- 1Department of Environment, Land and Infrastructure Engineering (DIATI), Politecnico Di Torino, Torino, Italy
- 2Department of Civil, Environmental and Architectural Engineering (ICEA), University of Padua, Padua, Italy
The influence of climatic factors on landslides triggers and displacement rates is a crucial research topic, especially due to the growing need to understand the evolution of climate change in historical periods of intense precipitation and anomalous temperature increases. Italy, highly prone to hydrogeological instability, extremely its mountainous regions such as the Alps, stands as a pertinent subject area for instability scenarios. However, the interpretation of climate effects on landslides is still an open issue. This work proposed a simplified methodology for investigating the displacements of three slow-moving landslides located in the Western Alps of Piemonte region, in response to significant meteorological events evaluated from reference normal of precipitation and temperature trends over the reference period 1991–2020. Another purpose is to emphasize the advantages of using different monitoring techniques by comparing displacement time series measured with in situ and remote sensing instruments, to detect ground deformation processes of these gravitational phenomena. The existence of a robust monitoring network, coupled with InSAR dataset support, has allowed detecting climatic factors’ impact on displacement rates for the outlined case studies. The results have demonstrated the relationships between the identified climatic events and variations in displacement time series, as well as the potential of integrating field observations and InSAR techniques to improve the interpretation of landslide dynamics. Although this study has laid the basis for understanding the influence of climatic factors on landslide displacements, there is still much to investigate and refine. The proposed preliminary analysis will further improve the ability to predict, monitor and mitigate landslide risk under changing climate conditions.
1 Introduction
Mountainous regions are characterized by geological and morphological features that increase the susceptibility to a wide range of slope instabilities. In recent decades, there has been growing awareness of the profound influence that climatic factors, specifically heavy rainfall and anomalous temperatures, have on landslide movements in this context.
Evaluating the effects of climate change on landslides is still an open issue for the scientific community, partly because of problems in predicting the phenomena. Even more challenging is the quantitative assessment of future variations in the occurrence and the frequency of slope instabilities related to climate changes, in particular in rainfall regimes and patterns (Gariano et al., 2017).
Rising global temperatures is unequivocal (IPCC, 2021), and its effects have been observed in many regions, especially in the most vulnerable ones, such as mountain areas where water resources are threatened (Gizzi et al., 2023). Significant changes may also be expected in precipitation regime, since the capacity of air to hold moisture is a function of temperature. In general, global warming is therefore likely to lead to an overall greater frequency and magnitude of heavy precipitation events (IPCC, 2023).
The assessment of how climate variables could effectively influence slope instability mechanisms is therefore crucial in this framework.
Several authors have carried out research focusing on relationships between climate trends and landslide activations or changes in displacement rates. The studies revealed a wide range of impacts of climate variations on landslides, including contradictory, uncertain, and undetermined effects (Gariano and Guzzetti, 2016).
As an initial step, a whole understanding of the climate pattern in a given location is essential to identify possible series of anomalies with respect to reference values. In pursuit of this objective, the World Meteorological Organization (WMO, 2023) has defined climate normals with the aim of comparing observations and providing average values that are representative of the current or past climate, establishing a reference period of 30 years (WMO, 2017).
Over the years, various approaches have been proposed to evaluate long-term precipitation and temperature regimes and, in particular, their influence on slope instability. Nigrelli et al. (2021) have investigated the temperature indices of the European Alps in the period 1990–2019, revealing the identification of one or more climate anomalies possibly correlated with instances of ground instability in specific case studies. Paranunzio et al. (2019) performed a probabilistic analysis assuming a set of the most representative climate variables on the occurrence of slope failures at high-elevation sites. As a result, 92% of the case studies were attributable to climate anomalies.
In mountainous regions, the occurrence of landslides is influenced by the microclimatic conditions at different altitudes. For instance, the warming and thawing of permafrost may reduce the stability of rock slopes, leading to debris flow. Above the elevation of 1500 m, the expected decrease of snow season in future years will likely affect the frequency, number, and seasonality of landslide reactivations. Analysis of landslide areas in Piemonte (northwestern Italy) adds further evidence to the observed changes in triggering climatic conditions and their impact on landslide frequency. In this region, it has been demonstrated that landslides have become more frequent during the 21st century, particularly in early spring, often triggered by moderate rainfall (Stoffel et al., 2014).
On the other hand, slow-moving landslides (Cruden and Varnes, 1996) which advance at rates of a few millimetres to several metres per year, can persist for long periods of years or decades. While these gravitational phenomena rarely cause fatalities, they pose a significant threat to infrastructure and can escalate into fast-moving landslides causing widespread casualties (Lacroix et al., 2020). The enduring motion of these landslides offers a unique chance to detect landslide processes and mechanisms, governed by much more complex dynamics, as deformations in most cases depend on variations in the groundwater regime (Yokoyama et al., 2022). The rate of landslide movement is controlled, both in the accelerating and decelerating phases, by pore-water pressure variations that are, in turn, strictly correlated to the net rainfall regime and snowmelt. This results in a decrease in effective stress which in turn reduces the frictional force acting on the landslide body; thus, the mass mobility increases along the slip surface (Cascini et al., 2010; Handwerger et al., 2013). Due to the involvement of several features, including rainfall conditions, pore water pressure, soil strength and movement rates, a numerical model is the most efficient way to investigate the behavior of rainfall-induced landslide (Lee et al., 2021).
However, the proposed approach does not explain the slope deformation mechanisms from a geotechnical perspective, but it proposes a simplified model to recognize the relationship between climatic events and changing trends in complex landslide phenomena.
In this view, the role of the monitoring network is fundamental for understanding the spatial distribution and temporal evolution of displacements. The data acquired both in field and by remote sensing techniques are useful to detect mass movements, sometimes imperceptible, in response to predisposing factors, in particular landslides acceleration due to climate events occurrence.
Due to the complexity which characterize slow-moving processes, the InSAR approach stands out as a valuable analysis tool thanks to its spatial accuracy and high temporal resolution of measures (Cigna et al., 2011; Pedretti et al., 2023).
1.1 Aims of the study
Given the above considerations, this work aims to explore potential relationships among changes in climatic trends and landslide displacements within mountain basins in Piemonte Region (Italy), focusing on three case studies of slow-moving landslides, monitored for the presence of sensitive elements such as inhabited centers and roads. The analysis described in the methodology is subdivided in several steps (see Figure 1): (i) geomorphological framework of the study area and choice of landslide monitoring data; (ii) evaluation of temperature and precipitation time series over the reference 30-year period 1991–2020 of the study area; (iii) identification of significant events and anomalies with respect to the reference climate trends; (iv) effects of climatic factors on landslide displacements observed for each case study from field monitoring stations and InSAR. Finally, in the discussion section, the results obtained are argued and integrated with additional information concerning landslide areas to better understand the outputs of the analysis.
2 Materials and methods
For the analysis purposes, a characterization of the area of interest was set up by implementing a geodatabase in Geographical Information System (GIS) environment, capable of managing a large amount of collected spatial data, as suggested by Giardino et al. (2004). Geomorphological and climate data were included in the geodatabase, to provide a framework of the area in terms of landslide hazard (Audisio et al., 2017). Furthermore, for a detailed view of landslide deformations, displacement assessment included time series from regional monitoring network catalogues and satellite datasets with spatial and temporal information.
