- 1DiSTeM, Università di Palermo, Palermo, Italy
- 2Department of Lithospheric Research, University of Vienna, Vienna, Austria
- 3Dipartimento di Fisica e Scienze della Terra, Università di Ferrara, Ferrara, Italy
- 4Dipartimento di Scienze dell’Ambiente e della Terra, Università di Milano Bicocca, Milano, Italy
- 5Instituto Volcanológico de Canarias (INVOLCAN), Tenerife, Canary Islands, Spain
- 6Instituto Tecnológico y de Energías Renovables (ITER), Tenerife, Canary Islands, Spain
- 7Istituto Nazionale di Geofisica e Vulcanologia, Sezione di Milano, Milano, Italy
The 2021 Tajogaite eruption at La Palma has represented a unique opportunity to investigate the characteristics of the mantle source feeding modern volcanism in the Canary Islands. With the aim of track the fingerprint of carbon in the local oceanic lithosphere-asthenosphere system, we report the isotopic composition of CO2 (δ13C values versus Vienna Pee Dee Belemnite) in olivine- and clinopyroxene-hosted fluid inclusions (FI) from the 2021 Tajogaite lavas and from lavas/ultramafic xenoliths (olivine-clinopyroxenites, clinopyroxenites, dunites and harzburgites) from the nearby 1677 San Antonio eruption cone/lavas, in an attempt to characterize the origin and evolution of carbon within the local mantle source. Our results indicate that the 2021 and 1677 lavas exhibit δ13C values ranging from −4.94‰ to −2.71‰ and CO2/3He ratios from 3.37 to 6.14 × 109. Ultramafic xenoliths fall in a comparable range of values despite showing higher CO2 concentrations. Our δ13C values fall within the range of carbon isotope results previously reported for the Dos Aguas cold spring located in the Taburiente Caldera (northern La Palma), suggesting an apparent carbon isotope homogeneity at the scale of the entire island. The (relatively narrow) δ13C vs. CO2/3He ratio range of La Palma samples is interpreted to reflect either i) variable extents of open-system degassing of a common mantle endmember having δ13C of ∼1.7‰, or ii) mixing between depleted mantle-like carbon (−6‰ < δ13C < −4‰) and crustal carbon (δ13C = 0‰) endmembers. Both models testify a crustal carbon component recycled in the local mantle. This component, also detected in mantle xenoliths from the neighboring island of El Hierro and the easternmost Lanzarote, indicates a regional characteristic of the mantle beneath the Canary Islands, interpreted as a result of infiltration of carbon-rich melts during past metasomatic events in the local mantle.
1 Introduction
Volcanic eruptions open avenues to explore the composition and geodynamic evolution of the Earth’s upper mantle. The eruptions of Ocean Island Basalt (OIB) volcanoes (such as in the Canary Islands, Samoa, Cook Islands, Azores, and Pitcairn; Anderson, 1912; Machado et al., 1962; Duncan et al., 1974; Rose and Koppers, 2019; Carracedo et al., 2022; exceptions are Hawaii, Iceland and the Piton de la Fournaise volcano at Reunion Islands), are especially attractive owing to their prevalent effusive nature and hence relatively easy access to eruption sites, relative to arc volcanoes which are characterized by more explosive activity. Moreover, OIB eruptions promote the investigation of deeper portions of the Earth’s mantle when compared with Middle Oceanic Ridge Basalts, MORB (Herzberg et al., 2007; Hofmann, 2007; Dasgupta et al., 2010; Jackson, 2016), and eventually favors the exploration of the dynamics of mantle plumes (Dasgupta et al., 2010; Jackson, 2016).
The Canary Islands are among the most studied OIB localities. This is partly due to the relatively densely inhabited environment (and, hence, the considerable volcanic risk), and also the scientific controversies and debate within the Earth science community regarding the origin of volcanic activity in the archipelago, and the characteristics of the feeding mantle source (Carracedo et al., 1998; Anguita and Hernán, 2000; Troll and Carracedo, 2016). One of the biggest open questions regarding the origin of volcanism in the Canary Islands is how the local mantle source has evolved after the breakup of Pangea (Hoernle et al., 1995; Carracedo et al., 1998; Anguita and Hernán, 2000; Gurenko et al., 2009; Troll and Carracedo, 2016). It has been suggested that the mantle underneath the Canary Islands is compositionally heterogeneous due to the coexistence of different mantle components. The mantle end-members that have been proposed (Hoernle et al., 1991; Hoernle and Schmincke, 1993; Simonsen et al., 2000; Gurenko et al., 2006; 2009; 2010; Day et al., 2010; Day and Hilton, 2011; Sandoval-Velasquez et al., 2021b; Day and Hilton, 2021; Sandoval Velasquez et al., 2022a) to contribute (in variable proportions) to melting and volcanism include: i) an HIMU (high-μ = elevated 238U/204Pb) mantle component; ii) a Depleted MORB mantle (DMM), where MORB stands for Middle Ocean Ridge Basalt; iii) an enriched mantle (EM) component; and iv) a high 3He/4He ratio (> 9Ra) mantle component (represented at La Palma), whose origin would be intimately connected with both old and recent geodynamic processes (e.g., paleo-subduction events, the opening of the Atlantic Ocean, and the influence of a mantle plume).
According to the historical record, a minimum of 13 eruptions in the last 500 years have occurred in the Canary Islands (Troll and Carracedo, 2016; Longpré, 2021). Most of the recent eruptions have been registered in La Palma, one of the youngest islands of the archipelago (<1.7 Ma; Guillou et al., 1998; Guillou et al., 2001; Carracedo et al., 2001; Klügel et al., 2017). On 19 September 2021, after 50 years of quiescence, the largest historical eruption of La Palma started with the formation of a fissure on the Cumbre Vieja ridge (southwestern flank of the island), a ∼18 km long rift-like volcanic structure where the recent volcanism has concentrated since 150 ka (Guillou et al., 1998; Carracedo et al., 2001; Troll and Carracedo, 2016; Klügel et al., 2017). The fissural phase of the eruption rapidly evolved into a central eruption at the Tajogaite cone (Carracedo et al., 2022; Pankhurst et al., 2022; del Fresno et al., 2023) that released approximately 200 million m3 of volcanic material (pyroclasts+lava, DRE; Carracedo et al., 2022) over almost 3 months of activity (the eruption ended on 13 December 2021). The petrological analysis revealed that the erupting magma was a high-temperature and low-viscosity primitive-basanitic melt derived from ∼3% partial melting of a fertile asthenospheric mantle (Day et al., 2022; Pankhurst et al., 2022). According to Day et al. (2022), the mantle source that fed the 2021 eruption is a homogenous reservoir containing a pyroxenitic component inherited from the reaction between the peridotite and recycled crustal components, eventually determining the oxidized and HIMU-like signature of La Palma lavas.
Regarding the origin of La Palma volcanism, carbon isotope data can provide essential insights into the compositional properties of the mantle source, especially in order to test if the mantle has been modified during its history by any metasomatic agent transporting recycled carbon-rich crustal materials, and to what extent. This is because metasomatic melts/fluids in OIB context are systematically carbon-rich (e.g., Barry et al., 2014; Boudoire et al., 2018), and because carbon isotopes are powerful tracers of recycled crustal components in the mantle (Pineau and Mathez, 1990; Sano and Marty, 1995; Gennaro et al., 2017; Sandoval-Velasquez et al., 2021a; Sandoval-Velasquez et al., 2021b; Sandoval-Velasquez et al., 2022c). Indeed, the observations made by Day et al. (2022) are consistent with recent carbon isotopic studies in FI trapped in mantle xenoliths from the neighboring island of El Hierro, whose positive δ13C signatures (from −2.38‰ to +0.96‰) relative to the upper mantle range (from −8‰ to −4‰; Sano and Marty, 1995) suggest mantle metasomatism caused by the infiltration of carbonated silicate melts, perhaps inherited from recycled crustal materials (Frezzotti et al., 2002a; Frezzotti et al., 2002b; Sandoval-Velasquez et al., 2021b). It has been also argued that such a recycled crustal carbon component may be a regional characteristic of the local mantle (as the HIMU endmember; Sandoval-Velasquez et al., 2022a), but the limited carbon isotope information existing for other islands (such as La Palma, Tenerife, Gran Canaria, La Gomera or Lanzarote) has so far impeded testing this hypothesis.
