- 1College of Resources and Environment, Yangtze University, Wuhan, China
- 2Key Laboratory of Exploration Technologies for Oil and Gas Resources, Ministry of Education, Yangtze University, Wuhan, China
- 3No. 7 Oil Production Plant, PetroChina Changqing Oilfield Company, Xian, China
- 4Technology Development Department, XMotors.ai Inc., Santa Clara, CA, United States
Deep tight gas reservoirs are considered important hydrocarbon exploration targets. High-quality reservoir prediction is critical for successfully exploring and developing deeply buried tight sandstone gas. Previous research has found that the reservoir quality of deeply buried tight sandstones is controlled by diagenesis and sedimentary facies. However, the variation of diagenetic alterations in different facies is still poorly studied on deltaic tight gas sandstone. In this study, core analysis, wireline log data, and 3D seismic were studied for the characterization of diagenetic alterations and sedimentary facies. The tight sandstones were formed in braided river delta deposits. Gravel-bearing coarse-grained sandstone facies and cross-bedded sandstone facies developed in tight sandstones. The Gravel-bearing coarse-grained sandstone is formed in the mid-channel bar of deltaic distributary channels. The major diagenetic processes developed in the tight sandstone include compaction, cementation, and dissolution. Constructive diagenesis can generate secondary pores, mainly including dissolution and kaolinite metasomatism, which can effectively improve reservoir physical properties. Through the diagenesis alterations linked to different lithofacies in cored wells, there are obvious diagenesis variations in different lithofacies. Despite strong compaction, the reservoirs in coarse sandstone facies have developed internal dissolution. After compaction, cementation, and dissolution to increase porosity, the reservoir retains intergranular and secondary pores and forms relatively high-quality reservoirs. The fine sandstone facies with cross-bedding are strongly compacted, with internal ductile particles being compacted and deformed, and the particle orientation is clearly arranged. The reservoir is tight, and the development of dissolution in the reservoir is weak, resulting in poor reservoir quality. In addition, reservoirs located at the interface between sandstone and mudstone are often affected by diagenesis, resulting in the development of calcareous cementation, leading to poor reservoir quality. Therefore, high-quality reservoirs are mostly distributed in coarse sand lithofacies, mainly distributed in the mid-channel bar of distributary channel deposits. Sedimentary facies control the original physical properties of the reservoir with different content and texture; the quality of the original reservoir is subject to diagenetic alteration in different ways. The points in this study could offer insights better to predict deep tight reservoir quality in continental basins.
1 Introduction
Tight sandstone gas has become one of the world’s most important unconventional oil and gas resources and has huge resource reserves and broad development prospects in continental basins. However, in continental basins, tight sandstone gas reservoirs are characterized by rapid sedimentary facies change, complex sand body distribution, and strong heterogeneity of reservoir quality. In the process of production and development, there are many problems, such as low single-well productivity, great differences in gas productivity in different exploration wells, and rapid production decline. Reservoir heterogeneity strongly affects the fluid flow and recovery factors of reservoirs (Wardlaw and Taylor, 1976; Wardlaw and Cassan, 1979; Weber, 1982; Wang, et al., 2023). Therefore, in recent years, the formation mechanism of tight sandstone reservoirs and the distribution of high-quality reservoirs have been important topics of continental hydrocarbon-bearing basin exploration. According to previous studies, diagenetic alterations in sandstones are important controlling factors of the reservoir quality. Sedimentary research of reservoirs is also important as the sedimentary facies could be related to sedimentary structure, grain size, and sorting of those hydrocarbon-bearing sandstones. The spatial distribution of diagenetic alternations in different sedimentary facies is also studied (Morad et al., 2000; El-ghali et al., 2006a; El-ghali et al., 2006b; Morad et al., 2010). In continental basins, reservoirs were well developed in the delta facies. Most of the high-quality reservoirs could be developed in deltaic distributary channel facies. However, the spatial distribution of diagenetic alternations and reservoir quality within deltaic distributary channel facies is still understudied in lacustrine basins.
The Ordos Basin is China’s largest oil and gas-bearing continental basin and is rich in tight gas reservoirs (Yang et al., 2005; Yang et al., 2012; Xu et al., 2018). Tight sandstone gas reservoirs have been found in the Upper Paleozoic deposits of the basin. Most of the reservoirs developed among those deposits in the Taiyuan Formation, Shanxi Formation, and Lower Shihezi Formation. In recent years, natural gas exploration has had a great breakthrough in the southwest of the Yishan Slope structural belt, Ordos Basin. In 2018, the Qingyang gas field was discovered in the southwest of the basin. The gas reservoir is developed in the upper Paleozoic fluvial-delta sandstone deposit, which belongs to the typical ultra-low permeability tight reservoir (Fu et al., 2019; Zhu et al., 2021). Recent exploration indicates that the Upper Paleozoic natural gas resources have good development potential. The efficient development of those natural gas mainly depends on the distribution model of high-quality reservoirs about the deep buried tight gas reservoirs. Therefore, the Upper Paleozoic deposits could be an analog to analyze the distribution model of high-quality reservoirs in tight sandstone.
This study focuses on the distribution of diagenetic alterations and reservoir quality within deltaic distributary channel sandstone using 3D seismic, core, and wireline log curves data. Deltaic distributary channels of the Upper Paleozoic Shihezi Formation from the proximal to distal in the Hongde area, southwestern Ordos Basin were selected as targets. The study aims were listed as follows: 1) To describe and interpret the sedimentary facies of the deltaic distributary channel deposits and horizontal distribution; 2) To get diagenesis features and alternations in lithofacies of distributary channel deposits; 3) To discuss diagenetic alterations linked to depositional lithofacies, Then, high-quality reservoir quality distribution model within deltaic distributary channel was clarified in the Lower Shihezi Formation.
