Corrigendum: Preservation of chemical and isotopic signatures within the Weißseespitze millennial old ice cap (Eastern Alps), despite the ongoing ice loss
- 1Department of Environmental Sciences, Informatics and Statistics, Ca’ Foscari University of Venice, Venice, Italy
- 2CNR-Institute of Polar Sciences (ISP-CNR), Mestre, Italy
- 3Institute for Interdisciplinary Mountain Research of the Austrian Academy of Sciences, Innsbruck, Austria
- 4GeoSphere Austria, Vienna, Austria
Alpine ice core research has long focused on a few suitable drilling sites at high elevation in the Western European Alps, assuming that the counterparts at lower elevation in the eastern sector are unsuitable for paleoenvironmental studies, due to the presence of melting and temperate basal conditions. However, it has been demonstrated that even in the Eastern Alpine range, below 4,000 m a.s.l., cold ice frozen to bedrock can exist. In fact, millennial-old ice has been found at some locations, such as at the Weißseespitze (WSS) summit ice cap (Ӧtztal Alps, 3,499 m a.s.l.), where about 6 kyrs appear locked into 10 m of ice. In this work, we present a full profile of the stable water isotopes (δ18O, δ2H), major ions (Na+, K+, Mg2+, Ca2+, NH4+, Cl−, NO3−, SO42−), levoglucosan, and microcharcoal for two parallel ice cores drilled at the Weißseespitze cap. We find that, despite the ongoing ice loss, the chemical and isotopic signatures appear preserved, and may potentially offer an untapped climatic record. This is especially noteworthy considering that chemical signals of other archives at similar locations have been partially or full corrupted by meltwater (i.e., Silvretta glacier, Grand Combin glacier, Ortles glacier). In addition, the impurity concentration near the surface shows no signs of anthropogenic contamination at WSS, which constrains the age at the surface to fall within the pre-industrial age.
1 Introduction
European Alpine glaciers represent unique targets for ice core studies focusing on reconstructing environmental and climatic conditions in the Holocene. Since its beginning, a primary aim of ice core research in the Alps was retrieving continuous stratigraphic climate records, which restricted it to glaciers without significant melting on the surface throughout the year. In this strict view, only few suitable drilling sites exist as they are mostly confined to above 4,000 m altitude and hence located in the Western Alps, which have been exploited in numerous successful studies over the past 4 decades (Bohleber, 2019, and references therein). Following evidence that old ice may also exist in the Eastern Alps and at elevations below 4,000 m (Haeberli et al., 2004), new efforts targeted the either direct access to the ice at the glacier base (Bohleber et al., 2018) or the drilling of ice cores at both temperate (Pavlova et al., 2015; Festi et al., 2017), partially temperate but cold-based (Gabrielli et al., 2016) and predominantly cold ice sites (Bohleber et al., 2020a). In concert with state-of-the-art radiocarbon ice dating (Uglietti et al., 2016; Hoffmann et al., 2018), the access to the stagnant cold ice at the glacier base revealed that millennial-old ice is still preserved even if the remaining thickness is only around 10 m or less, adding important information for reconstructing the Holocene neoglaciation history of the Alps (Bohleber et al., 2020a). Retrieving the paleoclimate and environmental information potentially stored in these ice cores’ chemical and isotopic stratigraphy means facing additional complexity to what it is already known for the “classical” ice core targets in the Western Alps (Wagenbach et al., 2012). First, due to ongoing and prolonged mass loss also the age of the ice at the surface becomes an unknown parameter that requires separate dating efforts with innovative approaches (Festi et al., 2021). Second, due to their lower elevation, these sites are typically much closer to the equilibrium line altitude of the glacier, making them not only more vulnerable to present warming conditions but also a potential sensitive indicator of past climate shifts impacting their energy and mass balance. In fact, prolonged periods of stagnation or mass loss may have occurred and resulted in stratigraphic discontinuities at such sites (Fischer et al., 2022). Third, meltwater percolation can corrupt and ultimately erase completely the chemical and isotopic information in the stratigraphy, although the degree of the disturbances may depend on the impurity species and the degree of percolation (Eichler et al., 2001; Avak et al., 2018). A more comprehensive understanding of these complex settings is much needed, as they may also affect the highest summits in the future considering ongoing warming conditions.
Here we present recent progress in evaluating these challenges for the new ice cores drilled at the Weißseespitze cap, Eastern Alps (WSS). We use for this purpose profiles of the stable water isotopes (δ2H and δ18O), major ion chemistry as well as a full profile of microcharcoal and levoglucosan. The latter represents a novelty for ice core studies over the alpine range, since only a preliminary work at Col du Dome is currently available (Legrand et al., 2007). Levoglucosan was measured on the WSS ice core to investigate if evidence of past biomass burning events could be detected, since levoglucosan is particularly useful when source and deposition sites are close to each other (Alves et al., 2017).