In this paper, we extend our knowledge of the carbon isotopic signature of the mantle source feeding the magmatism at La Palma, by presenting: the first carbon isotope data on FI entrapped in olivine and pyroxenes phenocrysts from the 2021 Tajogaite lavas and from the 1677 San Antonio lavas, as well as from representative ultramafic xenoliths (olivine-clinopyroxenites, clinopyroxenites, dunites and harzburgites) brought to the surface during the San Antonio eruption (see Figure 1). Our aim is to test our hypothesis about regional crustal carbon recycling in the mantle beneath the Canary Islands. We also take this opportunity to present new FI data from peridotite xenoliths collected from Lanzarote, considered as representative of the local lithospheric mantle (Siena et al., 1991). Our results contribute to deepening the understanding of the mantle source feeding recent volcanism at La Palma and contribute to the debate regarding the geodynamics of the Canary Islands.
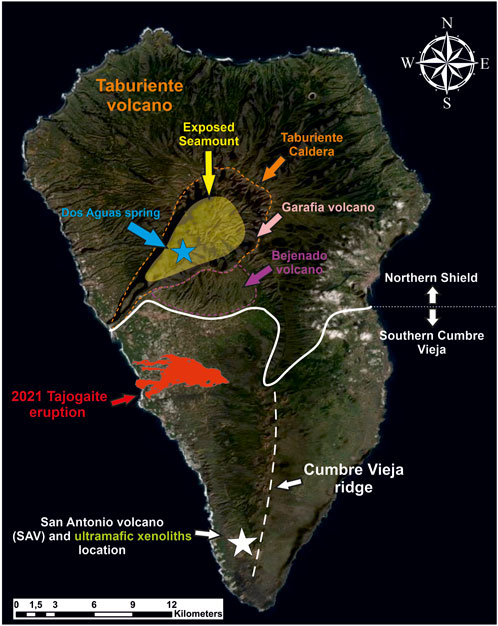
FIGURE 1. Map of La Palma Island and location of the study areas. Seamount, Taburiente, Bejenado and Northern shield contours were designed after Troll and Carracedo (2016). 2021 Tajogaite lava field was designed using the topographical information published by COPERNICUS (https://emergency.copernicus.eu/mapping/list-of-components/EMSR546).
2 Volcanological evolution of La Palma
La Palma is located in the western part of the archipelago and is one of the youngest and most active islands of the Canary archipelago (Troll and Carracedo, 2016; Klügel et al., 2017). The oldest rocks found at La Palma are seamounts dated ca. 2–4 Ma ago that represent the submarine construction of the island (Figure 1; Troll and Carracedo, 2016; Klügel et al., 2017). The island presumably emerged 1.8–1.2 Ma ago, as recorded by an angular unconformity identified between the seamount edifice and the first volcanic deposits of the northern shield (Guillou et al., 1998; Guillou et al., 2001; Klügel et al., 2017). Today, the island consists of two major volcanic complexes: the northern shield (last eruption > 560 ka), which comprises the emerged seamounts, the Garafia, Cumbre Nueva, Taburiente and Bejenado volcanoes; and the southern Cumbre Vieja ridge, where recent (< 150 ka) volcanism has concentrated (Guillou et al., 1998; Guillou et al., 2001; Troll and Carracedo, 2016; Klügel et al., 2017) (Figure 1). Before 2021, the Cumbre Vieja had experienced 7 eruptions in the last 500 years, the most remembered being those that built the San Antonio, San Juan and Teneguía cones in 1677, 1949 and 1971, respectively (Carracedo et al., 2001; Troll and Carracedo, 2016). Erupted rocks at La Palma comprise basanites, alkali basalts, tephrites and phonolites with chemical signatures typical of OIB settings (Day et al., 2010; Day and Hilton, 2011; Klügel et al., 2017).
3 Samples and methods
The carbon concentration and isotope data presented here stand on the results of initial FI screening and noble gas investigations (Sandoval Velasquez et al., 2022b; Sandoval-Velasquez et al., 2023). Based on these results, we selected the aliquots with the highest concentrations (> 1.0 × 10−7 mol/g) of CO2 (n = 14) to determine the carbon isotopic composition of FI trapped in Tajogaite and San Antonio rocks. The isotopic determinations were conducted in the stable isotopes laboratory of INGV, Sezione di Palermo (Italy). The 2021 Tajogaite lavas were sampled between September 29th and 9 November 2021, with lava eruption dates recorded in the field by INVOLCAN staff and cross-checking with near-real-time satellite information reported by Copernicus (https://emergency.copernicus.eu/mapping/list-of-components/EMSR546). For this work, 4 lava samples were selected. We added to this set one lava sample and 9 xenoliths (3 pyroxenites, and 6 peridotite xenoliths) from the historical San Antonio volcano (Figure 1). Detailed information about sampling, petrology, FI composition and noble gas-Sr-Nd-W systematics of the samples are provided in a companion article (Sandoval-Velasquez et al., 2023). Finally, 4 representative peridotite xenoliths (spinel harzburgites) from Lanzarote, already characterized in terms of petrography and mineral chemistry (Siena et al., 1991), were also integrated in this study to determine the concentration and isotopic signature of C in olivine- and clinopyroxene-hosted FI. These samples were collected from Quaternary alkali basic lavas belonging to the cycles III and IV proposed by Fuster et al. (1968); the most recent volcanic deposits of the island.
After crushing, olivines and pyroxenes were hand-picked using a binocular microscope; aliquots of 0.1–3 g of crystals were thoroughly cleaned in an ultrasonic bath in 6.5% HNO3 and HCl to remove possible carbonates attached to the crystal lattices, deionized water and high purity-acetone. Then, samples were precisely weighed and loaded into a crusher system consisting of a stainless-steel sample holder, a hydraulic crusher, and a glass sampler to trap and freeze CO2. A Pfeiffer Hi-Cube turbo molecular pump was used to ensure the vacuum. The gas mixture trapped as FI (mainly CO2) was released after crushing the crystals using a piston at a pressure of about 200 bar. The gas mixture was then purified in a Pyrex glass line composed of a 626B Baratron® Absolute Capacitance Manometer MKS (measuring range 10−3–10 mbar) and two traps to quantify and cryogenically purify the gas mixture, respectively. The glass line was also connected to a pump to ensure low vacuum. After purification, the CO2 was trapped in a glass sampler (adjusted to atmospheric pressure by adding pure helium). The sampler was then transferred to a Thermo (Finnigan) Delta Plus XP CF-IRMS [connected to a Trace GC gas chromatograph and a Thermo (Finnigan) GC/C III interface] to estimate the 13C/12C ratios. The analytical error (1σ) is ∼0.3‰. As reported in Table 1, values are expressed in parts per mil (‰) relative to the Vienna Pee Dee Belemnite international standard using the delta notation (δ13C). Further information about analytical procedures can be found in Gennaro et al. (2017).