2 Geological setting
The Ordos Basin is surrounded by the Yinshan Mountains in the north, the Qinling Mountains in the south, the Luliang Mountains in the east, and the Tengger Desert in the west. The basin is sub-divided into six structural units, including the Yishan slope, the Weibei uplift, the Yimeng uplift, the Western Shanxi fold belt, the Western obduction zone, and the Tianhuan depression (Figure 1). The Hongde area is in the southwest of Ordos Basin. The tectonic unit is located on the western of the Yishan slope, south of the Tianhuan depression, with the Qingyang gas field in the southeast of the area, and the Upper Paleozoic tight sandstone has great potential for natural gas exploration (Wang et al., 2016; Fu et al., 2019). The Late Paleozoic stratigraphy in the Hongde area was a set of clastic rock from the marine-continental transitional facies, with a total sedimentary rock thickness of about 700 m (Zhu S. F. et al., 2021). The Benxi Formation, the Taiyuan Formation, the Shanxi Formation, the Lower Shihezi Formation, the Upper Shihezi Formation, and the Shiqianfeng Formation were developed sequentially from the bottom up, in which the coal measure source rocks of the Benxi Formation, the Taiyuan Formation, and the Shanxi Formations continuously supplied hydrocarbons to the overlying strata (Meng et al., 2021).
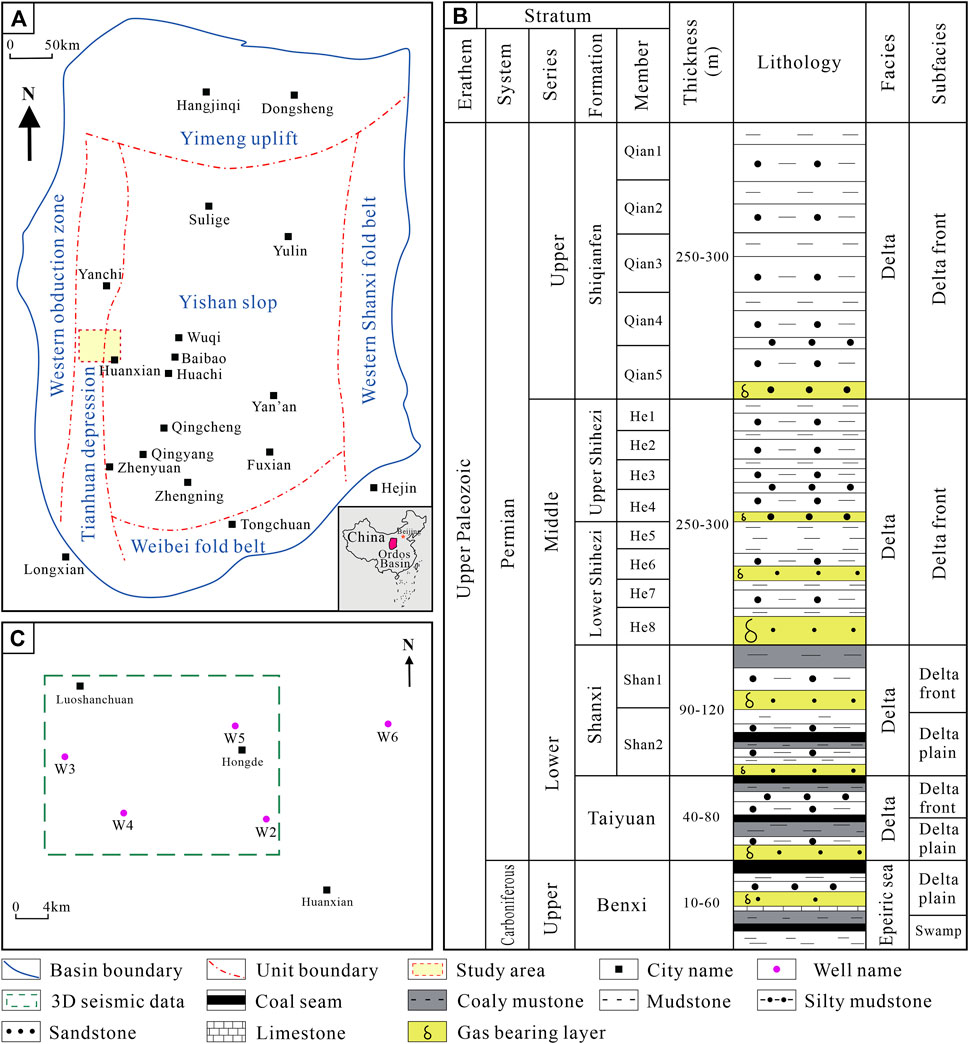
FIGURE 1. Location of the Hongde area, with tectonic setting of the Ordos Basin and the stratigraphy division. (A) Map showing the location and division of tectonic units of the Ordos Basin (modify from Liu et al. (2015)). (B) Stratigraphical section of the Upper Paleozoic strata. (C) Gas exploration wells and seismic data cover range in study area.
The target layer in this paper is the He 8 member of the Permian Shihezi Formation, which is the major oil and gas-producing layers in this area. Previous research concluded that the sedimentary environment of the Permian in the Ordos Basin was relatively warm and humid, and the sedimentary water depth was around 20 m. The study area is characterized by relatively flat topographic slope, non-development of large-scale fault, tectonic stability, and strong hydrodynamic conditions that can carry a large number of sediments, which provide favorable conditions for shallow-water delta sedimentation (Xiao et al., 2008; Guo et al., 2020; Zhu et al., 2021; Xia et al., 2022).
The Upper Paleozoic tight sandstone reservoirs in the study area have deep burial depths (range from 3500–4500 m), tight reservoirs (average porosity <7%, average permeability <0.5×10−3 μm2), complex microscopic pore-throat structures, and a variety of pore types (Cui et al., 2021; Qi et al., 2021). Recent oil and gas exploration and development studies show that the tight sandstone gas reservoir of the He 8 member of the Permian Shihezi Formation in the Hongde area is rich, and some exploration wells have already discovered natural gas reservoirs, which has an excellent prospect for natural gas exploration. It is urgent to research the reservoir quality of the He 8 member of the Permian Shihezi Formation, which is still in the initial period.
3 Data and methods
This study used the cores, log curves, 3D seismic data, and reservoir physical property (porosity and permeability) data. The data used in this study are from the PetroChina Changqing Oilfield Company. Detailed descriptions, photographs, and sediment particle size analysis from 56 m of core records in 4 cored wells that penetrated the He 8 member were taken for identification of lithofacies. 3D seismic data that cover the central part of the study area (289 km2). Porosity and permeability data come from 220 tight sandstone samples from 4 wells. Wireline log curve data was used to identify sedimentary facies in other wells without cores. Detailed descriptions of the tight sandstone core samples in the He 8 member of the Shihezi Formation were taken to identify and interpret lithofacies. The lithofacies and vertical association of the tight sandstone deposits are summarized based on color, grain size, and sedimentary structure. The characteristics of lithofacies from the different depositional sequences were interpreted after core description. The log curve characteristics of different sedimentary facies were studied in the Shihezi Formation.