2.1 Study area
The study area is located in the Western Alps (Piemonte region, Italy) and involves the basins of the Dora Riparia (1,210 km2 within the Italian territory) and Chisone (604 km2) rivers, which belongs to the Pellice main basin (Figure 2). The whole area, with elevation ranging from to 211–3534 m a.s.l., is located within the Alpine environment particularly affected by rockfalls, large complex landslides and Deep-Seated Gravitational Slope Deformation (DSGSD) (Bordoni et al., 2023; Forno et al., 2016). The landslide risk is further aggravated by the high anthropogenic pressure from tourism and due to dense network of infrastructure, especially in the Susa Valley (Dora Riparia main basin), which includes the municipalities located on alluvial fans such as Bardonecchia, Oulx, Cesana Torinese, and Claviere. From a geomorphological point of view, both basins are characterized by the presence of lithotypes which, due to their geomechanical properties, are particularly prone to erosion phenomena and hydrogeological instability. Fractured metamorphic lithoids (mainly gneisses and schists) are widespread in the area, followed by massive lithoids, fractured sedimentary formations, glacial and alluvial deposits. The latter are more common in the valley bottoms and at the outlets of the main valleys.
In this context, the upper Susa Valley and the upper Chisone Valley stand out for an exceptional concentration of DSGSD and large landslides, accounting for 38% of the Western Alps, these valleys represent one of the best examples of the interaction between the recent tectonic evolution of the relief and the slope dynamics (Fioraso et al., 2010).
At least two-thirds of the municipalities belonging to the Dora Riparia and Chisone basins are exposed to high and very high hazard (Italian platform on hydrogeological instability–IdroGEO). Roto-translational slides phenomena and rockfalls prevail above 1500 m, due to the snow melting and ice thawing. In the medium-lower sector of the Dora Riparia basin, complex landslides and flows are widespread, involving more or less altered and disaggregated rock substrates.
From a climatic perspective, the study area is protected by the alpine chain from the Atlantic and the Mediterranean moist currents, which reduces rainfall in quantity and intensity. In the catchment areas of interest, average precipitation ranges from 800 mm/year in the plains to around 1000 mm/year at higher elevation (Autorità di Bacino del fiume Po, 2023a; 2023b).
Above 2000 m, precipitation is predominantly snowfall. The largest contribution of flood flows comes from the effect of snowmelt in the spring and summer seasons.
For the aims of the study, different types of landslides (DSGSD, roto-translational and complex landslide) embedded in this very fragile morpho-structural context will be analysed (Fioraso et al., 2011) whose movements are closely dependent on intense rainfall events and rapid snow melting.
2.1.1 Sauze d’oulx
The lower-middle slope of the Sauze d'Oulx and Oulx municipalities, situated on the topographic right of the Susa Valley, is affected by a DSGSD (Figure 3). This gravitational phenomenon, interesting an area of 5.4 km2 and ranging in elevation from 1087 m to 1768 m a.s.l., represents one of the largest landslides of the Western Alps. The morphological and hydrogeological attributes distinguishing the Sauze d'Oulx phenomenon exhibit certain differences compared to other DSGSDs in this area. The collapsed slope is characterized by a fan shaped morphology and it is subjected to a rapid evolution, movement similar to rock flow controlled by a deep-seated continuous creep process involving serpentinites. Geomorphologically, the deformation is characterized by numerous pre-existing shear surfaces, attributed to the prevalence of calcareous schist and serpentines, rendering the entire slope fractured and highly permeable. Consequently, surface water circulation is absent, and water infiltration contributes to an aquifer located at significant depth.
Over time, multidisciplinary approach has been developed to comprehend the kinematic evolution DSGSD. The existence of an integrated monitoring system, based on an inclinometer system, satellite and ground-based interferometric techniques and a network of GPS stations, has made it possible to define a kinematic model of the phenomenon, providing information on the horizontal and vertical components of surface movement (Fioraso et al., 2010). Specifically, the surveys demonstrated the existence of gravitational activity traces, both ancient and recent, due to the presence of scarps, counter-slopes and closed depletions. Deformation time series have been continuously monitored since January 1999 through a network managed by Arpa Piemonte, which has installed instrumentation in proximity of buildings with the aim to record damages due to movements.
2.1.2 Pragelato
In the Pragelato municipality, at Plan locality, there is an evident roto-translational slide phenomenon evolving into local flows (Figure 4), with an extension of 0.25 km2. In the upper slope, between 2075 m and 1940 m a.s.l., the substrate is intensely fractured up to the landslide detachment zone, this ensures a significant infiltrative contribution that nourish widespread water springs. The accumulation body ranges between 1940 m and the valley floor where the Plan locality is located (1680 m a.s.l.).
The evolution of the landslide body, at the bottom, directly involves the road sector of the regional road S.R. 23, for a length about 1300 m in correspondence with the hairpin bends that descend to the Pragelato plain. In fact, lesions are found on the retaining walls of the road massif with a width of the order of about 20 m. The landslide activity, monitored by ground-based instruments and satellite techniques, is given by the combination of two movements, starting with the upstream roto-translative sliding along some fractures in the substrate of calcareous schist, and developing into a flow in the accumulation zone, characterized by incoherent soils. The complexity of the phenomenon is therefore due to the complex geological characteristics of the slope.
2.1.3 Sestriere
The complex landslide involves the Sestriere municipality for 2 km2 and altitude between 1522 and 2064 m a.s.l. The phenomenon is connected to a large DSGSD extending to the top of the relief. The locality of Champlas du Col is included in the median sector of the landslide, while the western part of Sauze Cesana is located on the bottom of slope (Figure 5). Surface movements, such as flows and rotational slides, are very active and threat the S.R. 23, heavily damaged in spring 2017. The historical analysis allowed to recognize a complex system with multiple sliding surfaces. At the landslide top, the presence of counter-slope areas, the widespread state of fracturing of the rock and the snowfall allow for the infiltration of meteoric inputs. In the lower part of the landslide, the presence of fluvial deposits at a depth of 79–89 m is evident, demonstrating the advancement of the landslide along a sliding surface below the current valley floor. Upstream of the S.R. 23, movements occur along horizons with different permeability, due to the presence of gravel alternating with silt and sand. These incoherent soil deposits cover the fractured substrate with a thickness of about one hundred metres. As part of the project to upgrade the S.R. 23, a monitoring plan (starting in 2005) of the main landslides affecting this area, which had already been started previously (in 2001), was set up according to the directives of the Piemonte Region Technical Services. Concerning the interferometric data, several datasets are available in ascending and descending geometry, but the southern exposure of the slope does not allow a precise assessment of the magnitude of the surface displacements. Most of monitoring points are concentrated in proximity of Champlas du Col buildings and along the S.R. 23 for recurring damages caused by slow but continuous movements.