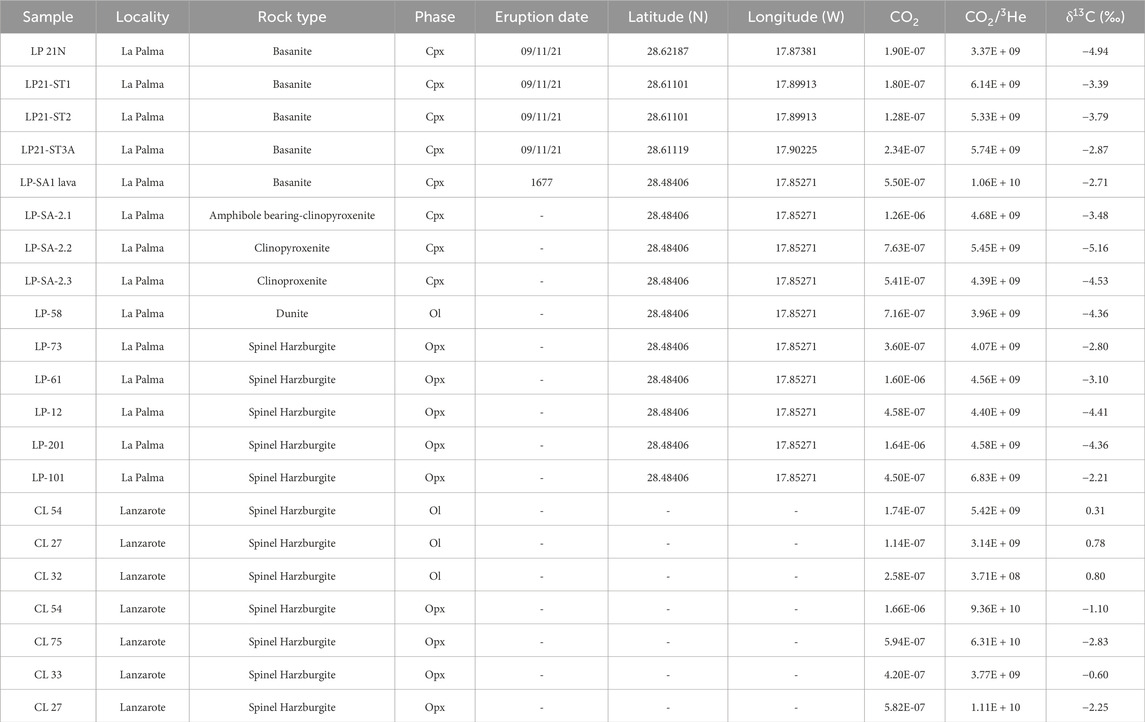
TABLE 1. CO2 concentrations and isotopic ratios. List of the lavas and ultramafic xenoliths from La Palma and Lanzarote. CO2/3He values were estimated using the information presented in Sandoval-Velasquez (2022), Sandoval-Velasquez et al. (2022a), Sandoval-Velasquez et al. (2022b), Sandoval-Velasquez et al. (2023).
4 Results
A petrographic description of the studied La Palma samples is provided as Supplementary Material. Regarding FI composition, in the 2021 Tajogaite lavas, the concentration of CO2 varies in a narrow range from 1.28 × 10−7 to 2.34 × 10−7 mol/g, a value lower than that registered in the 1677 San Antonio lava FI (5.50 × 10−7 mol/g) (Table 1). The highest CO2 concentrations are observed in the San Antonio pyroxenite and peridotite xenoliths, with values up to 1.64 × 10−6 mol/g (Figure 2). All La Palma samples show CO2/3He ratios between 3.37 × 109 and 1.06 × 1010 that broadly correlate with increasingly positive δ13C compositions (Figure 3). Most of the determined δ13C values (from −5.16 to −2.21‰) are heavier than the MORB range (from −8 to −4‰; Sano and Marty, 1995) except for samples LP-21N (Opx), LP-58 (Ol), LP-12 (Opx), LP-202 (Opx), LP-SA-2.2 (Px) and LP-SA-2.3 (Px), which show an upper mantle-like signature (Figure 2). The C isotope compositions of lavas, pyroxenite, and peridotite xenoliths from La Palma are essentially overlapping (Figures 2, 3).
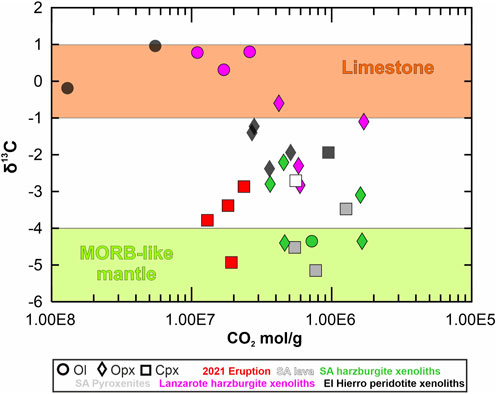
FIGURE 2. δ13C ratio vs. CO2 concentration (mol/g) of mineral-hosted FI in the studied samples from La Palma, which include the lava flow from the recent 2021 Tajogaite eruption, the 1677 lava flow from San Antonio (SA), as well as pyroxenitic and peridotitic xenoliths from San Antonio. The MORB-like mantle and crustal limestone ranges at δ13C = −4 to −7‰ and at δ13C = −1 to −1‰, respectively, are also reported for comparison after Sano and Marty (1995). El Hierro data is from Sandoval-Velasquez et al. (2021b).
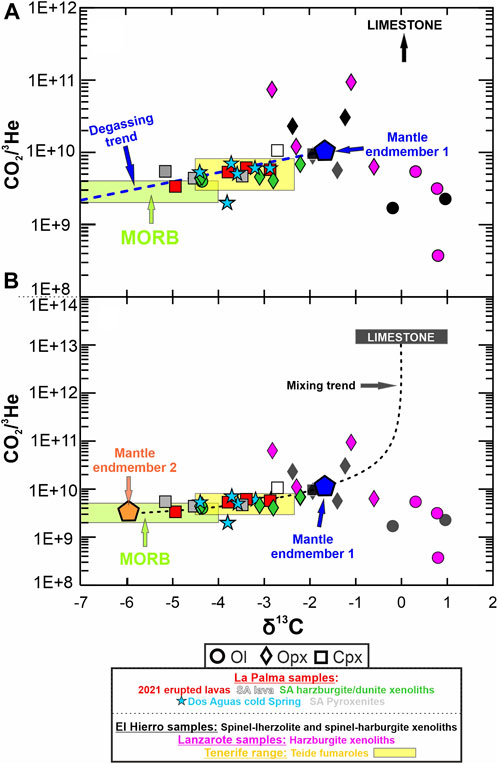
FIGURE 3. δ13C vs. CO2/3He ratios of the studied samples from La Palma compared to literature data from the entire Canary archipelago. In (A,B), two alternative models to explain the origin of the carbon isotopic signature of the Canary Islands mantle source are proposed. (A) The blue polygon represents the proposed mantle endmember for La Palma in case of an open-degassing model. The mantle endmember was fixed at δ13C = 1.7‰ and CO2/3He = 8.10 × 109. The open-system degassing trend was estimated after Hoefs (2015); a Δ melt-vapor distribution coefficient of 2.5 was considered. (B) A binary mixing model between two different endmembers: 1) a mantle endmember (orange polygon) at δ13C = −6‰ and a CO2/3He = 3 × 109 and a limestone endmember at δ13C = 0‰ and a CO2/3He = 1 × 1013. δ13C data from Dos Aguas Spring, Tenerife and El Hierro were taken from the literature (Melián et al., 2012; Padrón et al., 2015; Torres-González et al., 2020; Sandoval-Velasquez et al., 2021a; Day and Hilton, 2021; Padrón et al., 2022). MORB and Limestone ranges were designed after Sano and Marty (1995). SA, San Antonio.
In Lanzarote samples, CO2 is also the predominant phase in FI [on average 5.09x10−7 mol/g (n = 8)]; pyroxenes exhibit the highest concentrations compared with olivine (Figure 2). Unlike La Palma peridotites, the isotopic composition of CO2 (δ13C) reveals more positive values than the MORB range. Two groups of data were identified: pyroxenes with values that vary between −2.83 and −0.60‰ and olivines from +0.31 to +0.80‰.
5 Discussion
Sandoval Velasquez et al. (2022b), Sandoval-Velasquez et al. (2023) previously reported on the noble gas (He-Ar) signature of the same FI studied here, showing that Tajogaite lavas exhibit a uniform, temporally invariant MORB-like 3He/4He composition (7.38 ± 0.22 Ra) during the eruption, consistent with the He isotopic signature of San Antonio lavas. However, ultramafic xenoliths from San Antonio, which represent the local oceanic lithospheric mantle composition, are characterized by lower (< 7 Ra) 3He/4He compositions, suggesting the presence a more radiogenic component in the Cumbre Vieja local lithospheric mantle. Based on this information, Sandoval-Velasquez et al. (2023) proposed that the Cumbre Vieja magmatism is fed by a source homogeneous for He isotopes, whose uniform isotopic signature (7.37 ± 0.17 Ra) resulted from the prolonged and efficient mixing of three distinct mantle fluid components: a depleted mantle (MORB-like: 8 ± 1 Ra) He source, a radiogenic (< 7 Ra) He component, and a high 3He/4He component (Ra > 9; the latter contributing for only 6%–15%).
