- 1Chinese Academy of Geological Sciences, Beijing, China
- 2School of Geosciences, Yangtze University, Wuhan, China
- 3No. 6 Geological Party, Xizang Bureau of Geology and Mineral Exploration and Development, Lhasa, China
Although high-silica granites can provide unique insights into the maturity of the continental crust and rare metal enrichment, the origin of high-silica granitic magmatism remains uncertain. In this paper, we present an integrated study of zircon U-Pb geochronology and trace elements, whole-rock geochemistry, and Sr-Nd isotopes for two typical high-silica granites (namely, the Longbao granitic porphyry and the Yushu granite) found in the North Qiangtang terrane, central Tibetan Plateau. Zircon geochronological data indicate that these high-silica granites crystallized at 217–214 Ma. All the samples from the Longbao granitic porphyry and the Yushu granite exhibited high SiO2, low MgO, depletion of Ba, Nb, Sr, P, and Ti, and enrichment of Th and U. They exhibited relatively high (87Sr/86Sr)i ratios of 0.7120–0.7136 and low εNd(t) values of −8.58 ∼ −7.58; together with their old ages according to the two-stage Nd model (1.6–1.7 Ga), these features indicate the involvement of crustal materials. Geochemical and isotopic variation indicated that the high-silica granites studied were mainly produced by the dehydration melting of a muscovite (Ms)-bearing source, and that the Triassic turbidites might be a good candidate for the magma source. Combining this evidence with new regional studies, it can be concluded that partial melting of Triassic turbidites induced by slab roll-back might be the key factor controlling the origin of Late Triassic magmatism in the North Qiangtang terrane.
1 Introduction
One of the distinguishing features that sets Earth apart from other planets in our Solar System and renders it able to sustain life is the felsic continental crust (Brown, 2013; Keller et al., 2015), and granitoids are essential for the formation of Earth’s stable continental crust (Campbell and Taylor, 1983). High-silica granite (SiO2>70 wt%) is a unique rock type among the granitoids found on Earth, and can serve as a valuable indicator of the compositional maturity of continental crust (Glazner et al., 2008; Lee and Morton, 2015; Wu et al., 2017). High-silica granites are enriched in incompatible elements (such as Li, Be, Nb, Ta, Zr, and Hf) and are closely associated with rare-metal mineralization (Wu et al., 2020; Shuai et al., 2021; Deng et al., 2022). However, the origin of high-silica granitic magmatism is uncertain. Previous studies have proposed various genetic models, including melting of metasedimentary or granitic rocks (e.g., Glazner et al., 2008; Frost et al., 2016), fractional crystallization (Lee and Morton, 2015), melt-crystal segregation (e.g., Chen et al., 2021), and magmatic-fluid interaction (Ballouard et al., 2016). Zircon is an accessory mineral widely occurring in felsic igneous rocks, and could play a crucial role in precisely recording the geological age, magma temperature, and long-term evolution of the silicic magma system (e.g., Zhu et al., 2023; Miller et al., 2003).
The Tibetan Plateau has generally been recognized as a natural laboratory for study of the Tethyan tectonic–magmatic evolution, mineralization, and continental dynamics (Xu et al., 2015). The central Tibetan Plateau is characterized by the development of the Palaeo-Tethyan orogenic system, which is composed of multiple suture zones and terranes, high-pressure metamorphic belts, and island arc groups (Xu et al., 2015). With the tectonic evolution of the Palaeo-Tethyan Ocean, Triassic intermediate-acidic magmatic rocks were widespread in the central Tibetan Plateau. However, lively debate continues on the origin, petrogenesis, and geodynamic mechanisms of these magmatic rocks. For example, there is debate as to whether the Triassic granitic magmatism was derived from the melting of the Precambrian basement materials or related to the melting of the Hohxil-Bayan Har-Songpan-Garzê (HBSG) turbidites (Liu et al., 2021; Liu et al., 2023). There are still two competing models, a “collision-related” model and a “subduction-related” model, put forward to describe the Triassic tectonic evolution of the North Qiangtang terrane and the adjacent HBSG terrane (Yuan et al., 2010; Liu et al., 2021).
In this study, we present a comprehensive study of zircon U-Pb geochronology, zircon trace elements, whole-rock geochemistry, and Sr-Nd isotopes for two suites of newly identified high-silica granites in the central Tibetan Plateau. Those new results are used to constrain the petrogenesis of the Late Triassic high-silica granites, to reveal the Triassic crustal melting, and to further understand the geodynamic relationships of these granites with the Palaeo-Tethyan Ocean.
2 Geological background and sample description
There are four main Palaeo-Tethyan sutures in the central Tibetan Plateau, namely, from north to south, the A’nyemaqen-Kunlun suture, the Garzê-Litang suture, the Jinshajiang suture, and the Longmuco-Shuanghu suture. The HBSG terrane appears as an inverted triangular structure, from which the vast Triassic deep marine turbidites develop (Figure 1A; de Sigoyer et al., 2014). This voluminous series of turbidites are dominantly derived from the Dabie ultrahigh-pressure metamorphic rocks, the Qingling-Dabie orogen, the Kunlun arc, the South China terrane, or the Central Qiangtang ultrahigh-pressure metamorphic rocks (Weislogel et al., 2006; Zhang et al., 2012; Ding et al., 2013; Jian et al., 2019; Chen et al., 2023). Late Triassic to Early Jurassic magmatic rocks are widespread in the HBSG terrane, and mainly include granitoids, minor diorites, and volcanic rocks (Liu et al., 2021). The NQ terrane consists of Paleozoic to Mesozoic sedimentary rocks, carbonate rocks, and interbedded volcanic rocks, and is underlain by the Precambrian crystalline basement, with ages of 991–1,044 Ma (He et al., 2013). With the southward subduction of the Garzê-Litang Paleo-Tethyan Ocean, Triassic volcanic rocks, diorites, and granitoids are linearly distributed along the northern margin of the NQ terrane (Liu et al., 2016a; Liu et al., 2016b; Liu et al., 2021; Liu et al., 2022; Tan et al., 2020; Yang et al., 2012).
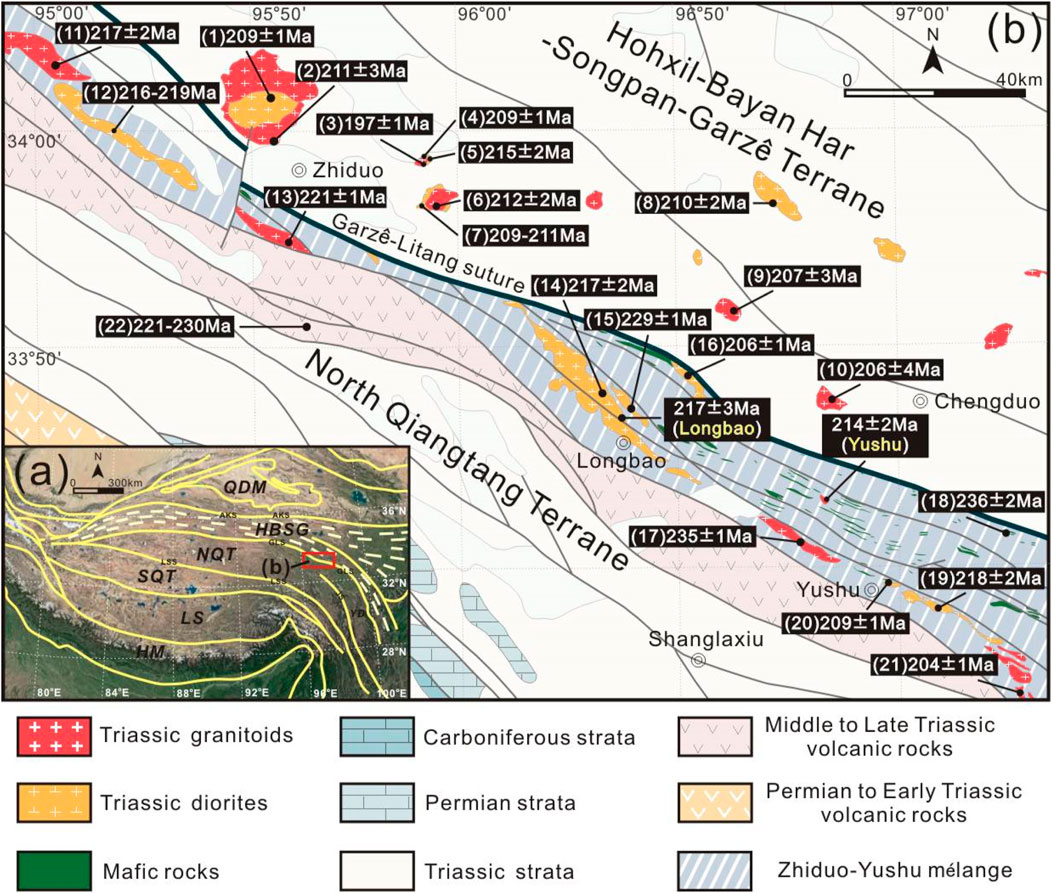
Figure 1. (A) Simplified tectonic map of the Tibetan Plateau. Continental terranes: HM, Himalaya; LS, Lhasa; SQT, South Qiangtang; NQT, North Qiangtang; HBSG, Hohxil-Bayan Har-Songpan-Garzê; QDM, Qaidam; AKS, A’nyemaqen-Kunlun suture; GLS, Garzê-Litang suture; JS, Jinshajiang suture; LSS, Longmuco-Shuanghu suture. (B) Simplified geological map of the study area in the central Tibetan Plateau, showing the main strata and Triassic igneous rocks. Age data are compiled from (1, 4, 7, 8, 14, and 19) Liu et al. (2021) (2, 7, 9, 10, and 16) Liu et al. (2019) (3, 6, and 12) Wang et al. (2008) (5, 11, 12, and 13) Tan et al. (2020) (15 and 17) Wang (2009) (18) Liu et al. (2016a) (20) Zhang et al. (2013) (21) Roger et al. (2003) (22) Zhao et al. (2014), Yang et al. (2012), Liu et al. (2019), and Liu et al. (2016b).
