the channel of the delta plain, a small amount of gravel carried by the distributary channel in the delta front can only have gravel deposition at the bottom.
4.1.3 Parallel bedding fine sandstone facies
4.1.3.1 Description
The thickness of the parallel bedding fine sandstone facies is relatively less, ranging from 0.6 to 1.5 m. It is brown, and the particle size is uniform. Parallel bedding composed of parallel lamellar fine sand and silt is developed (Figure 3G).
4.1.3.2 Interpretation
Parallel bedding sandstone commonly forms in the bottom of flat riverbeds due to the influence of hydrodynamic forces. The parallel bedding sandstone is generally deposited in the jet flow and high-energy environment. The relatively less thickness of the parallel bedding fine sandstone facies in the study area can be attributed to its formation as an underwater distributary channel at the delta front position during the rainy season. The increase in water volume and the faster flow rate are the lithofacies formed by deposition in the distributary channel.
4.1.4 Anti-rhythmic bedding fine sandstone facies
4.1.4.1 Description
The thickness of the fine sandstone facies with anti-rhythm bedding is medium, ranging from 0.5 to 3 m. Typically, the bottom is fine sandstone, and the grain size gradually increases upward (Figure 3H). The core is characterized by dark gray, less muddy, well-sorted fine sandstone facies, and calcareous cementation is common at the upper portion of the core.
4.1.4.2 Interpretation
The anti-rhythm bedding fine sandstone facies is regularly deposited within a sedimentary environment as a result of hydrodynamic force changes. The rhythmic characteristics reflect that the sediments are also affected by the periodic activities of rivers, waves, and tides, and the turbulent nature of the water environment. Most of sediments can be deposited by coarse-grained sandstone, so there is less mud and good sorting. It can be interpreted as a mouth bar near the shoreline. The sandstone at the top of the anti-rhythm fine sandstone has coarse grain size and good physical properties. Therefore, during the later diagenetic process, when the calcium-rich pore water flows along the reservoir alongside fresh water, it is easier to fill the upper part of the coarse grain size and high physical properties, precipitate, and generate calcareous cement at the top.
4.1.5 Trough cross-bedding fine sandstone facies
4.1.5.1 Description
The sedimentary thickness of the interlaced bedding siltstone facies is medium, ranging from 0.3 m to 1.5 m. The core is gray or brown, less argillaceous, well-sorted siltstone facies, mainly characterized by trough cross-bedding. Additionally, they possess a medium grain size (Figure 3I).
4.1.5.2 Interpretation
The trough cross-bedding reflects the strong hydrodynamic forces, indicating that the sandstone facies of the trough cross-bedding are deposited as a result of strong river channel erosion and are also affected by certain waves and tides. Due to the backing of the river water by the lake water, the muddy sediments are taken away by the lake water under the effects of scouring and bumping actions of the lake water, and pure sandstone is retained. The core exhibits the attributes of sand purity and effective sorting. It can be interpreted as mouth bar microfacies.
4.1.6 Climbing bedding siltstone facies
4.1.6.1 Description
The siltstone facies characterized by climbing bedding siltstone facies are mostly light brown, and the sedimentary thickness is relatively less, ranging from 0.2 to 0.5 m. The climbing bedding phenomenon is observed in siltstone with good sedimentary sorting and a large particle size has climbing bedding (Figure 3J).
4.1.6.2 Interpretation
The color of the climbing bedding fine lithofacies can indicate its characteristics of an oxidative sedimentary environment. The climbing bedding siltstone is often the product of sand wave migration. The climbing bedding siltstone facies in the study area are mostly well-sorted and coarse-grained siltstone, indicating that the sedimentary hydrodynamic forces are strong. The formation of climbing bedding indicates that a large amount of suspended matter is fully supplied during sand wave migration. The lithofacies is formed by the periodic rapid accumulation of sediments and the relative slowing down of flow velocity. It is usually developed in the underwater natural levee sedimentary environment (Table 1).
4.1.7 Wavelike cross-bedding siltstone facies
4.1.7.1 Description
The ripple bedding siltstone facies are mostly dark gray or black fine-grained deposits. The lithology is mainly siltstone, with high shale content and small sedimentary thickness, ranging from 0.3 to 0.8 m. It has clear wavy cross-bedding, showing parallel or non-parallel undulating surfaces (Figure 3K).
4.1.7.2 Interpretation
The profound hue of the wavelike cross-bedded siltstone reflects its long-term stability within an environment characterized by significant reduction processes. The presence of a finer grain size indicates that the hydrodynamic force is weak and deposits at a more distal position. The wavy bedding is formed by the migration of small-scale, non-linear flow ripples, which can be interpreted as sand sheet microfacies (Table 1).
4.1.8 Massive siltstone facies
4.1.8.1 Description
The massive siltstone facies exhibits a predominantly brown or grayish white color, with a lower proportion of shale. It also has a finer grain size and better sorting than the massive fine sandstone facies, which are generally a whole cycle of homogeneous rhythm. The sedimentary thickness is relatively less, typically ranging from 0.3 to 1 m, and it typically appears as unstructured siltstone (Figure 3L).
4.1.8.2 Interpretation
Similar to the massive fine sandstone, the massive siltstone phase also mainly shows the color inherited from its environment, and the brown hue resulting from a highly oxidizing environment. This observation provides evidence for the shallow water characteristics of the sedimentary environment within the designated study area. The siltstone is a fine-grained sandstone facies in the study area. It is considered to be formed by rapid deposition of suspended matter at the farthest end of the delta front as the flood enters the lake during the rainy season. This lithofacies sedimentary position is farther from the shore, where water flow slows down, and the hydrodynamic force becomes weaker. It can be interpreted as representing distal bar microfacies (Table 1).