5.1 The δ13C signature of the southern Cumbre Vieja ridge and the northern shield
The mineral-hosted FI in the 2021 Tajogaite lavas exhibit very homogeneous CO2/3He (between 3.37 × 109 and 6.14 × 109) and δ13C values (between −4.94‰ and −3.39‰) (Figure 3). Most of the FI δ13C values plot above the upper mantle range (−8‰ to −4‰; Sano and Marty, 1995) (Figure 2), similarly to the San Antonio lava that shows the heaviest δ13C composition (−2.71‰) among the magmatic samples (Figures 2, 3). The FI in lavas and ultramafic xenoliths exhibit overlapping δ13C signatures (Figure 2), strongly suggesting that the heavy FI carbon isotope signature of the lavas is source-inherited and not affected by crustal contamination processes. In fact, petrographic observations showed that San Antonio peridotites trapped secondary FI whose origin would be related to mantle metasomatism (see Supplementary Material); in all peridotites (clinopyroxenites, harzburgites and dunite) FI trails typically originate from glass microveins permeating the rocks or are intimately associated with reaction rims (composed of secondary olivine, clinopyroxene and glass) around primary orthopyroxenes. When considering the consistency of δ13C values in both lavas (basanites; Sandoval-Velasquez et al., 2023) and xenoliths, and the composition of the interstitial glass found in San Antonio peridotites, the above-mentioned metasomatism seems to be associated with the infiltration of the lithospheric mantle by basanitic/trachitic melts (see Sandoval-Velasquez et al., 2023), the latter probably generated in a deep mantle region (asthenosphere) similar to that feeding the recent volcanism of La Palma.
Prior to our study, the only available δ13C information for the Cumbre Vieja Ridge refers to dissolved CO2 in groundwater samples from a small well, periodically sampled during the October 2017 to February 2018 seismic-deformation unrest (Torres-González et al., 2020). This unrest has more recently been interpreted as an early sign of volcanic activity resumption that ultimately culminated in the 2021 eruption (Carracedo et al., 2022; Padrón et al., 2022; Pankhurst et al., 2022). As reported by Torres-González et al. (2020), the highest δ13C value measured during the unrest was −7.1‰, while the lowest was −10.3‰, which is well below the δ13C values reported here for the Tajogaite/San Antonio FI in our study. According to Torres-González et al. (2020), the δ13C in La Palma groundwaters could plausibly have fractionated due to biological processes. Moreover, it should be considered that δ13C of dissolved CO2 and TDIC (Total Dissolved Inorganic Carbon) in thermal fluids often fractionates by multi-step CO2 dissolution and carbonate precipitation/dissolution processes (Simmons and Christenson, 1994; Barry et al., 2014; Güleç and Hilton, 2016; Venturi et al., 2017). Such a fractionation may explain the discrepancy between the δ13C in groundwaters and the δ13C in FI data.
In the Northern Shield, δ13C values are available for the Dos Aguas spring, a CO2-rich bubbling cold spring located in the Taburiente Caldera (Figure 1). Before the 2021 Tajogaite eruption, the Dos Aguas spring was the only visible gas emission on La Palma (Pérez et al., 1994; Hilton et al., 2000; Padrón et al., 2012; Padrón et al., 2015; Torres-González et al., 2020; Day and Hilton, 2021; Padrón et al., 2022). This site is also especially important because it is the only locality where a high 3He/4He contribution, higher than the MORB-like range (8 ± 1 Ra) (Graham, 2002), has been detected in the Canary Islands. At the Dos Aguas spring, relatively constant high 3He/4He ratios (up to 10.24 Ra, where Ra is the atmospheric 3He/4He) have been reported through the last decades by different authors (i.e., Pérez et al., 1994; Padrón et al., 2012; Padrón et al., 2015; Padrón et al., 2022; Torres-González et al., 2020; Day and Hilton, 2021). The carbon isotopic composition of the Dos Aguas spring (measured during the last 30 years) has shown homogeneous δ13C values between −3.6‰ and −2.9‰ and upper mantle-like CO2/3He ratios, matching the range of the Tajogaite and San Antonio samples (Figures 3A, B) and providing further evidence of the existence of a heavy C isotopic reservoir beneath La Palma.
The fact that variable sample types (spring gases and mineral-hosted FI) from three localities >10 km apart from each other (Dos Aguas, Tajogaite and San Antonio) exhibit similar δ13C compositions is relevant and suggests their feeding from a common, relatively homogeneous deep source. As mentioned above, the low δ13C values reported from the Cumbre Vieja groundwaters are likely an underestimation of deep sources due to shallow depth fractionation processes; therefore these data will not be discussed further.
5.2 Inferences about the δ13C signature of the Canary Islands
The overall similarity of δ13C values in lavas and mantle xenoliths from La Palma (Figures 2, 3) suggests that the heavy C isotope signature recorded by the lavas comes from a13C-enriched mantle source. Recent studies in FI trapped in mantle xenoliths have proved that the local upper mantle beneath the Canary Islands has been intensely metasomatized by silicate and carbonate-rich melts (Admunsen, 1987; Neumann, 1991; 2004; Neumann and Wulff-Pedersen, 1997; Frezzotti et al., 2002a; Frezzotti et al., 2002b; Oglialoro et al., 2017). Admunsen (1987) and Neumann and Wulff-Pedersen (1997) first observed the presence of interstitial silicate glasses in mantle xenoliths from La Palma, El Hierro, Tenerife and Lanzarote. These authors suggested that interstitial glasses reflect metasomatic reactions between silicate melts and the surrounding peridotites at mantle conditions. Subsequently, Frezzotti et al (2002a), Frezzotti et al. (2002b) recognized the presence of carbonates (Mg-calcite and dolomite) inside FI trapped in ultramafic xenoliths from Tenerife and La Gomera, confirming the infiltration of volatile-rich carbonate melts in the western portion of the local upper mantle. Similarly, petrological studies performed in mantle xenoliths from El Hierro, which together with La Palma define the westernmost and most youthful extent of the archipelago indicated the formation of interstitial microveins composed of carbonate droplets (calcite and Mg-calcite) inherited from deep infiltration of volatile-rich, carbonate-silicate melts (Oglialoro et al., 2017; Remigi et al., 2019; Colombo, 2020).
Accordingly, Sandoval-Velasquez et al. (2021b) reported heavy δ13C (> −2.38‰) CO2 isotopic compositions in FI from the same suite of mantle xenoliths from El Hierro. These data proved the crustal affinity of the fluids that have interacted with the local mantle. They likely are derived from either recycled sediments, altered oceanic crust, or oceanic lithosphere materials.
Our results show that CO2 contents in FI in Lanzarote peridotite xenoliths are within the same range of El Hierro measurements (Figure 2), which indicates that the fluids were trapped under mantle conditions and supports the existence of a comparable C-rich fluid pervading the mantle beneath the eastern islands. This is corroborated by the variability of δ13C values (−2.83‰ < δ13C < +0.80‰), which fall in the same range of El Hierro mantle xenoliths displaying also a similar systematic distribution between olivine and pyroxene crystals (Figures 3A, B). The analogous δ13C signature of FI in mantle xenoliths from both Lanzarote (easternmost island) and El Hierro (westernmost island of the archipelago) would suggest a regional permeation of the mantle beneath the archipelago with C-rich fluids that exhibit a heavy isotope signature (δ13C > −3‰). As pyroxene is more sensitive than olivine to metasomatism in the Canary mantle, it can be used as a more trustable proxy for unveiling the composition of metasomatic fluids (Frezzotti and Touret, 2014); by averaging the composition of FI entrapped in pyroxenes from El Hierro and Lanzarote xenoliths we infer the averaged “regional” (Canary archipelago) source mantle characteristics at δ13C of −1.71‰ and CO2/3He of 8.10 × 109 (“Mantle endmember 1” in Figures 3A, B).
Our results for lavas, pyroxenites and mantle xenoliths from La Palma plot between this inferred Canary Mantle endmember 1 and the MORB range (Figures 3A, B). Interestingly, the Teide fumaroles on Tenerife Island exhibit similarly intermediate compositions (see yellow band in Figures 3A, B). Therefore, we envisage two distinct mechanisms/processes that can explain the positive δ13C vs CO2/3He tendency and (more broadly) the more 13C-depleted compositions at La Palma relative to El Hierro and Lanzarote.
