- 1Sustainable Energy Technologies Center, College of Engineering, King Saud University, Riyadh, Saudi Arabia
- 2Department of Chemistry, College of Science, King Saud University, Riyadh, Saudi Arabia
- 3Engineer Abdullah Bugshan Research Chair for Dental and Oral Rehabilitation, College of Dentistry, King Saud University, Riyadh, Saudi Arabia
- 4Department of Power Engineering and Turbomachinery, Silesian University of Technology, Gliwice, Poland
Due to the rising demand for energy and the imperative to achieve net-zero carbon emissions, there is a growing focus on nuclear energy for its high efficiency as a clean energy source with minimal direct greenhouse gas emissions. The Kingdom of Saudi Arabia has set forth ambitious plans to construct multiple nuclear power plants in the near future. It is worth noting that phosphate rocks and desalination concentrate both contain relevant concentrations of naturally occurring uranium, presenting potential domestic uranium sources for the envisaged nuclear reactor fleet. This study offers a first systematic overview of the potential quantities of uranium that could theoretically be recovered during seawater desalination and phosphoric acid production in Saudi Arabia using best available technologies. It was found that in 2021 approximately 447–596 t natural uranium could have theoretically been recovered during phosphoric acid production in the Kingdom of Saudi Arabia. In addition, there were also 6.5 t uranium associated with seawater that was desalinated in 2021. If recovered the amounts would theoretically be able to provide 12%–16% (uranium from phosphoric acid) and 0.2% (uranium from seawater desalination) of the annual uranium requirements of the projected Saudi nuclear power plant fleet in 2040. As a result, we strongly recommend fostering research on unconventional uranium recovery during phosphoric acid production by promoting public-private partnerships that have the potential to develop industrial scale solutions.
1 Introduction
According to the World Bank the Kingdom of Saudi Arabia has one of the highest per capita electricity consumptions (9,444 kWh) in the world and according to the International Energy Agency nearly 34% of this electricity is produced by consuming domestic oil resources. Residential electricity consumption accounts for 49.4%, with a 2% annual growth rate (Damoom et al., 2019). The continued rise in electricity demand may have serious environmental implications because of the large CO2 emissions, and oil used domestically reduces potential revenues from exporting it leading to potential negative economic implications as well. Thus, alternative energy resources should be investigated to address power shortages, revenue generation, and environmental pollution.
Nuclear and renewable energy are both viable low-carbon energy options that can reduce the domestic oil consumption in the Kingdom of Saudi Arabia. Under the Vision 2030 initiative, specifically the Saudi Green Initiative, the Kingdom of Saudi Arabia plans to achieve net zero emission by 2060, reduce carbon emissions by more than 278 million t per year and transform 50% its electricity production to renewable energy sources using non-fossil fuels (KSA Vision, 2030). To meet these initiatives, the construction of 15 nuclear reactors with a total capacity of 17.6 GW has been proposed by K.A.CARE (King Abdullah City for Atomic and Renewable Energy) that is tasked with the energy transition in the Kingdom of Saudi Arabia. Nuclear power plant construction is supported by a majority of citizens (Alruwaished et al., 2023; Alzahrani et al., 2023) and the nuclear power program initiative is planned to be completed by 2040 (WNA, 2023a). The projected 15 large nuclear power reactors will each require approximately 25 t of slightly enriched (approximately 4.5%) uranium or approximately 250 t of natural uranium that is then enriched using modern centrifuge enrichment technology, per year for continuous operation (NuclearPower, 2023). In addition, some 1,000 t of natural uranium equivalent will be needed for every “fresh” or new reactor core.
Uranium is a relatively common element in the earth’s crust, with an average concentration of 2.8 ppm (WNA, 2023b) and can be found in trace amounts in various rocks, such as phosphate ores, referred to here as phosphate rocks, that are mined for their phosphorus (P) content, seawater and different uranium ores. Figure 1 provides an overview of the uranium concentrations in seawater, different phosphate rocks, and different uranium ores. The Kingdom of Saudi Arabia is a major phosphate rock producing country and has the largest fleet of desalination plants in the world. Although uranium recovery from phosphate rocks is presently not economically viable this might change with increasing uranium prices and coextraction of other valuable byproducts such as rare earth elements (Wu et al., 2017; Hakkar et al., 2021). In addition, reduced uranium concentrations in fertilizers as a result of stricter environmental regulations as well as strategic considerations (Al-Sahidi and Haghirian, 2020) might create incentives for domestic production of natural uranium. This work aims to provide a first estimate about the potential of providing natural uranium as a byproduct from phosphate rock processing to phosphoric acid and seawater desalination in the Kingdom of Saudi Arabia.