Based on the study of lithofacies and log curves facies analysis of the He 8 member, sedimentary facies interpretation was conducted on the wells in the Hongde area. The sedimentary variation was revealed through a comparison of lithofacies from the deltaic distributary channels developed in the Shihezi Formation. The vertical sedimentary facies variation was studied through well-tied profiles from the proximal to the distal direction of the deltaic deposits of the He 8 member. The diagenesis features and alternations in different lithofacies were studied using thin sections. The high-quality reservoirs were identified using porosity and permeability in those wells. This study used wireline log curves from four exploration wells and 3D seismic data to predict the deltaic tight sandstones. Seismic amplitude attribute extraction and seismic inversion were used in this study for tight sandstone reservoir prediction. The horizontal distribution of the deltaic tight sandstone is predicted through sedimentary facies and sand body thickness contour map. With the assistance of wireline log curves and 3D seismic data, the vertical and horizontal distributions of high-quality reservoirs were investigated through sedimentary lithofacies and sand body thickness contour map. The relationship between diagenetic alterations and depositional lithofacies in deltaic distributary channels is analyzed for further discussion. Basis on horizontal and vertical analysis, the high-quality reservoir quality distribution model within the deltaic distributary channel is discussed in the discussion chapter.
4 Results
4.1 Lithofacies characteristics and interpretation
During the depositional period of the He 8 member, due to the rapid decrease in baseline, cold and dry climate, and increased stratigraphic slope, the sediment supply rate increased (Zhu S. F. et al., 2021). As a result, the shoreline continued to advance towards the edge of the delta front, and the branching and merging of channels were more frequent, mainly developing braided river deltas. The subaqueous distributary channel sand bodies are the major types of sand bodies, with gravel-bearing coarse-grained sandstone facies, cross-bedding coarse sandstone facies, and black mudstone facies commonly found in the He 8 member (Figure 2).
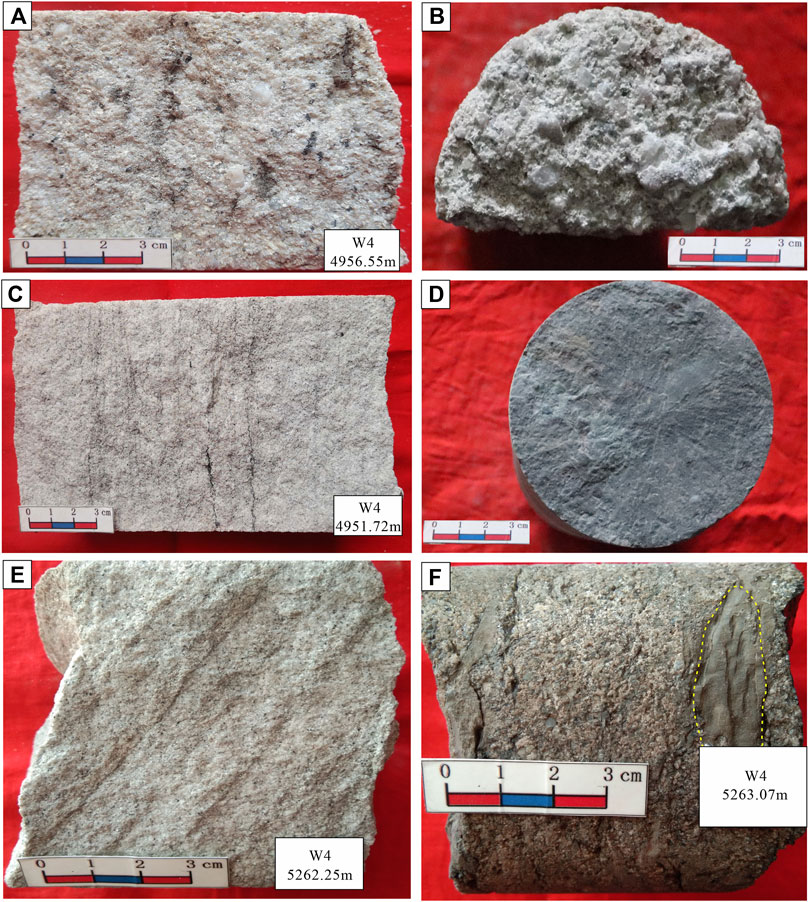
FIGURE 2. Typical lithofacies photographs from cores. (A) Massive gravel-bearing coarse sandstone (Sg), well W4, 4956.55 m. (B) Massive gravel-bearing coarse sandstone (Sg), well W5, 4459.8 m. (C) Sandy gravel-bearing lithic sandstone, well W4, 4951.72 m. (D) Black mudstone, well W5, 4462.3 m. (E) Cross-bedding coarse sandstone (Sp), well W3, 5262.25 m. (F) Massive coarse sandstone with black mud clasts (mark by yellow line) in well W3 (Sm), 5263.07 m.
In the distributary channel microfacies, the lithology is relatively coarse, with the development of sandstone and gravel-bearing coarse sandstone. The overall sorting is medium to good, and the sedimentary structure is mainly composed of cross-bedding and parallel bedding. At the bottom, fine conglomerate and visible erosion surfaces are often developed, and the main thickness is 5–8 m. Vertically, there are mainly positive and compound positive rhythms. In terms of logging response characteristics, the microfacies of the distributary channel exhibit a bell-shaped or composite shape of gamma ray and resistivity curves, with generally higher amplitudes (Figure 3). The distributary channel can develop mid-channel bar deposits inside, and coarse-grained lithology is usually developed in the mid-channel bar, including conglomerate and gravel-bearing coarse sandstone. The sorting is generally medium to good, with visible massive bedding and parallel bedding. In terms of logging response characteristics, mid-channel bar deposits often exhibit a box-shaped gamma ray curve.