In this study, we investigated two different suites of typical Late Triassic granitic rocks developed in the northern margin of the NQ terrane, which are represented by the Yushu granite and the Longbao granitic porphyry (Figure 1). The Yushu granite intrudes into the Zhiduo-Yushu mélange, with an exposed area of ∼1.56 km2. This pluton shows medium-to coarse-grained granular textures, and its granularity increases from the edge to the center (Figure 2A). The Yushu granite is composed of quartz (30%–38%), K-feldspar (30%–35%), plagioclase (15%–25%), muscovite (3%–5%), biotite (2%–4%), and minor accessory minerals including garnet, apatite, zircon, and opaque minerals. Most of the K-feldspar grains are generally coarse-grained perthites, and their inclusions consist of plagioclase, quartz, and muscovite (Figure 2D). Plagioclase occurs as subhedral crystals, and exhibits some alterations by secondary muscovite (Figure 2E). Flaky muscovite and biotite occur as subhedral to anhedral crystals, and they are partly replaced by a small amount of chlorite (Figures 2D–G). Garnet shows euhedral or subhedral grain with minor muscovite inclusions (Figure 2G).
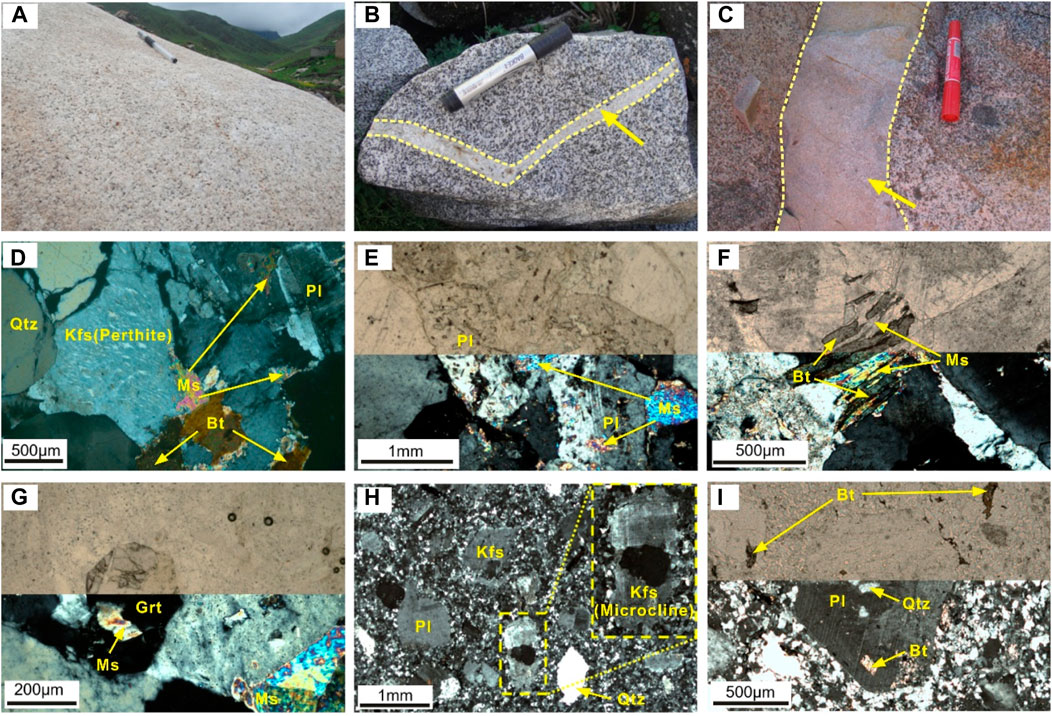
Figure 2. Field photographs and microscope photographs for (A,D–G) the Yushu granite and (B,C and H,I) the Longbao granitic porphyry. The upper halves of the microscope photographs (e, f, g, and (I) show images obtained with plane-polarized light, and the bottom halves, images obtained with perpendicular polarized light. Mineral abbreviations: Qtz, quartz; Kfs, K-feldspar; Pl, plagioclase; Bt, biotite; Ms, muscovite; Grt, garnet.
The Longbao granitic porphyry intrudes as dykes into the host diorite pluton and shows obvious chilled margins (Figures 2B, C). This granitic porphyry consists of phenocrysts (35%–40%) and matrixes (60%–65%). The phenocrysts mainly contain subhedral to xenomorphic fine-grained quartz (∼30%), K-feldspar (∼35%), plagioclase (∼32%), and biotite (∼3%). Some quartz phenocrysts show the dissolution harbor (Figure 2H). K-feldspar phenocrysts mainly contain microcline and orthoclase (Figure 2H). Plagioclase phenocrysts display typical polysynthetic twinning, and contain some quartz and biotite inclusions (Figure 2I). The matrixes are composed of the feldspar-quartz microcrystals and some cryptocrystalline substance (Figure 2H).
3 Methods
3.1 Zircon U-Pb dating and trace element composition
The selection of typical samples HX06-2 (Longbao) and RN03-1 (Yushu) for U-Pb isotopic dating was based on petrographic observations. Zircon grains were separated by conventional heavy liquid and magnetic methods and then purified under a binocular microscope. Cathodoluminescence (CL) images illustrate the internal morphology of the analyzed zircon grains (Figure 3). Zircon U-Pb geochronology analyses were conducted synchronously by LA-ICP-MS at the State Key Laboratory of Geological Processes and Mineral Resources (GPMR), China University of Geosciences, Wuhan. The details of instrument operating conditions and data processing can be found in Liu et al. (2010). Uncertainties for individual analyses are quoted at the 1 sigma level, and errors for weighted mean ages are quoted at 95% confidence. A common Pb correction was applied using the method described by Andersen (2002). Weighted mean 206Pb/238U ages were calculated and plotted using the ISOPLOT software.
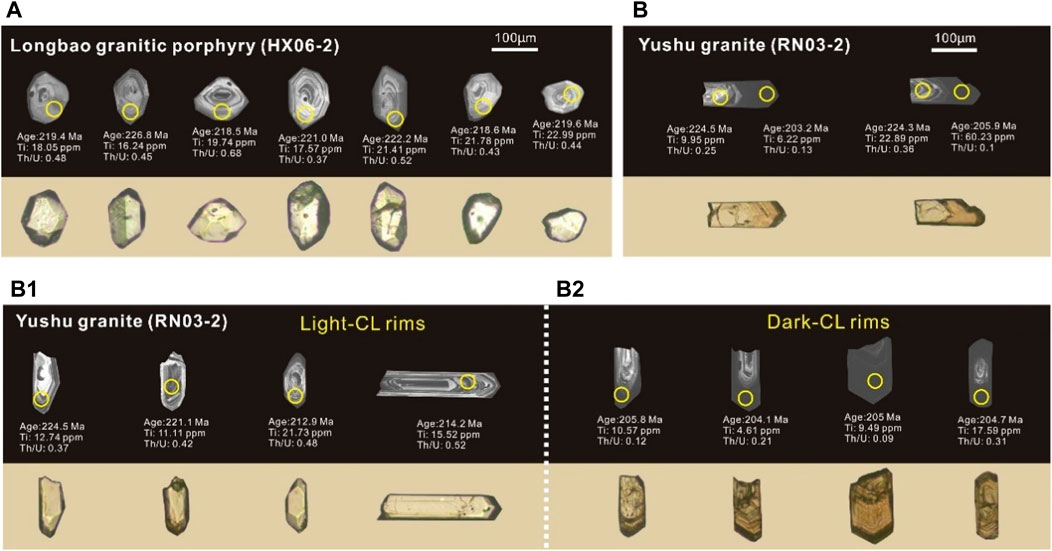
Figure 3. Representative cathodoluminescence and transmitted light images of zircons from the Longbao granitic porphyry (A) and the Yushu granite (B, B1, B2) samples. The corresponding ages, trace element concentrations, and elemental ratios are presented below the CL images. Yellow circles denote the positions of analysis of LA-ICP-MS for the U-Pb isotope, with spot size of 32 μm.
3.2 Whole-rock geochemistry and Sr-Nd isotopes
The whole-rock major element analyses were performed at the Analytical Institute of the Bureau of Geology and Mineral Resources, Hubei Province, China. The analytical precision of the spectrometer employed was better than 5%. Trace elements were measured using an Agilent 7500a ICP-MS instrument at the GPMR, following the methods of Liu et al. (2008). The samples were digested with a mixture of HF + HNO3 for ICP-MS analyses.
Whole-rock Sr-Nd isotopic compositions were determined using a Thermo-type Neptune Plus MC-ICP-MS instrument at the GPMR. Mass fractionation corrections for the isotopic ratios were based on 88Sr/86Sr = 8.375209 and 146Nd/144Nd = 0.721900. The JNdi-1 and NBS 987 standards were measured at a mean 88Sr/86Sr ratio of 0.710274 ± 0.000009 and a mean 146Nd/144Nd ratio of 0.512118 ± 0.000009, respectively.
4 Results
4.1 Zircon internal texture and U-Pb geochronology
A Longbao granitic porphyry sample (HX06-2) and a Yushu granite sample (RN03-2) were selected for zircon U-Pb isotopic dating; the results are listed in Table 1.
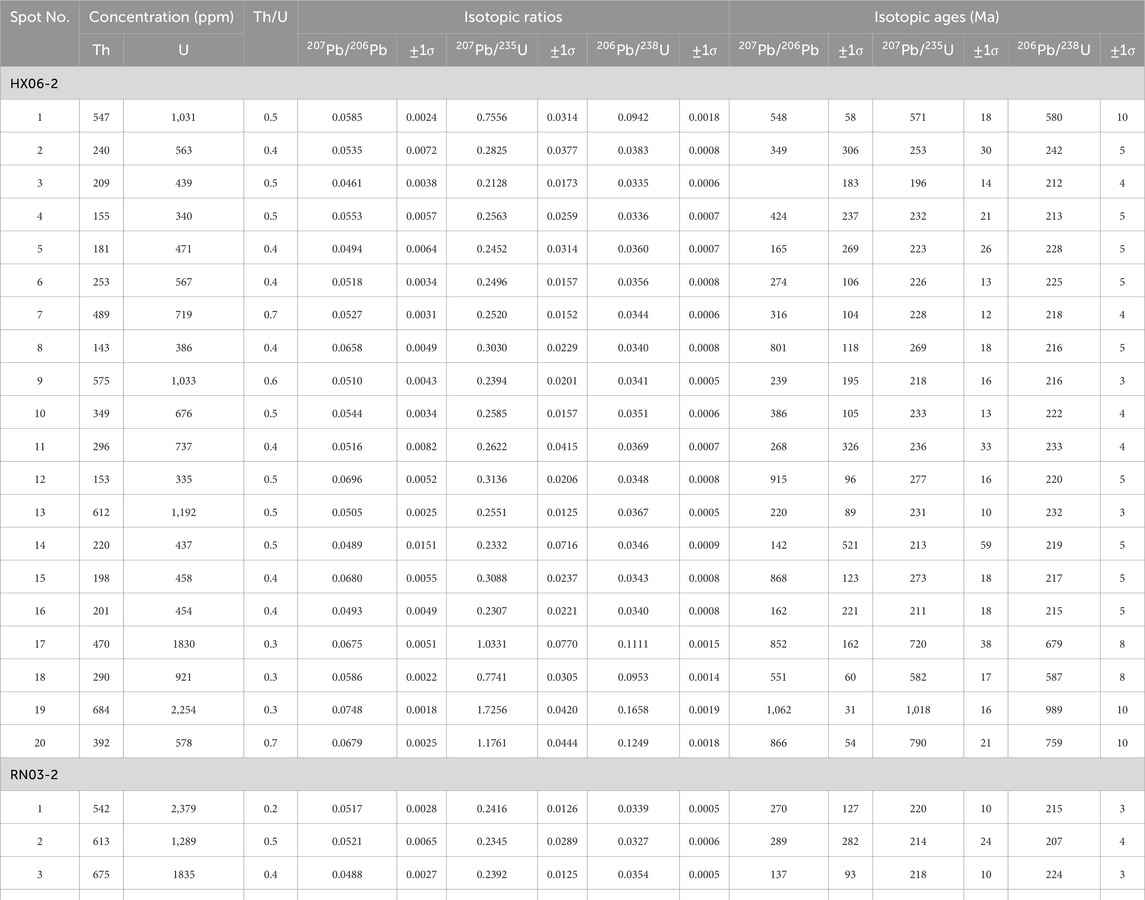
Table 1. LA-ICP-MS zircon U-Pb data for the Longbao granitic porphyry (HX06-2) and the Yushu granite (RN03-2), central Tibetan Plateau.