2 Materials and methods
2.1 Glaciological settings of the ice core drilling site at Weißseespitze
The Weißseespitze ice cap (around 3,500 m a.s.l.) covers the top sections of Gepatschferner glacier in the Austrian Alps (Figures 1A, B), located only 12 km from the famous Tyrolean Iceman find site. Its limited ice thickness combined with a dome-shaped glacier geometry entails minimal to no ice flow, confirmed by differential GPS measurements at stakes in 2018 and 2019 (Fischer et al., 2022). Historical photographs dating back to about 1888, maps and digital elevation models reveal that the ice body today is the remnant of an approximately 40 m thicker ice cap diminished by prolonged ice loss (Figures 1C, D). Despite the prolonged ablation, englacial borehole temperatures remained permanently sub-zero at 1 m below surface, with −3°C at 9 m of depth (Fischer et al., 2022). A first ice core was drilled to bedrock (11 m in total, including about 1 m of snow cover but no firn) at the ice divide with nearly flat bed conditions in March 2019. An additional parallel core (8.7 m of depth to bedrock) was drilled at the same location in March 2021. The few visible layers of refrozen meltwater in the cores indicate that there was only limited occasional melt at this site when the ice formed. The main part of the ice cores includes bubble-rich glacier ice with bandings (Supplementary Figure S3), the likely result of dry metamorphism of snow (Figure 1E). Initial analysis supported this view, e.g., by stable oxygen and hydrogen ratios, exhibiting a range typical for the seasonal variation in snow at this altitude, and no systematic deviation from the meteoric water line (Bohleber et al., 2020a). The 2019 surface at WSS is older than 1950s, indicated by the absence of elevated tritium levels within the first 4 m of the core. The aerosol-based micro-14C dating indicated a minimum age of (0.623 ± 0.350) ka cal BP at 5.46 m of depth, and a maximum age of (5.884 ± 0.739) ka cal BP just above the bed. Further details on the glaciological settings have already been described in former studies (Bohleber et al., 2020a; Fischer et al., 2022).
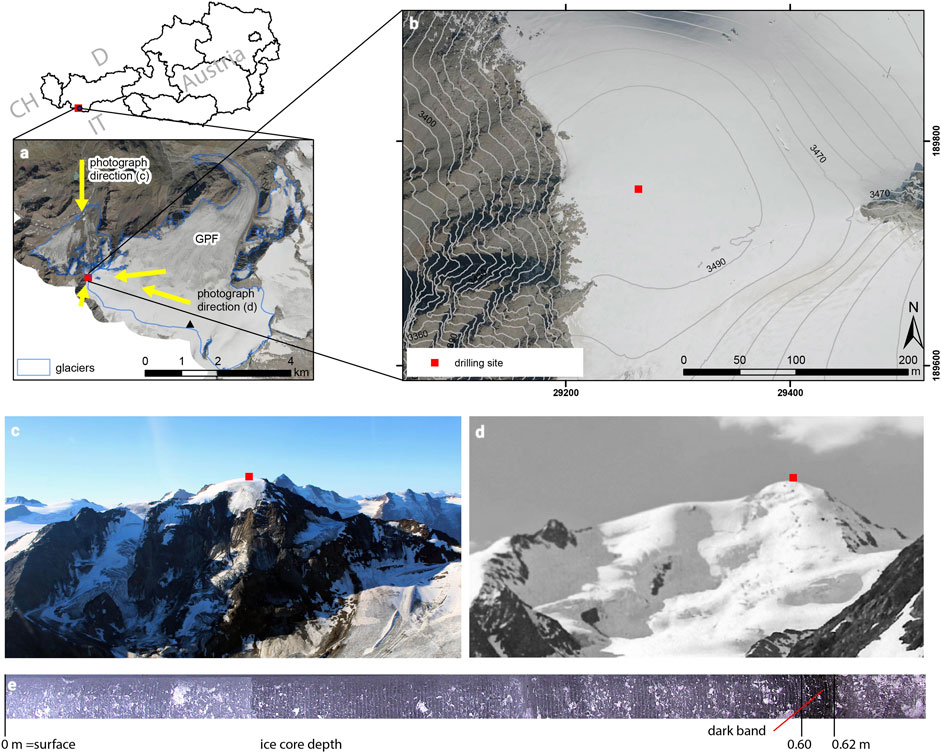
FIGURE 1. The ice core drilling site at the summit of the Weißseespitze ice cap (A,B). Datenquelle: Land Tirol—data. tirol.gv.at. Panoramic view of the Weißseespitze ice cap in 2019 (C), and 1930 (D). One of the ice cores taken in 2019 (E).
2.2 Ice core processing and analysis
To prepare for Continuous Flow Analysis (CFA) at Ca’ Foscari University of Venice, the 2019 core was processed to obtain 23 ice sticks (bags) with 32 × 32 mm sections. Only the top 8.5 m were considered suitable for the analysis, given the high concentration of visible debris at the bottom. The ice was cut with a modified commercial band saw, and refined with a decontaminated stainless-steel blade over a polyethylene tabletop accessorized with guide rails for cutting. The table, rails, and the blade were carefully cleaned with acetone and methanol to remove contamination before every use. All the exposed ice surfaces were rapidly scraped with a stainless-steel knife cleaned with 0.1% ultra-pure HNO3 (Romil, Cambridge, United Kingdom). This knife was used to remove the outer thin contaminated ice layer, and more mass was scraped from the two base surfaces which were to be placed on the melting head. Several mm of ice from each end were removed by using a second clean knife to ensure perfect contact to the melting head surface. The sections were stored in clean PTFE bags until the analyses conducted with the novel set-up of the Continuous Flow Analysis system realized at Ca’ Foscari, in collaboration with the National Research Council—Institute of Polar Science (CNR-ISP). This technique allows to continuously measure insoluble dust particles (1 acquisition sec−1) and levoglucosan (1 cm of resolution) within the meltwater stream, while sets of discrete samples (2.6 cm of ice depth equivalent per sample) were reserved for the off-line analysis of water stable isotopes and major ions, conducted via Cavity Ring-Down Spectrocopy (CRDS, Picarro inc.), and Ion Chromatrography (IC), respectively.