2 Methodology
In this work the amount of natural uranium that is required to sustain the future nuclear reactor fleet of the Kingdom of Saudi Arabia is estimated and it was further analyzed how much of this future uranium requirements could theoretically be supplied domestically from presently considered unconventional uranium resources associated with domestic phosphoric acid production and seawater desalination. All data used for the analysis is openly accessible, has been taken from authoritative sources such as the United States Geological Survey (USGS) or the Saline Water Conversion Corporation (SWCC) and has been referenced appropriately. It was assumed that during phosphate rock processing, the wet-phosphoric acid (WPA) process is used during which roughly 90% of the uranium transfers from the phosphate rock to the WPA from which approximately 95% could then be recovered using best available technologies (BAT) that have already been employed on industrial scale in Belgium, Iraq, Taiwan and the United States of America in the 1980s and 1990s before decreasing uranium prices made this practice uneconomic (Haneklaus, 2017a, b). In case of seawater desalination concentrate or direct uranium recovery from seawater the situation is less straightforward since similar industrially proven BAT technologies do, to the best of our knowledge, not exist yet. It was therefore decided to report the annual amount of uranium that is associated with desalinated seawater in the Kingdom of Saudi Arabia.
3 Results and discussion
3.1 Uranium from phosphate rocks
Phosphate rock, that is used to produce mineral fertilizers, is among the fifth most mined materials on earth making phosphate rocks one of the most relevant unconventional uranium resource. The recovery of uranium during fertilizer production has the added advantage that the radiotoxic heavy metal will largely be removed from the final fertilizer product and not be dissipated on agricultural soils from where it might leach into waterbodies (Bigalke et al., 2017; Hill et al., 2024; Mwalongo et al., 2024). Worldwide it is estimated that uranium recovered from the more than 400 fertilizer plants operating around the world could theoretically substitute some 8%–15% of global uranium mining (Gabriel et al., 2013; Ulrich et al., 2014; Haneklaus, 2021) with Morocco, one of the largest phosphate rock producing countries in the world, being (theoretically) able to shoulder 1.5% of this singlehandedly (Qamouche et al., 2021).