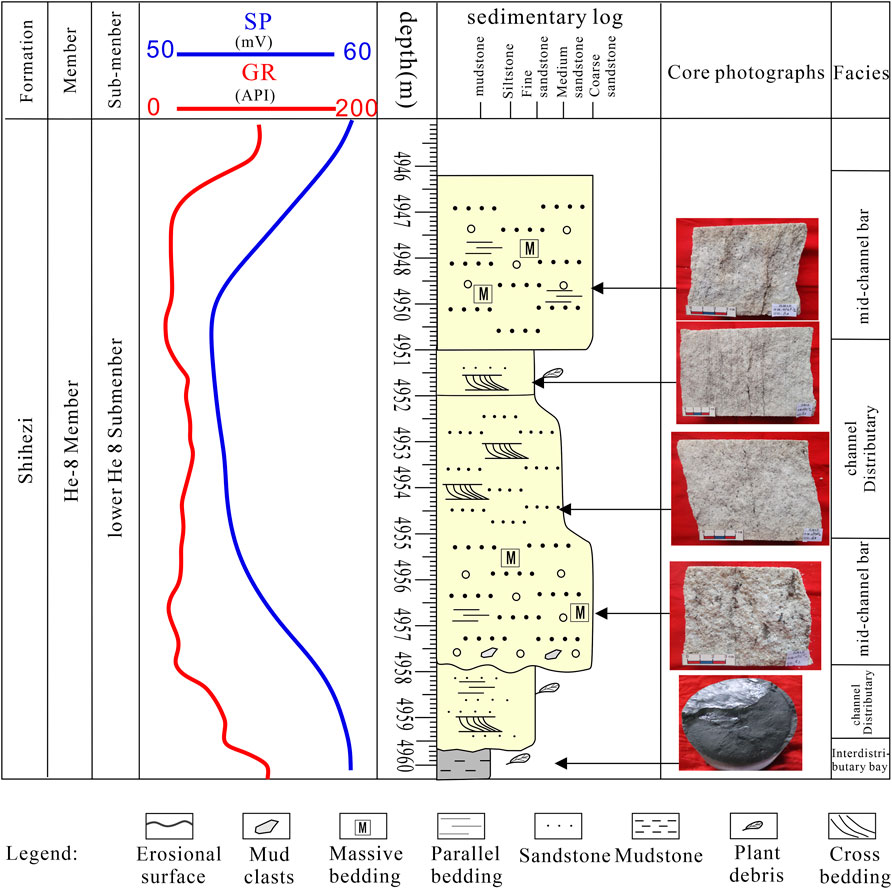
FIGURE 3. Sedimentary microfacies interpretation and lithofacies description of well W4, and typical lithofacies photographs.
4.2 Physical properties
By analyzing the tight sandstone samples of the He 8 member in the study area. Porosity ranges between 0.8% and 16.3%, with a mean value of 5.1%, and the permeability ranges between 0.001 mD and 10 mD, with a mean value of 0.42 mD. The samples with porosity less than 10% and permeability less than 0.5 mD accounted for 87% of the total samples. Porosity and permeability distributions of different lithofacies vary greatly (Figure 4).

FIGURE 4. Reservoir quality of the sandstone from the He 8 member. (A) The distribution of reservoir porosity. (B) The distribution of reservoir permeability. (C) Plots of the relationship between porosity and permeability from the reservoir test data.
4.3 Diagenesis features and pores in the reservoirs
This study takes the microscopic features as the core and combines thin section analysis to analyze and conclude that the major diagenetic processes developed in the study area include compaction, pressure dissolution, cementation and metasomatism, and dissolution. According to the impact of diagenesis on reservoir properties, diagenesis can be divided into two categories: constructive diagenesis and destructive diagenesis. Constructive diagenesis can generate secondary pores, mainly including dissolution and kaolinite metasomatism, which can effectively improve reservoir physical properties. On the contrary, destructive diagenesis can reduce pores, mainly through mechanical compaction, pressure solution, and cementation, which significantly reduces reservoir physical properties. Although kaolinite cementation can fill pores and block throats, the intracrystalline pores formed by it do indeed constitute gas storage spaces in the study area. The pore types of the tight sandstone reservoir in the He 8 member of the study area are mainly intragranular dissolved pores, Intracrystalline pores, and intragranular pores followed by a certain amount of residual intergranular pores (Figure 5). The pore types between different layers are similar, but there are significant differences between different lithologies. In quartz sandstone, due to its strong compaction resistance, the content of intragranular pores and intragranular dissolved pores is relatively high.
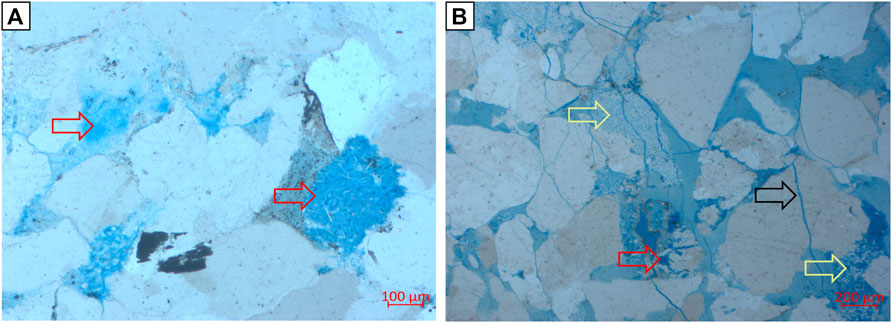
FIGURE 5. Photomicrographs showing the lithology and pores from thin section observations. (A) Intragranular dissolved pores, (mark by red arrow) W4 4950.8 m; (B) Intracrystalline pores (mark by yellow arrows) and intragranular pores (mark by red arrow), note the fractures in quartz grains, (mark by black arrow) W5 4457.1 m.
4.4 Distribution of facies prediction using 3D seismic
The single-well facies in the He 8 member are interpreted by sedimentary interpretation from cored wells. Draw the well-tie profile of sedimentary facies comparison through single-well facies (Figure 6A). Moreover, the plane distribution of sand body is predicted based on the RMS seismic attributes in the Hongde area. The distribution of thick sandstone is also consistent with the high-value area on the RMS seismic attribute map (Figure 7A). The distribution trend of sand bodies is described in plain view. Then, the sedimentary facies were predicted in the He 8 member. From the thickness contour map of the sandstone in the He 8 member, the delta lobe has a continuous distribution pattern (Figure 6B).