The overall CFA system coupled with Fast Liquid Chromatography tandem Mass Spectrometry (FLC—MS/MS), is illustrated in Barbaro et al. (2022), while the optimization of the method for levoglucosan continuous measurements is presented in Spagnesi et al. (2023).
In order to investigate the localization of the impurities in the ice matrix and their potential removal through meltwater percolation under temperate conditions, exemplary sections were analyzed at the University of Venice by 2D chemical imaging with laser ablation inductively-coupled plasma mass spectrometry (LA-ICP-MS). The LA-ICP-MS set-up comprised an Analyte Excite ArF excimer 193 nm laser (Teledyne CETAC Photon Machines) and an iCAP-RQ quadrupole ICP-MS (Thermo Scientific), connected via a rapid aerosol transfer line for fast washout. Samples surfaces are decontaminated with ceramic ZrO2 blades (American Cutting Edge, United States), and the sample is then placed on a cryogenic sample holder. A glycol-water mixture (−35°C) is used to cool the sample surface to −23± 2°C which is further cleaned by preablation with an 80 × 80 μm square spot before each measurement. Further details are described in Bohleber et al. (2020b).
Sample stripes of about 8 × 2 × 1 cm were cut from bags 2 and 18, at depths of 0.08–0.10 and 6.25–6.75 m, respectively. Images were recorded using a 40 micron spot over areas that showed visual evidence of grains and grain boundaries. Due to the comparatively large grains in bag 18, only one grain boundary was present within the image. Analytes were Na, Mg, Al, and Fe in order to consider species with mostly soluble (Na, Mg) as well as insoluble (Al, Fe) behavior.
The 8.4 m long ice core drilled in 2021 was cut in 106 continuous samples at 10 cm resolution from the surface to 6.6 m of depth, and at 5 cm resolution from 6.6 m to the bottom. The ice was cut with a modified commercial band saw. Samples were stored frozen in plastic bags and sent frozen to the Laboratory of Palynology and Paleoecology of CNR-IGAG for microfossils extraction (including microcharcoal) and preparation. Decontamination was performed using fridge-cooled distilled water and left to melt covered at room temperature. Sample volume was measured and samples were then filtered with a 7-µm filter to concentrate the microfossils (pollen, spores, microcharcoal and other non-pollen-palynomorphs). The residue contained in the filter underwent chemical treatment with HCl 10% and Acetolysis solution (Erdtman, 1960) as usual in pollen analyses. Finally, glycerin was added and slides were prepared for pollen and microcharcoal analyses, according to Festi et al. (2019). Microscopy slides were prepared in the Laboratory of Palynology and Paleoecology of CNR-IGAG and analyzed at the Institute for Interdisciplinary Mountain Research of the Austrian Academy of Sciences using a Motic BA310 light microscope. Microcharcoal particles (>7 µm) were quantified along with pollen grains as usual in pollen analyses to reconstruct fire history. Microcharcoal particles are easy to recognize as they are characterized by their jet-black, opaque, angular particles in samples; otherwise, the clear or brown, amorphous, weakly -structured particles are considered as vegetal matter (Patterson et al., 1987). For each sample, the complete content was analyzed. In this work, we present the microcharcoal record obtained for the core.
3 Results
3.1 Water stable isotopes
Water stable isotopes (δ18O, δ2H) and deuterium excess were measured in discrete samples, resulting in the depth profiles shown in Figure 2. The upper part of the core, ranging from 0 to roughly −2.30 m of depth, is characterized by distinct decimeter-scale variability over several permil in δ18O. We find two marked minima (δ18O: −22‰, −20‰; δ2H: −165‰, −152‰) located at −0.63 m and −2.27 m of depth, respectively, with isotopic signals similar to higher elevation Alpine sites (Wagenbach et al., 2012; Bohleber et al., 2013). By comparison, the deeper part of the core below 6 m only shows minor variability around a stable mean (−14‰ ± 1‰). However, there is a clear decimeter-scale variability found over the entire depth range that appears perfectly in line with trends observed even at Western Alpine sites (e.g., Schotterer et al., 1997).
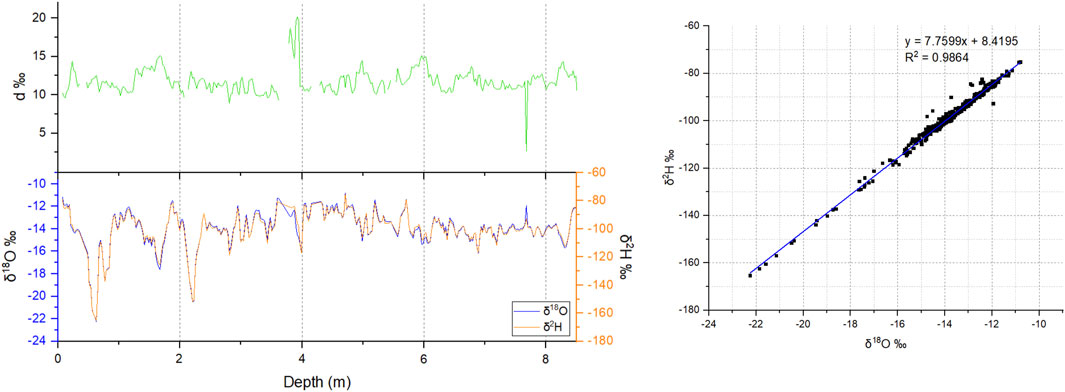
FIGURE 2. Deuterium excess (d), δ18O and δ2H profiles (‰) along the WSS ice core (left), and δ18O/δ2H linear regression (right).