2.2 Data
2.2.1 Climate data
In order to evaluate climate patterns in the study area for the 30-year reference period 1991–2020 according to the WMO, temperature and precipitation data for Piemonte region were considered. The dataset, the NorthWestern Italy Optimal Interpolation (NWIOI), provides daily data of maximum and minimum temperatures and precipitation with a time coverage from December 1957 until the day before the data download and it is updated daily by Arpa Piemonte. The domain covers the whole region (longitude 6.5–9.5 W and latitude 44.0–46.5 N), with a regular grid (0.125° resolution). For the purpose of the study, the period from 1 January 1991 to 31 December 2020 was selected and the data grids were processed by MATLAB® computational software to extrapolate cells covering area of the Dora Riparia and Chisone basins (see Supplementary Material S1). For simplicity, the western extremity belonging to France are neglected. From the daily data, monthly values (mean for temperature and sum for precipitation) are calculated according to specific criteria, for which climate analysis requires the use of long series of quality-controlled, complete, and homogeneous data. The monthly normals are then used to calculate seasonal and annual normal (ISPRA, 2022). The spatial mean of each cell value was then calculated from the clipped area to obtain a daily mean of precipitation and temperature, maximum and minimum, for the whole domain. Finally, the annual values of the two variables were obtained by evaluating the cumulative and mean of the daily data, respectively for precipitation and temperature. In order to better assess the presence of possible anomalies in the precipitation regime and to identify the wettest years, days with heavy rainfall greater than 10 mm were extrapolated from the dataset. This approach allowed to detect the pattern of intense meteorological events over the period 1991–2020 and, in the next step, to investigate possible accelerations in deformations trend under climatic conditions of the wettest years. On the other hand, the climatic pattern of temperatures over the 30-year reference period is depicted, analyzing the deviations of annual mean temperatures from the reference normal, which is given by the average of annual temperatures in 1991–2020.
For the local-scale climate analysis of the sites, weather stations close to the landslides in both planimetry and elevation were considered. For case a, weather data are provided from the Chateau Beaulard station (1800 m a.s.l.), for case b the Pragelato weather station (1525 m a.s.l.) and for case c the Sestriere station (2020 m a.s.l.) (Figure 6). The database of the regional meteorological network can be consulted on the Arpa Piemonte geoportal.
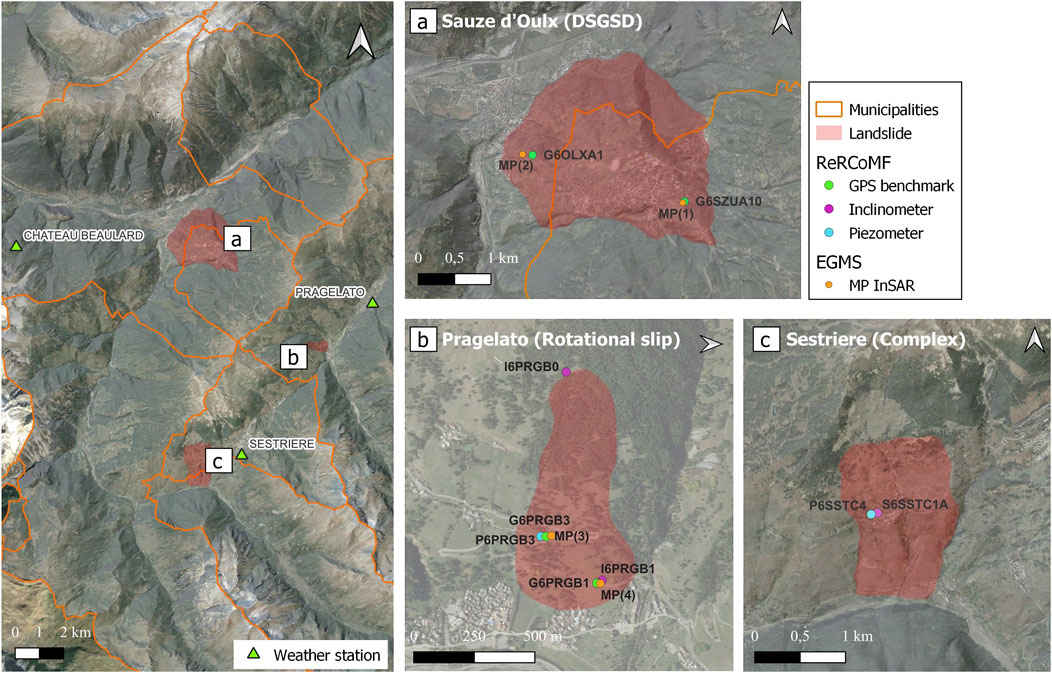
Figure 6. Details of the three sites (A–C) with corresponding weather stations (left) and monitoring points.
2.2.2 Landslides catalog
The distribution of landslides in basins territory is collected in the Landslide Information System in Piemonte (Sistema Informativo Frane in Piemonte - SIFraP) inventory, the database of landslides at regional scale. This system is composed of three levels of detail depending on the level of information available for each site. In particular, the case studies presented in this paper are categorized in the level II sheets so these are phenomena described in detail for morphological features and their continuous displacement monitoring. The catalog is available on the Arpa Piemonte geoportal and it contains all the landslide geometries that can be visualized and processed in GIS environment. Each landslide is identified by a code and details about the type of movement, state of activity, date of observation, and location are given (DSGSD - Sauze d’Oulx, Rotational slide–Pragelato, Complex - Sestriere).
2.2.3 Monitoring data
Since the 1990s, Arpa Piemonte has been monitoring about 220 landslide sites through the Regional Landslide Control Network (Rete Regionale Controllo Movimenti Franosi–ReRCoMF) for assessing time series of landslide displacements by using deep, shallow and strain gauge measurement points (SNPA, 2021). The database of instruments distinguished by typology, functionality and technical characteristics of the installation is available in the geoportal and, such as landslide areas, the monitoring network can also be imported into a GIS environment.
Displacement measures acquired by Global Positioning System (GPS) benchmarks, inclinometers, and piezometers were analysed in this work. These data with related technical reports have been provided from Arpa Piemonte upon request. The choice of instrumental data used for the three case studies was guided in relation to a suitable time coverage for the period of interest. In addition, some site was discarded a few years after their installation and therefore not considered. For instance, in the case of the landslides in Sauze d’Oulx and Sestriere, the GIS some inclinometers were active in 2002–2004 and several GPS stations have recently been installed, between 2017 and 2019, thus not useful for analysis. GPS data are processed for the Sauze d’Oulx and Pragelato landslides, while inclinometer data are considered for the Sestriere landslide. Furthermore, in support of the analysis, some additional information collected in the level II SIFraP report about the other instruments installed on the landslide body will be discussed. As it can be seen in Figure 6 the landslide areas and the distribution of monitoring points (Table 1) are shown.
In cases a and b, an integrated approach using satellite data was adopted, by comparing the information provided by Synthetic Aperture Radar Interferometry (InSAR) products with the GPS dataset. The joint analysis provided a comprehensive view of the deformation field of the landslide. An effective integration of monitoring data from different sources can be critical in reducing uncertainties, as individual monitoring techniques often have inherent limitations. Consequently, it can be difficult to achieve complete multi-parametric data coverage due to these isolated limitations. (Carla et al., 2019). The InSAR technique allows to monitor ground deformations and geological processes over extended areas, improving the conditions for slow-moving landslides detection (Cascini et al., 2010; Solari et al., 2019; Cignetti et al., 2023). SAR images are acquired by Sentinel-1 satellite since April 2014, which operates in C-band mode (wavelength of 5.6 cm) thus providing high spatial resolution data (Del Soldato et al., 2021). Time-series deformations are available on the European Ground Motion Service (EGMS) open platform of the Copernicus service (Shahbazi et al., 2022) and in particular, for the aim of this work, ortho data which constitute the EGMS level 3 product (after basic and calibrated data) were considered. They are the result of processing satellite data acquired in ascending and descending trajectory and therefore provide information on vertical and horizontal (east-west) displacements with a constant revisiting time of 6 days from January 2016 to December 2021. Both components are available in raster format with spatial resolution of 100 m and also in vector points (Measurements Points, abbreviated as MP) referred to the center of the resolution cell. For the purpose of the analysis, only east-west displacements are considered for comparison with the horizontal component of the GPS station records.