Zircons from the sample HX06-2 were euhedral-subhedral prismatic crystals, and they had lengths of ∼50–130 μm, with an aspect ratio of 1:1 to 2:1. Most of them exhibited apparent oscillatory zoning, and some showed resorbed cores on CL images. Ten analyses of the zircon rims yielded younger 206Pb/238U ages of 212–222 Ma. The weighted mean 206Pb/238U age was 217 ± 3 Ma (MSWD = 0.51; Figures 4A–C), which represents the crystallization age of the Longbao granitic porphyry. The other ten spots yielded relatively old 206Pb/238U ages of 225–989 Ma, which can be interpreted as the ages of the inherited zircons.
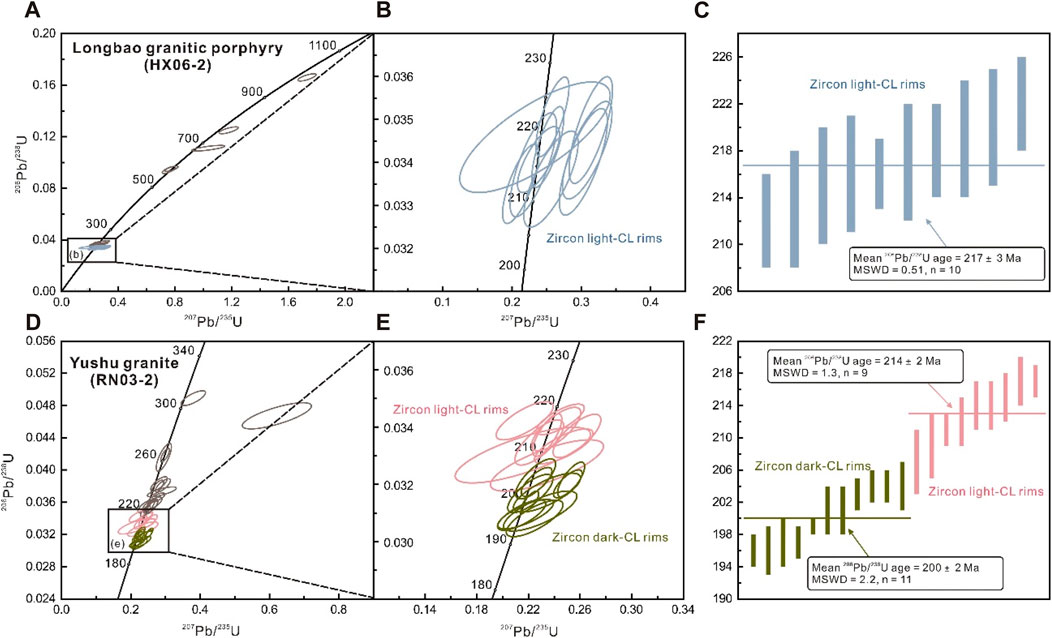
Figure 4. Zircon U-Pb concordia diagrams (A,B) and (D,E) and weighted mean ages (C,F) for magmatic zircons of the Longbao granitic porphyry and the Yushu granite from the central Tibetan Plateau. Weighted mean ages for pooled 206Pb/238U analyses are quoted with the corresponding 95% confidence interval.
Zircons from the sample RN03-2 were euhedral to subhedral prismatic crystals with light yellow to brown colors. Their lengths ranged from 40 μm to 150 μm. Unlike the monotonous oscillatory zoning of zircon in the Longbao granitic porphyry, zircons from the Yushu granite showed complex internal structures, as revealed by CL and transmitted light images (Figure 3B). Zircon grains from the Yushu granite could be subdivided into two groups based on zircon internal structure and age. Group 1 zircons showed light-CL rims and oscillatory zonings on CL images, and they were transparent under transmitted light (Figure 3B), which makes them similar to the zircons from the Longbao granitic porphyry. Group 2 zircons exhibited distinct dark-CL rims on CL images, and these zircons were murky brown and generally translucent under transmitted light (Figure 3B). Nine spots on the zircon with light-CL rims yielded 206Pb/238U ages ranging from 207 Ma to 217 Ma, with a weighted mean 206Pb/238U age of 214 ± 2Ma (MSWD = 1.3). In the other group of zircons with dark-CL rims, eleven rim spots yielded 206Pb/238U ages of 196–204 Ma, with a weighted mean 206Pb/238U age of 200 ± 2 Ma (MSWD = 2.2; Figures 4D–F). In addition, fifteen analysis spots on old inherited cores yielded 206Pb/238U ages between 221 and 308 Ma.
4.2 Zircon trace element composition and Ti-in-zircon geothermometer
Trace element concentrations of zircon grains from the Longbao granitic porphyry sample (HX06-2) and the Yushu granite sample (RN03-2) were determined by LA-ICP-MS; the results are tabulated in Table 2. The results of magmatic zircon analysis were plotted to assess the conditions of zircon growth during the generation of the respective intrusions (Figure 5).
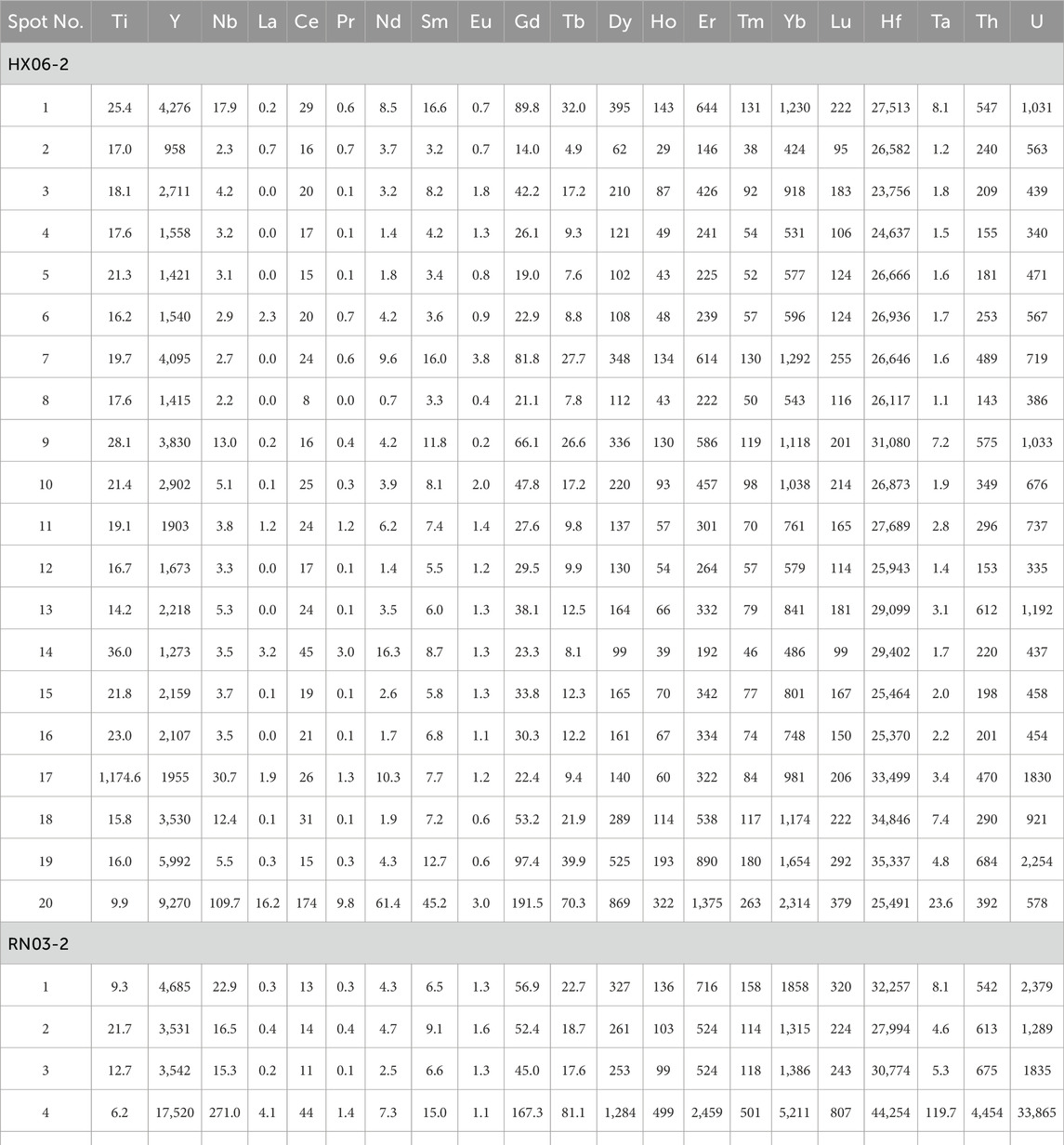
Table 2. Trace elements of zircons for the Longbao granitic porphyry (HX06-2) and the Yushu granite (RN03-2) in the central Tibetan Plateau.