Overall, the deuterium excess record (d) does not show any clear trend, with values ranging between 10 and 15‰, similarly to what observed by Fröhlich et al. (2008) for stations located north and south of the main ridge of the Austrian Alps. Only one marked maximum, located around 4 m of depth, deviates from this general trend: this peak corresponds to a minimum in δ18O and δ2H; other negatively-correlated values between d and δ18O (δ2H) can be observed at −1.67, −4.99, −5.96, and −8.28 m of depth. The anticorrelation between δ18O and d-excess observed can be due to (a) seasonal variations of d-excess, with lower values associated to summer, and higher values related to winter in the Northern Hemisphere (Xia et al., 2022), although at the present resolution seasonal variations of the isotopic signal are not detectable, and we can only refer to “warmer” and “colder” periods; (b) abundant snowfalls that originated from areas characterized by vapor with higher d-excess (i.e., Mediterranean region or recycled moisture in continental areas) (e.g., Fröehlich et al., 2008).
The slope in the co-isotopic plot is 7.76 ± 0.05, with a R2 for the linear fit of 0.9864. This value is very close to the meteoric water line, and confirms previous results obtained by Bohleber et al. (2020a), for the same drill site, albeit at coarser depth resolution.
3.2 Major ions chemistry and levoglucosan
The obtained levoglucosan profile shows concentration ranges between 0.07 and 51.07 ng mL−1, with a major peak found at ∼ 6.40 m (Figure 3).
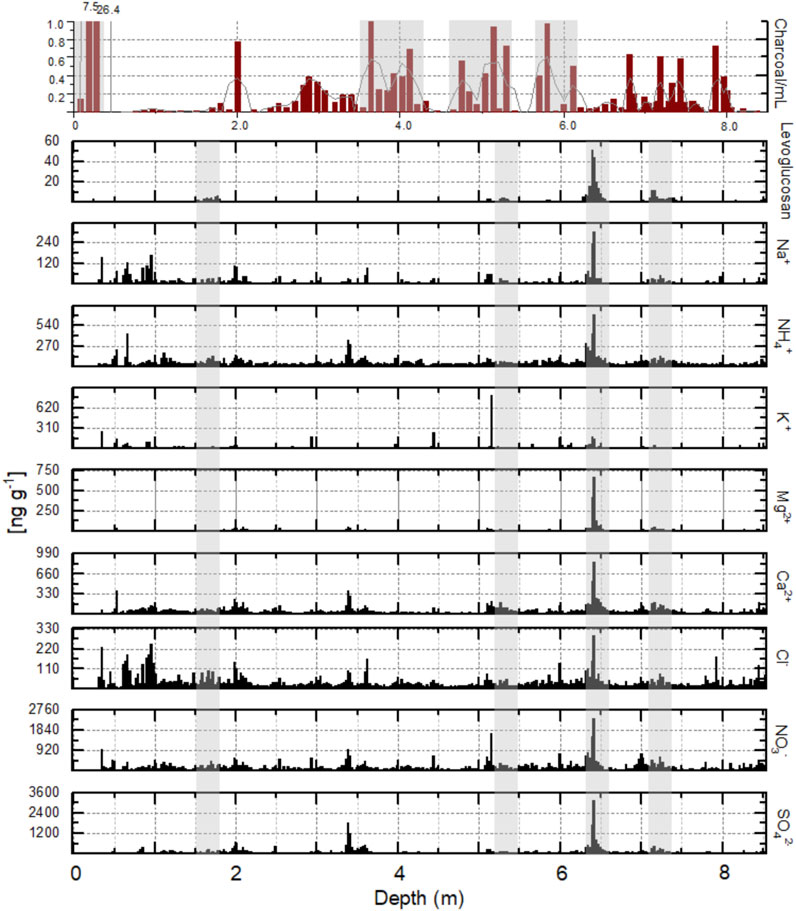
FIGURE 3. Full levoglucosan and chemistry profiles along the whole WSS longest core depth drilled in 2019. Concentrations on y-axes are expressed in ng mL−1. Grey bars indicate corresponding peaks between levoglucosan and major ions. Microcharcoal/mL profile along the 8.4 m length WSS core drilled in 2021 is presented in the above graph with brown bars.
Cationic (Na+, NH4+, K+, Mg2+, Ca2+), and anionic species (Cl−, NO3−, SO42−) were analyzed within the discrete samples collected during the melting campaign. The mean concentrations, SD, minimum, maximum, and median values of all the ionic compounds and water stable isotopes were computed over the whole core depth and the upper 1 m separately, but showing no significant differences between the two sets for NH4+, K+, Ca2+, NO3− (Table 1). The overall chemical composition is dominated by nitrate (NO3−, 37%), sulphate (SO42−, 23%), calcium (Ca2+, 12%), and ammonium (NH4+, 11%). Minor contributions were reported for Cl−, K+, Na+, and Mg2+, respectively, quantified as 6.93%, 3.82%, 3.05%, and 2.21% of the total ionic species.