The global potential for uranium recovery from phosphate rocks can be estimated using production data and phosphate rock reserve data as it is for instance reported annually by the USGS (USGS, 2023) as well as average concentrations of uranium in the considered phosphate rocks. Table 1 provides estimated amounts of uranium associated with phosphate rocks globally using 2021 production data of the USGS. The average uranium concentrations in phosphate rock by country were estimated using the dataset provided by Van Kauwenbergh (1997).
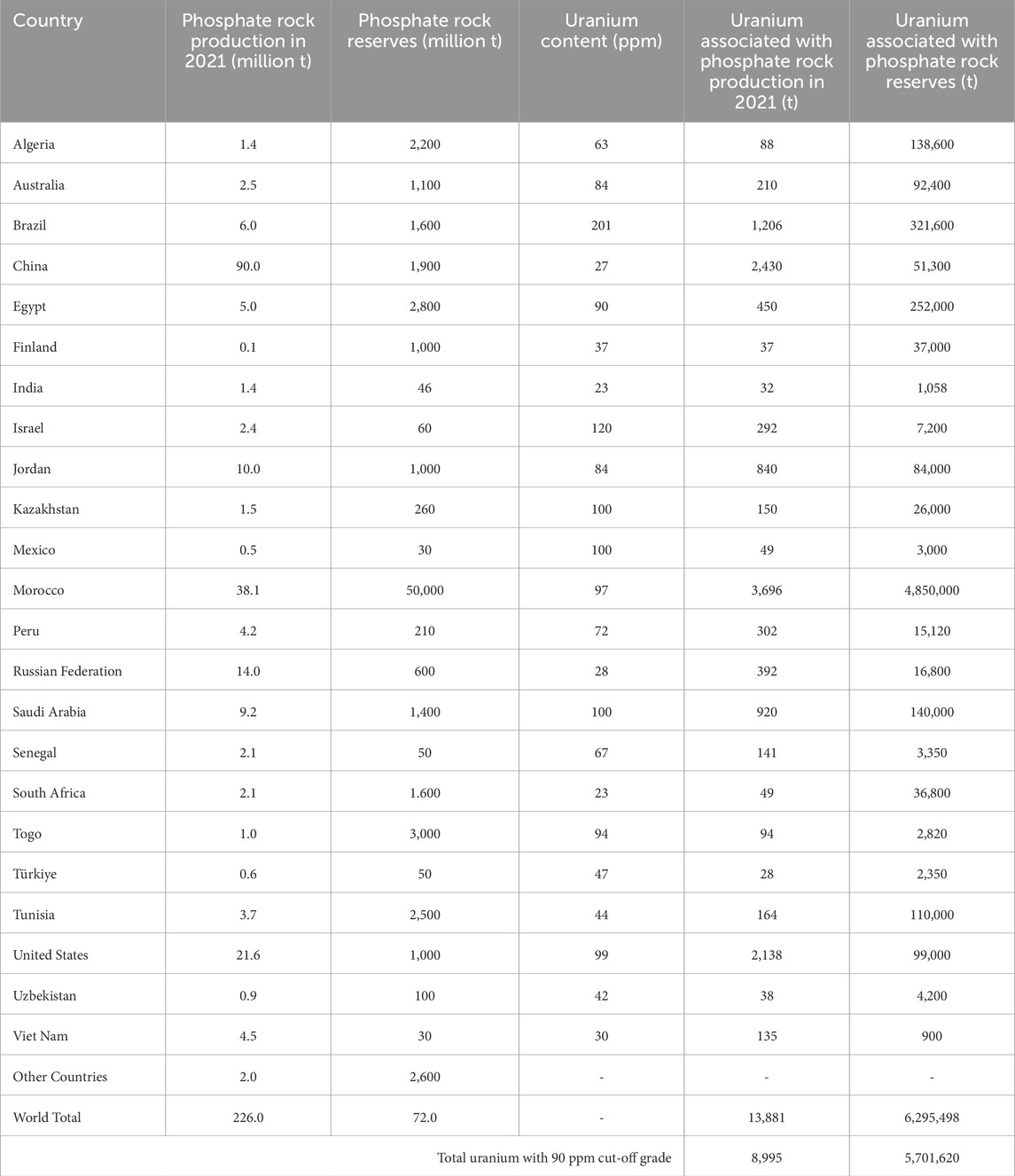
Table 1. Estimated amount of uranium associated with global phosphate rock production and reserves in 2021.
The quantitative assessment featured in Table 1 is a straightforward and simple analysis that provides insights concerning the amount of uranium linked to phosphate rock production and reserves globally. According to this simple assessment nearly 14,000 t of uranium are mined with phosphate rocks every year worldwide. Out of these 14,000 t, approximately 9,000 t have been observed as being connected to phosphate rocks with more elevated uranium concentrations of at least 90 ppm. Such phosphate rocks, or a cut-off grade of 90 ppm has been introduced in earlier analysis (Haneklaus, 2021) and it was proclaimed that such phosphate rocks might allow for commercial uranium recovery, while the rocks with lower uranium concentrations might not. The estimate in Table 1 provides a brief overview about the potential that uranium recovery from phosphate rocks could have on a global scale. In this context it is noteworthy that the uranium production in 2021 was approximately 47,500 t (NEA/IAEA, 2022; WNA, 2023c).
If uranium is recovered during phosphate rock development to mineral fertilizer using the historically proven solvent extraction process, approximately 95% of the uranium present in the WPA can be recovered and it can be estimated that 90% of this uranium transfers to the WPA. The amount of uranium that could theoretically be recovered from phosphate rock production in 2021 would thus be approximately 7,691 t or roughly 16% of the uranium production that year considering phosphate rocks with a uranium content of at least 90 ppm and Equations 1–3 that are provided below.
Where Ci (ppm) is the country specific uranium concentration in phosphate rock and Pi (t) is the corresponding quantity of imported phosphate rock. UWPA is the concentration of uranium in the WPA and Urecoverable is the quantity of uranium that can be recovered from the WPA using best available technology (BAT), such as industrially proven solvent extraction.