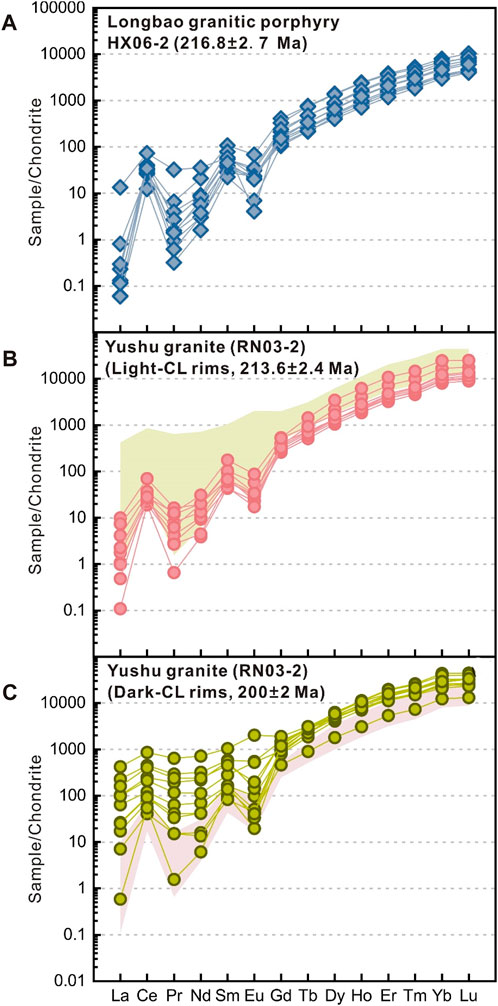
Figure 5. Zircon trace element (TE) results for the Longbao granitic porphyry (A) and the Yushu granite (B,C) samples. Graphs show CI chondrite-normalized (McDonough and Sun, 1995) rare earth element (REE) plots.
Zircons from the Longbao granitic porphyry exhibited steeply increasing chondrite-normalized REE patterns from La to Lu, and positive Ce and negative Eu anomalies (Figure 5A). The patterns for these zircons indicated low Hf, Y, Th, and U concentrations, with relatively high Ti concentrations and Th/U ratios (Figure 5B and Figure 6), which are similar to those of typical unaltered igneous zircons, as described by Hoskin and Schaltegger (2003).
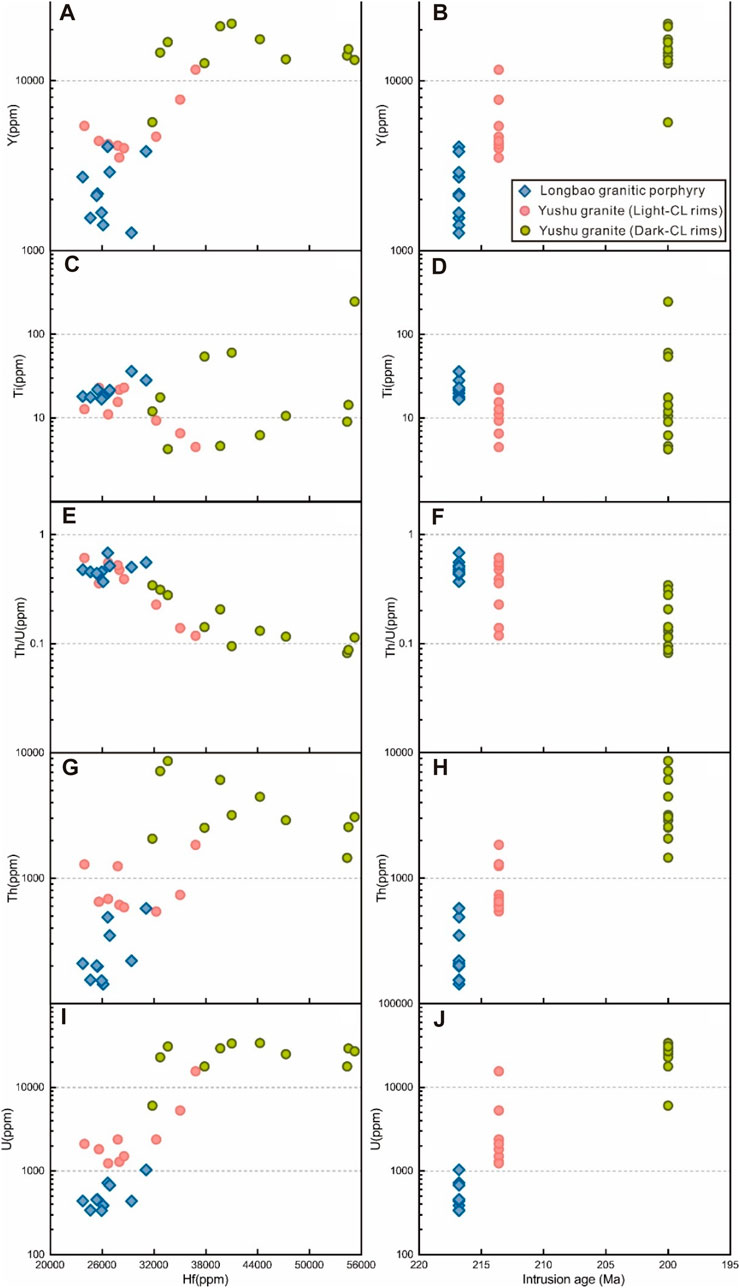
Figure 6. Zircon trace element concentrations and ratios plotted against Hf concentration (left column) and age (right column) for the Longbao granitic porphyry and the Yushu granite samples.
The two groups of zircons from the Yushu granite exhibited markedly different trace element compositions. Zircons with light-CL rims had low Hf and Y concentrations, with moderate Th and U concentrations and Th/U ratios. Additionally, the chondrite-normalized REE patterns were steep, with positive Ce and negative Eu anomalies (Figure 5C). These could also be comparable to the characteristics of typical unaltered igneous zircons. However, zircons with dark-CL rims showed enrichment in LREE and MREE and high abundances of Hf, Y, Th, and U, with low Th/U ratios (Figure 6). These features are distinct from those of unaltered magmatic zircon, which could be interpreted as indicative of hydrothermal origin (Hoskin, 2005). Thus, the weighted mean 206Pb/238U age of 214 ± 2Ma can be interpreted as the crystallization age of the Yushu granite, while the younger weighted mean 206Pb/238U age of 200 ± 2 Ma for the dark rims may reflect the Pb loss caused by the late hydrothermal process. To estimate the crystallization temperature for minerals removed from their original petrologic context, we selected the Ti-in-zircon thermometry method of Ferry and Watson (2007). It is necessary to consider the activity factors of
4.3 Whole-rock major and trace elements
The results of whole-rock geochemical analyses and the relevant parameters calculated are listed in Table 3. LOI values were relatively low (0.25–0.59 wt%), and neither the mobile nor the immobile elements exhibited any correlation with LOI (Supplementary Figure S1), suggesting insignificant alteration.
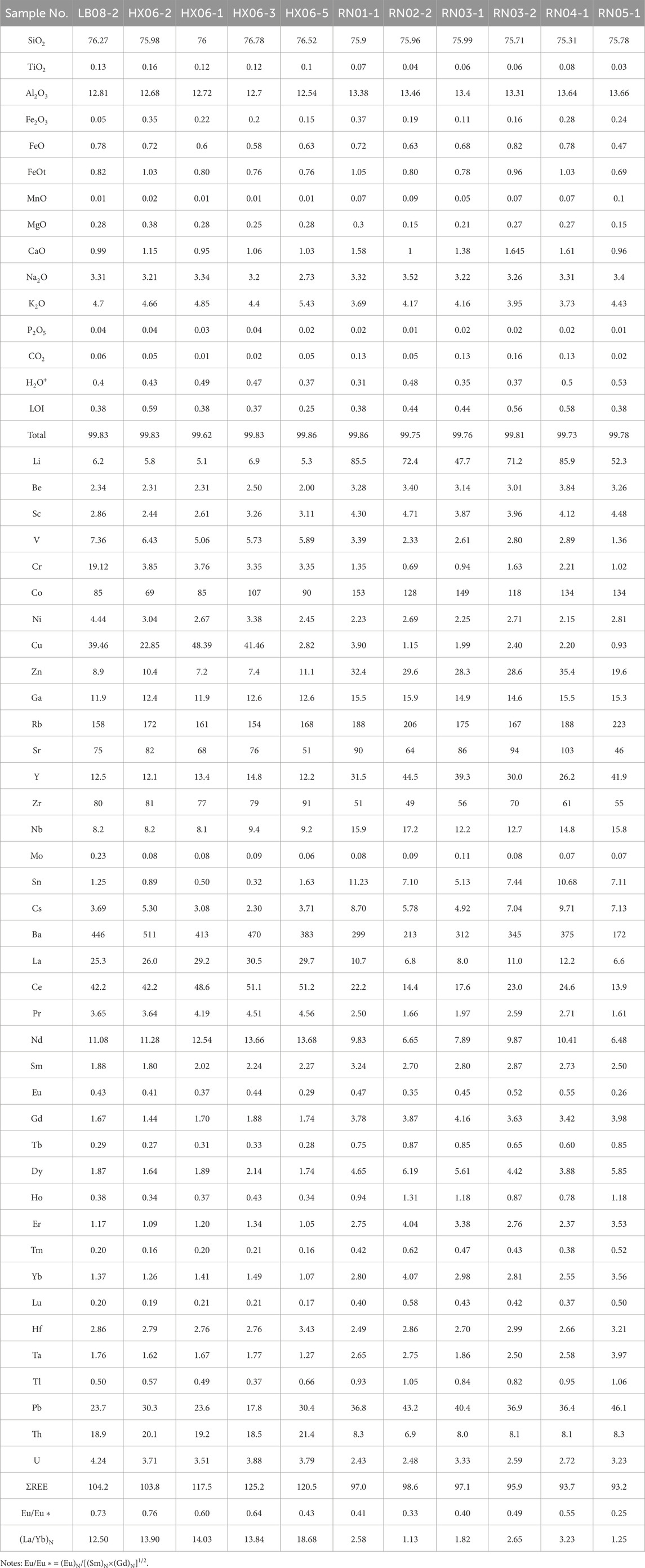
Table 3. Bulk-rock major and trace elements data for the Yushu granite and the Longbao granitic porphyry, central Tibetan Plateau.