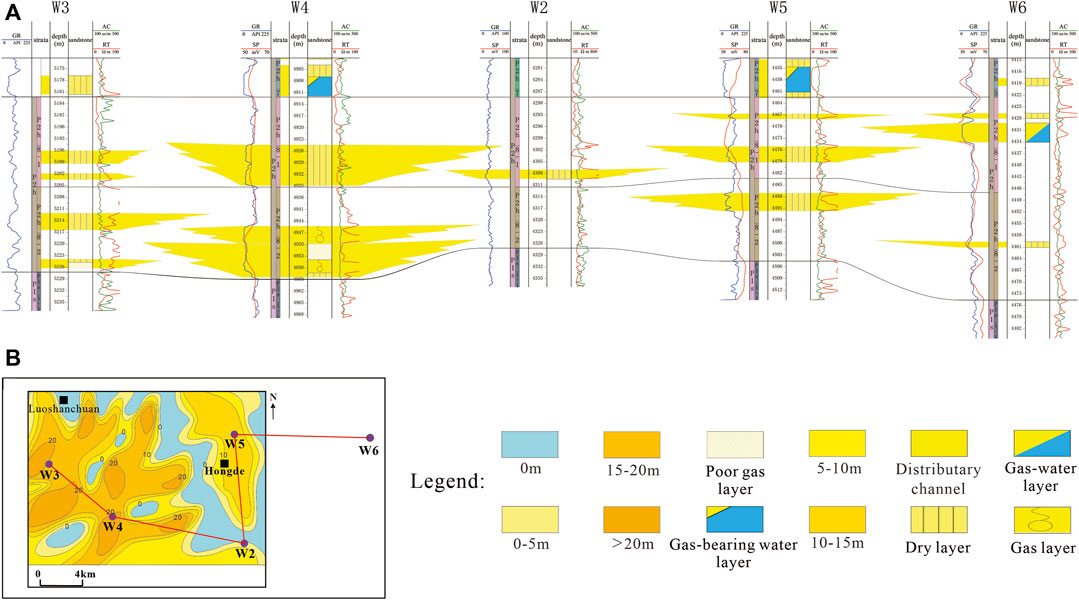
FIGURE 6. (A) Well-tie profile of sand bodies and distributary channel in the He 8 member. Note the position of the profile on the sand body thickness contour map (B).
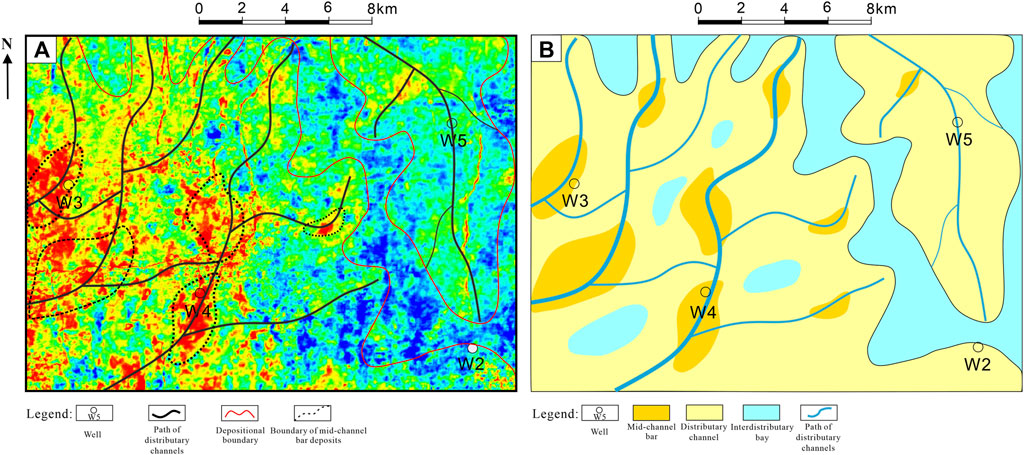
FIGURE 7. Sedimentary facies distribution of the He 8 member in the Hongde area. (A) RMS amplitude of the He 8 member. (B) Sedimentary facies of the He 8 member.
The He 8 member of the Upper Paleozoic sedimentary environment in the research area is delta deposits under shallow water gentle slope conditions, with distributary channels and mid-channel bar deposits. On the plane, the distributary channels are well developed on the delta lobe. The mid-channel bars are distributed in a long strip or lens shape in the middle of the distributary channel. The W4 well developed the mid-channel bars facies (Figure 7B).
5 Discussion
5.1 Diagenetic alterations linked to depositional lithofacies
By comparing the diagenesis of different lithofacies in typical core wells, it was found that there are apparent differences in the development of reservoir diagenesis of different lithofacies. Despite strong compaction, the gravel-bearing coarse-grained sandstone reservoirs in coarse sandstone lithofacies have developed internal dissolution. After compaction, cementation to reduce porosity, and dissolution to increase porosity, the secondary pores were preserved in the sandstone and formed relatively high-quality reservoirs (Figure 8). The cross-bedding fine sandstone lithofacies were strongly compacted, with internal ductile particles being compacted and deformed. The particle orientation is clearly observed in those lithofacies. The reservoir in those lithofacies is tight, and dissolution in the reservoirs is weak. This could be the major reasons for the formation of poor reservoir quality. In addition, diagenesis at the interface between sandstone and mudstone is often affected by geological fluid from the mudstone during compaction, resulting in the development of calcareous cementation. This leads to a poor reservoir quality near the mudstone. Therefore, the distribution characteristics of lithofacies are the major factors controlling reservoir quality. The high-quality reservoirs are mostly distributed in coarse sandstone lithofacies, mainly distributed in the mid-channel bar deposits (Figure 9).