In the first scenario (model A), we ascribe the δ13C and CO2/3He compositions of La Palma lavas, xenoliths and Teide fumaroles to degassing processes starting from a regionally homogeneous Canary mantle (corresponding to the Canary “Mantle endmember 1” above) (Figure 3A). Several authors have demonstrated that, upon increasing extents of degassing (e.g., during volatile exsolution into a separate fluid phase), the original δ13C composition of a system (a melt, or even the mantle) evolves toward progressively more negative (13C-depleted) compositions (Javoy et al., 1978; Mattey, 1991; Boudoire et al., 2018). This occurs because, during degassing, 13C is preferentially partitioned into a CO2-rich fluid phase relative to the coexisting melt of basaltic composition, leaving this latter (or a degassed, residual mantle reservoir) enriched in 12C instead (Javoy et al., 1978; Mattey, 1991; Porcelli et al., 1992; Deines et al., 2002; Aubaud et al., 2005; Aubaud, 2022).
To quantitatively test this hypothesis (Figure 3A), we model an open-system degassing process starting from the composition of the Mantle endmember 1 at δ13C = −1.71‰ and CO2/3He = 8.10 × 109 (which are the estimated averages for the pyroxenes from El Hierro and Lanzarote xenoliths), and using the mathematical expression of Hoefs (2015), Eq. 1:
where Rv/Rlo is the instantaneous isotope ratio of the vapor leaving the liquid and the remaining liquid, α is the fractionation factor and f is the fraction of the residual liquid. As observed in Figure 3A, all samples from La Palma and the Tenerife range fit the modeled degassing curve, indicating the likelihood of the process. These model calculations imply that a common 13C-enriched, homogeneous mantle source (enriched in a recycled crustal carbon component) may exist beneath the Canary Islands, with all the inter- and intra-island variability caused by degassing processes. If this hypothesis is true, then the fluids trapped in the La Palma ultramafic xenoliths would not have a primary origin, but would represent secondary FI formed after the interaction with the transporting melt (here represented by the SA lava: sample LP-SA1 lava). Petrographic observations on the mantle xenoliths seem to support the secondary origin of trapped fluids (see Supplementary Material). Thus, trapping melt-derived fluids would explain why ultramafic xenoliths fit the same degassing trend.
In the second scenario (model B; Figure 3B) the intermediate δ13C vs. CO2/3He compositions of La Palma FI can be interpreted as reflecting binary mixing between two distinct reservoirs, i.e., a crustal limestone component (at δ13C = 0‰ and CO2/3He = 1 × 1013, Sano and Marty, 1995) and a depleted (MORB-like) Canary “Mantle endmember 2” (at δ13C between −6‰ and −4‰ and CO2/3He = 3 × 109; the latter representing the lowest CO2/3He observed in our dataset). In this interpretation, the Canary mantle source would be regionally heterogeneous, and modelled by a depleted (MORB-like) mantle endmember that was variably refertilized and enriched in carbon via interaction with a recycled crustal carbon component (illustrated by a limestone at δ13C = 0‰ and CO2/3He = 1.0 × 1013). The Canary “Mantle endmember 2”-limestone mixing curve also explains the compositional variability of both FI and surface fluids. In this scenario, the maximum estimated contribution of the crustal limestone component at La Palma would be <10%.
Unfortunately, the currently available information makes it challenging to discriminate between the two models above (degassing vs mixing). We caution that additional δ13C data, coupled to barometric determinations (in both lavas and ultramafic xenoliths) and more detailed petrographic and FI observations are required to prove a robust relationship exists between the extent of magma ascent and depressurization, the secondary origin of FI in mantle xenoliths and increasingly negative δ13C signature. In addition to the evidences reported in support of scenario 1, we note that our FI δ13C results overlap with those obtained from the Dos Aguas Spring, a fact that more likely reflects a variability due to magma degassing. Alternatively, the variability of δ13C values in spring gases should reflect the degassing of melts having distinct carbon isotopic signatures, implying a local and spatially-narrow mantle heterogeneity, which seems unlikely considering that the same δ13C signature of Mantle endmember 1 was measured in mantle xenoliths from the easternmost island of Canary (i.e., Lanzarote). In any case, both models suggest the existence of a recycled crustal component in the mantle source beneath La Palma. As previously stated, this mantle component could also be present in the eastern portions of the archipelago (as suggested by δ13C of Lanzarote samples and the petrologic studies performed in mantle xenoliths from La Gomera and Tenerife; Admunsen, 1987; Neumann, 1991; Neumann, 2004; Neumann and Wulff-Pedersen, 1997; Frezzotti et al., 2002a; Frezzotti et al., 2002b; Sandoval-Velasquez et al., 2022b), supporting a regional pattern. Further studies of FI in ultramafic xenoliths from islands such as La Gomera, Tenerife, Gran Canaria and Fuerteventura, coupled with a detailed comprehension of the FI composition and a comprehensive geodynamic framework, will clarify the extent of the recycled crustal component.
The hypothesis that the recycled crustal carbon is a regional characteristic of the upper mantle beneath the Canary Islands agrees well with the classic “Blob model” (Hoernle and Schmincke, 1993) and the more recent “edge-driven mantle convection model” (Gurenko et al., 2010). These theories would explain how a crustal component is brought to the surface by a HIMU-like mantle plume (which spreads beneath the archipelago), being the most plausible scenario to explain the δ13C variability observed along the archipelago (Figure 4).
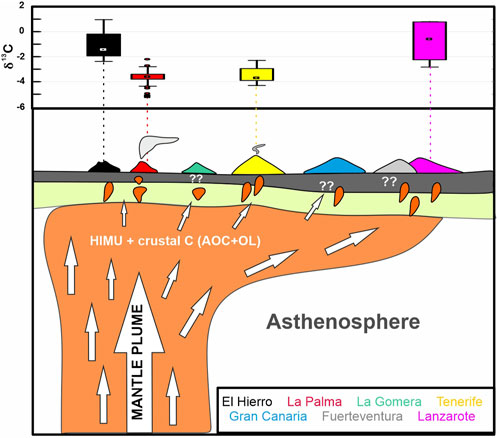
FIGURE 4. Diagram showing the origin of the crustal carbon identified in free gas and FI trapped in lavas and ultramafic xenoliths from La Palma, El Hierro, Tenerife and Lanzarote islands. δ13C boxplots were designed using the data presented in this work and from the literature, please see Figure 3 for references. Modified from Hoernle and Schmincke (1993), Anguita and Hernán (2000), Troll and Carracedo (2016).
6 Conclusion
In this paper, we reported the first carbon isotopic data from FI of the Tajogaite lavas erupted in 2021 at La Palma. We also included new δ13C information obtained from lavas and ultramafic xenoliths collected in the San Antonio volcano and reviewed previously published analytical δ13C data on volcanic springs/fumaroles (particularly from La Palma and Tenerife) and FI (from El Hierro and La Palma) with the aim of better characterizing the isotopic signature of the local mantle source. The first δ13C data of FI trapped in peridotite xenoliths from Lanzarote are also reported.
Our findings show that the δ13C of La Palma fluids, including free gases and mineral-hosted FI, range between −5.16‰ and −2.21‰. Conversely, δ13C from Lanzarote xenoliths are more positive (from −2.83 to +0.80‰) and very similar to isotopic data previously reported in mantle xenoliths from El Hierro, pointing to a regional infiltration of the mantle beneath the Canary Islands by crustal C-rich fluids with heavy isotope signature (δ13C > −3‰). With these new data we can propose that the variability of δ13C at La Palma can be explained either by an open-degassing process starting from a mantle endmember with δ13C = −1.70‰ or by a binary mixing between a mantle reservoir (δ13C = −6.0‰) and a crustal carbon reservoir with a δ13C–0‰. In both cases, our data prove the presence of a crustal carbon reservoir in the local mantle under La Palma.
Data availability statement
The original contributions presented in the study are included in the article/Supplementary Material, further inquiries can be directed to the corresponding author.