Samples of the Longbao granitic porphyry were characterized by high SiO2 (75.98–76.78 wt%) and K2O (4.40–5.43 wt%) content, but low CaO (0.99–1.15 wt%), TiO2 (0.10–0.16 wt%), FeOT (0.76–1.03 wt%), MgO (0.25–0.38 wt%), and P2O5 (0.02–0.04 wt%) content (Table 3). These were plotted in the field of high-K calc-alkaline and magnesian series in classification diagrams (Figures 7B, C). In addition, those samples were slightly peraluminous, with A/CNK ratios ranging from 1.02 to 1.06 (Figure 7D). Those samples had higher total rare earth element concentrations (∑REE = 103.8–125.2 ppm) than the Yushu granite samples. In chondrite-normalized REE diagrams, the samples were characterized by enrichment of LREE relative to HREE [(La/Yb)N = 12.50–18.68], a flat HREE pattern [(Gd/Yb)N = 0.92–1.32], and variable negative Eu anomalies (δEu = 0.43–0.76; Figure 8A). On a primitive mantle-normalized spider diagram, all the samples showed obvious depletion of Ba, Nb, Sr, P, and Ti, but enrichment of Th, U, and Rb (Figure 8B).
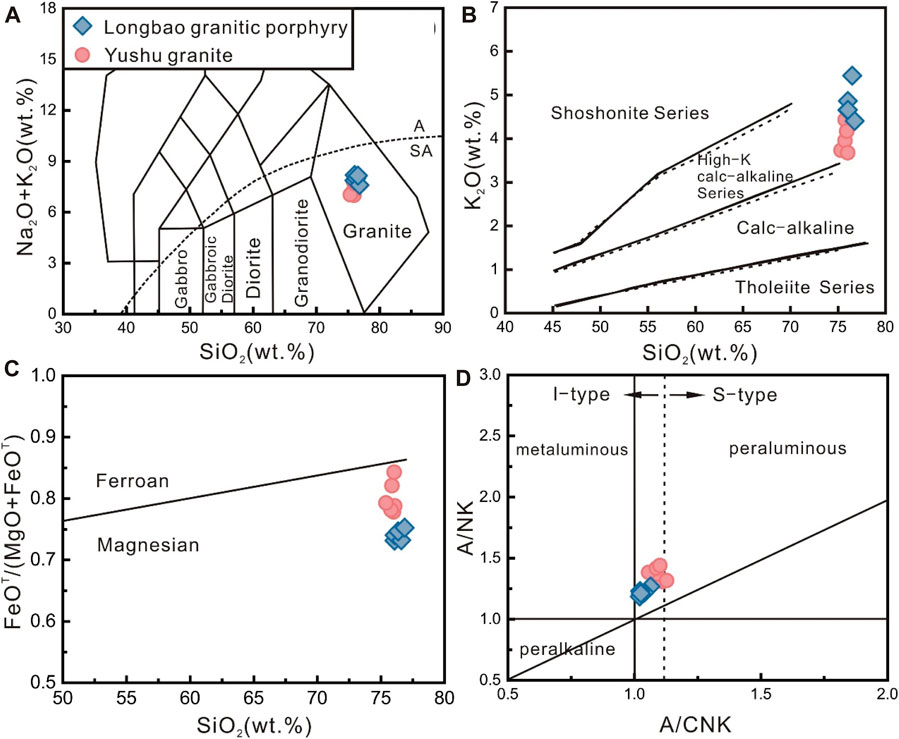
Figure 7. Classification diagrams for the granites studied. (A) (Na2O+ K2O) vs SiO2 diagram (Middlemost, 1994); alkaline field (A) and sub-alkaline field (SA) after Irvine and Baragar (1971). (B) K2O vs SiO2 diagram (Peccerillo and Taylor, 1976). (C) FeOT/(FeOT + MgO) vs SiO2 diagram (Frost et al., 2001). (D) A/NK vs A/CNK diagram (Maniar and Piccoli, 1989).
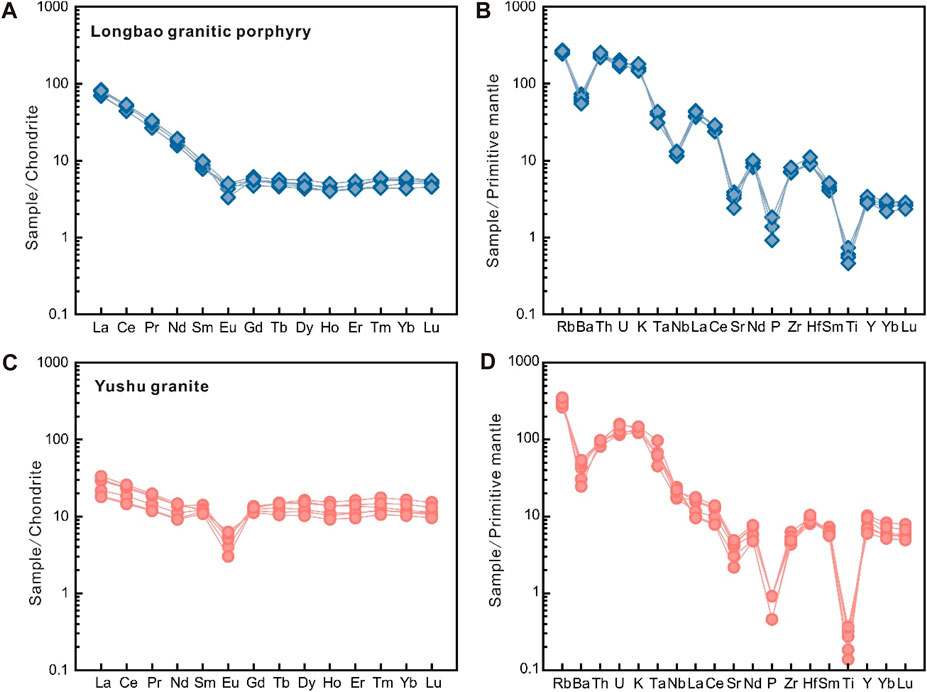
Figure 8. Chondrite-normalized REE patterns (A,C) and primitive mantle-normalized spider diagrams (B,D) for the granites studied. Normalizing values of chondrite and primitive mantle are from Taylor and McLennan (1985) and Sun and McDonough (1989), respectively.
Samples of the Yushu granite showed high SiO2 (75.31–75.99 wt%) and K2O (3.69–4.43 wt%) content, but low CaO (0.95–1.65 wt%), TiO2 (0.03–0.08 wt%), FeOT (0.69–1.05 wt%), MgO (0.15–0.30 wt%), and P2O5 (0.01–0.02 wt%) content (Table 3). They were plotted in the field of high-K calc-alkaline and magnesian series according to classification diagrams (Figures 7B, C). In addition, these samples were slightly to strongly peraluminous, with A/CNK ratios ranging from 1.05 to 1.13 (Figure 7D). The samples were characterized by moderate concentrations of total rare earth elements (∑REE = 93.2–98.6 ppm) and negative Eu anomalies (δEu = 0.25–0.55). On a chondrite-normalized diagram, all the samples exhibited flat REE patterns [(La/Yb)N = 1.13–3.23 (Gd/Yb)N = 0.77–1.13] (Figure 8C). On a primitive mantle-normalized spider diagram, all the samples were enriched in large-ion lithophile elements (LILEs), such as Rb, Th, and U, and exhibited depleted high field strength elements (HFSEs), such as Sr, Ba, P, and Ti (Figure 8D).
4.4 Whole-rock Sr-Nd isotopes
Whole-rock Sr-Nd isotopic compositions of the granites studied are listed in Table 4; initial Sr and Nd isotopic ratios (ISr) and εNd(t) values were calculated based on the timing of 215 Ma. The sample from the Longbao granitic porphyry showed an initial 87Sr/86Sr ratio of 0.7120 and εNd(t) value of −7.58, with the corresponding depleted-mantle model age (T2DM) of 1.61 Ga. The samples from the Yushu granite were characterized by relatively high (87Sr/86Sr)i ratios, in the range of 0.7121–0.7136, and low εNd(t) values of −8.58 to −7.97, with a T2DM age of 1.64–1.69 Ga.
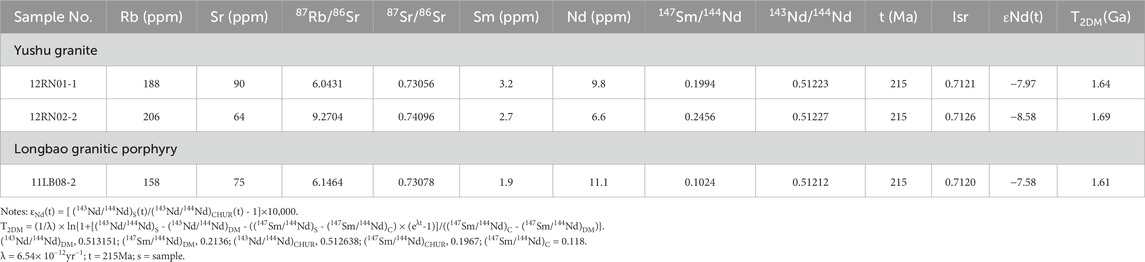
Table 4. Bulk-rock Sr-Nd isotopic compositions of the typical samples in the central Tibetan Plateau.
5 Discussion
5.1 Genetic types
In a seminal paper, Chappell and White (1974) classified the granites of the Lachlan Fold Belt (LFB) in south-eastern Australia into two end-members: I- and S-type granites. This classification has become well-established in most granites that have diverse sources and has developed into the current I-S-M-A classification of granitoids. M-type granites, which are distinguished by relatively low K2O (<1.9 wt%) and Rb (<48 ppm) values, are derived directly from the melting of subducted oceanic crust or overlying mantle (Saito et al., 2004). A-type (alkaline, anhydrous, and anorogenic) granites exhibit notably high values of 10,000×Ga/Al (>2.6) and Zr+Nb+Ce+Y (>350 ppm) (Whalen et al., 1987), which are associated with ferroan granitic melts (Frost et al., 2001). The samples of Yushu granite and Longbao granitic porphyry were plotted in the field of high-K calc-alkalic and magnesian series (Figure 7) and exhibited low values of 10,000×Ga/Al; these characteristics do not correspond with those of the A- and M-type granites. The granites studied consisted primarily of plagioclase, alkali-feldspar, and quartz, with minor biotite and an absence of amphibole (Figure 2), tending to be close to haplogranite (near minimum-temperature melt; King et al., 1997). Additionally, it is very difficult to discriminate them as I-type or S-type, because both high-silica I- and S-type granites usually have analogous geochemical compositions and mineral assemblages (Wu et al., 2017).
5.2 Magma source and crustal melting
It is widely accepted that Sr-Nd isotopes can trace the magma sources of highly evolved granites, because it is difficult for magma differentiation to affect εNd(t) values and Sm/Nd ratios (DePaolo, 1988). Samples from the Longbao granitic porphyry and the Yushu granite exhibited relatively high initial 87Sr/86Sr isotope ratios (0.7120–0.7136) and negative εNd(t) values (−8.58 to −7.58), suggesting a large contribution from crustal components.