It is noteworthy that it is extremely unlikely that there will be large scale recovery of uranium at the more than 400 mineral phosphate fertilizer plants operational worldwide at the moment. The very brief calculation provided here does, however, indicate the relevant potential that uranium recovery from phosphoric acid has as an additional raw material source.
Detailed country-specific assessments of annual uranium recovery potentials are accessible for Argentina (19 t uranium in 2017) (Lopez et al., 2019), China (648 t uranium in 2016) (Shang et al., 2021), the EU (334 t uranium from phosphate rock imports in 2017) (Tulsidas et al., 2019), the Philippines (14–26 t uranium annually from phosphate rock imports in 2020–2022) (Diwa et al., 2023), the United Republic of Tanzania (30–40 t uranium annually as byproduct from local Minjingu phosphate rock mining and processing) (Haneklaus et al., 2024) and the United States of America (2,116 t uranium in 2014) (Kim et al., 2016; Steiner etal., 2020).
For the Kingdom of Saudi Arabia such an assessment has to the best of our knowledge not been performed yet. In this context, the International Atomic Energy Agency (IAEA) estimated that some 187,100 t uranium are associated with phosphate rocks in Saudi Arabia (NEA/IAEA, 2022) (a slightly higher estimate than the one in Table 1) and Al-Eshaikh et al. (2016) recommended the consideration of phosphate rocks and desalination concentrate as potential domestic sources of uranium in the Kingdom of Saudi Arabia. Since 2011, Saudi Arabia is a major phosphate rock producer (see Figure 2) and the USGS estimated that the country produced 9.2 million t phosphate rocks in 2021 and 8.0 million t in 2020 respectively (USGS, 2023). The average concentration of uranium in phosphate rocks in Saudi Arabia is probably in the order of 60–80 ppm with maximum local concentrations of 100–150 ppm (Khater et al., 2016; Mehboob et al., 2021), so that in 2021 some 447–596 t uranium could have theoretically been recovered during phosphate rock processing in Saudi Arabia if 90% of the uranium in the phosphate rock transfers to the WPA from where recovery usually takes place, and this recovery happens at an overall efficiency of 95%. It is noteworthy that previous studies (Haneklaus, 2021) have set the cut-off grade for (potentially economic) uranium recovery from phosphate rock at 90 ppm so that it needs to be investigated if uranium recovery from phosphates might at all make sense in Saudi Arabia. The set cut-off grade is obviously an oversimplification that does not consider specific rock types ore processing methods. There is a good chance that the artificially introduced cut-off grade can be revised if more innovative processing methods are used for uranium recovery, other impurities are better understood that could increase uranium recovery efficiencies or the throughput of the fertilizer plants are increased, resulting in advantages economics of scale. The historic potential uranium recovery from phosphate rocks for the Kingdom of Saudi Arabia is depicted in Figure 2 using USGS phosphate rock production data.
3.2 Uranium from water desalination
Uranium is also naturally present in seawater in concentrations as low as 3.3 parts per billion or 0.0033 ppm. As a result of the very large potential quantities of uranium in seawater that are estimated to be in the order of 4.5 billion metric t or 500 times of all identified terrestrial reserves, uranium recovery from seawater is actively researched. The uranium concentration in desalination concentrates or brine from desalination plants, basically concentrated seawater, can be approximately two times higher than the uranium concentration in regular seawater and can show far fewer impurities than the seawater itself (Loganathan et al., 2017; Zhang et al., 2021; Altay et al., 2022). There have been several different approaches for uranium recovery from seawater and/or desalination concentrate. The used approaches are usually based on adsorption, ion-exchange, coagulation and coprecipitation, solvent extraction, membrane filtration, biological separation, and foam separation. Among these methods, adsorption is often considered to be the most convenient, efficient, and low-cost technique (Sodaye et al., 2009; Loganathan et al., 2017). The Kingdom of Saudi Arabia has depended on desalinated water since the 1950s and has since then come to be the major desalinated water producer in the world. As of 2023, sixty percent of the country’s water comes from desalination, with groundwater accounting for less than 40 percent. As of 2023, the Kingdom of Saudi Arabia had a total of 33 desalination plants in seventeen locations run by the SWCC, responsible for approximately 69 percent of desalination in the Kingdom and 20 percent of the worldwide desalination capacity (ArabNews, 2023). Data on the water desalination per year in Saudi Arabia was sourced from the SWCC. In 2021 a total of 2,154 t of seawater was desalinated in the Kingdom of Saudi Arabia. Approximately 6.46 t uranium were associated with this seawater and could have (theoretically) been recovered. Figure 3 provides an overview of the uranium quantities associated with seawater used for desalination in the Kingdom of Saudi Arabia.