Author contributions
AS-V: Conceptualization, Data curation, Formal Analysis, Investigation, Methodology, Software, Visualization, Writing–original draft. FC: Data curation, Formal Analysis, Investigation, Writing–review and editing. TN: Conceptualization, Investigation, Supervision, Writing–review and editing. AA: Conceptualization, Formal Analysis, Funding acquisition, Investigation, Supervision, Validation, Visualization, Writing–review and editing. MC: Conceptualization, Investigation, Methodology, Resources, Supervision, Visualization, Writing–review and editing. MF: Investigation, Supervision, Validation, Visualization, Writing–review and editing. MA: Investigation, Resources, Visualization, Writing–review and editing. EP: Investigation, Resources, Visualization, Writing–review and editing. Matthew MP: Writing–review and editing, Investigation, Resources, Visualization. NP: Writing–review and editing, Investigation, Resources, Visualization. AR: Conceptualization, Formal Analysis, Investigation, Methodology, Supervision, Validation, Visualization, Writing–review and editing.
Funding
The author(s) declare financial support was received for the research, authorship, and/or publication of this article. This research was funded by the Italian Minister (PRIN2017LMNLAW and PRIN2022HA8XCS), by the Deep Carbon Observatory, by the RETURN Extended Partnership funded by the European Union Next-Generation EU (National Recovery and Resilience Plan–NRRP, Mission 4, Component 2, Investment 1.3–D.D. 1243 2/8/2022, PE0000005), by the National Biodiversity Future Center (National Recovery and Resilience Plan–NRRP, Mission 4, Component 2, Investment 1.4, CN00000033) and by the projects VOLRISKMAC II (MAC2/3.5b/328), co-financed by the EC Cooperation Transnational Program MAC 2014-2020, and “Cumbre Vieja Emergencia”, financed by the Spanish Ministry of Science and Innovation.
Acknowledgments
We thank Mariano Tantillo and Mariagrazia Misseri for helping in samples preparation and CO2 extraction from minerals, and Igor Oliveri and Giorgio Capasso for the isotope analysis of carbon of CO2 performed in the stable isotope laboratory of INGV-Palermo. Finally, we also thank the Chief editor Prof. Valerio Acocella, editor Prof. Carmen Solana, Prof. Karoly Nemeth and an anonymous Reviewer whose comments and suggestions improved our manuscript.
Conflict of interest
The authors declare that the research was conducted in the absence of any commercial or financial relationships that could be construed as a potential conflict of interest.
Publisher’s note
All claims expressed in this article are solely those of the authors and do not necessarily represent those of their affiliated organizations, or those of the publisher, the editors and the reviewers. Any product that may be evaluated in this article, or claim that may be made by its manufacturer, is not guaranteed or endorsed by the publisher.
Supplementary material
The Supplementary Material for this article can be found online at: https://www.frontiersin.org/articles/10.3389/feart.2024.1303872/full#supplementary-material
References
Admunsen, H. E. F. (1987). Peridotite xenoliths from Gran Canaria,Canary Islands; evidence for metasomatic processes and partial melting in the lower oceanic crust. Neues Jahrb. Für Mineral. Abh. 156, 121–140.
Anguita, F., and Hernán, F. (2000). The Canary Islands origin: a unifying model. J. Volcanol. Geotherm. Res. 103, 1–26. doi:10.1016/S0377-0273(00)00195-5
Aubaud, C. (2022). Carbon stable isotope constraints on CO2 degassing models of ridge, hotspot and arc magmas. Chem. Geol. 605, 120962. doi:10.1016/j.chemgeo.2022.120962
Aubaud, C., Pineau, F., Hékinian, R., and Javoy, M. (2005). Degassing of CO2 and H2O in submarine lavas from the Society hotspot. Earth Planet. Sci. Lett. 235, 511–527. doi:10.1016/j.epsl.2005.04.047
Barry, P. H., Hilton, D. R., Füri, E., Halldórsson, S. A., and Grönvold, K. (2014). Carbon isotope and abundance systematics of Icelandic geothermal gases, fluids and subglacial basalts with implications for mantle plume-related CO2 fluxes. Geochim. Cosmochim. Acta 134, 74–99. doi:10.1016/j.gca.2014.02.038
Boudoire, G., Rizzo, A. L., Di Muro, A., Grassa, F., and Liuzzo, M. (2018). Extensive CO2 degassing in the upper mantle beneath oceanic basaltic volcanoes: first insights from Piton de la Fournaise volcano (La Réunion Island). Geochim. Cosmochim. Acta 235, 376–401. doi:10.1016/j.gca.2018.06.004
Carracedo, J. C., Badiola, E. R., Guillou, H., De la Nuez, J., and Pérez Torrado, F. J. (2001). Geología y vulcanología de La Palma y El Hierro, canarias occidentales. Estud. Geol. 57, 175–273. doi:10.3989/egeol.01575-6134
Carracedo, J. C., Day, S., Guillou, H., Rodríguez Badiola, E., Canas, J. A., and Pérez Torrado, F. J. (1998). Hotspot volcanism close to a passive continental margin: the Canary Islands. Geol. Mag. 135, 591–604. doi:10.1017/S0016756898001447
Carracedo, J. C., Troll, V. R., Day, J. M. D., Geiger, H., Aulinas, M., Soler, V., et al. (2022). The 2021 eruption of the Cumbre Vieja volcanic ridge on La Palma, canary islands. Geol. Today 38, 94–107. doi:10.1111/gto.12388
Colombo, E. (2020). Petrological study of peridotite xenoliths of El Hierro island: characterization of the lithospheric mantle beneath the Canary Islands (Spain) (Master’s Thesis). Milan: University of Milano-Bicocca.
Dasgupta, R., Jackson, M. G., and Lee, C.-T. A. (2010). Major element chemistry of ocean island basalts — conditions of mantle melting and heterogeneity of mantle source. Earth Planet. Sci. Lett. 289, 377–392. doi:10.1016/j.epsl.2009.11.027
Day, J. M. D., and Hilton, D. R. (2011). Origin of 3He/4He ratios in HIMU-type basalts constrained from Canary Island lavas. Earth Planet. Sci. Lett. 305, 226–234. doi:10.1016/j.epsl.2011.03.006
Day, J. M. D., and Hilton, D. R. (2021). Heterogeneous mantle-derived helium isotopes in the CanaryIslands and other ocean islands. Geology 49, 120–124. doi:10.1130/G47676.1
Day, J. M. D., Pearson, D. G., Macpherson, C. G., Lowry, D., and Carracedo, J. C. (2010). Evidence for distinct proportions of subducted oceanic crust and lithosphere in HIMU-type mantle beneath El Hierro and La Palma, Canary Islands. Geochim. Cosmochim. Acta 74, 6565–6589. doi:10.1016/j.gca.2010.08.021
Day, J. M. D., Troll, V. R., Aulinas, M., Deegan, F. M., Geiger, H., Carracedo, J. C., et al. (2022). Mantle source characteristics and magmatic processes during the 2021 La Palma eruption. Earth Planet. Sci. Lett. 597, 117793. doi:10.1016/j.epsl.2022.117793
Deines, P. (2002). The carbon isotope geochemistry of mantle xenoliths. Earth-Sci. Rev. 58, 247–278. doi:10.1016/S0012-8252(02)00064-8
del Fresno, C., Cesca, S., Klügel, A., Domínguez Cerdeña, I., Díaz-Suárez, E. A., Dahm, T., et al. (2023). Magmatic plumbing and dynamic evolution of the 2021 La Palma eruption. Nat. Commun. 14, 358. doi:10.1038/s41467-023-35953-y
Duncan, R. A., McDougall, I., Carter, R. M., and Coombs, D. S. (1974). Pitcairn Island—another Pacific hot spot? Nature 251, 679–682. doi:10.1038/251679a0
Frezzotti, M. L., Andersen, T., Neumann, E.-R., and Simonsen, S. L. (2002a). Carbonatite melt–CO2 fluid inclusions in mantle xenoliths from Tenerife, Canary Islands: a story of trapping, immiscibility and fluid–rock interaction in the upper mantle. Lithos 64, 77–96. doi:10.1016/S0024-4937(02)00178-0
Frezzotti, M.-L., and Touret, J. L. R. (2014). CO2, carbonate-rich melts, and brines in the mantle. Geosci. Front. 5, 697–710. doi:10.1016/j.gsf.2014.03.014
Frezzotti, M.-L., Touret, J. L. R., and Neumann, E.-R. (2002b). Ephemeral carbonate melts in the upper mantle: carbonate-silicate immiscibility in microveins and inclusions within spinel peridotite xenoliths, La Gomera, Canary Islands. Eur. J. Mineral. 14, 891–904. doi:10.1127/0935-1221/2002/0014-0891
Fuster, J. M., Cendrero, A., Gastesi, E., Ibanola, E., and Lopez-Ruiz, J. (1968). Geology and Volcanology of the canary islands-fuerteventura. Madrid: Consejo Sup. Invest. Cient.