Two potential candidates involving the widespread Triassic turbidites and the Precambrian basement rocks have been proposed for the crustal sources of Triassic intermediate to acid rocks in the central Tibetan Plateau (Liu et al., 2021). Previous studies have demonstrated that the granites derived from melting of the Precambrian basement rocks had higher initial 87Sr/86Sr isotope ratios (0.7240–0.7390) and noticeably more negative εNd(t) values (−13.26 to −11.13; Tao et al., 2014; Liu et al., 2023), which are distinct from those of the high-silica granites studied here. The Sr-Nd isotopic compositions of the two suites of high-silica granites overlapped with those of the Triassic turbidites in the HBSG terrane (Figure 9), further indicating that the widespread turbidites might be a good candidate for the crustal sources.
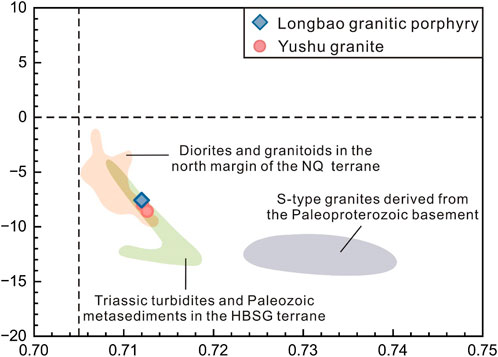
Figure 9. Plot of εNd(t) vs (87Sr/86Sr)i for the granites studied. Data sources: Triassic turbidites and Paleozoic metasediments in the HBSG terrane are from de Sigoyer et al. (2014), She et al. (2006), Zhang et al. (2012), and Zhao et al. (2022); Late Triassic S-type granitoids that were derived from the Paleoproterozoic basement rocks in the NQ terrane are from Liu et al. (2023) and Tao et al. (2014).
High-silica granites are usually considered to originate from pure crustal anatexis, and are usually linked with the process of crustal evolution (Clemens and Stevens, 2012). It is widely accepted that the continental crustal material is not water-enriched, and that the dehydration–melting reaction is the controlling factor in generating the crustal magma (Weinberg and Hasalová, 2015; Wu et al., 2020). Partial melting under vapor-absent and/or vapor-present conditions could produce different residual mineral phases and could cause various distributions of trace elements in the melts (Harris and Inger, 1992). Under vapor-absent conditions, the initial melt would have lower Ca, Sr, and Ba content and higher Rb/Sr ratios compared to vapor-present conditions, because more micas (enrichment of Rb than Sr) and less plagioclase (enrichment of Sr than Rb) are consumed (Harris and Inger, 1992; Gao et al., 2017). Although fractional crystallization of plagioclase could also result in low CaO and Sr content and a high Rb/Sr ratio, this was not the case for the high-silica granites examined. All the samples from the Longbao granitic porphyry had δEu values above 0.4, and this was also the case for most samples from the Yushu granite. These features indicate a relatively low to moderate degree of plagioclase fractionation (Gao et al., 2017; Zeng and Gao, 2017). Furthermore, fractional crystallization might be not the main factor controlling the generation of high-silica magma, because felsic melts usually have particularly high viscosity (Glazner, 2014). Thus, the low CaO and Sr content and the high Rb/Sr ratio might be more likely to have originated from the composition of the magma source, supporting the dehydration melting reaction (Figure 10). In addition, the proportions of An, Ab, and Or could represent the differences between vapor-absent and vapor-present conditions (Weinberg and Hasalová, 2015). On FeO-Na2O/K2O and An-Ab-Or diagrams based on experimental petrological data, the granites studied exhibited trends following the dehydration melting of Bt-Ms schists reported by Patiño Douce and Harris (1998) (Supplementary Figures S1A,B. Moreover, experimental studies have shown that melts derived from Ms-bearing and/or Bt-bearing sources exhibit higher K2O/Na2O ratios (>1.0) than melts derived from Amp-bearing sources (<1.0) (Gao et al., 2016). Samples from the Longbao granitic porphyry and the Yushu granite had relatively high ratios of K2O/Na2O (>1.0), suggesting that they might originate from Bt- and/or Ms-bearing sources. The variation in Rb/Sr, Sr, and Ba further supports the melting of a Ms-bearing crustal source (Figure 10).
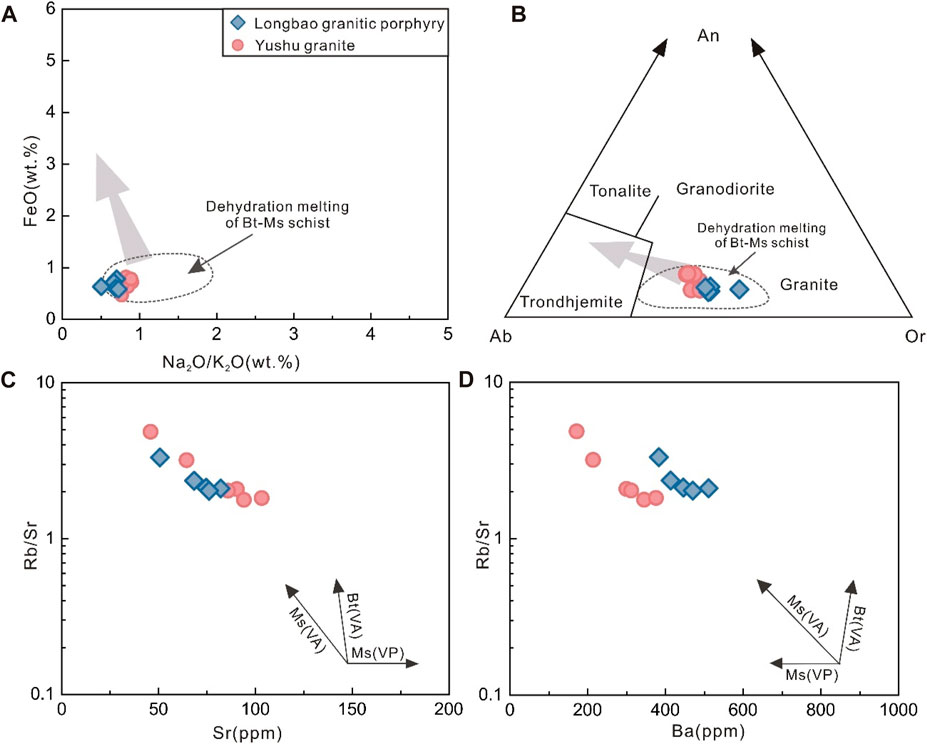
Figure 10. (A) FeO vs Na2O/K2O diagram. (B) An-Ab-Or normative triangle diagram. The circle of dehydration melting of Bt-Ms schist is from Patiño Douce and Harris (1998). Gray arrows point in the direction of melt composition change from dehydration melting to water-present melting. (C) Rb/Sr vs Sr diagram. (D) Rb/Sr vs Ba diagram (Inger and Harris, 1993). VP, vapor-present; VA, vapor-absent; Bt, biotite; Ms, muscovite.
5.3 Geodynamic relationship with the paleo-tethyan ocean
The Triassic tectonic evolution of the NQ terrane and adjacent HBSG terrane remains hotly debated, and two conflicting models, the “collision-related model” and the “subduction-related model,” have been employed to interpret the generation of Triassic magmatism in the central Tibetan Plateau (Zhang et al., 2007; Yuan et al., 2010; Liu et al., 2021).
The “collision-related model” proposes that the Palaeo-Tethyan ocean closed during Middle Triassic times, and the subsequent crustal thickening and lithospheric delamination triggered the Middle to Late Triassic magmatism (Zhang et al., 2007; Yuan et al., 2010). However, it can be argued based on the identification of a Middle to Upper Triassic submarine fan in the HBSG terrane (Ding et al., 2013) that the ocean could not have closed until Late Triassic times. The zircon U-Pb ages of MORB-type mafic rocks from the ophiolites in the Garzê-Litang suture zone are 239–232 Ma, implying that the Palaeo-Tethyan ocean still existed during Middle Triassic times (Liu et al., 2016a). A series of adakites-high-Mg andesites-Nb-rich basalts with ages of ∼229 Ma have been identified in the Tuotuohe area, indicating that the oceanic subduction might have begun at ∼229 Ma (Wang et al., 2008). With the southward subduction of the Garzê-Litang ocean, arc volcanic rocks with ages of 230–209 Ma (Yang et al., 2012; Zhao et al., 2014; Liu et al., 2016b; Liu et al., 2020; Liu et al., 2022) developed along the northwest–southeast direction (Figure 1). In addition, new paleolatitude data indicate that the final closure of the Palaeo-Tethyan ocean might have occurred at 213–204 Ma (Song et al., 2015). The timing of the ductile deformation of the collision stage may be recorded in metamorphic minerals (e.g., biotite and muscovite), and 40Ar-39Ar analyses of these have yielded ages of 201–193 Ma (Yang et al., 2012; Zhang et al., 2013). Therefore, the high-silica granites (217–214 Ma) in this study were probably formed in a subduction environment.
Moreover, samples from the Longbao granitic porphyry and the Yushu granite exhibited high apparent Ti-in-zircon temperatures, indicating a significant thermal anomaly at the deep crustal levels. Wang et al. (2008) proposed that the oceanic lithosphere might have subducted to depths of ∼85 km at ∼229 Ma. With this deepening of the depths of subduction, intensive eclogite-facies metamorphism increased the density of the subducted oceanic lithosphere, subsequently leading to slab roll-back (Liu et al., 2021). Slab roll-back in turn would induce upwelling of the asthenosphere and provide sufficient heat for crustal melting. If this was the case, partial melting of a Ms-bearing crustal source would have induced the Late Triassic magmatism in the North Qiangtang terrane, including the Longbao granitic porphyry and the Yushu granite.
6 Conclusion
(1) Two suites of Late Triassic high-silica granites have been identified in the North Qiangtang terrane in the central Tibetan Plateau. The results of zircon U-Pb dating indicate that the crystallization ages of those high-silica granites are 217–214 Ma.
(2) The Late Triassic high-silica granites were mainly produced by dehydration melting of a Ms-bearing source, and the Triassic turbidites might be a good candidate for the magma source.
(3) The high-silica granites (217–214 Ma) examined in this study were probably formed in a subduction environment, and partial melting of Triassic turbidites induced by slab roll-back might be the key factor controlling the origin of Late Triassic magmatism in the North Qiangtang terrane.
Data availability statement
The original contributions presented in the study are included in the article/Supplementary Material, further inquiries can be directed to the corresponding author.
Author contributions
CJ: Writing–original draft. BL: Writing–review and editing. YC: Investigation, Writing–review and editing. ZW: Writing–review and editing. CC: Writing–review and editing.