4.2 Subsurface facies analysis from wireline logs
Several sets of wireline logs are commonly utilized in the interpretation of sedimentary facies, including GR, AC , and resistivity (RT). Gamma ray logs are analyzed for the purpose of detecting a clean interval (indication of more sandy) and a dirty interval (indication of more muddy). Given the fact that the GR value is high at muddy units and low at sandy units (Qiu et al., 2020), we can make appropriate interpretations. It is often difficult to identify the bedding type of lithofacies by using cable logging. Therefore, this paper summarizes and classifies the four characteristic types of cable logging curves observed in the study area and compares them with conventional core analysis results. At the same time, the results described by core observation are attributed to the logging curve, and five sedimentary microfacies logging curves are summarized (Figure 4). The method aims to identify the lithofacies type in a single well and summarizes the logging curve response types for each sedimentary microfacies.
4.2.1 Uniform trend
In the logging response, it is shown as GR high-amplitude box type, and the curve is generally homogeneous, reflecting the stable sedimentary environment with abundant source supply and rapid accumulation under stable hydrodynamic conditions (Figure 5A; Table 1). It is generally interpreted as underwater distributary channel deposition. The smooth curve can be interpreted as massive fine sandstone, while the curve with certain dentate characteristics can be considered horizontal bedding fine sandstone.
4.2.2 Fining-up trend
In the cable logging response, the trend of widening at the top, narrowing at the bottom, and an upward tail end is called the bell superposition mode, indicating that the sedimentary hydrodynamics of the sediment from bottom to top is weakened, and the upper clay content is increased (Figure 5B; Table 1). The specific performance observed is that the gamma logging value increases, and the core is mostly positive rhythm bedding of fine sandstone after homing. In Chang 6, the thick sands with bell-shape/cylinder-shape stacking patterns which indicate strong and stable hydrodynamics are commonly interpreted as underwater distributary channels of the delta front.
4.2.3 Coarsening-up trend
The coarsening trend of funnel-shaped superposition in the cable logging response indicates that the bottom-up hydrodynamic force of the sediment is enhanced. With the enhancement of hydrodynamic force, the upper sand is more pure, and the gamma logging value and clay content are gradually reduced (Figure 5C; Table 1). The logging response observed in the Chang 6 member shows a funnel-shaped stacking coarsening trend of this obvious anti-rhythmic deposition. After the core is returned, it is an obvious anti-rhythmic bedding fine sandstone facies, trough cross-bedding fine sandstone facies, or wavy cross-bedding siltstone facies. Through statistical classification, the smooth curves were found to be mostly proximal mouth bar deposits of anti-rhythm layered fine sandstone facies. The curve dentate is obviously interpreted as the proximal mouth bar microfacies of trough cross-bedding; the sand sheet microfacies has obvious curve dentate and low amplitude (Figure 5D; Table 1).
4.2.4 Protruding trend
The corresponding logging is peak shape, indicating that the sedimentary hydrodynamic force is strong and the sedimentary grain size is coarse (Figure 5E; Table 1). It is in line with the characteristics of strong river erosion of underwater natural levees, forming fine-siltstone deposits with thin sedimentary thickness and small development scale. After conducting core homing, it has been confirmed that the observed curve shape can be interpreted as a sedimentary microfacies characteristic of an underwater natural levee.
4.2.5 Irregular trend
The logging response shows an irregular trend, which usually exists in the case of thin sandstone or interbedded mudstone and sandstone interbedded (Figure 5F; Table 1). At this time, the sedimentary hydrodynamic force is weak or unstable, indicating that the sedimentary position is far away from the shore and is affected by rivers, tides, and waves. In most areas of the Chang 6 member, the sedimentary thickness is medium, and the logging curve shows an irregular trend, which can be interpreted as a sand sheet microfacies with far end and sand mudstone interaction. The irregular trend of extremely thin layers is generally massive bedding siltstone following core homing. This can be interpreted as a distal bar according to the vertical superposition mode.
4.2.6 Measurement of conventional cores and gamma ray value
The calibration of GR logs with conventional cores is conducted in Chang 6. Core description is used to assist wireline logging to determine and validate facies interpretation. The calibration work uses the logging data of conventional core measurement to calibrate the cable gamma logging. It can be seen that the phase group determined by the Chang 6 member clearly calibrated cable logging (Figure 4). The lithofacies within the core changes rapidly in the vertical direction, and the core calibration can be carried out well by using such characteristics. In the GR curve response, the upper amplitude is often greater than the lower logging response amplitude in the same isochronous interface between mudstones, indicating that the core from the bottom to the top is the process of transition from siltstone phase to fine sandstone, which verifies the hydrodynamic enhancement. It shows that the top of the vertical tends to develop more near-shore microfacies.
4.3 Sedimentary facies associations
The analysis of sedimentary microfacies is mainly carried out through the description and interpretation of conventional cores. The core description and log facies analysis reveal the characteristics of sedimentary microfacies within the Chang 6 member. Previous research has indicated that the delta front subfacies are mainly developed in the study area. Through analysis of cast thin sections, the sandstone of the Chang 6 member is mainly composed of minerals such as feldspar and quartz. Generally speaking, sandstone is relatively mature in mineralogy. The sandstone within the studied interval has a high degree of vertical heterogeneity. Through core observation, analysis of cast thin sections, and well logging facies analysis, the Chang 6 member can be further divided into underwater distributary channels, mouth bars, sand sheet, distal sand bars, and underwater natural levee microfacies based on the differences observed in lithologic composition and vertical distribution.
4.3.1 Underwater distributary channel
According to previous studies, when sedimentation occurs in this area, it has the characteristics of low accommodation space and sufficient source supply (Lin et al., 2008). Mainly developed in the vertical block, the top is flat and the bottom is convex lenticular, with large distribution of river deposits on the plane. This kind of microfacies has the widest distribution and the largest development scale in the study area. Due to the strong hydrodynamic characteristics of the sedimentary environment, the underwater distributary channel tends to deposit thicker and coarser sediments. Microscopically, the underwater distributary channel at the bottom is composed of a massive fine sandstone. It consists of large and fine-grained quartz particles cemented together with a small amount of feldspar and rock debris. The debris is distributed in a directional manner, mainly cemented by a chlorite film, and intergranular pores are developed (Figures 6A, B). Therefore, based on a fundamental perspective, the underwater distributary channel deposits in the Chang 6 member of the Yanchang Formation are mainly brown–gray massive fine sand, with less shale content. In the central position of the delta front, most of them are positive rhythm bedding fine sandstone facies or block bedding fine sandstone facies, which are the main sand body types of the delta front subfacies, with the largest thickness within each microfacies. On the thin section of the casting body, larger and oriented debris particles are also visible, with calcite cementation present in some areas (Figure 6C). The physical properties of this type of microfacies are superior to those of other microfacies in the study area because their intergranular pores are more developed. In addition, the strong and stable hydrodynamic erosion can lead to the formation of parallel bedding fine sandstone facies. This type of underwater distributary channel has slightly poorer physical properties than the first two. It exhibits mica deformation in the cast thin section, is mainly composed of intergranular pores, and shows an uneven distribution of pores (Figure 6D). The underwater distributary channel on the core is often composed of these three lithofacies (Figure 7).