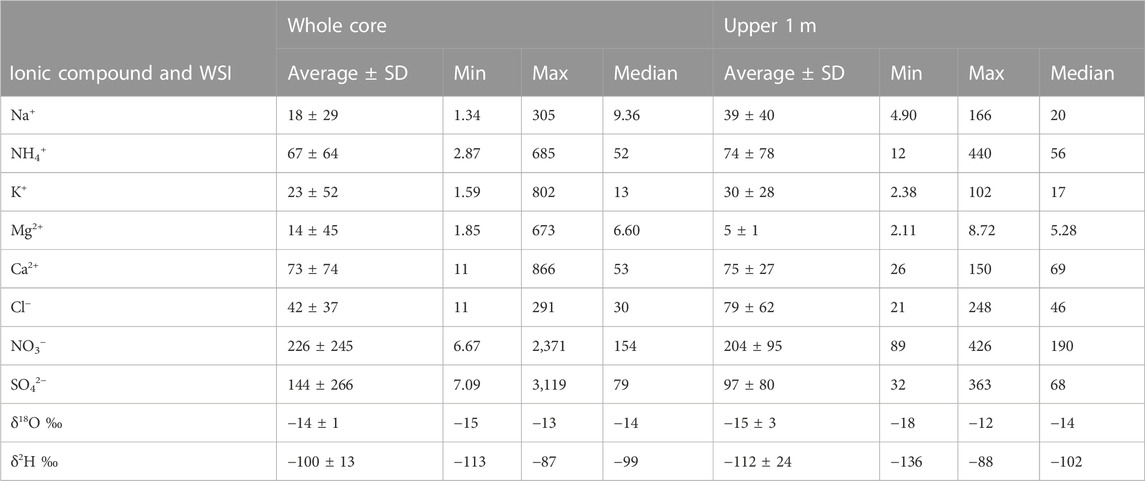
TABLE 1. Ionic compound and water stable isotopes (WSI) average, min, max, and median values for the whole core depth and the upper 1 m (concentrations are expressed in ng mL−1).
As investigated by LA-ICP-MS, impurities (Na, Mg, Al, Fe, Sr) are predominantly localized at grain boundaries, which are visible in Supplementary Figure S1 as lines of bright intensity. Supplementary Figure S1 shows the near surface sample (WSS bag 02), and the examples of Na, Mg and Al in a separate color channel. The second sample from bag 18 in shown in the supplement, with the same basic finding (Supplementary Figure S2).
3.3 Microcharcoal
Microcharcoal concentration in the core ranges from 0 to 26.4 particles per mL (Figure 3). Only 23 over 106 samples contained no microcharcoal and in the 8.4 m long profile, several peaks can be identified. The most prominent one is found 8 cm below the surface of the core, from 8 to 28 cm of depth and reaches the maximum concentration of 26.4 particles mL−1. Applying a smoothing, three further composite peaks stand out at 3.5–4 m, 4.7–5.2 m, and 5.6–6.2 m of depth.
4 Discussion and conclusion
The fact that no deviation from the meteoric water line is indicated in the co-isotopic plot suggests the absence of substantial melting and refreezing processes on the cm-scale (Craig, 1961), thus constituting an analogue situation to what was previously observed for other cold-based alpine summit sites (Bohleber et al., 2018; Bohleber et al., 2020a). This is also consistent with the fact that no clear visual evidence of refrozen ice manifesting as transparent bubble-free layers was found in visual analysis of this ice core. Throughout the entire record, notably including the near-surface layers, we find distinct variability in the chemical and isotopic signals obtained from the ice core. We can further exclude substantial percolation of meltwater, as this would have likely led to the continuous removal of impurities by gradual washing out, hence reducing and eventually removing any variability (Schotterer et al., 2004; Pavlova et al., 2015). This is consistent with what we have found in the exemplary LA-ICP-MS maps, which show a typical degree of impurity localization at grain boundaries previously observed in cold polar ice conditions (Bohleber et al., 2020b).
Furthermore, it is noteworthy that the near-surface layers show no distinct difference in concentration levels with respect to the rest of the core (Table 1). This indicates that the current surface at WSS is not only missing the 3H bomb horizon conventionally associated with the year 1963 as detected previously by Bohleber et al. (2020a), but that the present surface is in fact significantly older than 1950s and falls within the pre-industrial time period. Indeed, within the last 80–100 years, a distinct increase in most impurity species was expected, as observed in other Alpine ice cores due to anthropogenic emissions, in particular for NO3−, SO42−, and NH4+ (Schwikowski et al., 1999a; Schwikowski et al., 1999b; Preunkert et al., 2003; Wagenbach et al., 2012; Bohleber, 2019; Eichler et al., 2023).
Concerning the distribution of impurities along the 2019 WSS ice core, it is worth mentioning the visual correspondence of four peaks of levoglucosan and the investigated ions at 1.60–1.90 m, 5.00–5.50 m, 6.40 m, and 7.10–7.20 m of depth, with a major peak revealed at 6.40 m (Figure 3). Notably, the 6.40 m depth outstanding layer could be explained by a prolonged exposure to the atmosphere or an exceptional transport of impurities coming from different emitting sources. However, a post-depositional cause cannot be ruled out in this case.