Funding
The author(s) declare financial support was received for the research, authorship, and/or publication of this article. This work has obtained financial support from the National Natural Science Foundation of China (Grant 41602122 and 41502050), the China Geological Survey (Grants DD20230024 and DD20230315), the basic scientific research project of the Chinese Academy of Geological Sciences (Grant JKY202209).
Acknowledgments
We thank Zhao-Chu Hu, Lian Zhou, and Hai-Hong Chen for their help with the analytical work.
Conflict of interest
The authors declare that the research was conducted in the absence of any commercial or financial relationships that could be construed as a potential conflict of interest.
Publisher’s note
All claims expressed in this article are solely those of the authors and do not necessarily represent those of their affiliated organizations, or those of the publisher, the editors and the reviewers. Any product that may be evaluated in this article, or claim that may be made by its manufacturer, is not guaranteed or endorsed by the publisher.
Supplementary material
The Supplementary Material for this article can be found online at: https://www.frontiersin.org/articles/10.3389/feart.2024.1354185/full#supplementary-material
SUPPLEMENTARY FIGURE S1:Plots of FeOT, MgO, TiO2, P2O5, Na2O, K2O, Nb, Sr, Rb, Ba, La, and Th versus LOI for the studied granites studied.
References
Andersen, T. (2002). Correction of common lead in U–Pb analyses that do not report 204Pb. Chem. Geol. 192, 59–79. doi:10.1016/s0009-2541(02)00195-x
Ballouard, C., Poujol, M., Boulvais, P., Branquet, Y., Tartèse, R., and Vigneresse, J. L. (2016). Nb-Ta fractionation in peraluminous granites: a marker of the magmatic-hydrothermal transition. Geology 44, 231–234. doi:10.1130/g37475.1
Brown, M. (2013). Granite: from genesis to emplacement. Geol. Soc. Am. Bull. 125, 1079–1113. doi:10.1130/b30877.1
Campbell, I. H., and Taylor, S. R. (1983). No water, no granites - No oceans, no continents. Geophys. Res. Lett. 10, 1061–1064. doi:10.1029/gl010i011p01061
Chen, G., Hu, F., Robertson, A. H., Garzanti, E., Zhang, S., and Wu, F. Y. (2023). A combined methodology for reconstructing source-to-sink basin evolution, exemplified by the Triassic Songpan–Ganzi basin, central China. Sediment. Geol. 458, 106529. doi:10.1016/j.sedgeo.2023.106529
Chen, J. Y., Yang, J. H., Zhang, J. H., Sun, J. F., Zhu, Y. S., and Hartung, E. (2021). Generation of Cretaceous high-silica granite by complementary crystal accumulation and silicic melt extraction in the coastal region of southeastern China. GSA Bull. 134, 201–222. doi:10.1130/b35745.1
Clemens, J. D., and Stevens, G. (2012). What controls chemical variation in granitic magmas? Lithos 134-135, 317–329. doi:10.1016/j.lithos.2012.01.001
Deng, G., Jiang, D., Zhang, R., Huang, J., Zhang, X., and Huang, F. (2022). Barium isotopes reveal the role of deep magmatic fluids in magmatic-hydrothermal evolution and tin enrichment in granites. Earth Planet. Sci. Lett. 117724, 594.
DePaolo, D. J. (1988). Age dependence of the composition of continental crust: evidence from Nd isotopic variations in granitic rocks. Earth Planet. Sci. Lett. 90, 263–271. doi:10.1016/0012-821x(88)90130-6
de Sigoyer, J., Vanderhaeghe, O., Duchêne, S., and Billerot, A. (2014). Generation and emplacement of Triassic granitoids within the Songpan Ganze accretionary-orogenic wedge in a context of slab retreat accommodated by tear faulting, Eastern Tibetan plateau, China. J. Asian Earth Sci. 88, 192–216. doi:10.1016/j.jseaes.2014.01.010
Ding, L., Yang, D., Cai, F. L., Pullen, A., Kapp, P., Gehrels, G. E., et al. (2013). Provenance analysis of the mesozoic hoh-xil-songpan-ganzi turbidites in northern tibet: implications for the tectonic evolution of the eastern paleo-tethys ocean. Tectonics 32, 34–48. doi:10.1002/tect.20013
Ferry, J. M., and Watson, E. B. (2007). New thermodynamic models and revised calibrations for the Ti-in-zircon and Zr-in-rutile thermometers. Contributions Mineralogy Petrology 154, 429–437. doi:10.1007/s00410-007-0201-0
Frost, B., Barnes, C., Collins, W., Arculus, R., Ellis, D., and Frost, C. (2001). A geochemical classification for granitic rocks. J. Petrology 42, 2033–2048. doi:10.1093/petrology/42.11.2033
Frost, C. D., Swapp, S. M., Frost, B. R., Finley-Blasi, L., and Fitz-Gerald, D. B. (2016). Leucogranites of the teton range, Wyoming: a record of archean collisional orogeny. Geochimica Cosmochimica Acta 185, 528–549. doi:10.1016/j.gca.2015.12.015
Gao, L. E., Zeng, L. S., and Asimow, P. D. (2017). Contrasting geochemical signatures of fluid-absent versus fluid-fluxed melting of muscovite in metasedimentary sources: the Himalayan leucogranites. Geology 45, 39–42. doi:10.1130/g38336.1
Gao, P., Zheng, Y. F., and Zhao, Z. F. (2016). Experimental melts from crustal rocks: a lithochemical constraint on granite petrogenesis. Lithos 266-267, 133–157. doi:10.1016/j.lithos.2016.10.005
Glazner, A. F. (2014). Magmatic life at low Reynolds number. Geology 42, 935–938. doi:10.1130/g36078.1
Glazner, A. F., Coleman, D. S., and Bartley, J. M. (2008). The tenuous connection between high-silica rhyolites and granodiorite plutons. Geology 36, 183. doi:10.1130/g24496a.1
Harris, N. B. W., and Inger, S. (1992). Trace element modelling of pelite-derived granites. Contributions Mineralogy Petrology 110, 46–56. doi:10.1007/bf00310881
He, S. P., Li, R. S., Wang, C., Gu, P. Y., Shi, C., and Zha, X. F. (2013). Reaseach on the formation age of Ningduo rock group in Changdu block: evidence for the existence of basement in the North Qiangtang. Earth Sci. Front. 20, 15–24.
Hoskin, P. W. O. (2005). Trace-element composition of hydrothermal zircon and the alteration of Hadean zircon from the Jack Hills, Australia. Geochimica Cosmochimica Acta 69, 637–648. doi:10.1016/j.gca.2004.07.006
Hoskin, P. W. O., and Schaltegger, U. (2003). The composition of zircon and igneous and metamorphic petrogenesis. Rev. Mineralogy Geochem. 53, 27–62. doi:10.2113/0530027
Inger, S., and Harris, N. (1993). Geochemical constraints on leucogranite magmatism in the langtang valley, Nepal Himalaya. J. Petrology 34, 345–368. doi:10.1093/petrology/34.2.345
Irvine, T. N., and Baragar, W. R. A. (1971). A guide to the chemical classification of the common volcanic rocks. Can. J. Earth Sci. 8, 523–548. doi:10.1139/e71-055
Jian, X., Weislogel, A., and Pullen, A. (2019). Triassic sedimentary filling and closure of the eastern paleo-Tethys Ocean: new insights from detrital zircon geochronology of Songpan-Ganzi, Yidun, and west Qinling flysch in eastern Tibet. Tectonics 38 (2), 767–787. doi:10.1029/2018tc005300
Keller, C. B., Schoene, B., Barboni, M., Samperton, K. M., and Husson, J. M. (2015). Volcanic–plutonic parity and the differentiation of the continental crust. Nature 523, 301–307. doi:10.1038/nature14584
King, P. L., White, A. J. R., Chappell, B. W., and Allen, C. M. (1997). Characterization and origin of aluminous A-type granites from the Lachlan Fold Belt, southeastern Australia. J. Petrology 38, 371–391. doi:10.1093/petroj/38.3.371
Lee, C. T. A., and Morton, D. M. (2015). High silica granites: terminal porosity and crystal settling in shallow magma chambers. Earth Planet. Sci. Lett. 409, 23–31. doi:10.1016/j.epsl.2014.10.040
Liu, B., Ma, C. Q., Guo, Y. H., Xiong, F. H., Guo, P., and Zhang, X. (2016a). Petrogenesis and tectonic implications of Triassic mafic complexes with MORB/OIB affinities from the western Garzê-Litang ophiolitic mélange, central Tibetan Plateau. Lithos 260, 253–267. doi:10.1016/j.lithos.2016.06.009
Liu, B., Ma, C. Q., Huang, J., Xiong, F. H., Zhang, X., and Guo, Y. H. (2016b). Petrogenetic mechanism and tectonic significance of Triassic Yushu volcanic rocks in the northern part of the North Qiangtang Terrane. Acta Petrologica Mineralogica 35, 1–15.
Liu, B., Ma, C. Q., Tang, Y. J., Dong, H., Xu, Y., Zhao, S. Q., et al. (2021). Triassic high-Mg andesitic magmatism induced by sediment melt-peridotite interactions in the central Tibetan Plateau. Lithos 106266, 398–399.
Liu, B., Tang, Y. J., Xing, L. Y., Xu, Y., Zhao, S. Q., Sun, Y., et al. (2022). Triassic calc-alkaline lamprophyre dykes from the North Qiangtang, central Tibetan Plateau: evidence for a subduction-modified lithospheric mantle. Geol. Mag. 159 (3), 407–420. doi:10.1017/s001675682100100x
Liu, B., Xu, Y., Li, Q., Sun, Y., Zhao, S. Q., Huang, J., et al. (2020). Origin of Triassic mafic magmatism in the North Qiangtang terrane, central Tibetan Plateau: implications for the development of a continental back-arc basin. J. Geol. Soc. 177, 826–842. doi:10.1144/jgs2019-130
Liu, B., Xu, Y., Ma, C. Q., Li, F. L., Zhao, S. Q., Zhan, J. M., et al. (2023). Petrogenesis and geodynamic setting of the ningduo peraluminous granites from the North Qiangtang terrane. Earth Sci., 1–32.