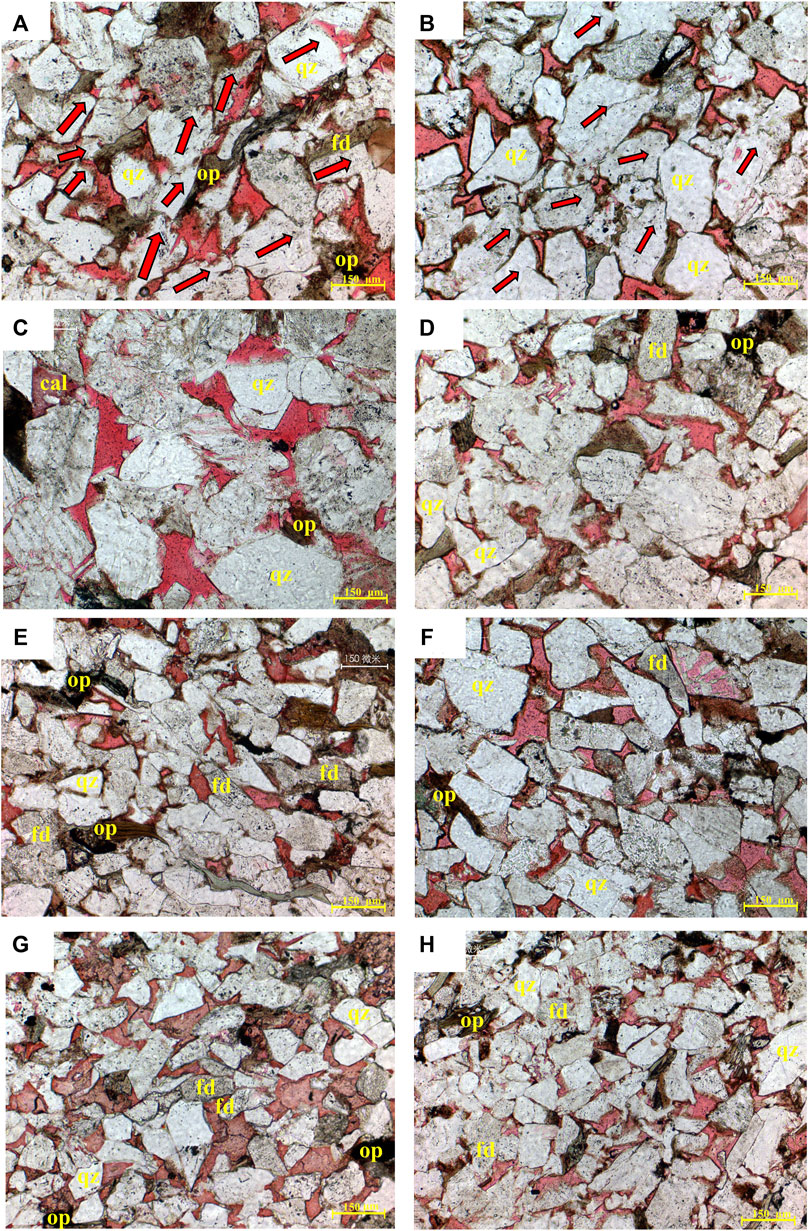
Figure 6. Thin-section samples from LJ75-61 and LJ75-60, the depth from h to a gradually deepens; qz, quartz; fd, feldspar; op, oil patch; cal, calcite.
4.3.2 Underwater natural levee
It is developed on the side of the underwater distributary channel under the background of the delta front. It has been scoured by water for a long time. The sedimentary environment is turbulent, and the hydrodynamic force is strong. Therefore, the sediment thickness is less, but the grain is coarse (Figure 7). The underwater natural levee deposits on the core are mainly climbing bedding, with the lateral and underwater cross-shore deposit transition. At the microscopic scale, it is a combination of fine to silty sized quartz, some feldspar, and rock fragments cemented together (Figure 6E). The underwater cross-shore deposits in the study area are less observed on the core and logging curves. It can be inferred that the position is located near the lake of the delta front as a whole, with the influence of the river being predominant. The seasonal flood action causes less crevasse or overflow and is scoured, eroded, and covered by the later developed underwater distributary channel tributaries.
4.3.3 Mouth bar
The typical mouth bar sand body is typically lenticular, and there is obvious erosion occurring at the bottom. The proportion of front mud is less. On the core, it is mainly composed of pure and well-sorted silt and fine sand, and trough cross-bedding is visible. Or from bottom to top, the grain of the sediment becomes coarser, mainly manifested as anti-rhythm bedding fine sandstone facies (Figure 7). Similar to the underwater distributary channel in the middle of the Chang 6 member, the main component of the estuary dam is quartz of fine sand size cemented with feldspar and rock debris, with relatively developed intergranular pores. Therefore, it also has the characteristic of relatively good physical properties (Figure 6F). The near-end mouth bar microfacies is a sedimentary microfacies developed under the strong action of hydrodynamic forces between river water and lake water when the river enters the lake.