At the same time, four outstanding periods are also present in the microcharcoal data analyzed in the 2021 core. This core was drilled at about 10 m distance from the 2019 core. Notably, the measured ice ablation at the surface shows a strong gradient on a very short distance between the stakes placed at the drilling sites, with up to 110 cm of ablation within the period 2019–2021. Considering these surface changes and that both levoglucosan and microcharcoal are proxies of biomass burning, it appears plausible to match the near-surface microcharcoal peak in 2021 with the first levoglucosan peak of the 2019 core. The striking correspondence of the four levoglucosan peaks with the four main microcharcoal concentration peaks supports the hypothesis that at least four main fire activity phases are recorded in the WSS ice record. Regional past fires occurred in the alpine area may have been recorded in the ice, furnishing a good correspondence between levoglucosan and microcharcoal trends. Indeed, the differential partition of levoglucosan and microcharcoal, respectively associated to submicron organic aerosol and higher particles dimensions, appears not crucial in case of short-range transport. However, the comparison with other nearby archives (e.g., peat bogs) would be helpful to clearly disentangle this feature, thus is strongly suggested for the future.
A more detailed interpretation of the impurity record and comparison with other Alpine ice core records would benefit greatly from constraining the seasonal bias in net snow deposition, which is to be expected at an exposed summit site. Monitoring today’s conditions at the surface is unsuitable for this purpose because it is dominated by ablation all throughout the year. Acknowledging the present uncertainty in the snow deposition bias, the impurity levels can indicate the general pre-industrial background in precipitation for this region.
We thus conclude that, despite the intense ablation which is acting at the surface of the Weißseespitze summit ice cap today, the cold ice remains mostly impermeable to meltwater which must be running off along the snow/firn layers. This is backed by the sub-zero temperatures measured inside the boreholes (Bohleber et al., 2020a; Fischer et al., 2022). As a result of these conditions, the ice contains preserved isotopic and chemical signals observed along the ice core depth. This is not to be expected a-priori considering what was revealed for other Alpine sites, such as the nearby Ortles (Gabrielli et al., 2016), the Silvretta glacier (Pavlova et al., 2015; Steinlin et al., 2015) or the Grand Combin glacier (Huber et al., 2022), where the archives have been partially lost, and flat signals (i.e., Silvretta glacier) or depleted impurity concentrations (i.e., Grand Combin) have been recorded. Furthermore, the chemical signal in the upper meters of the ice does not show any evidence of the anthropogenic increase during the 20th century known from other ice core. In particular, nitrates, sulphates, and ammonium present pre-industrial concentrations. These results corroborate the previous age constraints from 3H, showing the absence of the 1963 bomb horizon at the surface, but also indicate that today’s surface is much older and falls within the pre-industrial era. The lack of temporal overlap between the WSS record and instrumental data hampers constraining the seasonal representativeness and snow deposition bias so far. However, four major peaks have been recognized standing out for levoglucosan but also other impurity species. Based on the absence of evidence for disturbances by melting and refreezing, these events may stem from either singular biomass burning events or a surface with prolonged exposure to the atmosphere during a hiatus, but there is still an open question on the major peak’s origin found at 6.40 m of depth, since a post-depositional event cannot be ruled out with absolute certainty in this case. With a future more robust estimate of the age-depth relation at WSS, comparisons with similar ice core alpine records (e.g., ice cores from Alto dell’Ortles) or other natural archives (e.g., peatbogs), may offer new valuable insights regarding the regional significance of these outstanding horizons.
Data availability statement
Original datasets are available in a publicly accessible repository: The original contributions presented in the study are publicly available. This data can be found here: http://doi.org/10.5281/zenodo.10200834.
Author contributions
AS: Conceptualization, Data curation, Formal Analysis, Investigation, Methodology, Writing–original draft, Writing–review and editing. PB: Conceptualization, Data curation, Funding acquisition, Investigation, Writing–original draft, Writing–review and editing. EB: Conceptualization, Formal Analysis, Investigation, Methodology, Writing–review and editing. MF: Formal Analysis, Funding acquisition, Investigation, Methodology, Writing–review and editing. FD: Formal Analysis, Investigation, Methodology, Writing–review and editing. GD: Formal Analysis, Investigation, Methodology, Writing–review and editing. MS-W: Writing–review and editing. DF: Formal Analysis, Investigation, Writing–review and editing. JG: Methodology, Supervision, Writing–review and editing. AG: Supervision, Writing–review and editing. AF: Funding acquisition, Project administration, Resources, Supervision, Writing–review and editing. CB: Supervision, Validation, Writing–review and editing.
Funding
The author(s) declare financial support was received for the research, authorship, and/or publication of this article. This research was funded in part by the Austrian Science Fund (FWF) (I 5246-N and P34399-N).
Acknowledgments
PB gratefully acknowledges funding from the European Union’s Horizon 2020 research and innovation program under the Marie Skłodowska-Curie grant agreement no. 101018266.
Conflict of interest
The authors declare that the research was conducted in the absence of any commercial or financial relationships that could be construed as a potential conflict of interest.
Publisher’s note
All claims expressed in this article are solely those of the authors and do not necessarily represent those of their affiliated organizations, or those of the publisher, the editors and the reviewers. Any product that may be evaluated in this article, or claim that may be made by its manufacturer, is not guaranteed or endorsed by the publisher.