Liu, Y., Xiao, W. J., Windley, B. F., Li, R. S., Ji, W. H., Zhou, K. F., et al. (2019). Late Triassic ridge subduction of Paleotethys: insights from high-Mg granitoids in the Songpan-Ganzi area of northern Tibet. Lithos 334-335, 254–272. doi:10.1016/j.lithos.2019.03.012
Liu, Y. S., Gao, S., Hu, Z. C., Gao, C. G., Zong, K. Q., and Wang, D. B. (2010). Continental and oceanic crust recycling-induced melt-peridotite interactions in the trans-north China orogen: U-Pb dating, Hf isotopes and trace elements in zircons from mantle xenoliths. J. Petrology 51, 537–571. doi:10.1093/petrology/egp082
Liu, Y. S., Zong, K. Q., Kelemen, P. B., and Gao, S. (2008). Geochemistry and magmatic history of eclogites and ultramafic rocks from the Chinese continental scientific drill hole: subduction and ultrahigh-pressure metamorphism of lower crustal cumulates. Chem. Geol. 247, 133–153. doi:10.1016/j.chemgeo.2007.10.016
Maniar, P. D., and Piccoli, P. M. (1989). Tectonic discrimination of granitoids. Geol. Soc. Am. Bull. 101, 635–643. doi:10.1130/0016-7606(1989)101<0635:tdog>2.3.co;2
McDonough, W. F., and Sun, S. S. (1995). The composition of the Earth. Chem. Geol. 120, 223–253. doi:10.1016/0009-2541(94)00140-4
Middlemost, E. A. K. (1994). Naming materials in the magma/igneous rock system. Earth-Science Rev. 37, 215–224. doi:10.1016/0012-8252(94)90029-9
Miller, C. F., McDowell, S. M., and Mapes, R. W. (2003). Hot and cold granites? Implications of zircon saturation temperatures and preservation of inheritance. Geology 31, 529. doi:10.1130/0091-7613(2003)031<0529:hacgio>2.0.co;2
Patiño Douce, A. E., and Harris, N. (1998). Experimental constraints on himalayan anatexis. J. Petrology 39, 689–710. doi:10.1093/petrology/39.4.689
Peccerillo, A., and Taylor, S. R. (1976). Geochemistry of eocene calc-alkaline volcanic rocks from the Kastamonu area, Northern Turkey. Contributions Mineralogy Petrology 58, 63–81. doi:10.1007/bf00384745
Roger, F., Arnaud, N., Gilder, S., Tapponnier, P., Jolivet, M., Brunel, M., et al. (2003). Geochronological and geochemical constraints on Mesozoic suturing in east central Tibet. Tectonics 22. doi:10.1029/2002tc001466
Saito, S., Arima, M., Nakajima, T., and Kimura, J. I. (2004). Petrogenesis of Ashigawa and Tonogi granitic intrusions, southern part of the Miocene Kofu Granitic Complex, central Japan: M-type granite in the Izu arc collision zone. J. Mineralogical Petrological Sci. 99, 104–117. doi:10.2465/jmps.99.104
Schiller, D., and Finger, F. (2019). Application of Ti-in-zircon thermometry to granite studies: problems and possible solutions. Contributions Mineralogy Petrology 174, 51. doi:10.1007/s00410-019-1585-3
She, Z., Ma, C., Mason, R., Li, J., Wang, G., and Lei, Y. (2006). Provenance of the triassic songpan–ganzi flysch, west China. Chem. Geol. 231, 159–175. doi:10.1016/j.chemgeo.2006.01.001
Shuai, X., Li, S. M., Zhu, D. C., Wang, Q., Zhang, L. L., and Zhao, Z. D. (2021). Tetrad effect of rare earth elements caused by fractional crystallization in high-silica granites: an example from central Tibet. Lithos, 384–385.
Song, P. P., Ding, L., Li, Z. Y., Lippert, P. C., Yang, T. S., Zhao, X. X., et al. (2015). Late triassic paleolatitude of the Qiangtang block: implications for the closure of the paleo-tethys ocean. Earth Planet. Sci. Lett. 424, 69–83. doi:10.1016/j.epsl.2015.05.020
Sun, S. S., and McDonough, W. F. (1989). Chemical and isotopic systematics of oceanic basalts: implications for mantle composition and processes. Geol. Soc. Spec. Publ. 42, 313–345.
Tan, J., Wei, J. H., Zhao, S. Q., Li, Y. J., Liu, Y., Liu, X. Y., et al. (2020). Petrogenesis of Late Triassic high-Mg diorites and associated granitoids with implications for Paleo-Tethys evolution in the northeast Tibetan Plateau. GSA Bull. 132, 955–976. doi:10.1130/b35225.1
Tao, Y., Bi, X. W., Li, C. S., Hu, R. Z., Li, Y. B., and Liao, M. Y. (2014). Geochronology, petrogenesis and tectonic significance of the Jitang granitic pluton in eastern Tibet, SW China. Lithos 184-187, 314–323. doi:10.1016/j.lithos.2013.10.031
Taylor, S. R., and McLennan, S. M. (1985). The continental crust: its composition and evolution. An examination of the geochemical record preserved in sedimentary rocks. Oxford: Blackwell Scientific Publications, 328.
Wang, Q., Wyman, D. A., Xu, J., Wan, Y., Li, C., Zi, F., et al. (2008). Triassic Nb-enriched basalts, magnesian andesites, and adakites of the Qiangtang terrane (Central Tibet): evidence for metasomatism by slab-derived melts in the mantle wedge. Contributions Mineralogy Petrology 155, 473–490. doi:10.1007/s00410-007-0253-1
Wang, Z. L. (2009). Study of multiple orogeny and metallogenesis inYushu area, northern segment of sanjiang. Beijing: Chinese Academy of Geological Sciences, 122.
Weinberg, R. F., and Hasalová, P. (2015). Water-fluxed melting of the continental crust: a review. Lithos 212-215, 158–188. doi:10.1016/j.lithos.2014.08.021
Weislogel, A. L., Graham, S. A., Chang, E. Z., Wooden, J. L., Gehrels, G. E., and Yang, H. (2006). Detrital zircon provenance of the late triassic songpan-ganzi complex: sedimentary record of collision of the North and south China blocks. Geology 34 (2), 97–100. doi:10.1130/g21929.1
Whalen, J. B., Currie, K. L., and Chappell, B. W. (1987). A-type granites: geochemical characteristics, discrimination and petrogenesis. Contributions Mineralogy Petrology 95, 407–419. doi:10.1007/bf00402202
Wu, F. Y., Liu, X. C., Ji, W. Q., Wang, J. M., and Yang, L. (2017). Highly fractionated granites: recognition and research. Sci. China Earth Sci. 60, 1201–1219. doi:10.1007/s11430-016-5139-1
Wu, F. Y., Liu, X. C., Liu, Z. C., Wang, R. C., Xie, L., Wang, J. M., et al. (2020). Highly fractionated Himalayan leucogranites and associated rare-metal mineralization. Lithos, 352–353.
Xu, Z., Dilek, Y., Cao, H., Yang, J., Robinson, P., Ma, C., et al. (2015). Paleo-tethyan evolution of tibet as recorded in the east cimmerides and west cathaysides. J. Asian Earth Sci. 105, 320–337. doi:10.1016/j.jseaes.2015.01.021
Yang, T. N., Hou, Z. Q., Wang, Y., Zhang, H. R., and Wang, Z. L. (2012). Late paleozoic to early mesozoic tectonic evolution of northeast tibet: evidence from the triassic composite western jinsha-garzê-litang suture. Tectonics 31. doi:10.1029/2011tc003044
Yuan, C., Zhou, M. F., Sun, M., Zhao, Y. J., Wilde, S., Long, X. P., et al. (2010). Triassic granitoids in the eastern Songpan Ganzi Fold Belt, SW China: magmatic response to geodynamics of the deep lithosphere. Earth Planet. Sci. Lett. 290, 481–492. doi:10.1016/j.epsl.2010.01.005
Zeng, L. S., and Gao, L. E. (2017). Cenozoic crustal anatexis and the leucogranites in the Himalayan collisional orogenic belt. Acta Petrol. Sin. 33 (5), 1420–1444.
Zhang, H. F., Parrish, R., Zhang, L., Xu, W. C., Yuan, H. L., Gao, S., et al. (2007). A-type granite and adakitic magmatism association in Songpan–Garze fold belt, eastern Tibetan Plateau: implication for lithospheric delamination. Lithos 97, 323–335. doi:10.1016/j.lithos.2007.01.002
Zhang, H. R., Yang, T. N., Hou, Z. Q., Song, Y. C., Cheng, X. F., Ding, Y., et al. (2013). Chronology and geochemistry of mylonitic quartz diorites in the Yushu melange, central Tibet. Acta Petrol. Sin. 29, 3871–3882.
Zhang, K. J., Li, B., and Wei, Q. G. (2012). Diversified provenance of the songpan-ganzi triassic turbidites, Central China: constraints from geochemistry and Nd isotopes. J. Geol. 120, 69–82. doi:10.1086/662716
Zhao, H., Chen, B., Huang, C., Bao, C., Yang, Q., and Cao, R. (2022). Geochemical and Sr–Nd–Li isotopic constraints on the genesis of the Jiajika Li-rich pegmatites, eastern Tibetan Plateau: implications for Li mineralization. Contributions Mineralogy Petrology 177 (1), 4. doi:10.1007/s00410-021-01869-3
Zhao, S. Q., Tan, J., Wei, J. H., Tian, N., Zhang, D. H., Liang, S. N., et al. (2014). Late Triassic Batang Group arc volcanic rocks in the northeastern margin of Qiangtang terrane, northern Tibet: partial melting of juvenile crust and implications for Paleo-Tethys ocean subduction. Int. J. Earth Sci. 104, 369–387. doi:10.1007/s00531-014-1080-z
Keywords: high-silica granites, crustal melting, triassic turbidites, north qiangtang, central Tibetan Plateau
Citation: Ji C, Liu B, Chen Y, Wu Z and Chen C (2024) Petrogenesis of late triassic high-silica granites in the North Qiangtang terrane, central Tibetan Plateau. Front. Earth Sci. 12:1354185. doi: 10.3389/feart.2024.1354185
Received: 12 December 2023; Accepted: 13 February 2024;
Published: 02 April 2024.
Edited by:
Wei-Qiang Ji, Chinese Academy of Sciences (CAS), ChinaReviewed by:
Fangyang Hu, Institute of Geology and Geophysics (CAS), ChinaGong-Jian Tang, Chinese Academy of Sciences (CAS), China
Copyright © 2024 Ji, Liu, Chen, Wu and Chen. This is an open-access article distributed under the terms of the Creative Commons Attribution License (CC BY). The use, distribution or reproduction in other forums is permitted, provided the original author(s) and the copyright owner(s) are credited and that the original publication in this journal is cited, in accordance with accepted academic practice. No use, distribution or reproduction is permitted which does not comply with these terms.
*Correspondence: Bin Liu, YmlubGl1QHlhbmd0emV1LmVkdS5jbg==