Gennaro, M. E., Grassa, F., Martelli, M., Renzulli, A., and Rizzo, A. L. (2017). Carbon isotope composition of CO 2 -rich inclusions in cumulate-forming mantle minerals from Stromboli volcano (Italy). J. Volcanol. Geotherm. Res. 346, 95–103. doi:10.1016/j.jvolgeores.2017.04.001
Graham, D. W. (2002). Noble gas isotope geochemistry of mid-Ocean Ridge and Ocean Island basalts: characterization of mantle source reservoirs. Rev. Mineral. Geochem. 47, 247–317. doi:10.2138/rmg.2002.47.8
Guillou, H., Carracedo, J. C., and Day, S. J. (1998). Dating of the Upper Pleistocene–Holocene volcanic activity of La Palma using the unspiked K–Ar technique. J. Volcanol. Geotherm. Res. 86, 137–149. doi:10.1016/S0377-0273(98)00074-2
Guillou, H., Carracedo, J. C., and Duncan, R. A. (2001). K–Ar, 40Ar–39Ar ages and magnetostratigraphy of Brunhes and Matuyama lava sequences from La Palma Island. J. Volcanol. Geotherm. Res. 106, 175–194. doi:10.1016/S0377-0273(00)00294-8
Güleç, N., and Hilton, D. R. (2016). Turkish geothermal fields as natural analogues of CO 2 storage sites: gas geochemistry and implications for CO 2 trapping mechanisms. Geothermics 64, 96–110. doi:10.1016/j.geothermics.2016.04.008
Gurenko, A. A., Hoernle, K. A., Hauff, F., Schmincke, H.-U., Han, D., Miura, Y. N., et al. (2006). Major, trace element and Nd–Sr–Pb–O–He–Ar isotope signatures of shield stage lavas from the central and western Canary Islands: insights into mantle and crustal processes. Chem. Geol. 233, 75–112. doi:10.1016/j.chemgeo.2006.02.016
Gurenko, A. A., Hoernle, K. A., Sobolev, A. V., Hauff, F., and Schmincke, H.-U. (2010). Source components of the Gran Canaria (Canary Islands) shield stage magmas: evidence from olivine composition and Sr–Nd–Pb isotopes. Contrib. Mineral. Petrol. 159, 689–702. doi:10.1007/s00410-009-0448-8
Gurenko, A. A., Sobolev, A. V., Hoernle, K. A., Hauff, F., and Schmincke, H.-U. (2009). Enriched, HIMU-type peridotite and depleted recycled pyroxenite in the Canary plume: a mixed-up mantle. Earth Planet. Sci. Lett. 277, 514–524. doi:10.1016/j.epsl.2008.11.013
Herzberg, C., Asimow, P. D., Arndt, N., Niu, Y., Lesher, C. M., Fitton, J. G., et al. (2007). Temperatures in ambient mantle and plumes: constraints from basalts, picrites, and komatiites. Geochem. Geophys. Geosystems 8. doi:10.1029/2006GC001390
Hilton, D. R., Macpherson, C. G., and Elliott, T. R. (2000). Helium isotope ratios in mafic phenocrysts and geothermal fluids from La Palma, the Canary Islands (Spain): implications for HIMU mantle sources. Geochim. Cosmochim. Acta 64, 2119–2132. doi:10.1016/S0016-7037(00)00358-6
Hoefs, J. (2015). Stable isotope geochemistry. Cham: Springer International Publishing. doi:10.1007/978-3-319-19716-6
Hoernle, K., and Schmincke, H.-U. (1993). The role of partial melting in the 15-ma geochemical evolution of gran Canaria: a blob model for the canary hotspot. J. Petrol. 34, 599–626. doi:10.1093/petrology/34.3.599
Hoernle, K., Tilton, G., and Schmincke, H.-U. (1991). SrNdPb isotopic evolution of Gran Canaria: evidence for shallow enriched mantle beneath the Canary Islands. Earth Planet. Sci. Lett. 106, 44–63. doi:10.1016/0012-821X(91)90062-M
Hoernle, K., Zhang, Y.-S., and Graham, D. (1995). Seismic and geochemical evidence for large-scale mantle upwelling beneath the eastern Atlantic and western and central Europe. Nature 374, 34–39. doi:10.1038/374034a0
Hofmann, A. W. (2007). “Sampling mantle heterogeneity through oceanic basalts: isotopes and trace elements,” in Treatise on geochemistry (Elsevier), 1–44. doi:10.1016/B0-08-043751-6/02123-X
Jackson, M. G. (2016). “Oceanic island basalts,” in Encyclopedia of geochemistry, encyclopedia of Earth sciences series. Editor W. M. White (Cham: Springer International Publishing), 1–5. doi:10.1007/978-3-319-39193-9_248-1
Javoy, M., Pineau, F., and Iiyama, I. (1978). Experimental determination of the isotopic fractionation between gaseous CO2 and carbon dissolved in tholeiitic magma: a preliminary study. Contrib. Mineral. Petrol. 67, 35–39. doi:10.1007/BF00371631
Klügel, A., Galipp, K., Hoernle, K., Hauff, F., and Groom, S. (2017). Geochemical and volcanological evolution of La Palma, canary islands. J. Petrol. 58, 1227–1248. doi:10.1093/petrology/egx052
Longpré, M.-A. (2021). Reactivation of Cumbre Vieja volcano. Science 374, 1197–1198. doi:10.1126/science.abm9423
Machado, F., Parsons, W. H., Richards, A. F., and Mulford, J. W. (1962). Capelinhos eruption of fayal volcano, Azores, 1957–1958. J. Geophys. Res. 1896-1977 67, 3519–3529. doi:10.1029/JZ067i009p03519
Mattey, D. P. (1991). Carbon dioxide solubility and carbon isotope fractionation in basaltic melt. Geochim. Cosmochim. Acta 55, 3467–3473. doi:10.1016/0016-7037(91)90508-3
Melián, G., Tassi, F., Pérez, N., Hernandez, P., Sortino, F., Vaselli, O., et al. (2012). A magmatic source for fumaroles and diffuse degassing from the summit crater of Teide Volcano (Tenerife, Canary Islands): a geochemical evidence for the 2004-2005 seismic-volcanic crisis. Bull. Volcanol. 74, 1465–1483. doi:10.1007/s00445-012-0613-1
Neumann, E.-R. (1991). Ultramafic and mafic xenoliths from Hierro, Canary Islands: evidence for melt infiltration in the upper mantle. Contrib. Mineral. Petrol. 106, 236–252. doi:10.1007/BF00306436
Neumann, E.-R., Griffin, W. L., Pearson, N. J., and O'reilly, S. Y. (2004). The evolution of the upper mantle beneath the canary islands: information from trace elements and Sr isotope ratios in minerals in mantle xenoliths. J. Petrol. 45, 2573–2612. doi:10.1093/petrology/egh063
Neumann, E.-R., and Wulff-Pedersen, E. (1997). The origin of highly silicic glass in mantle xenoliths from the canary islands. J. Petrol. 38, 1513–1539. doi:10.1093/petroj/38.11.1513
Oglialoro, E., Frezzotti, M. L., Ferrando, S., Tiraboschi, C., Principe, C., Groppelli, G., et al. (2017). Lithospheric magma dynamics beneath the El Hierro volcano, canary islands: insights from fluid inclusions. Bull. Volcanol. 79, 70. doi:10.1007/s00445-017-1152-6
Padrón, E., Pérez, N. M., Hernández, P. A., Sumino, H., Melián, G., Barrancos, J., et al. (2012). Helium emission at Cumbre Vieja volcano, La Palma, canary islands. Chem. Geol. 312–313, 138–147. doi:10.1016/j.chemgeo.2012.04.018
Padrón, E., Pérez, N. M., Hernández, P. A., Sumino, H., Melián, G. V., Alonso, M., et al. (2022). Early precursory changes in the 3 He/4 He ratio prior to the 2021 tajogaite eruption at Cumbre Vieja volcano, La Palma, canary islands. Geophys. Res. Lett. 49. doi:10.1029/2022GL099992
Padrón, E., Pérez, N. M., Rodríguez, F., Melián, G., Hernández, P. A., Sumino, H., et al. (2015). Dynamics of diffuse carbon dioxide emissions from Cumbre Vieja volcano, La Palma, canary islands. Bull. Volcanol. 77, 28. doi:10.1007/s00445-015-0914-2
Pankhurst, M. J., Scarrow, J. H., Barbee, O. A., Hickey, J., Coldwell, B. C., Rollinson, G. K., et al. (2022). Rapid response petrology for the opening eruptive phase of the 2021 Cumbre Vieja eruption, La Palma, Canary Islands. Volcanica 5, 1–10. doi:10.30909/vol.05.01.0110
Pérez, N. M., Nakai, S., Wakita, H., Sano, Y., and Williams, S. N. (1994). 3He/4He isotopic ratios in volcanic-hydrothermal discharges from the canary islands, Spain: implications on the origin of the volcanic activity. Mineral. Mag. 58, 709–710. doi:10.1180/minmag.1994.58A.2.107
Pineau, F., and Mathez, E. A. (1990). Carbon isotopes in xenoliths from the Hualalai Volcano, Hawaii, and the generation of isotopic variability. Geochim. Cosmochim. Acta 54, 217–227. doi:10.1016/0016-7037(90)90209-4
Porcelli, D. R., O’Nions, R. K., Galer, S. J. G., Cohen, A. S., and Mattey, D. P. (1992). Isotopic relationships of volatile and lithophile trace elements in continental ultramafic xenoliths. Contrib. Mineral. Petrol. 110, 528–538. doi:10.1007/BF00344086
Remigi, S., Frezzotti, M. L., and Ferrando, S. (2019). Generation of CO2 - SO2 fluxes in the lithospheric mantle beneath El Hierro (Canary Islands) on metasomatic reactions of carbonate-rich silicate melts, Intervento presentato a: ecrofi. Budapest: Università di Milano Biccoca. Milan.