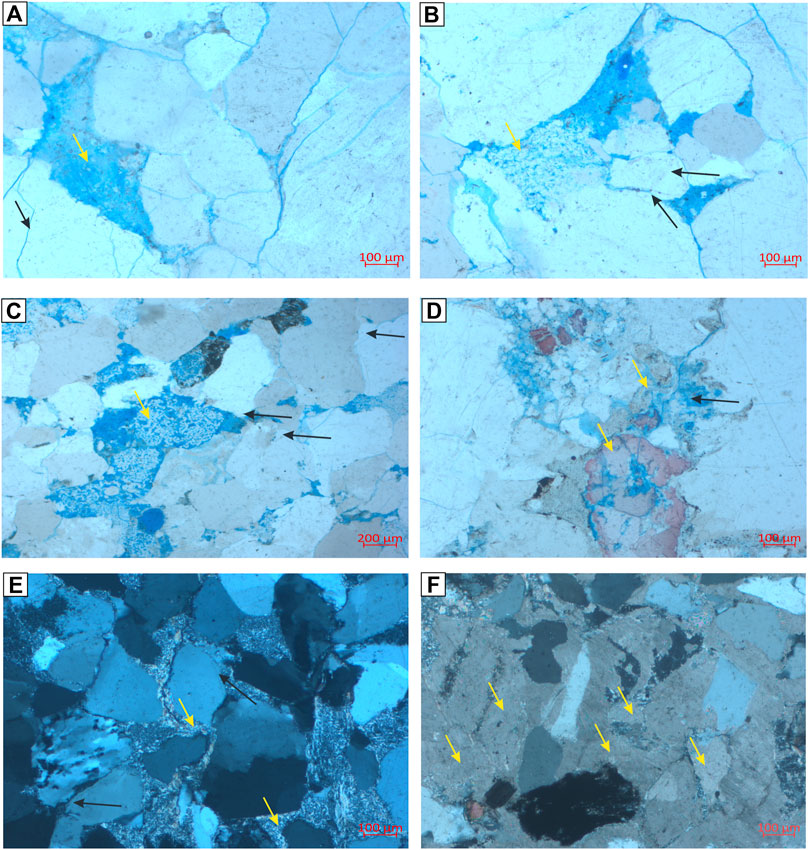
FIGURE 8. Photomicrographs showing the lithology, pore. (A) Intragranular dissolved pores, (mark by yellow line), note the fractures in quartz grains (mark by black line) W4, 4956.55 m. (B) Intragranular dissolved pores (mark by yellow line) and intergranular pores, (mark by black lines) W4, 4955.32 m. (C) Intragranular dissolved pores (mark by yellow lines) and intergranular pores, (mark by black lines) W4, 4947.03 m. (D) Intragranular dissolved pores (mark by yellow lines) and intergranular pores, (mark by black lines) W4, 4954.34 m. (E) Intragranular dissolved pores (mark by black lines) and intergranular pores, (mark by yellow lines) W4, 4952.24 m. (F) Intragranular dissolved pores, (mark by yellow lines) W4, 4958.11 m.
5.2 High-quality reservoir quality distribution model within deltaic distributary channel
Deposition controls the original physical properties of the reservoir (Li et al., 2017), mainly reflected in the impact of differences in sedimentary fabric and components on the quality of the original reservoir. The coarse the particle size of the rock and the lower the shaliness content, the higher the quality of the original reservoir and the better the porosity and permeability. There are significant differences in sedimentary fabrics and components among different types of sandstone in the study area, resulting in significant differences in their original porosity and permeability. In the previous study of sedimentary facies, it was believed that the He 8 section developed braided river shallow water delta deposits. Through the thickness contour map of well controlled sand bodies and seismic data, it was found that thick sand is mainly distributed inside the mid-channel bar of the delta distributary channel. Through core observation and thin section analysis, it was found that the lithofacies types are mostly gravel-bearing coarse sandstone facies and gravel-bearing sandstone facies. Through microscopic analysis, it is believed that the gravel-bearing coarse sandstone facies and gravel-bearing sandstone facies developed in the core beach of the distributary channel are the main high-quality reservoir lithofacies. Dissolution is a critical diagenetic process in developing tight sandstone reservoirs in coal-bearing strata. The dissolution phenomenon is relatively common in the sandstone reservoirs of the Shihezi Formation in the research area, and there are two main types of dissolution: one is the dissolution of rock debris, and the other is the dissolution of intergranular cement. The medium-to-coarse sandstone and massive bedding pebbly medium-to-coarse sandstone are formed into high-quality reservoirs after compaction, cementation to reduce porosity, and dissolution to increase porosity. High-quality reservoirs are distributed in the middle of distributary channels. The intersection diagram of single well sand body thickness and porosity shows that high-quality reservoirs are developed in thick sand intervals, and there is a clear correlation between reservoir quality and sand body thickness (Figure 10). The logging curve of the thick sand intervals is box-shaped. Based on the formation and preservation mechanism of reservoirs and the development characteristics of gas reservoirs, a sweet spot development model of dominant rock facies in reservoirs has been established (Figure 11).
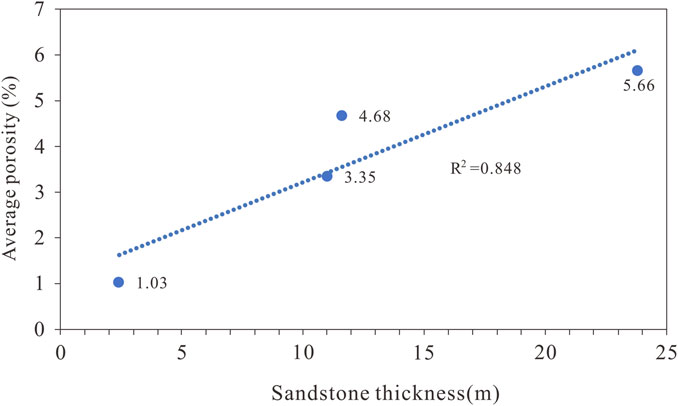
FIGURE 10. Cross plot between sandstone thickness and average porosity of the sandstone in He 8 member.
6 Conclusion
(1) Based on cores, well log curves and 3D seismic data, the He 8 member of the Permian Shihezi Formation in the Hongde area, western Ordos basin mainly developed braided river deltaic deposits. The He 8 member sandstone section develops thick sandstone in distributary channels. The deltaic sandstone gradually pinches out from southwest to northeast. The mid-channel bars were developed in distributary channels.