2.3 Analysis of landslide displacements
The method is based on a purely analytical approach, which is performed with MATLAB® and the open-source QGIS (version 3.28) software by processing climate data and measurements of continuous landslide displacements. Starting from the analysis of precipitation regime over the 30-year period 1991–2020, the rainiest years were identified in order to looking for a possible influence on significant accelerations during those periods in the landslide areas (Ardizzone et al., 2023).
For the cases of Sauze d’Oulx and Pragelato, a similar procedure is adopted, as already mentioned, which compares GPS and satellite data with the aim to overcome some limitations of these techniques. GPS is an optical instrument most commonly used for 3D surface displacement measurements. For the continuously operated mode, the accuracy of GPS measurement can reach the level of sub-centimeter, especially in the horizontal direction. Despite its precision, the limited availability of GPS measurements with a dense geographical distribution is a consequence of deployment constraints and high operational costs (Hu et al., 2014). Another drawback is related to the annual frequency reading which inhibits a detailed investigation of surface deformations over time and space; an aspect complemented by InSAR data that are extremely useful for landslide studies, since they grant millimeter accuracy due to short revisiting time. Moreover, InSAR techniques have more advantages: (i) good cost/benefit ratio; (ii) acquisition in all weather conditions and both day/night; (iii) data coverage of remote and inaccessible areas. Nevertheless, the interferometric approach is also affected by issues strictly related to (i) geometrical distortions between the LOS (Line of Sight) and the slope; (ii) atmospheric contribution and aliasing phase; (iii) the presence of snow, vegetation or variable land cover of the area, especially in the mountain environment. (Solari et al., 2020; Del Soldato et al., 2021).
Given the above considerations, merging GPS and InSAR information should be cautious because of their complementary features.
In order to carry out the displacement analysis for cases a) and b), it is necessary to make both datasets comparable at spatial and temporal scales, so the GPS benchmark falling within the raster cell (100 m) of the InSAR east-west mean velocity grid was considered. As the InSAR technique has an acquisition frequency of 6 days, the comparison is made by extracting from the satellite time series the daily data concurrent with the GPS acquisition date, which is annual. In this regard, the time intervals of the GPS and InSAR data that were used in the analysis for the case studies a) and b) are shown in Table 2.
A distinctive methodology that excludes interferometric data, was employed to understand changes in displacement for the Sestriere landslide (case c), emphasizing the correlation between aquifer recharge processes following precipitation inputs and rise of displacements. In this case, the analysis focused on variations in piezometer readings for the groundwater levels and inclinometer measurements for displacements.
3 Results
3.1 Climate trends in the period 1991–2020
The rainfall and the temperatures pattern of the reference period 1991–2020 for the study area was investigated. The main purpose was to assess the evolution of climate factors, both long-term trends and inter-annual variability and extreme events.
The graphs below show the most interesting results obtained from the analysis conducted using the data provided by regular grids on Piemonte region, from which areas of the Dora Riparia and Chisone basins were extracted.
The annual distributions of daily rainfall greater than or equal to 10 mm (ISPRA, 2022) are shown and it can be deduced that the years with the most significant events are 2016 (with a value of 156.75 mm on 24/11/2016) followed by 2000, 2011, 2018 (Figure 7). The trend of the maximum daily rainfall, or rather the maximum annual rainfall, has also been plotted and shows a statistically significant increase.
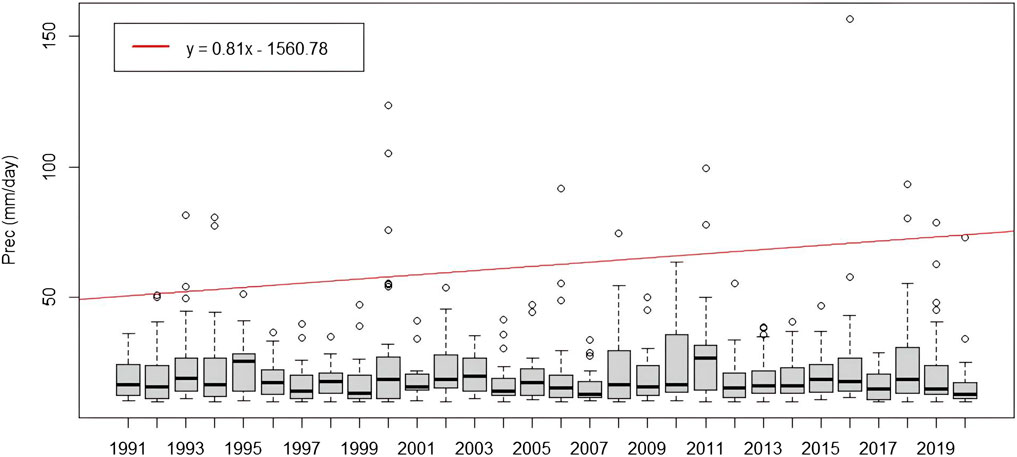
Figure 7. Annual distributions of daily precipitation greater than 10 mm in the period 1991–2020. Red line indicates the annual maximum values trend.
Since the heaviest rainfall occurs in spring and autumn, the precipitation trend in these seasons were also investigated. Following the WMO guidelines for the calculation of normal climate values, the monthly cumulative precipitation were calculated from the daily values. Seasonal precipitation were then determined by cumulating the monthly data (March, April, May for the spring season and September, October, November for the autumn season) (Supplementary Material S2A, S2B).
A similar approach is adopted for temperature regime. The maximum and minimum values were used to calculate the mean daily temperatures for the 30 years, from which the annual temperatures were derived for each year. Consequently, the resulting mean temperature over the period 1991–2020 is established as a reference value, and it is given as 7.5°C. Therefore, anomalies are evaluated by comparing the annual mean temperatures of each year to this value in order to identify meaningful deviations from the average. It can be observed how recent years have been increasingly affected by above-average temperatures (Figure 8).
The graphical results are consistent with the annual climate reports on temperature and precipitation for Piemonte region compared to the reference period 1971–2000. The reports state that 2016 and 2018 were among the wettest years and therefore investigated to develop the study, while 2022 was the hottest year in the entire dataset since 1958 (Arpa Piemonte, 2023e).
3.2 Influence on landslide displacements
The analysis of precipitation trends at the two basins scale has made it possible to identify the years affected by extreme events for which possible changing in movement trends can be investigated for the three case studies. Weather stations related to the sites are considered for a local scale analysis. For cases a) and b) in the graphs showing GPS and InSAR displacements trend, cumulative precipitation every 6 days are also depicted, having the same temporal resolution of the InSAR, to recognize possible accelerations or decelerations in the deformation time series following precipitation events.
3.2.1 Sauze d’Oulx
The Chateau Beaulard weather station (1800 m a.s.l.) reported significant daily rainfall values during precipitation events that have affected Piemonte in recent years. In particular, 120 mm on 25/11/2016, 103.6 mm on 9/1/2018 and also the years 2019, 2020, 2021 with heavy autumn rainfall (Supplementary Material S3). Therefore, the state of activity the DSGSD focused on these periods was investigated coupling GPS and InSAR data.