Rose, J., and Koppers, A. A. P. (2019). Simplifying age progressions within the cook-austral islands using ARGUS-VI high-resolution 40 Ar/39 Ar incremental heating ages. Geochem. Geophys. Geosystems 20, 4756–4778. doi:10.1029/2019GC008302
Sandoval-Velasquez, A. (2022). Noble gas and CO2 isotopic signatures of the lithospheric mantle underneath Mexico and the Canary Islands: clues from mantle xenoliths and arc lavas (PhD). Palermo: Universita degli Studi di Palermo.
Sandoval-Velasquez, A., Rizzo, A. L., Aiuppa, A., and Coltorti, M., 2022a. The radiogenic nature of the lithospheric mantle beneath Lanzarote (canary islands). Presented at the congresso SGI-SIMP, società geologica italiana. doi:10.3301/ABSGI.2022.02
Sandoval-Velasquez, A., Rizzo, A. L., Aiuppa, A., Remigi, S., Padrón, E., Pérez, N. M., et al. (2021b). Recycled crustal carbon in the depleted mantle source of El Hierro volcano, Canary Islands. Lithos 400–401, 106414. doi:10.1016/j.lithos.2021.106414
Sandoval-Velasquez, A., Rizzo, A. L., Aiuppa, A., Straub, S. M., Gomez-Tuena, A., and Espinasa-Perena, R. (2022c). The heterogeneity of the Mexican lithospheric mantle: clues from noble gas and CO2 isotopes in fluid inclusions. Front. Earth Sci. 10, 973645. doi:10.3389/feart.2022.973645
Sandoval-Velasquez, A., Rizzo, A. L., Casetta, F., Ntaflos, T., Aiuppa, A., Alonso, M., et al. (2022b). “The nature of the lithospheric mantle under La Palma (Canary Islands): new data on noble gases and CO2 isotopes from the 2021 Cumbre Vieja eruption,” in Conferenza A. Rittmann.
Sandoval-Velasquez, A., Rizzo, A. L., Casetta, F., Ntaflos, T., Aiuppa, A., Alonso, M., et al. (2023). The noble gas signature of the 2021 Tajogaite eruption (La Palma, Canary Islands). J. Volcanol. Geotherm. Res. 443, 107928. doi:10.1016/j.jvolgeores.2023.107928
Sandoval-Velasquez, A., Rizzo, A. L., Frezzotti, M. L., Saucedo, R., and Aiuppa, A. (2021a). The composition of fluids stored in the central Mexican lithospheric mantle: inferences from noble gases and CO2 in mantle xenoliths. Chem. Geol. 576, 120270. doi:10.1016/j.chemgeo.2021.120270
Sano, Y., and Marty, B. (1995). Origin of carbon in Fumarolic gas from island arcs. Chem. Geol. 119, 265–274. doi:10.1016/0009-2541(94)00097-R
Siena, F., Beccaluva, L., Coltorti, M., Marchesi, S., and Morra, V. (1991). Ridge to hot-spot evolution of the atlantic lithospheric mantle: evidence from Lanzarote peridotite xenoliths (canary islands). J. Petrol. Special_Volume Special_Volume, 271–290. doi:10.1093/petrology/Special_Volume.2.271
Simmons, S. F., and Christenson, B. W. (1994). Origins of calcite in a boiling geothermal system. Am. J. Sci. 294, 361–400. doi:10.2475/ajs.294.3.361
Simonsen, S., Neumann, E.-R., and Seim, K. (2000). Sr–Nd–Pb isotope and trace-element geochemistry evidence for a young HIMU source and assimilation at Tenerife (Canary Island). J. Volcanol. Geotherm. Res. - J. Volcanol. Geotherm. Res. 103, 299–312. doi:10.1016/S0377-0273(00)00228-6
Torres-González, P. A., Luengo-Oroz, N., Lamolda, H., D’Alessandro, W., Albert, H., Iribarren, I., et al. (2020). Unrest signals after 46 years of quiescence at Cumbre Vieja, La Palma, canary islands. J. Volcanol. Geotherm. Res. 392, 106757. doi:10.1016/j.jvolgeores.2019.106757
Troll, V. R., and Carracedo, J. C. (2016). The geology of the canary islands. Elsevier. doi:10.1016/C2015-0-04268-X
Venturi, S., Tassi, F., Bicocchi, G., Cabassi, J., Capecchiacci, F., Capasso, G., et al. (2017). Fractionation processes affecting the stable carbon isotope signature of thermal waters from hydrothermal/volcanic systems: the examples of Campi Flegrei and Vulcano Island (southern Italy). J. Volcanol. Geotherm. Res. 345, 46–57. doi:10.1016/j.jvolgeores.2017.08.001
Keywords: Canary Islands, La Palma, 2021 Tajogaite eruption, Ocean island volcanism, fluid inclusions, carbon isotopes, recycled carbon
Citation: Sandoval-Velasquez A, Casetta F, Ntaflos T, Aiuppa A, Coltorti M, Frezzotti ML, Alonso M, Padrón E, Pankhurst M, Pérez NM and Rizzo AL (2024) 2021 Tajogaite eruption records infiltration of crustal fluids within the upper mantle beneath La Palma, Canary Islands. Front. Earth Sci. 12:1303872. doi: 10.3389/feart.2024.1303872
Received: 28 September 2023; Accepted: 29 January 2024;
Published: 14 February 2024.
Edited by:
Carmen Solana, University of Portsmouth, United KingdomReviewed by:
Karoly Nemeth, Institute of Earth Physics and Space Science (EPSS), HungaryPengchun Li, Chinese Academy of Sciences (CAS), China
Copyright © 2024 Sandoval-Velasquez, Casetta, Ntaflos, Aiuppa, Coltorti, Frezzotti, Alonso, Padrón, Pankhurst, Pérez and Rizzo. This is an open-access article distributed under the terms of the Creative Commons Attribution License (CC BY). The use, distribution or reproduction in other forums is permitted, provided the original author(s) and the copyright owner(s) are credited and that the original publication in this journal is cited, in accordance with accepted academic practice. No use, distribution or reproduction is permitted which does not comply with these terms.
*Correspondence: A. Sandoval-Velasquez, YW5kcmVzbGliYXJkby5zYW5kb3ZhbHZlbGFzcXVlekBjb21tdW5pdHkudW5pcGEuaXQ=