(2) The lithology of He 8 members of Upper Paleozoic in the study area is mainly lithic quartz sandstone, and quartz sandstone. The main reservoir space of the tight sandstones in He 8 members is intergranular pores, intergranular solution and intracrystalline pores, with porosity of 5.14% and permeability of 0.42 mm D. The reservoir quality is mainly controlled by its pore radius.
(3) From the diagenesis features study of those tight reservoirs The Upper Paleozoic He 8 member in the study area experienced the diagenesis of rigid particle compaction and fracture, plastic particle compaction and deformation, quartz secondary enlargement, kaolinite and calcite cementation and metasomatism, grain dissolution. Diagenetic materials mainly come from the grain dissolution and transformation between clay minerals. Compaction and are destructive diagenesis and dissolution are main constructive diagenesis in the tight sandstones.
(4) Through microscopic analysis, the gravel-bearing coarse sandstone lithofacies developed in the mid-channel bar of the distributary channel are the main high-quality reservoir lithofacies. Although these lithofacies sandstones have undergone strong compaction, the quartz content in the sandstone components is relatively high, and some pores can still be preserved. The internal dissolution of the reservoir develops, forming tight gas reservoirs. High quality reservoirs exhibit characteristics of locally developed planar and vertically developed layers, and are likely to exhibit discontinuous and dispersed distribution within three-dimensional subsurface deltaic-lacustrine deposits.
(5) The favorable lithofacies in deltaic sand bodies in the He 8 member in the western Ordos basin could form lithologic traps, which have important exploration and development value for lithologic natural gas reservoirs. The high-quality reservoirs are pinched out as sweet spot in the sandstone deposits. forming a favorable zone of the lithologic traps.
Data availability statement
The original contributions presented in the study are included in the article/Supplementary material, further inquiries can be directed to the corresponding authors.
Author contributions
XW: Conceptualization, Methodology, Writing–original draft. ZS: Conceptualization, Methodology, Writing–original draft. YB: Conceptualization, Methodology, Writing–original draft. WW: Methodology, Writing–review and editing. ZY: Methodology, Writing–review and editing. BW: Formal Analysis, Writing–review and editing. XZ: Writing–review and editing, Formal Analysis. LD: Writing–review and editing, Project administration. ZW: Writing–review and editing, Project administration.
Funding
The author(s) declare that financial support was received for the research, authorship, and/or publication of this article. This research was supported by National Natural Science Foundation of China (No. 42202177)
Acknowledgments
The authors wish to thank the No. 7 Oil Production Plant, PetroChina Changqing Oilfield Company for providing various data for this study. We are also greatly appreciate reviewers and editors for their constructive comments for their precious advice.
Conflict of interest
Authors XW, ZS, YB, WW, ZY, and BW were employed by the PetroChina Changqing Oilfield Company. Author XZ was employed by the XMotors.ai Inc.
The remaining authors declare that the research was conducted in the absence of any commercial or financial relationships that could be construed as a potential conflict of interest.
Publisher’s note
All claims expressed in this article are solely those of the authors and do not necessarily represent those of their affiliated organizations, or those of the publisher, the editors and the reviewers. Any product that may be evaluated in this article, or claim that may be made by its manufacturer, is not guaranteed or endorsed by the publisher.
References
Cui, G. X., Wei, Q. L., Xiao, L., Wang, S., Hu, R., and Wang, C. H. (2021). Reservoir characteristics of permian lower He 8 member in longdong area, Ordos Basin. Geoscience 35 (4), 1088–1097. doi:10.19657/j.geoscience.1000-8527.2021.04.028
El-ghali, M. A. K., Morad, S., Ai-Aasm, I., Ramseyer, K., and Mansurberg, H. (2006b). Distribution of diagenetic alterations in glaciogenic sandstones within a depositional facies and sequence stratigraphic framework: evidence from the Upper Ordovician of the Murzuq Basin, SW Libya. Sediment. Geol. 190, 299–327. doi:10.1016/j.sedgeo.2006.05.020
El-ghali, M. A. K., Tajori, K. G., Mangsurberg, H., Ogle, N., and Kalin, R. M. (2006a). Origin and timing of siderite cementation in Upper Ordovician glaciogenic sandstones from the Murzuq basin, SW Libya. Mar. Pet. Geol. 23, 459–471. doi:10.1016/j.marpetgeo.2006.02.002
Fu, J. H., Wei, X. S., Luo, S. S., Zuo, Z. F., Zhou, H., Liu, B. X., et al. (2019). Discovery and geological knowledge of the large deep coal-formed Qingyang gas field, Ordos Basin, NW China. Petroleum Explor. Dev. 46 (6), 1111–1126. doi:10.1016/s1876-3804(19)60267-3
Guo, Y. Q., Wang, M. X., Guo, B. C., Cai, Z. C., Hui, L., He, Z. Q., et al. (2020). Sedimentary system characteristics and paleographic evolution of Upper Paleozoic of northern west margin. Ordos Basin. J. Northwest Univ. Nat. Sci. Ed. 50 (1), 93–104. doi:10.16152/j.cnki.xdxbzr.2020-01-013
Li, Z., Wu, S. H., Xia, D. L., Zhang, X. F., and Huang, M. (2017). Diagenetic alterations and reservoir heterogeneity within the depositional facies: a case study from distributary-channel belt sandstone of Upper Triassic Yanchang Formation reservoirs (Ordos Basin, China). Mar. Pet. Geol. 86, 950–971. doi:10.1016/j.marpetgeo.2017.07.002