For the evaluation of the slope deformations, two points have been considered, since the displacement rate changes from the upper part of the landslide body to the bottom of the slope. In addition, these points have been identified to facilitate the comparison between the datasets obtained with the two techniques.
Since G6OLXA1 does not fall in the InSAR values domain, because it is placed outside the raster cell, the nearest MP is considered for comparison. The MP(2) is located at a distance of 125 m from G6OLXA1 and therefore it can be considered reliable for the analysis (Figure 9).
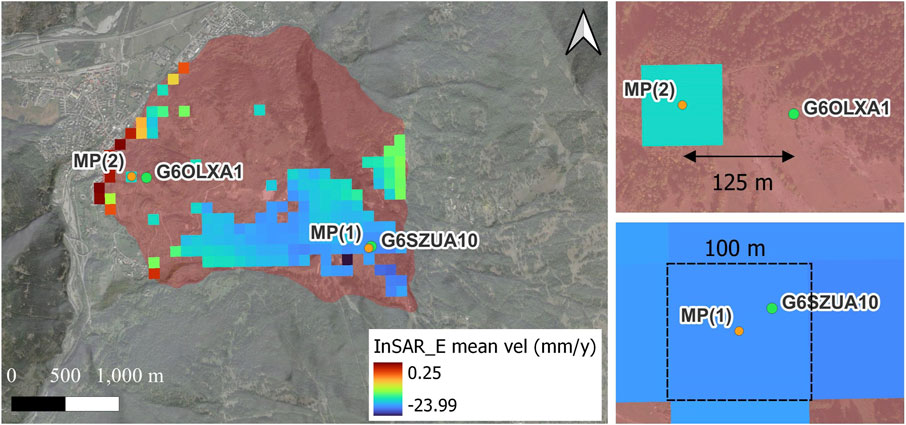
Figure 9. InSAR data coverage of DSGSD in Sauze d'Oulx. Negative velocity values indicate westward displacements, positive values indicate eastward displacements (left). The location of MPs and GPS marks is shown in detail (right).
The comparison analysis between GPS and satellite measurements confirms the trend of annual displacements in the order of 2 cm/year, as reported in the SIFraP sheet. In particular, the upper sector of the landslide, where the G6SZUA10 is located, moves at a speed of approximately 3 cm/year, unlike the G6OLXA1 located at the foot of the landslide body, which reports lower displacement rates. The InSAR data time series, which shows a similar trend (Figure 10; Figure 11), report annual displacement velocity in the order of 2 cm. Specifically, 19.39 mm/year for MP(1) (Table 3). While, at MP(2) the annual velocity is equal to 16.6 mm/year, confirming a lower displacement rate at the foot of the landslide body (Table 4). In both cases, the INSAR makes it possible to detect slight accelerations of movement close to the indicated precipitation events, which are not perceived by the GPS survey due to the temporal frequency with which it operates.
3.2.2 Pragelato
The impact of the November 2016 event rainfall on displacement rates was also investigated for the Pragelato landslide (Supplementary Material S4). Again, it can be deduced that InSAR is more sensitive than GPS in extrapolating possible changes in deformation trends due to precipitation events.
The GPS measurements report slow and progressive movements downstream (between 1 and 2 cm/year) as confirmed by InSAR mean values, showing eastward displacements (Figure 12). The G6PRGB1 and G6PRGB3 in the period 2016–2017 reported a displacement magnitude of about 2 cm, showing a relevant acceleration of the movement, probably correlated with heavy precipitation occurring.
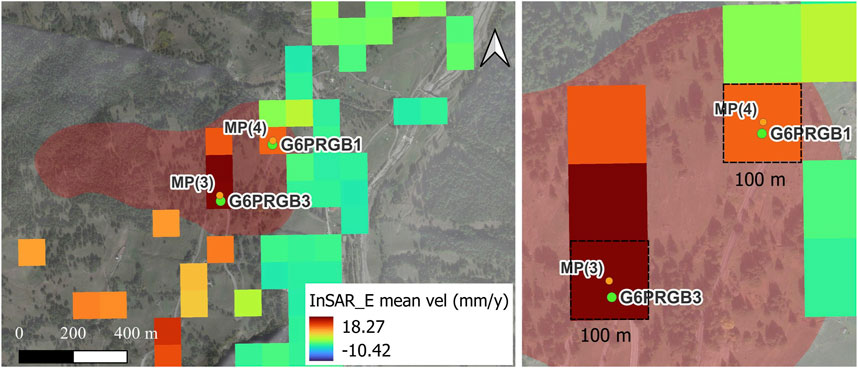
Figure 12. InSAR data coverage of landslide in Pragelato. Negative velocity values indicate westward displacements, positive values indicate eastward displacements (left). The location of MPs and GPS marks is shown in detail (right).
In addition, significant accelerations were also detected by G6PRGB3 in 2018 and 2019 with a displacement since the last reading of 2.9 cm and 2.4 cm respectively, due to heavy rainfalls in the spring and autumn of 2018. In Table 5 and Table 6 measures from GPS are reported and compared with InSAR at the MP (18.2 mm/year for MP(3) and 12.2 mm/year for MP(1)), while in Figure 13 and Figure 14 it can be noticed the pattern of displacements time series.
3.2.3 Sestriere
The complex landslide located at Champlas du Col is characterised by a rate of movement of few cm/year, varying with depth, with significative accelerations in the spring period due to snow melting as reported by Arpa Piemonte.
In this case study, the displacements recorded by the fixed-probe inclinometer S6SSTC1A with daily readings were analyzed and compared with the trend of spring maximum temperatures. In particular, since the inclinometer was installed in 2014, displacements data from that year were evaluated.
The inclinometer detects two movement surfaces (at depths of 18 and 30 m) with movement rates of even more than 4 cm/year (2013–2017 period). The shallowest movements are more circumscribed but particularly active phenomena and at high risk since their acceleration can involve built-up areas and roads.
The displacements recorded by the inclinometer S6SSTC1A at a depth of 18 m were investigated, by evaluating seasonal shifts from the sum of monthly increments. Therefore, the mean displacement over the period 2014–2022 was assessed for each season (Table 7).
Ground snow and groundwater level trends since 2014 have also been examined in support of the analysis (Supplementary Material S5A, S5B).
It has been proven that the greatest displacements occur in spring (3.43 mm), coinciding with the period of snowmelt which contributes to the increase of groundwater level. For instance, in the spring 2018 (Figure 15), the inclinometer recorded a significant displacement increase of approximately 5 mm, probably due to the abundant snowfall during the winter (maximum of 196 cm ground snow).
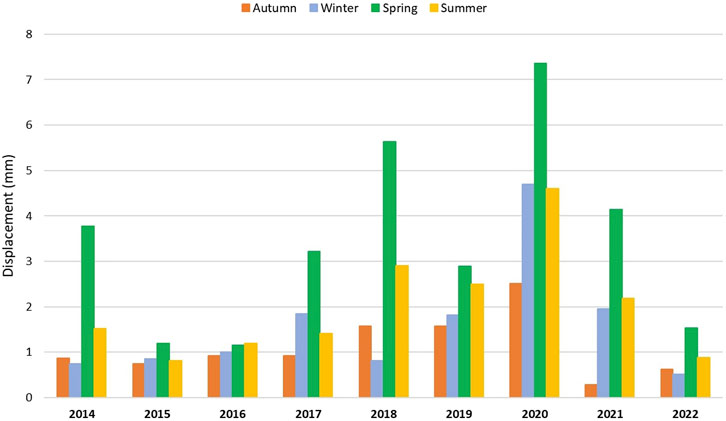
Figure 15. Seasonal displacements (2014–2022) recorded by the inclinometer S6SSTC1A for the Sestriere landslide.