4 Conclusion and recommendations
The analysis presented here estimates that the Kingdom of Saudi Arabia will after the erection of 15 large commercial nuclear power plants in 2040 have annual natural uranium requirements of approximately 3,750 t per year. In addition, another 1,000 t per newly installed reactor unit will be required for every fresh nuclear reactor core. This uranium is often provided by the vendor or developer of the nuclear power plant. The brief calculation presented here shows that unconventional uranium from phosphate rock production in the Kingdom of Saudi Arabia could have provided between 447–596 t uranium (12%–16% of the projected future natural uranium requirements of the Kingdom of Saudi Arabia) in 2021. An additional 6.46 t uranium (0.2% of future natural uranium requirements) was associated with seawater used for desalination in Saudi Arabia in 2021. Domestic phosphate rock processing and water desalination are both expected to increase so that the amount of unconventional uranium that can theoretically be recovered with technologies being available today will also increase. Recovering uranium from phosphate rocks and desalination concentrate reduces the environmental risks associated with potential dissipation of this radiotoxic heavy metal and the raw material could simply be stored after extraction for future use. It is therefore suggested to seriously consider uranium recovery in the Saudi phosphate and desalination industry.
Data availability statement
The original contributions presented in the study are included in the article/supplementary material, further inquiries can be directed to the corresponding author.
Author contributions
SU-D: Writing–review and editing. AA: Writing–review and editing. RK: Writing–review and editing. NH: Writing–original draft.
Funding
The author(s) declare that financial support was received for the research, authorship, and/or publication of this article. This work received support by the Institutional funds for research and innovation, Deanship of Scientific research, King Saud University through the project number IFKSUDR_E158.
Conflict of interest
The authors declare that the research was conducted in the absence of any commercial or financial relationships that could be construed as a potential conflict of interest.
Publisher’s note
All claims expressed in this article are solely those of the authors and do not necessarily represent those of their affiliated organizations, or those of the publisher, the editors and the reviewers. Any product that may be evaluated in this article, or claim that may be made by its manufacturer, is not guaranteed or endorsed by the publisher.
References
Al-Eshaikh, M. A., Kadachi, A. N., and Mansoor Sarfraz, M. (2016). Determination of uranium content in phosphate ores using different measurement techniques. J. King Saud Univ.-Eng. Sci. 28, 41–46. doi:10.1016/j.jksues.2013.09.007
Alruwaished, A., Alshehri, S., Mukrish, A., Shams, A., and Al-Athel, K. (2023). Nuclear fission energy initiatives in the kingdom of Saudi Arabia. Arab. J. Sci. Eng. doi:10.1007/s13369-023-08105-z
Al-Sahidi, M., and Haghirian, M. (2020). A quest for the Arabian atom? Geopolitics, security, and national identity in the nuclear energy programs in the Middle East. Energy Res. Soc. Sci. 69, 101582. doi:10.1016/j.erss.2020.101582
Altay, M. B., Kalıpçıoğlu, C., and Kurt, Z. (2022). Comparative life cycle assessment of uranium recovery from brine. Resour. Conservation Recycl. 181, 106237. doi:10.1016/j.resconrec.2022.106237
Alzahrani, S. M., Alwafi, A. M., and Alshehri, S. M. (2023). A framework of examining the factors affecting public acceptance of nuclear power plant: case study in Saudi Arabia. Nucl. Eng. Technol. 55 (3), 908–918. doi:10.1016/j.net.2022.11.009
ArabNews (2023). The rise and rise of water desalination in Saudi Arabia. Available at: https://www.arabnews.com/node/2160116/business-economy.