4.3.4 Sand sheet
The sand sheet is often located far in front of the mouth bar. A transitional sedimentary microfacies between the mouth bar and the far sand bar is mostly a sheet or banded deposits that are transported toward the front of the mouth bar by the intermittent strong river action. Compared to the mouth bar, the grain size of the sand sheet is finer, and the thickness is less than that of the near mouth bar. The casting thin section clearly shows the characteristic of particle reduction, with the main body composed of sandy quartz and certain intergranular pores (Figure 6G). The distribution of intergranular pores is influenced by particle arrangement. The sand sheet is mainly composed of silt, which is mainly characterized by the sedimentary characteristics of sand–mud interbedding, and is characterized by wavy cross-bedded siltstone facies on the core (Figure 7). The development of a sand sheet reflects that the study area is biased toward a constructive delta, and the Chang 6 period is in a regressive environment.
4.3.5 Distal bar
The distal bar is situated at the end of the river channel. The sand body is very thin, which is a thin layer of the siltstone phase. Due to the long distance from the shore, the flow velocity decreases, and the water body is deeper. Therefore, the distal bar is often developed in the sedimentary environment with weak river action and less influence of wave and tide. The sedimentary environment is stable, and the hydrodynamic force is weak. The core shows a thin layer of massive sandstone siltstone facies (Figure 7). In addition, it is believed that the reservoir quality of the Chang 6 member is mainly controlled by the type and distribution of sedimentary microfacies. Core and wireline logging indicate that fine sandstones with positive rhythm characteristics can be interpreted as underwater distributary channels, indicating a gradual decline in hydrodynamic forces within the vertical sequence. The cast thin sections of Chang 6 member at different depths show that the minerals found in the lower and middle sequences are well-sorted, presenting subangular to sub circular shapes, with minerals arranged in a directional manner, and residual intergranular pores accounting for approximately 95%, reflecting relatively strong and stable fluid dynamics (Figure 6B; Table 1). In contrast, the mineral sorting in the upper sequence is relatively poor, presenting angular to subangular shapes, mica deformation, and residual intergranular pores accounting for approximately 80% of the total. Some micropores are also developed, with uneven pore distribution, indicating weak and unstable fluid dynamics (Figure 6H; Table 1). The distal bar microfacies developed at the top position exhibit powdery sand particle cementation on the cast thin section, with fewer pores developed, which significantly impacts the reservoir’s quality. Therefore, it can be seen that the difference in fluid dynamics leads to different sedimentary characteristics and controls the heterogeneity of the reservoir.
4.4 Sedimentary modeling and evolution
The summary of sedimentary characteristics of the Chang 6 member is as follows (Figure 8; Table 1): During the sedimentary period of the Chang 6 member, the accommodation space is small, and the underwater distributary channel is widely distributed on the delta front. Due to the decline of the base level, the available accommodation space is small and the supply is sufficient. Therefore, the delta front is dominated by river action and is a highly constructive delta sedimentary model (Rood et al., 2019; Dou et al., 2022). At the position where the river enters the lake, the underwater distributary channel of the delta front gradually bifurcates and migrates, and the accumulated sand bodies gradually expand. During the deposition process, the channel sand body has the characteristics of vertical superposition in the vertical direction, and the horizontal direction is mainly the mutual superposition of underwater distributary channels and sand dam. The underwater distributary channel is mainly filled with fine sandstone and the thickness decreases laterally. It is the most important and widely distributed sedimentary microfacies and a high-quality reservoir in the study area. However, it has formed low-permeability reservoirs due to the filling and plugging of pores by transported fine-grained sediments, which reduces porosity and permeability and calcareous cementation during diagenesis. The decrease in accommodation space during the Chang 6 period led to slow accumulation and extensive superposition of underwater distributary channels on the plane, and the channel sand bodies on the profile were vertically stacked. The composite superposition of different genetic sedimentary bodies leads to the development of the Chang 6 reservoir with strong heterogeneity.
4.5 Facies distribution and reservoir heterogeneity
Based on the interpretation of conventional core and cable logging, the distribution of sedimentary microfacies can be predicted. After identifying the horizontal and vertical phase changes, it provides a basis for identifying reservoir geometry, sealing characteristics, sedimentary layers, and determining reservoir heterogeneity.
On the vertical sequence scale of approximately 150 m covering the Chang 6 member, the microfacies from the same period of deposition overlap each other in the vertical direction according to the law of phase sequence. The sedimentary layer in the study area obviously begins with the thick distributary channel deposits in the lower part of the Chang 6 member, and the thickness of the single channel is approximately 1–5 m. The thickness of sandstone in underwater distributary channels is formed by the superposition of multiple channel sandstones. These channels develop in a highly constructive and small accommodating delta front sedimentary environment, providing a foundation and conditions for frequent channel diversion, migration, and movement. The middle of the Chang 6 member is the middle of the delta front and a large number of underwater distributary channels and mouth bars are superimposed on each other. The distal thin sand body is often located in the upper part of the Chang 6 member near the pre-delta, which is composed of thick vertical superimposed deposits dominated by mudstone and rare thin sandstone (Figure 9).
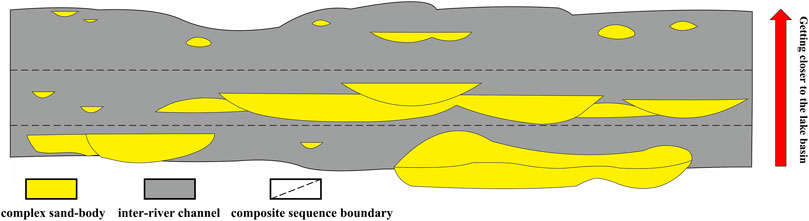
Figure 9. The spatial superposition relationship of composite sand bodies indicates that the connectivity of the middle and lower sand bodies in the Chang 6 member is high, while the connectivity of the upper sand bodies is low.