4 Discussion
The assessment of the climatic variables time series in the Dora Riparia and Chisone basins revealed heavy precipitation occurrence and rise of temperatures starting from a first general evaluation of climatic pattern of the area, which have resulted in accelerations for landslides investigated as confirmed by monitoring instruments.
For the purpose of the study, the geological and morphological conditions predisposing the area to slope instability were evaluated in the preliminary analysis, resulting in a significant level of landslide hazard for the Western Alps. The three case studies selected belong to this framework, all of which are being monitored as they are affected by slow but persistent movements over time and involve built-up areas and infrastructure. The choice of monitoring network instruments was guided not only by the availability of long and gap-free time series, but also by the typology of gravitational phenomenon to be investigated. In support of field observation, the contribution of satellite data is emphasized for the detection of slow-moving ground processes, thus identifying significant changes in the time series displacement under extreme rainfall events. However, due to the approach chosen, it should be emphasised that any acceleration or changes in the InSAR time series cannot be correlated certainty with to the effects of the precipitation event, but may be due to radar acquisition errors. This is because the proposed approach is very simplified and is intended to provide a preliminary guide for future studies. The joint combination of in situ and remote sensing techniques, such as GPS and InSAR, enables the comprehension of the deformation field of the landslide, which could be imperceptible when it comes to slow surface movements.
In the case of the DSGSD in Sauze d’Oulx, being a very large and complex landslide body, there have been different displacement rates at the top and the bottom of the slope over the years. It is among the most important DSGSD in the area and it has been monitored for more than 20 years due to its not negligible acceleration (2–3 cm/year). Therefore, rates of movements recorded by GPS benchmark G6SZUA10 and G6OLXA1 located at different elevations were analysed and supplemented by InSAR data, which provide a more detailed temporal resolution. The investigation time window starts in 2016, the year from which the InSAR data are available, with the aim to assess the possible responses of the displacements trend after the exceptional rainfall event occurred in November 2016. The comparison between GPS and InSAR acquisitions is based on a simplified approach which neglects measurement discrepancies due to the poor coverage on the landslide slope of Sentinel-1 data. Moreover, this type of analysis focuses on surface deformations, excluding the displacements in depth at the sliding surface, which must instead be analyzed with inclinometer. The displacements measured by inclinometer probes were not considered as most of them were discarded after only a few years of monitoring activity and therefore not useful for analysis purposes. However, the Arpa Piemonte reports state that the manual inclinometer I6OXA3 (active since 2001), with quarterly reading, recorded a remarkable movement increase between July and December 2016, with 2018 as the year of greatest acceleration, and also during the second half of 2020. This information supports the displacement rates recorded by GPS benchmarks during the wettest periods investigated.
For the Pragelato landslide, the same method based on GPS and InSAR data was adopted, identifying the benchmarks that fall within the Sentinel-1 product raster cell. As in the case of Sauze d’Oulx, possible accelerations of displacements since 2016 have been investigated due to the occurrence of significant precipitation events. The demonstrated effectiveness and adaptability by integrating GPS and InSAR, coupled with the accessibility of free data from EGMS, as well as the possibility of solving some limitations of these techniques through their combination confirm its performant use in several applications (Del Soldato et al., 2021).
The landslide in Pragelato moves with speed about few cm/year. The Arpa Piemonte reports state that manual inclinometer measurements conducted at the top (I6PRGB0) and at the foot of the landslide body (I6PRGB1) show almost constant and extremely slow movements, with the exception of the 2016–2017 period with greater displacements as confirmed by G6PRGB3 measures. At the end of the 2016, the piezometer P6PRGB3 (Supplementary Material S4) recorded significant groundwater increases following heavy autumn rains.
Finally, for the Sestriere landslide, the displacements were investigated by considering the action of snowmelt and precipitation events on the fluctuations of the water table. Therefore, inclinometer S6SSTC1A data were processed from its installation, analyzing the displacements occurred over the seasons in the period 2014–2022. It was found that significant displacement increases occur in spring, particularly relevant are the ones recorded in 2018 and 2020. In support of the analysis, the Arpa Piemonte reports state that piezometer P6SSTC4 (Supplementary Material S5B) recorded an increase of the groundwater level of approximately 5 m in spring 2018 (from −13.07 m on 4 March to −8.71 m on 10 May), probably due to heavy precipitation in May 2018 and as a consequence of rising temperatures in the previous year. This could have caused an acceleration of the landslide movements.
5 Conclusion
The Western Alps in Piemonte are affected by a high degree of susceptibility to landslides due to the features of the geological formations covering this area. In addition, climatic conditions of the mountain environment, which are constantly changing, contribute to slope instability and thus movement accelerations.
In this work, an analytical approach was proposed to investigate the impact of the climatic anomalies of temperature and rainfall compared to the reference period 1991–2020, on the degree of activity of three slow-moving landslides.
However, since climate change is still an open issue for its complexity, no absolute and reliable interpretation has yet been provided. Consequently, it becomes equally difficult to interpret the response of the kinematic mechanisms of landslides to the occurrence of extreme events. For this reason, a number of researchers have analyzed historical records of landslides by attempting to compare them with climate variables, especially precipitation and temperature. In general, the studies reveal a wide range of influences and consequences of climate change on landslides, including contradictory, uncertain and undetermined effects.
As weather conditions are constantly changing, it is increasingly likely that the magnitude and frequency of instability phenomena will also change. Mountains are “sentinels of changes” and respond more promptly and effectively than other geographical environments to changes in climate (Gariano and Guzzetti, 2016). Whereas the frequency and/or the intensity of the rainstorms will increase, shallow landslides, including rock falls, debris flows and debris avalanches, and also ice falls and snow avalanches in high mountain areas, are also expected to increase (Stoffel et al., 2014). In this framework, attention is paid to slow-moving landslides as they provide an interesting learning environment for the dynamics that govern their movements.
The methodology proposed in this paper constitutes a simplified approach that suggests the use of different tools and data available in order to have as many answers as possible, given the complexity of this scenario. Hence, the merging between in situ and remote monitoring techniques enhance the interpretation of instability processes, but it requires a preliminary data processing due to the different spatial and temporal acquisition modes before comparison can be made. Certainly, statistical approaches such as that adopted by Paranunzio et al. (2015) are more capable of providing an interesting clarification of the relationship between climatic factors and the triggering of slope failures and displacement mechanisms. In this case, for example, analytical spatial interpolation models could be implemented to overcome issues associated with comparing GPS and InSAR data as well as the installation of GPS benchmark with automated acquisition and not annual frequency readings, which does not reliably interpret the evolution of displacements. In addition, future developments could include geological and geotechnical slope modeling to investigate instability dynamics, supplementing monitoring analysis that is generally limited to surface deformation detection.
Data availability statement
The original contributions presented in the study are included in the article/Supplementary Material, further inquiries can be directed to the corresponding author.
Author contributions
RN: Data curation, Formal Analysis, Methodology, Software, Writing–original draft, Writing–review and editing. SP: Data curation, Methodology, Supervision, Writing–review and editing. GT: Data curation, Methodology, Supervision, Writing–review and editing. MD: Supervision, Writing–review and editing.