Supplementary material
The Supplementary Material for this article can be found online at: https://www.frontiersin.org/articles/10.3389/feart.2023.1322411/full#supplementary-material
References
Alves, C. A., Vicente, E. D., Rocha, S., and Vicente, A. M. (2017). Organic tracers in aerosols from the residential combustion of pellets and agro-fuels. Atmos. Health 10, 37–45. doi:10.1007/s11869-016-0406-3
Avak, S. E., Schwikowski, M., and Eichler, A. (2018). Impact and implications of meltwater percolation on trace element records observed in a high-Alpine ice core. J. Glaciol. 64 (248), 877–886. doi:10.1017/jog.2018.74
Barbaro, E., Feltracco, M., Spagnesi, A., Dallo, F., Gabrieli, J., De Blasi, F., et al. (2022). Fast Liquid Chromatography coupled with tandem mass spectrometry for the analysis of vanillic and syringic acids in ice cores. Anal. Chem. 94 (13), 5344–5351. doi:10.1021/acs.analchem.1c05412
Bohleber, P. (2019). “Alpine ice cores as climate and environmental archives,” in Oxford research Encyclopedia of climate science (Oxford University Press). doi:10.1093/acrefore/9780190228620.013.743
Bohleber, P., Hoffmann, H., Kerch, J., Sold, L., and Fischer, A. (2018). Investigating cold based summit glaciers through direct access to the glacier base: a case study constraining the maximum age of Chli Titlis glacier, Switzerland. Cryosphere 12 (1), 401–412. doi:10.5194/tc-12-401-2018
Bohleber, P., Roman, M., Šala, M., and Barbante, C. (2020b). Imaging the impurity distribution in glacier ice cores with LA-ICP-MS. JAAS 35 (10), 2204–2212. doi:10.1039/D0JA00170H
Bohleber, P., Schwikowski, M., Stocker-Waldhuber, M., Fang, L., and Fischer, A. (2020a). New glacier evidence for ice-free summits during the life of the Tyrolean Iceman. Sci. Rep. 10, 20513. doi:10.1038/s41598-020-77518-9
Bohleber, P., Wagenbach, D., Schöner, W., and Böhm, R. (2013). To what extent do water isotope records from low accumulation Alpine ice cores reproduce instrumental temperature series? Tellus B 65, 20148. doi:10.3402/tellusb.v65i0.20148
Craig, H. (1961). Isotopic variations in meteoric waters. Science 133, 1702–1703. doi:10.1126/science.133.3465.1702
Eichler, A., Legrand, M., Jenk, T. M., Preunkert, S., Andersson, C., Eckhardt, S., et al. (2023). Consistent histories of anthropogenic western European air pollution preserved in different Alpine ice cores. Cryosphere 17, 2119–2137. doi:10.5194/tc-17-2119-2023
Eichler, A., Schwikowski, M., and Gӓggeler, H. W. (2001). Meltwater-induced relocation of chemical species in Alpine firn. Tellus 53B, 192–203. doi:10.3402/tellusb.v53i2.16575
Festi, D., Carturan, L., Kofler, W., dalla Fontana, G., de Blasi, F., Cazorzi, F., et al. (2017). Linking pollen deposition and snow accumulation on the Alto dell'Ortles glacier (South Tyrol, Italy) for sub-seasonal dating of a firn temperate core. Cryosphere 11, 937–948. doi:10.5194/tc-11-937-2017
Festi, D., Kofler, W., and Oeggl, K. (2019). Comments on Brugger and others (2018) ‘A quantitative comparison of microfossil extraction methods from ice cores. J. Glaciol. 65, 344–346. doi:10.1017/jog.2019.10
Festi, D., Schwikowski, M., Maggi, V., Oeggl, K., and Jenk, T. M. (2021). Significant mass loss in the accumulation area of the Adamello glacier indicated by the chronology of a 46 m ice core. Cryosphere 15, 4135–4143. doi:10.5194/tc-15-4135-2021
Fischer, A., Stocker-Waldhuber, M., Frey, M., and Bohleber, P. (2022). Contemporary mass balance on a cold Eastern Alpine ice cap as a potential link to the Holocene climate. Sci. Rep. 12 (1331), 1–13. doi:10.1038/s41598-021-04699-2
Fröhlich, K., Kralik, M., Papesch, W., Rank, D., Scheifinger, H., and Stichler, W. (2008). Deuterium excess in precipitation of Alpine regions – moisture recycling. Isot. Environ. Health Stud. 44 (1), 1–10. doi:10.1080/10256010801887208
Gabrielli, P., Barbante, C., Bertagna, G., Bertò, M., Binder, D., Carton, A., et al. (2016). Age of the Mt. Ortles ice cores, the tyrolean iceman and glaciation of the highest summit of south tyrol since the northern Hemisphere climatic optimum. Cryosphere 10, 2779–2797. doi:10.5194/tc-10-2779-2016
Haeberli, W., Frauenfelder, R., Kӓӓb, A., and Wagner, S. (2004). Characteristics and potential climatic significance of “miniature ice caps” (crest-and cornice-type low-altitude ice archives). J. Glaciol. 50, 129–136. doi:10.3189/172756504781830330
Hoffmann, H., Preunkert, S., Legrand, M., Leinfelder, D., Bohleber, P., Friedrich, R., et al. (2018). A new sample preparation system for Micro-14C dating of glacier ice with a first application to a high Alpine ice core from Colle Gnifetti (Switzerland). Radiocarbon 60 (2), 517–533. doi:10.1017/RDC.2017.99
Huber, C. J., Salionov, D., Burgay, F., Eichler, A., Jenk, T. M., Bjelic, S., et al. (2022). Molecular reconstruction of organic aerosol composition from a firn core collected at Grand Combin, Swiss Alps. IPICS. Abstract 205. https://www.psi.ch/fr/media/64927/download?attachment 25.