Based on the above methods, the logging interpretation and physical property analysis of 86 wells across the whole area are carried out. The analysis results show that the Chang 6 member mainly develops underwater distributary channels and mouth bar. Due to the strong hydrodynamic force present in the sedimentary environment of the two, the sediments are well-sorted and the grain size is coarse. Therefore, the physical properties of these two microfacies are better in the statistical results. The average porosity of the underwater distributary channel is 11.42%, and the average permeability is 2.74 mD; the average porosity of the mouth bar is found to be 11.57%, and the average permeability is 2.41 mD. The sedimentary thickness of the sand sheet is relatively medium, and the deposition is mostly good siltstone, with an average porosity of 10.53% and an average permeability of 1.55 mD. Underwater natural levees are mostly developed on the side of the river channel, with coarse sedimentary grain size, relatively less sedimentary thickness, and few sample points. The average porosity is 10.34%, and the average permeability is 1.34 mD. The average porosity of the distal bar is 9.90%, and the average permeability is 0.99 mD. The different sedimentary environments of each sedimentary microfacies lead to the difference in physical properties, and the complex superposition between different microfacies or the same microfacies leads to strong heterogeneity of reservoir in the vertical and plane of the study area. Through statistical analysis, it is considered that the physical properties of sedimentary microfacies are found to be best in the underwater distributary channels and mouth bar, followed by sand sheet microfacies and underwater natural levee microfacies, with distal bars having the worst physical properties (Figure 10).
4.6 Analysis of oil and gas potential
Through the analysis conducted in Sections 4.3 and 4.5, we found that among the three oil formations in Chang 6, the reservoir in Chang 62 is the most developed. The Chang 62 reservoir is a well-connected body formed by the combination of composite river channels and mouth bar, with good connectivity. In the thin section of the casting, larger particles, well-developed intergranular pores, and a small amount of calcite cementation are evident. The rock properties are relatively good, so during production, the oil saturation of this section is generally high (Figure 11A). The Chang 63 reservoir is characterized by a single, large-scale underwater distributary channel that is independently distributed. On the cast thin section, it exhibits large particles, an oriented arrangement, and a large number of intergranular pores. Therefore, the reservoir exhibits strong planar heterogeneity during production (Figure 11B). The Chang 61 reservoir is mainly composed of sand sheet and distal bar, with small particles at the microscopic scale; exhibits poor sorting; mica deformation; and undeveloped intergranular pores. The oil saturation of the Chang 61 section with poor physical properties is significantly lower than that in the first two. The reservoir exhibits strong heterogeneity, with the remaining oil concentrated in areas characterized by good physical properties (Figure 11C). In addition, the reservoir quality of Chang 63 and Chang 62 is better than that of Chang 61. After systematic research using the above methods, it was found that the perforation results of 86 single wells in the work area showed that the perforation intervals of the development wells were basically all located in Chang 62 of the main oil layer (Figure 12). The perforated sedimentary microfacies that have been perforated include an underwater distribution channel (85%), a mouth bar (12%), and a sand sheet (3%). The research results indicate that the initial reservoir development work mainly focuses on the underwater distributed channel microfacies with the best physical properties. When the current development enters the high water content level, the next development direction should focus on the non-main microphases of Chang 62, such as mouth bar and sand sheet with slightly poorer physical properties. These microfacies types of reservoirs should be areas of residual oil enrichment in the Wuliwan low-permeability reservoir in the later stage of development. In addition, within the range of oil formations, the research results show that the reservoir development quality of Chang 63 is slightly better than that of Chang 61. In addition, its development level is relatively low, which can serve as a replacement development layer of Chang 62. Therefore, it can be predicted that the remaining oil enrichment area of the Wuliwan low-permeability reservoir is located in the mouth bar and sand sheet microfacies of the Chang 62 section, as well as in the reservoir of the Chang 63 section. These reservoirs will be the focus of the next stage of oilfield development perforation method preparation and well location deployment.
5 Discussion
Generally, reservoirs developed in the sedimentary background of delta fronts controlled by rivers often have the best development potential in underwater distributary channels due to their large thickness, favorable physical properties, and strong lateral continuity (Ting et al., 2019; Xu et al., 2019). In the long-term development, this type of reservoir in the Chang 6 formation of the Ordos Basin has high water content and requires the replacement and development of different reservoir types (Doorwar et al., 2020; Jiang, 2020). The key issue in clarifying the potential of the remaining oil and gas is whether the characteristics of sedimentary evolution and heterogeneity effectively control the differences in various reservoirs and the distribution of remaining oil (Kra et al., 2022; Turner et al., 2024). The sedimentary heterogeneity characteristics from the study area down to the microfacies scale are still puzzling. The Chang 6 reservoir develops a sedimentary sequence from the nearshore end to the lake end, from bottom to top (Yuan et al., 2007; Zhang et al., 2018). In these sediments, even the microfacies of underwater distributary channels have different sedimentary characteristics in different locations (Yao et al., 2009). This article innovatively adopts a method in the research area, which combines core data, cable logging, and casting thin sections to study the sedimentary characteristics of reservoirs. On the basis of preliminary development research, this article aims to further subdivide the sedimentary characteristics of the Wuliwan low-permeability reservoir. The casting thin-section analysis method was innovatively adopted in the low-permeability oil reservoirs in the Wuliwan area to support the analysis results of core and logging. By combining three types of data, we have developed a method to improve the sedimentary model of low-permeability oil reservoirs situated in the delta front. The main five contents of the study are the combination of macroscopic core analysis and microscopic casting thin-section research to analyze the sedimentary characteristics of the study area and the combination of geological analysis and logging research to apply to single-well prediction. The research results indicate that sedimentary heterogeneity plays a controlling role in determining the characteristics of reservoirs. The changes in hydrodynamic characteristics and different sedimentary locations have led to significant differences in reservoir properties (Gong et al., 2019; Shehata et al., 2021; Nabawy et al., 2023). In the Chang 63 section, the underwater distributary channel is the best reservoir. Through statistical analysis of perforation information, the development level of the underwater distributary channel in this section is relatively low. In the Chang 62 section, the underwater distributary channel has been developed to a high extent and its potential concentrated in the microfacies of the estuary dam. The arrhythmic characteristics of the microfacies of the estuarine dam often lead to the presence of calcium cement in its upper part, which often needs to be avoided in the next stage of development (Yildiz, 2002; Bi et al., 2023). In addition, it was determined that the Wuliwan low-permeability oil reservoir has significant development potential primarily in the mouth bar microfacies of the Chang 62 section, as well as in the Chang 63 section oil reservoir.
In addition, this article reveals the impact of sedimentary heterogeneity on the quality and structure of low-permeability reservoirs, providing a case study that can provide a basis for other similar environmental studies. With the deepening of oilfield development, how to further subdivide the existing development units is the next issue that needs further discussion. Whether to continue classifying underwater distributary channels or to delineate their flanks and main bodies is the key to finding remaining oil.