Funding
The author(s) declare that no financial support was received for the research, authorship, and/or publication of this article.
Acknowledgments
Monitoring network data and monitoring instrument technical reports were provided by Arpa Piemonte. In particular, I wish to thank Luca Lanteri for his great availability and Professor Paolo Dabove (DIATI, Politecnico di Torino) for his valuable suggestions in dealing with geomatics aspects.
Conflict of interest
The authors declare that the research was conducted in the absence of any commercial or financial relationships that could be construed as a potential conflict of interest.
Publisher’s note
All claims expressed in this article are solely those of the authors and do not necessarily represent those of their affiliated organizations, or those of the publisher, the editors and the reviewers. Any product that may be evaluated in this article, or claim that may be made by its manufacturer, is not guaranteed or endorsed by the publisher.
Supplementary material
The Supplementary Material for this article can be found online at: https://www.frontiersin.org/articles/10.3389/feart.2024.1365469/full#supplementary-material
References
Ardizzone, F., Gariano, S. L., Volpe, E., Antronico, L., Coscarelli, R., Manunta, M., et al. (2023). A procedure for the quantitative comparison of rainfall and DInSAR-based surface displacement time series in slow-moving landslides: a case study in southern Italy. Remote Sens. 15 (2), 320. doi:10.3390/rs15020320
ARPA Piemonte (2023a). Banca dati metereologica. https://www.arpa.piemonte.it/rischinaturali/accesso-ai-dati/annali_meteoidrologici/annali-meteo-idro/banca-dati-meteorologica.html (Accessed 4 Apr 2023).
ARPA Piemonte (2023b). Banca dati ReRCoMF. https://www.arpa.piemonte.it/approfondimenti/temi-ambientali/geologia-e-dissesto/fenomenifranosi/rercomf-1 (Accessed Mar 2023).
ARPA Piemonte (2023c). Banca dati SIFraP. https://www.arpa.piemonte.it/approfondimenti/temi-ambientali/geologia-e-dissesto/bancadatiged/SIFraP (Accessed Mar 2023).
ARPA Piemonte (2023d). Dataset su griglia NWIOI. https://www.arpa.piemonte.it/rischinaturali/tematismi/clima/confronti-storici/dati/dati.html (Accessed 4 Apr 2023).
ARPA Piemonte (2023e). Rapporto climatico annuale. https://www.arpa.piemonte.it/rischinaturali/tematismi/clima/rapporti-di-analisi/annuale.html (Accessed 6 Jul 2023).
ARPA Piemonte (2023f). Scheda SIFraP ii livello frana Pragelato. https://webgis.arpa.piemonte.it/geodissesto/sifrap/sifrap_ii_liv_scheda.php?cod_frana=001-75366-00 (Accessed 16 Jun 2023).
ARPA Piemonte (2023g). Scheda SIFraP II livello frana Sauze d’Oulx. https://webgis.arpa.piemonte.it/geodissesto/sifrap/sifrap_ii_liv_scheda.php?cod_frana=001-00756-01 (Accessed 16 Jun 2023).
ARPA Piemonte (2023h). Scheda SIFraP ii livello frana Sestriere. https://webgis.arpa.piemonte.it/geodissesto/sifrap/sifrap_ii_liv_scheda.php?cod_frana=001-76807-00 (Accessed 10 Jul 2023).
Audisio, C., Nigrelli, G., Pasculli, A., Sciarra, N., and Turconi, L. (2017). A GIS spatial analysis model for landslide hazard mapping application in Alpine Area. Int. J. Sustain. Dev. Plan. 12 (5), 883–893. doi:10.2495/SDP-V12-N5-883-893
Autorità di Bacino del fiume Po Linee generali di assetto idrogeologico e quadro degli interventi (Bacino del Pellice). 2023b https://www.adbpo.it/PAI/3%20-%20Linee%20generali%20di%20assetto%20idraulico%20e%20idrogeologico/3.3%20-%20Elaborato%20Piemonte/Pellice.pdf (Accessed 10 Jul 2023).
Autorità di Bacino del fiume Po Linee generali di assetto idrogeologico e quadro degli interventi (Bacino della Dora Riparia). 2023a https://www.adbpo.it/PAI/3%20-%20Linee%20generali%20di%20assetto%20idraulico%20e%20idrogeologico/3.3%20-%20Elaborato%20Piemonte/Dora_Riparia.pdf (Accessed 10 Jul 2023).
Bordoni, M., Vivaldi, V., Bonì, R., Spanò, S., Tararbra, M., and Lanteri, L. (2022). A methodology for the analysis of continuous time-series of automatic inclinometers for slow-moving landslides monitoring in Piemonte region, northern Italy. Nat. Hazards 115, 1115–1142. doi:10.1007/s11069-022-05586-3
Carlà, T., Tofani, V., Lombardi, L., Raspini, F., Bianchini, S., Bertolo, D., et al. (2019). Combination of GNSS, satellite InSAR, and GBInSAR remote sensing monitoring to improve the understanding of a large landslide in high alpine environment. Geomorphology 335, 62–75. doi:10.1016/j.geomorph.2019.03.014
Cascini, L., Calvello, M., and Grimaldi, G. M. (2010). Groundwater modeling for the analysis of active slow-moving landslides. Journal of Geotechnical and Geoenvironmental Engineering 136 1220-1230. doi:10.1061/ASCEGT.1943-5606.0000323
Cigna, F., Del Ventisette, C., Liguori, V., and Casagli, N. (2011). Advanced radar-interpretation of InSAR time series for mapping and characterization of geological processes. Nat. Hazards Earth Syst. Sci. 11 (3), 865–881. doi:10.5194/nhess-11-865-2011
Cignetti, M., Godone, D., Notti, D., Giordan, D., Bertolo, D., Calò, F., et al. (2023). State of activity classification of deep-seated gravitational slope deformation at regional scale based on Sentinel-1 data. Landslides 20, 2529–2544. doi:10.1007/s10346-023-02114-7
Copernicus Egms, (2023). Copernicus EGMS. https://egms.land.copernicus.eu/ (Accessed 16 Jun 2023).
Cruden, D. M., and Varnes, D. J. (1996). “Landslide types and processes,” in Landslides investigation and mitigation (special report 247). Editors A. K. Turner, and R. L. Schuster (Washington, DC, USA: Transportation Research Board, US National Research Council), 36–75.
Del Soldato, M., Confuorto, P., Bianchini, S., Sbarra, P., and Casagli, N. (2021). Review of works combining GNSS and insar in Europe. Remote Sens. 13 (9), 1684. MDPI AG. doi:10.3390/rs13091684
Fioraso, G., Baggio, P., Bonadeo, L., and Brunamonte, F. (2011). Post-glacial evolution of gravitational slope deformations in the upper Susa and Chisone valleys (Italian western Alps). Italian J. Quat. Sci. 24, 104–106.
Fioraso, G., Tararbra, M., and Negro, N. (2010). La deformazione gravitativa profonda di Sauze d’Oulx (Alpi Occidentali): analisi dei meccanismi deformativi in relazione all’evoluzione tardo quaternaria dell’alta Valle di Susa. Italian J. Quat. Sci. 23 (1), 37–54.
Forno, M. G., Comina, C., Gattiglio, M., Gianotti, F., Lo Russo, S., Raiteri, L., et al. (2016). Preservation of quaternary sediments in DSGSD environment: the mont fallère case study (aosta valley, NW Italy). Alp. Mediterr. Quat. 29 (2), 181–191.