Liu, F., Zhu, X. M., Li, Z., Xu, L. M., Niu, X. B., Zhu, S. F., et al. (2015). Sedimentary characteristics and facies model of gravity flow deposits of Late Triassic Yanchang Formation in southwestern Ordos Basin, NW China. Pet. Explor. Dev. 42 (5), 633–645. doi:10.1016/S1876-3804(15)30058-6
Meng, X. L., Ai, Q. L., Wang, J. C., Bian, X. Y., Zhu, C. R., An, W. H., et al. (2021). Reservoir-forming conditions of deep tight sandstone gas reservoirs, Qingyang gasfield, Ordos Basin. Nat. Gas Explor. Dev. 44 (1), 104–110. doi:10.12055/gaskk.issn.1673-3177.2021.01.014
Morad, S., Al-Ramadan, K., Ketzer, J. M., and De Ros, L. F. (2010). The impact of diagenesis on the heterogeneity of sandstone reservoirs: a review of the role of depositional facies and sequence stratigraphy. AAPG Bull. 94 (8), 1267–1309. doi:10.1306/04211009178
Morad, S., Ketzer, J. M., and De Ros, L. F. (2000). Spatial and temporal distribution of diagenetic alterations in siliciclastic rocks: implications for mass transfer in sedimentary basins. Sedimentology 47, 95–120. doi:10.1046/j.1365-3091.2000.00007.x
Qi, R., He, F. Q., Wang, F. B., Jia, H. C., and Wang, W. (2021). Exploration breakthrough of upper paleozoic of well changtan 1 in southern Ordos Basin and its significance. China Pet. Explor. 26 (3), 68–78. doi:10.3969/j.issn.1672-7703.2021.03.006
Wang, Y., Cheng, H. F., Hu, Q. H., Jia, L. B., Wang, X. M., Gao, S. S., et al. (2023). Adsorption of methane onto mudstones under supercritical conditions: mechanisms, physical properties and thermodynamic parameters. Petroleum Sci. 20 (1), 34–47. doi:10.1016/j.petsci.2022.08.017
Wang, Z. L., Wei, L., Wang, X. Z., Wang, N. X., Fan, C. Y., Li, Y. J., et al. (2016). Accumulation process and mechanism of lower paleozoic gas reservoir in yan’an area, Ordos Basin. Acta Pet. Sin. 37 (1), 99–110. doi:10.7623/syxb2016S1010
Wardlaw, N. C., and Cassan, J. P. (1979). Oil recovery efficiency and the rock-pore properties of some sandstone reservoirs. Bull. Can. Petrol. Geol. 27, 117–138. doi:10.35767/gscpgbull.27.2.117
Wardlaw, N. C., and Taylor, R. P. (1976). Mercury capillary pressure curves and the interpretation of pore structure and capillary behavior in reservoir rocks. Bull. Can. Petrol. Geol. 24, 225–262. doi:10.35767/gscpgbull.24.2.225
Weber, K. J. (1982). Influence of common sedimentary structures on fluid flow in reservoir models. J. Petrol. Technol. 34, 665–672. doi:10.2118/9247-PA
Xia, H., Wang, L., Zhang, D. F., Wang, J. P., Fan, Q. Q., Feng, M., et al. (2022). Sequence architecture, sedimentary evolution and controlling factors of the Permian Shan-1 Member, Qingyang gas field, southwestern Ordos Basin. Oil Gas Geol. 43 (6), 1397–1488. doi:10.11743/ogg20220610
Xiao, J. X., Sun, F. J., He, N. X., Liu, R. E., Li, J., Xiao, H. P., et al. (2008). Permian Shanxi Formation and member 8 of xiashihezi Formation in Ordos Basin: palaeogeography and catchment area for sediments derived from north and south provenances. J. Palaeogeogr. 10 (4), 341–354. Available at: http://journal09.magtechjournal.com/gdlxb/CN/10.7605/gdlxb.2008.04.002.
Xu, Q. H., Shi, W. Z., Xie, X. Y., Busbey, A. B., Xu, L. T., Wu, R., et al. (2018). Inversion and propagation of the late paleozoic porjianghaizi fault (north Ordos Basin, China): controls on sedimentation and gas accumulations. Mar. Petrol. Geol. 91, 706–722. doi:10.1016/j.marpetgeo.2018.02.003
Yang, H., Fu, J., Liu, X., and Meng, P. (2012). Accumulation conditions and exploration and development of tight gas in the Upper Paleozoic of the Ordos Basin. Petrol. explor. Dev. 39 (3), 315–324. doi:10.1016/S1876-3804(12)60047-0
Yang, Y. T., Li, W., and Ma, L. (2005). Tectonic and stratigraphic controls of hydrocarbon systems in the Ordos basin: a multicycle cratonic basin in central China. AAPG Bull. 89, 255–269. doi:10.1306/10070404027
Zhu, R. J., Li, R. X., Liu, X. S., Yang, M. Y., Qin, X. L., Wu, X. L., et al. (2021). Characteristics of the diagenetic evolution of tight sandstone gas reservoir and its property in the upper paleozoic erathem in southwestern ordos basin. J. Lanzhou Univ. Nat. Sci. 57 (05), 637–649+658. doi:10.13885/j.issn.0455-2059.2021.05.009
Zhu, S. F., Cui, H., Chen, J. H., Luo, G. J., Wang, W. Y., Yang, Y., et al. (2021). Sedimentary system and sandstone reservoir petrology of a shallow water delta: case study of the Shan-1 and He-8 members in the western Ordos Basin. Acta Sedimentol. Sin. 39 (1), 126–139. doi:10.14027/j.issn.1000-0550.2020.115
Keywords: sedimentary facies, reservoir quality, tight sandstone, Shihezi formation, Ordos basin
Citation: Wu X, Shen Z, Bao Y, Wang W, Yan Z, Wang B, Zheng X, Dou L and Wen Z (2024) Diagenetic alterations and deep high-quality reservoirs within deltaic distributary channel facies: a case study from the Permian Shihezi formation in the Hongde area, southwestern Ordos basin, China. Front. Earth Sci. 12:1363309. doi: 10.3389/feart.2024.1363309
Received: 30 December 2023; Accepted: 16 January 2024;
Published: 06 February 2024.
Edited by:
Wenguang Wang, Northeast Petroleum University, ChinaReviewed by:
Yang Wang, Chang’an University, ChinaZaitian Dong, Liaoning Technical University, China
Bingshan Ma, Southwest Petroleum University, China
Copyright © 2024 Wu, Shen, Bao, Wang, Yan, Wang, Zheng, Dou and Wen. This is an open-access article distributed under the terms of the Creative Commons Attribution License (CC BY). The use, distribution or reproduction in other forums is permitted, provided the original author(s) and the copyright owner(s) are credited and that the original publication in this journal is cited, in accordance with accepted academic practice. No use, distribution or reproduction is permitted which does not comply with these terms.
*Correspondence: Luxing Dou, luxingdou@yangtzeu.edu.cn; Zhigang Wen, wzg728@sina.com