6 Conclusion
(1) This paper provides a comprehensive case study on the influence of sedimentary heterogeneity on the quality and structure of low-permeability reservoirs, which can provide a basis for other similar environmental studies.
(2) According to conventional core analysis results, the Chang 6 reservoir was deposited on the delta front. Eight lithofacies were classified based on sedimentary grain size and sedimentary structure, including mass fine sandstone, positive rhythm bedding fine sandstone, parallel bedding fine sandstone, anti-rhythm bedding bin sandstone, rough cross-bedding fine sandstone, bedding siltstone, wavelike cross-bedding siltstone, and mass siltstone. This paper analyzes the characteristics of various lithofacies sedimentary environments, which provides evidence for the influence of sedimentary characteristics on reservoir heterogeneity.
(3) The lower part of the Chang 6 member is deposited on the nearshore end of the delta front, while the middle part is the main body of the delta front, and the upper part is deposited on the lake side of the delta front. It has developed five distinct sedimentary microfacies, including underwater distributary channel, mouth bar, sand sheet, distal bar, and underwater natural levee. Among them, the underwater distributary channel and mouth bar have the best physical properties. This result provides a basis for predicting the distribution of high-quality reservoirs.
(4) The distribution of sedimentary microfacies and sedimentary environment indicates that lake fluctuations and sediment supply play an important role in controlling the sedimentary accommodation space of the Chang 6 member.
(5) Sedimentary heterogeneity controls reservoir quality from macro to micro scale. From the perspective of macroscopic characteristics, the sand bodies in the lower and middle parts of the Chang 6 Member have good connectivity, while the sand bodies in the upper part have poor connectivity. Microscopic characteristics such as the type of the sand body and the distribution of sedimentary microfacies play an important role in controlling the porosity and permeability of the reservoir.
Data availability statement
The original contributions presented in the study are included in the article/Supplementary Material; further inquiries can be directed to the corresponding author.
Author contributions
XZ: conceptualization, writing–original draft, writing–review and editing, data curation, investigation, methodology, and resources. XS: formal analysis, funding acquisition, and writing–review and editing. YW: investigation and writing–review and editing. DY: writing–review and editing and data curation. GQ: writing–review and editing and resources. BF: writing–review and editing.
Funding
The author(s) declare that financial support was received for the research, authorship, and/or publication of this article. This research was funded by Research on Key Technologies and Solutions for “the ballast stone project” in aging oil fields, grant number 101023yq1002001b28.
Acknowledgments
State Key Laboratory of Enhanced Oil Recovery, the Third Oil Production Plant of Changqing Oilfield Branch of China National Petroleum Corporation and Petroleum Research Institute of Changqing Oilfield Branch are thanked for their kind cooperation and valuable inputs. In addition, the reviewers and executive editor are also thanked for their constructive comments which helped greatly improve the manuscript.
Conflict of interest
The authors declare that the research was conducted in the absence of any commercial or financial relationships that could be construed as a potential conflict of interest.
Publisher’s note
All claims expressed in this article are solely those of the authors and do not necessarily represent those of their affiliated organizations, or those of the publisher, the editors, and the reviewers. Any product that may be evaluated in this article, or claim that may be made by its manufacturer, is not guaranteed or endorsed by the publisher.
References
Aro, O. E., Jones, S. J., Meadows, N. S., Gluyas, J., and Charlaftis, D. (2023). The importance of facies, grain size and clay content in controlling fluvial reservoir quality - an example from the triassic skagerrak formation, central north sea, UK. Pet. Geosci. 29. doi:10.1144/petgeo2022-043
Becker, I., Busch, B., Koehrer, B., Adelmann, D., and Hilgers, C. (2019). Reservoir quality evolution of upper carboniferous (westphalian) tight gas sandstones, lower saxony basin, nw Germany. J. Petroleum Geol. 42 (4), 371–392. doi:10.1111/jpg.12742
Bi, G., Han, F., Li, M., Wu, J., Cui, Y., and Wang, X. (2023). Research on productivity prediction model of three-dimensional directional wells in different reservoirs. J. energy Eng. 149 (4), 149. doi:10.1061/jleed9.eyeng-4839
Chen, B. Y., Li, R. X., Liang, J. W., and Huang, K. Z. (2014). Authigenic chlorite and its impaction on reservoir physical properties: an example from the Yanchang formation of the southwest Ordos Basin. Bull. Mineralogy, Petrology Geochem. 33, 389–394. (in Chinese with English abstract). doi:10.3969/j.issn.1007-2802.2014.03.014
Doorwar, S., Purswani, P., Ambastha, A., Jain, L., and Thach, S. (2020). Application of tracer-based workflow for calibrating reservoir heterogeneity. United States: SPE Reservoir Evaluation & Engineering.