Gariano, S. L., and Guzzetti, F. (2016). Landslides in a changing climate. Earth-Science Rev. 162, 227–252. Elsevier B.V. doi:10.1016/j.earscirev.2016.08.011
Gariano, S. L., Rianna, G., Petrucci, O., and Guzzetti, F. (2017). Assessing future changes in the occurrence of rainfall-induced landslides at a regional scale. Sci. Total Environ. 596, 417–426. doi:10.1016/j.scitotenv.2017.03.103
Giardino, M., Giordan, D., and Ambrogio, S. (2004). G.I.S. technologies for data collection, management and visualization of large slope instabilities: two applications in the Western Italian Alps. Nat. Hazards Earth Syst. Sci. 4, 197–211. doi:10.5194/nhess-4-197-2004
Gizzi, M., Narcisi, R., Mondani, M., and Taddia, G. (2023). Comprehending mountain springs’ hydrogeological perspectives under climate change in Aosta Valley (Northwestern Italy): new automated tools and simplified approaches. Italian J. Eng. Geol. Environ. 1, 73–80. doi:10.4408/IJEGE.2023-01.S-10
Handwerger, A. L., Roering, J. J., and Schmidt, D. A. (2013). Controls on the seasonal deformation of slow-moving landslides. Earth Planet. Sci. Lett. 377 (378), 239–247. doi:10.1016/j.epsl.2013.06.047
Hu, J., Li, Z. W., Ding, X. L., Zhu, J. J., Zhang, L., and Sun, Q. (2014). Resolving three-dimensional surface displacements from InSAR measurements: a review. Earth-Science Rev. 133, 1–17. Elsevier. doi:10.1016/j.earscirev.2014.02.005
IdroGEO, (2023). IdroGEO. https://idrogeo.isprambiente.it/app/ (Accessed 20 Mar 2023).
IPCC (2021). “Climate change 2021: the physical science basis,” in Contribution of working group I to the sixth assessment Report of the intergovernmental Panel on climate change. Editors V. Masson-Delmotte, P. Zhai, A. Pirani, S. L. Connors, C. Péan, S. Bergeret al. Cambridge, UK (Cambridge University Press. In Press).
IPCC (2023). “Summary for policymakers,” in Climate change 2023: synthesis report. Contribution of working groups I, II and III to the sixth assessment report of the intergovernmental panel on climate change core writing team. Editors H. Lee, and J. Romero (Geneva, Switzerland: IPCC), 1–34. doi:10.59327/IPCC/AR6-9789291691647.001
ISPRA (2022). I normali climatici 1991-2020 di temperatura e precipitazione in italia .https://www.isprambiente.gov.it/it/pubblicazioni/stato-dellambiente/i-normali-climatici-1991-2020-di-temperatura-e-precipitazione-in-italia [Accessed Mar 2023].
Lacroix, P., Handwerger, A. L., and Bièvre, G. (2020). Life and death of slow-moving landslides. Nat. Rev. Earth Environ. 1, 404–419. doi:10.1038/s43017-020-0072-8
Lee, W. L., Martinelli, M., and Shieh, C. L. (2021). An investigation of rainfall-induced landslides from the pre-failure stage to the post-failure stage using the material point method. Front. Earth Sci. 9, 1–13. doi:10.3389/feart.2021.764393
Nigrelli, G., and Chiarle, M. (2021). Evolution of temperature indices in the periglacial environment of the European Alps in the period 1990–2019. J. Mt. Sci. 18 (11), 2842–2853. doi:10.1007/s11629-021-6889-x
Paranunzio, R., Chiarle, M., Laio, F., Nigrelli, G., Turconi, L., and Luino, F. (2019). New insights in the relation between climate and slope failures at high-elevation sites. Theor. Appl. Climatol. 137 (3–4), 1765–1784. doi:10.1007/s00704-018-2673-4
Paranunzio, R., Laio, F., Nigrelli, G., and Chiarle, M. (2015). A method to reveal climatic variables triggering slope failures at high elevation. Nat. Hazards 76 (2), 1039–1061. doi:10.1007/s11069-014-1532-6
Pedretti, L., Bordoni, M., Vivaldi, V., Figini, S., Parnigoni, M., Grossi, A., et al. (2023). InterpolatiON of InSAR Time series for the dEtection of ground deforMatiOn eVEnts (ONtheMOVE): application to slow-moving landslides. Landslides 20 (9), 1797–1813. doi:10.1007/s10346-023-02073-z
Shahbazi, S., Crosetto, M., and Barra, A. (2022). Ground deformation analysis using basic products of the Copernicus Ground Motion Service. Int. Archives Photogrammetry, Remote Sens. Spatial Inf. Sci. - ISPRS Archives 43 (B3-2022), 349–354. doi:10.5194/isprs-archives-XLIII-B3-2022-349-2022
SNPA - Sistema Nazionale per la Protezione dell’Ambiente (2021). Linee guida per il monitoraggio delle frane. https://www.snpambiente.it/category/pubblicazioni/linee-guida-snpa/ (Accessed 3 Apr 2023).
Solari, L., Del Soldato, M., Montalti, R., Bianchini, S., Raspini, F., Thuegaz, P., et al. (2019). A Sentinel-1 based hot-spot analysis: landslide mapping in north-western Italy. Int. J. Remote Sens. 40 (20), 7898–7921. doi:10.1080/01431161.2019.1607612
Solari, L., Del Soldato, M., Raspini, F., Barra, A., Bianchini, S., Confuorto, P., et al. (2020). Review of satellite interferometry for landslide detection in Italy. Remote Sens. 12 (8), 1351. MDPI AG. doi:10.3390/RS12081351
Stoffel, M., Tiranti, D., and Huggel, C. (2014). Climate change impacts on mass movements - case studies from the European Alps. Sci. Total Environ. 493, 1255–1266. doi:10.1016/j.scitotenv.2014.02.102
WMO (2017). WMO guidelines on the calculation of climate normals. Geneva, Switzerland: WMO-No. 1203.
WMO (2023). Climatological normals. https://community.wmo.int/en/wmo-climatological-normals (Accessed May 2023).
Keywords: landslide, climate impacts, displacements trend, monitoring, InSAR, Alps
Citation: Narcisi R, Pappalardo SE, Taddia G and De Marchi M (2024) Assessing climate impacts on slow-moving landslides in the western Alps of Piemonte: integration of monitoring techniques for detecting displacements. Front. Earth Sci. 12:1365469. doi: 10.3389/feart.2024.1365469
Received: 04 January 2024; Accepted: 18 March 2024;
Published: 08 April 2024.
Edited by:
Salvatore Martino, Sapienza University of Rome, ItalyReviewed by:
Kun Fang, China University of Geosciences Wuhan, ChinaVeronica Tofani, University of Florence, Italy
Copyright © 2024 Narcisi, Pappalardo, Taddia and De Marchi. This is an open-access article distributed under the terms of the Creative Commons Attribution License (CC BY). The use, distribution or reproduction in other forums is permitted, provided the original author(s) and the copyright owner(s) are credited and that the original publication in this journal is cited, in accordance with accepted academic practice. No use, distribution or reproduction is permitted which does not comply with these terms.
*Correspondence: Salvatore Eugenio Pappalardo, c2FsdmF0b3JlLnBhcHBhbGFyZG9AdW5pcGQuaXQ=