Bigalke, M., Ulrich, A., Rehmus, A., and Keller, A. (2017). Accumulation of cadmium and uranium in arable soils in Switzerland. Environ. Pollut. 221, 85–93. doi:10.1016/j.envpol.2016.11.035
Damoom, M. M., Hashim, S., Aljohani, M. S., Aziz Saleh, M., and Xoubi, N. (2019). Potential areas for nuclear power plants siting in Saudi Arabia: GIS-based multi-criteria decision making analysis. Prog. Nucl. Energy 110, 110–120. doi:10.1016/j.pnucene.2018.09.018
Diwa, R. R., Ramirez, J. D., and Haneklaus, N. H. (2023). Uranium supply potential from imported phosphate rocks for the Philippine nuclear power program. Extr. Industries Soc. 15, 101303. doi:10.1016/j.exis.2023.101303
Gabriel, S., Baschwitz, A., Mathonnière, G., Fizaine, F., and Eleouet, T. (2013). Building future nuclear power fleets: the available uranium resources constraint. Resour. Policy 38, 458–469. doi:10.1016/j.resourpol.2013.06.008
Hakkar, M., Arhouni, F. E., Mahrou, A., Bilal, E., Bertau, M., Roy, A., et al. (2021). Enhancing rare earth element transfer from phosphate rock to phosphoric acid using an inexpensive fly ash additive. Miner. Eng. 172, 107166. doi:10.1016/j.mineng.2021.107166
Haneklaus, N. (2021). Unconventional uranium resources from phosphates. Encycl. Nucl. Energy 76, 286–291. doi:10.1016/B978-0-12-819725-7.00152-5
Haneklaus, N., Bayok, A., and Fedchenko, V. (2017a). Phosphate rocks and nuclear proliferation. Sci. Glob. Secur. 25, 143–158. doi:10.1080/08929882.2017.1394061
Haneklaus, N., Sun, Y., Bol, R., Lottermoser, B., and Schnug, E. (2017b). To extract, or not to extract uranium from phosphate rock, that is the question. Environ. Sci. Technol. 51, 753–754. doi:10.1021/acs.est.6b05506
Haneklaus, N. H., Mwalongo, D. A., Lisuma, J. B., Amasi, A. I., Mwimanzi, J., Bituh, T., et al. (2024). Rare earth elements and uranium in Minjingu phosphate fertilizer products: plant food for thought. Resour. Conservation Recycl. 107694, 107694. doi:10.1016/j.resconrec.2024.107694
Hill, R. C., Williams, G. D. Z., Wang, Z., Hu, J., El-Hasan, T., Duckworth, O. W., et al. (2024). Tracing the environmental effects of mineral fertilizer application with trace elements and strontium isotope variations. Environ. Sci. Technol. Lett. 11, 604–610. doi:10.1021/acs.estlett.4c00170
Khater, A. E. M., Galmed, M. A., Nasr, M. M., and El-Taher, A. (2016). Uranium and rare earth elements in Hazm El-Jalamid phosphate, Saudi Arabia: concentrations and geochemical patterns comparison. Environ. Earth Sci. 75, 1261. doi:10.1007/s12665-016-6063-x
Kim, H., Eggert, R. G., Carlsen, B. W., and Dixon, B. W. (2016). Potential uranium supply from phosphoric acid: a U.S. analysis comparing solvent extraction and Ion exchange recovery. Resour. Policy 49, 222–231. doi:10.1016/j.resourpol.2016.06.004
KSA vision 2030 (2023). KSA vision 2030. Available at: https://www.vision2030.gov.sa/en/.
Loganathan, P., Naidu, G., and Vigneswaran, S. (2017). Mining valuable minerals from seawater: a critical review. Environ. Sci. Water Res. Technol. 3, 37–53. doi:10.1039/C6EW00268D
López, L., Castro, L. N., Scasso, R. A., Grancea, L., Tulsidas, H., and Haneklaus, N. (2019). Uranium supply potential from phosphate rocks for Argentina's nuclear power fleet. Resour. Policy 62, 397–404. doi:10.1016/j.resourpol.2019.04.008
Mehboob, K., Alzahrani, Y. A., Fallatah, O., Qutub, M. M. T., and Younis, H. (2021). Radioactivity and radiation hazard indices assessment for phosphate rock samples from Al-jalamid, turaif, umm Wu’al, and as-sanam, Saudi Arabia. Arabian J. Sci. Eng. 46, 779–792. doi:10.1007/s13369-020-04929-1
Mwalongo, D. A., Haneklaus, N. H., Lisuma, J. B., Mpumi, N., Amasi, A. I., Mwimanzi, J. M., et al. (2024). Uranium dissemination with phosphate fertilizers globally: a systematic review with focus on east africa. Sustainability 16 (4), 1496. doi:10.3390/su16041496
NEA/IAEA (2022). Uranium 2020: resources, production and demand. Paris: OECD Publishing. doi:10.1787/2c4e111b-en
NuclearPower (2023). Natural uranium consumption in a nuclear reactor. Available at: https://www.nuclear-power.com/nuclear-power-plant/nuclear-fuel/fuel-consumption-of-conventional-reactor/natural-uranium-consumption/.