Dou, L., Wang, X., Liu, Y., Hou, J., Zhang, L., Liao, S., et al. (2022). River mouth jet plume related deposition in lacustrine shoal-water deltas:implications for reservoir characterization in lacustrine basins. J. Petroleum Sci. Eng. 219, 111011. doi:10.1016/j.petrol.2022.111011
Dutton, S. P., and Loucks, R. G. (2010). Diagenetic controls on evolution of porosity and permeability in lower Tertiary Wilcox sandstones from shallow to ultradeep (200-6700m) burial, Gulf of Mexico Basin, U.S.A. Mar. Petroleum Geol. 27, 69–81. doi:10.1016/j.marpetgeo.2009.08.008
Ehrenberg, S. N. (2019). Petrophysical heterogeneity in a lower cretaceous limestone reservoir, onshore abu dhabi, united arab emirates. AAPG Bull. 103 (3), 527–546. doi:10.1306/09061817298
Fang, Z., Chen, S., Pu, X., Yan, J., and Chen, X. (2022). Control of sedimentary environment on the lithofacies of lacustrine fine-grained sedimentary rocks in the second member of the kongdian formation in the cangdong sag, bohai bay basin, china. Geol. J. 6, 57. doi:10.1002/gj.4413
Gong, B., Keeledustin, J., Toumelin, E., and Clinch, S. (2019). Estimating net sand from borehole images in laminated deepwater reservoirs with a neural network. Petrophysics 60, 596–604. doi:10.30632/pjv60n5-2019a4
Guo, H. J., Jia, W. L., Peng, P. A., Lei, Y. H., Luo, X. R., Cheng, M., et al. (2014). The composition and its impact on the methane sorption of lacustrine shales from the Upper Triassic Yanchang formation, Ordos Basin, China. Mar. Petroleum Geol. 57, 509–520. doi:10.1016/j.marpetgeo.2014.05.010
Halisch, M., Weller, A., Sattler, C., Debschütz, W., and El-Sayed, A. M. A. (2009). A complex core-log case study of anisotropic sandstone, originating from Bahariya Formation, Abu Gharadig Basin, Egypt. Petrophysics 50, 478–497. doi:10.2451/2009PM0012
Hao, L. I., Shun-She, L., Wei, W., and Jun, L. (2014). Study on the sedimentary facies of chang-6 oil group of yanchang formation in fengdikeng-hongde area of ordos basin. Ground Water. doi:10.3969/j.issn.1004-1184.2014.06.086
Ji, L. M., Wang, S. F., and Xu, J. L. (2006). Acritarch assemblage in Yanchang Formation in eastern Gansu province and its environmental implications. Earth Sci. - J. China Univ. Geosciences 31, 798–806. (in Chinese with English abstract). doi:10.1007/s11442-006-0415-5
Ji, L. M., Zhu, Y. H., and Wang, S. F. (2008). Studies of modality assemble of Botryococcus from the Triassic Yanchang Formation in the Ordos Basin, Northwest China. Acta Palaeontol. Sin. 47, 185–194. (in Chinesewith English abstract). doi:10.1016/S1872-5791(08)60056-1
Jiang, Z. (2020). Using resistivity data to study the waterflooding process: a case study in tight sandstone reservoirs of ordos basin, china. Geophysics 86 (2), 1–65. doi:10.1190/geo2020-0401.1
Johnson, E. A., Liu, S., and Zhang, Y. (1989). Depositional environments and tectonic controls on the coal-bearing lower to middle jurassic yan’an Formation, southern Ordos Basin, China. Geology 17, 1123–1126. doi:10.1130/0091-7613(1989)017<1123:deatco>2.3.co;2
Kra, K. L., Qiu, L., Yang, Y., Yang, B., Ahmed, K. S., Camara, M., et al. (2022). Sedimentological and diagenetic impacts on sublacustrine fan sandy conglomerates reservoir quality: an example of the paleogene shahejie formation (es4s member) in the dongying depression, bohai bay basin (east china). Sediment. geology(Jan.), 427. doi:10.1016/J.SEDGEO.2021.106047
Li, W. H., Pang, J. G., Cao, H. X., and Wang, R. G. (2009). Depositional system and paleogeographic evolution of the late Triassic Yanchang stage in Ordos Basin. Journal of Northwest University (Natural Science Edition) 39, 501–506. (in Chinese with English abstract). doi:10.1042/BSR20080061
Lin, H. B., Hou, M. C., Chen, H. D., and Dong, G. Y. (2008). Characteristics and evolution of the sedimentary system of upper Triassic Yanchang Formation in Ordos Basin, China. J. ChengDu Univ. Technol. Sci. Technol. Ed. 35, 674–680. (in Chinesewith English abstract). doi:10.3969/j.issn.1671-9727.2008.06.011
Luo, J., Li, J., Yang, B., Dai, Y., Li, B., Han, Y., et al. (2007). Provenance for the Chang 6 and Chang 8 Member of the Yanchang Formation in the Xifeng area and in the periphery Ordos Basin: Evidence from petrologic geochemistry. Sci. China Earth Sci. 50 (S2), 75–90. doi:10.1007/s11430-007-6015-0
Ma, B., Cao, Y., Eriksson, K. A., and Wang, Y. (2019). Carbonate cementation patterns, potential mass transfer, and implications for reservoir heterogeneity in eocene tight-oil sandstones, dongying depression, bohai bay basin, china: evidence from petrology, geochemistry, and numerical modeling. AAPG Bull. 103 (12), 3035–3067. doi:10.1306/04101917330
Makeen, Y. M., Abdullah, W. H., Ayinla, H. A., Hakimi, M. H., and Sia, S. (2016). Sedimentology, diagenesis and reservoir quality of the upperabugabra formation sandstones in the Fula Sub-basin, Muglad Basin, Sudan. Mar. Petroleum Geol. 77, 1227–1242. doi:10.1016/j.marpetgeo.2016.08.015
Medici, G., West, L. J., and Mountney, N. P. (2019). Sedimentary flow heterogeneities in the Triassic UK Sherwood Sandstone Group: Insights for hydrocarbon exploration. Geol. J. 54, 1361–1378. doi:10.1002/gj.3233
Morad, S., Al-Ramadan, K., Ketzer, J. M., and De Ros, L. F. (2010). The impact of diagenesis on the heterogeneity of sandstone reservoirs: A review of the role of depositional facies and sequence stratigraphy. AAPG Bull. 94, 1267–1309. doi:10.1306/04211009178
Munawar, M. J., Lin, C., Chunmei, D., Zhang, X., Ma, C., Xiao, S., et al. (2018). Architecture and reservoir quality of low-permeable eocene lacustrine turbidite sandstone from the dongying depression, east china. Open Geosci. 10 (1), 87–112. doi:10.1515/geo-2018-0008
Nabawy, B. S., Aziz, E. A. A. E., Ramadan, M., and Shehata, A. A. (2023). Implication of the micro- and lithofacies types on the quality of a gas-bearing deltaic reservoir in the nile delta, egypt. Sci. Rep. 13 (1), 8873. doi:10.1038/s41598-023-35660-0
Nichols, G. J. (2009). Sedimentology and stratigraphy. 2nd ed. Oxford, England: Blackwell Scientific Publications, 419.