Legrand, M., Preunkert, S., Schock, M., Cerqueira, M., Kasper-Giebl, A., Afonso, J., et al. (2007). Major 20th century changes of carbonaceous aerosol components (EC, WinOC, DOC, HULIS, carboxylic acids, and cellulose) derived from Alpine ice cores. J. Geophys. Res.-Atmos. 112, D23S11. doi:10.1029/2006JD008080
Patterson, W. A., Edwards, K. J., and Maguire, D. J. (1987). Microscopic charcoal as a fossil indicator of fire. Quat. Sci. Rev. 6 (1), 3–23. doi:10.1016/0277-3791(87)90012-6
Pavlova, P. A., Jenk, T. M., Schmid, P., Bogdal, C., Steinlin, C., and Schwikowski, M. (2015). Polychlorinated biphenyls in a temperate alpine glacier: 1. Effect of percolating meltwater on their distribution in glacier ice. Environ. Sci. Technol. 49 (24), 14085–14091. doi:10.1021/acs.est.5b03303
Preunkert, S., Wagenbach, D., and Legrand, M. (2003). A seasonally resolved alpine ice core record of nitrate: comparison with anthropogenic inventories and estimation of preindustrial emissions of NO in Europe. J. Geophys. Res. 108 (21), 4681. doi:10.1029/2003JD003475
Schotterer, U., Fröhlich, K., Gӓggeler, H. W., Sandjordj, S., and Stichler, W. (1997). Isotope records from Mongolian and Alpine ice cores as climate indicators. Clim. Change 36, 519–530. doi:10.1023/A:1005338427567
Schotterer, U., Stichler, W., and Ginot, P. (2004). “The influence of post-depositional effects on ice core studies: examples from the alps, andes, and altai,” in Earth paleoenvironments: records preserved in mid- and low-latitude glaciers. Developments in paleoenvironmental research. Editors J. R. Green, and L. G. Thompson (Dordrecht: Springer), 9. doi:10.1007/1-4020-2146-1_3
Schwikowski, M., Brütsch, S., Gӓggeler, H. W., and Shotterer, U. (1999b). A high-resolution air chemistry record from an Alpine ice core: fiescherhorn glacier, Swiss Alps. J. Geophys. Res. 104 (D11), 13709–13719. doi:10.1029/1998JD100112
Schwikowski, M., Döscher, A., Gӓggeler, H. W., and Schotterer, U. (1999a). Anthropogenic versus natural sources of atmospheric sulphate from an Alpine ice core. Tellus Ser. B Chem. Phys. Meteorol. 51, 938–951. doi:10.3402/tellusb.v51i5.16506
Spagnesi, A., Barbaro, E., Feltracco, M., De Blasi, F., Zannoni, D., Dreossi, G., et al. (2023). An upgraded CFA – FLC-MS/MS system for the semi-continuous detection of levoglucosan in ice cores. Talanta 265, 124799. doi:10.1016/j.talanta.2023.124799
Steinlin, C., Bogdal, C., Pavlova, P. A., Schwikowski, M., Lüthi, M. P., Scheringer, M., et al. (2015). Polychlorinated biphenyls in a temperate alpine glacier: 2. Model results of chemical fate processes. Environ. Sci. Technol. 49, 14092–14100. doi:10.1021/acs.est.5b03304
Uglietti, C., Zapf, A., Jenk, T. M., Sigl, M., Szidat, S., Salazar, G., et al. (2016). Radiocarbon dating of glacier ice: overview, optimisation, validation and potential. Cryosphere 10 (6), 3091–3105. doi:10.5194/tc-10-3091-2016
Wagenbach, D., Bohleber, P., and Preunkert, S. (2012). Cold, alpine ice bodies revisited: what may we learn from their impurity and isotope content? Geogr. Ann. 94 (2), 245–263. doi:10.1111/j.1468-0459.2012.00461.x
Keywords: ice cores, alpine glaciers, Eastern Alps, impurities, stable water isotopes, levoglucosan
Citation: Spagnesi A, Bohleber P, Barbaro E, Feltracco M, De Blasi F, Dreossi G, Stocker-Waldhuber M, Festi D, Gabrieli J, Gambaro A, Fischer A and Barbante C (2023) Preservation of chemical and isotopic signatures within the Weißseespitze millennial old ice cap (Eastern Alps), despite the ongoing ice loss. Front. Earth Sci. 11:1322411. doi: 10.3389/feart.2023.1322411
Received: 16 October 2023; Accepted: 24 November 2023;
Published: 07 December 2023.
Edited by:
Mathias Bavay, WSL Institute for Snow and Avalanche Research SLF, SwitzerlandReviewed by:
Sumito Matoba, Hokkaido University, JapanWojciech Dobinski, University of Silesia in Katowice, Poland
Copyright © 2023 Spagnesi, Bohleber, Barbaro, Feltracco, De Blasi, Dreossi, Stocker-Waldhuber, Festi, Gabrieli, Gambaro, Fischer and Barbante. This is an open-access article distributed under the terms of the Creative Commons Attribution License (CC BY). The use, distribution or reproduction in other forums is permitted, provided the original author(s) and the copyright owner(s) are credited and that the original publication in this journal is cited, in accordance with accepted academic practice. No use, distribution or reproduction is permitted which does not comply with these terms.
*Correspondence: Azzurra Spagnesi, YXp6dXJyYS5zcGFnbmVzaUB1bml2ZS5pdA==