Qamouche, K., Chetaine, A., El Yahyaoui, A., Moussaif, A., Fröhlich, P., Bertau, M., et al. (2021). Uranium and other heavy metal sorption from Moroccan phosphoric acid with argan nutshell sawdust. Miner. Eng. 171, 107085. doi:10.1016/j.mineng.2021.107085
Shang, D., Geissler, B., Mew, M., Satalkina, L., Zenk, L., Tulsidas, H., et al. (2021). Unconventional uranium in China’s phosphate rock: review and outlook. Renew. Sustain. Energy Rev. 140, 110740. doi:10.1016/j.rser.2021.110740
Sodaye, H., Nisan, S., Poletiko, C., Prabhakar, S., and Tewari, P. K. (2009). Extraction of uranium from the concentrated brine rejected by integrated nuclear desalination plants. Desalination 235, 9–32. doi:10.1016/j.desal.2008.02.005
Steiner, G., Geissler, B., and Haneklaus, N. (2020). Making uranium recovery from phosphates great again? Environ. Sci. Technol. 54, 1287–1289. doi:10.1021/acs.est.9b07859
Tulsidas, H., Gabriel, S., Kiegiel, K., and Haneklaus, N. (2019). Uranium resources in EU phosphate rock imports. Resour. Policy 61, 151–156. doi:10.1016/j.resourpol.2019.02.012
Ulrich, A. E., Schnug, E., Prasser, H.-M., and Frossard, E. (2014). Uranium endowments in phosphate rock. Sci. Total Environ. 478, 226–234. doi:10.1016/j.scitotenv.2014.01.069
USGS (2023). Phosphate rock statistics and information. Available at: https://www.usgs.gov/centers/national-minerals-information-center/phosphate-rock-statistics-and-information.
Van Kauwenbergh, S. (1997). Cadmium and other minor elements in world resources of phosphate rock. Fertilizer Soc. Proc. 400.
WNA (2023a). Nuclear power in Saudi Arabia. Available at: https://world-nuclear.org/information-library/country-profiles/countries-o-s/saudi-arabia.aspx.
WNA (2023b). Supply of uranium. Available at: https://world-nuclear.org/information-library/nuclear-fuel-cycle/uranium-resources/supply-of-uranium.aspx.
WNA (2023c). World uranium mining production. Available at: https://world-nuclear.org/information-library/nuclear-fuel-cycle/mining-of-uranium/world-uranium-mining-production.aspx.
Wu, S., Wang, L., Zhao, L., Zhang, P., El-Shall, H., Moudgil, B., et al. (2017). Recovery of rare earth elements from phosphate rock by hydrometallurgical processes – a critical review. Chem. Eng. J. 335 (1), 774–800. doi:10.1016/j.cej.2017.10.143
Keywords: desalination, phosphoric acid, Saudi Arabia, uranium recovery, available technology
Citation: Ud-Din Khan S, Ahmad A, Khan R and Haneklaus N (2024) Uranium resources associated with phosphoric acid production and water desalination in Saudi Arabia. Front. Earth Sci. 12:1341059. doi: 10.3389/feart.2024.1341059
Received: 19 November 2023; Accepted: 01 July 2024;
Published: 26 July 2024.
Edited by:
Sitaraman Krishnan, Clarkson University, United StatesReviewed by:
Luis Eduardo Lopez, National Atomic Energy Commission, ArgentinaDanish, Guangdong University of Foreign Studies, China
Copyright © 2024 Ud-Din Khan, Ahmad, Khan and Haneklaus. This is an open-access article distributed under the terms of the Creative Commons Attribution License (CC BY). The use, distribution or reproduction in other forums is permitted, provided the original author(s) and the copyright owner(s) are credited and that the original publication in this journal is cited, in accordance with accepted academic practice. No use, distribution or reproduction is permitted which does not comply with these terms.
*Correspondence: Salah Ud-Din Khan, ZHJza2hhbkBrc3UuZWR1LnNh