Qiu, Z., He, N., Wang, H., Li, X., Li, T., Zhou, Q., et al. (2020). Thesedimentological reservoir characteristics of the Jurassic Sangonghe Formation, Southern Mahu Slope, Junggar Basin,Northwestern China. Geol. J. 56, 1478–1495. doi:10.1002/gj.4014
Rood, S. B., Polzin, M. L., and Herbison, B. (2019). “Reservoir Regulation and Vegetation in the Draw-down and Delta Zones of the Duncan Lake Reservoir, British Columbia,” in Regulated rivers II: science, restoration, and management of altered riverine environments.
Schmid, S., Worden, R. H., and Fisher, Q. J. (2004). Diagenesis and reservoir quality of the Sherwood Sandstone (Triassic), Corrib field, Slyne Basin, west of Ireland. Mar. Petroleum Geol. 21, 299–315. doi:10.1016/s0264-8172(03)00187-9
Shehata, A. A., Kassem, A. A., Brooks, H. L., Zuchuat, V., and Radwan, A. E. (2021). Facies analysis and sequence-stratigraphic control on reservoir architecture: example from mixed carbonate/siliciclastic sediments of raha formation, gulf of suez, egypt. Mar. Petroleum Geol. 131, 105160. doi:10.1016/j.marpetgeo.2021.105160
Soltani, P., Sadeghnejad, S., Dehaghani, A. H. S., and Ashena, R. (2019). Quantitative monitoring of cleaning time and wettability alteration of carbonate rocks during soxhlet cleaning. SPE Reserv. Eval. Eng. 04. doi:10.2118/197066-pa
Ting, H., Youbin, H., Ning, W., Jixin, W., Yaxin, W., Geoscience, S. O., et al. (2019). Reservoir characteristics of the lower part of the 2nd member of shahejie formation in xia 32 block in linnan oilfield and their main controlling factors. J. Geol. doi:10.3969/j.issn.1674-3636.2019.01.013
Turner, R., Ahmed, M., Bissell, R., Prothro, L. O., Shehata, A. A., and Coffin, R. (2024). Structural and Stratigraphic Controls on Reservoir Architecture: A Case Study from the Lower Oligocene Vicksburg Formation, Brooks County, Texas. Mar. Petroleum Geol. 160, 106627. doi:10.1016/j.marpetgeo.2023.106627
Umar, M., Friis, H., Khan, A., Kassi, A., and Kasi, A. (2011). The effects of diagenesis on the reservoir characters in sandstones of the Late Cretaceous Pab Formation, Kirthar Fold Belt, southern Pakistan. J. Asian Earth Sci. 40, 622–635. doi:10.1016/j.jseaes.2010.10.014
Worden, R. H., Mohammed, B., and Philip, S. (2018). The effect of oil emplacement on quartz cementation in a deeply buried sandstone reservoir. AAPG Bull. 102 (01), 49–75. doi:10.1306/02071716001
Xia, S. Q., Li, Q., Wang, X. D., Sun, C., Wang, Y., Hu, Z., et al. (2015). Application of 3D fine seismic interpretation technique in Dawangzhuang Area, Bohai Bay Basin, Northeast China. Arabian J. Geosciences 8, 87–97. doi:10.1007/s12517-013-1225-6
Xiao, H. P., Liu, R. E., Zhang, F. D., et al. (2009). Sedimentary model reconstruction and exploration significance of permian He 8 member in Ordos Basin, NW China. Petrol. Explor. Dev. 46 (2), 280–292. doi:10.1016/S1876-3804(19)60008-X
Xu, S., Hao, F., Xu, C., Zou, H., and Gao, B. (2019). Seismic geomorphology and sedimentology of a fluvial-dominated delta: implications for the neogene reservoirs, offshore bohai bay basin, china. AAPG Bull. 103 (10), 2399–2420. doi:10.1306/0130191522517122
Yao, S. P., Zhang, K., Hu, W. X., Fang, H. F., and Jiao, K. (2009). Sedimentary organic facies of the Triassic Yanchang Formation in the Ordos Basin. Oil Gas Geol. 30, 74–84. (in Chinese with English abstract). doi:10.11743/ogg20090111
Yildiz, T. (2002). Productivity of selectively perforated vertical wells. Spe J. 7 (02), 158–169. doi:10.2118/78665-pa
Yuan, Y., Hu, S., Wang, H., and Sun, F. (2007). Meso-Cenozoic tectonothermal evolution of Ordos basin, Central China: insights from newly acquired vitrinite reflectance data and a revision of existing paleothermal indicator data. J. Geodyn. 44, 33–46. doi:10.1016/j.jog.2006.12.002
Zhang, C., Yi, C., Dong, Q., Cai, Y. Q., and Liu, H. X. (2018). Geological and geochronological evidence for the effect of Paleogene and Miocene uplift of the northern Ordos Basin on the formation of the Dongsheng uranium district. China. J. Geodyn. 114, 1–18. doi:10.1016/j.jog.2017.11.010
Keywords: low-permeability oilfield, Ordos Basin, Wuliwan, Chang 6 member, sedimentary characteristics, the feature of logging curves
Citation: Zou X, Song X, Wang Y, Yan D, Qin G and Fang B (2024) The controlling effect of sedimentary characteristics on the heterogeneity of the Chang 6 reservoir in the Wuliwan area. Front. Earth Sci. 12:1405111. doi: 10.3389/feart.2024.1405111
Received: 22 March 2024; Accepted: 27 May 2024;
Published: 26 June 2024.
Edited by:
Irene Cornacchia, Italian National Research Council, ItalyReviewed by:
Amer A. Shehata, Texas A and M University Corpus Christi, United StatesMuhammad Jawad Munawar, University of the Punjab, Pakistan
Copyright © 2024 Zou, Song, Wang, Yan, Qin and Fang. This is an open-access article distributed under the terms of the Creative Commons Attribution License (CC BY). The use, distribution or reproduction in other forums is permitted, provided the original author(s) and the copyright owner(s) are credited and that the original publication in this journal is cited, in accordance with accepted academic practice. No use, distribution or reproduction is permitted which does not comply with these terms.
*Correspondence: Xinzhe Zou, zouxinzhe@petrochina.com.cn