- 1Lille College, Hohai Univesity, Nanjing, China
- 2Key Laboratory of Ministry of Education for Geomechanics and Embankment Engineering, Hohai University, Nanjing, China
- 3Research Institute of Geotechnical Engineering, Hohai University, Nanjing, Jiangsu, China
Generally, the soil requires to be compacted in highway construction. The expansive soil is a special type of soil that is highly susceptible to variations in water content, which affects the degree of compaction at the same compaction energy. In the present study, a series of wet compaction tests and dry compaction tests were carried out in the laboratory. Laboratory test results show that dry compaction will produce a higher optimum water content and a higher maximum dry unit weight compared to wet compaction, because its matric suction is smaller. Field compaction tests were also conducted, the results showed that there might be a risk of under-compacting soils during construction caused by different water content change path in actual field conditions.
1 Introduction
Soil dams and road embankments are important infrastructure projects that require the use of soils with specific properties. In order to ensure that these structures are stable and durable, it is necessary to test the soil’s maximum density and optimum water content. The laboratory proctor compaction test is a commonly method for this purpose (Gurtug and Sridharan, 2002; Zhao et al., 2008; Hirebelaguly Shivaprakash and Sridharan, 2021). However, there are many factors that can affect the test result. For example, the size and shape of the mould used in specimen preparation can have an impact on the maximum dry unit weight and optimum water content (Qian et al., 2020). Similarly, compaction effort, soil temperature, fines content, and other variables can also influence these parameters (Lachgueur et al., 2021; Zvonarić et al., 2021). One of the factors that has not been extensively studied is the effect of water content change path during specimen preparation. Specifically, it is unclear whether drying or adding water to reach a target water content could have an impact on the results of proctor compaction tests. Further research in this area could help engineers better understand how different methods of specimen preparation may affect soil properties relevant to construction projects like soil dams and road embankments. By identifying best practices for testing soils under various conditions, we can improve our ability to design safe and effective infrastructure projects that meet society’s needs for transportation networks and flood control measures.
Expansive soil, which is a unique type of soil that undergoes significant volume changes in response to variations in water content (Zhang et al., 2020; Yao et al., 2021; Zhang et al., 2022), has led to significant economic losses (Salahudeen et al., 2014). Lime stabilizer is widely used to improve the physical-chemical qualities of expansive soils in engineering projects for the advantages of easy field mixing procedures. When lime is combined with water, the dissolved calcium ions and hydroxide ions react with expansive clay minerals such as montmorillonite and kaolinite, the reaction products subsequently bond soil particles through chemical cementation, thereby enhancing soil strength (Nalbantoglu and Tuncer, 2001; Al-Rawas et al., 2005). In addition, the calcium ions reduce repulsive force between soil particles, if the attraction is in excess of repulsion, the particles tend to aggregate and flocculation, consequently the plasticity decrease (Hilt and Davidson, 1960; Sridharan, 1985; Khattab et al., 2007).
The target water content is crucial for ensuring the quality of filed compaction since it significantly affects physical and mechanical properties of unsaturated soils (Li et al., 2021; Gaspar et al., 2022). For example, during evaporation, the pore water pressure decreases with the increase curvature of the air–water interface, consequently the matric suction increases. Moreover, the reduction in water content leads to higher concentration of soluble ions and suspension-colloidal, the concentration can influence the attachment-detachment process of these substances and the tensile strength of aggregates (Dexter and Chant, 1991; Bai et al., 2019) which are the basic units of soil structure (Ye et al., 2017). In terms of macroscopic mechanical properties, matric suction is closely correlated with the shear strength of the soil. The relationship between matric suction and water content is described using the soil-water characteristic curve (SWCC), and scholars such as Bishop has established connections between matric suction and the strength of unsaturated soils (Kholghifard, 2020; Zhao et al., 2021). Several factors have been demonstrated to affect the SWCC, Gallage and Uchimura (2010) indicated that soils with low density exhibit lower residual suction compared to those with high density, while the influence of soil structure on SWCC can be negligible in the high suction range (Baker and Frydman, 2009). It is further found that the matric suction decreases with increase OCR(Vanapalli et al., 1999). For lime stabilized expansive soil, the amount of stabilizer added is a factor, the addition of stabilizers alters the thickness of the diffuse double layer (Sridharan et al., 1986), and the formation of aggregates by some particles changes the microstructure of the soil (Khattab et al., 2007).
In order to enhance the efficient utilization of compaction energy, it is essential to ensure that the water content of soils is near its optimum water content during field compaction. Two methods are commonly used to reach the optimum water content according to ASTM standard D698-12 (Mather and Etris, 2013): air-drying or adding water. However, in SWCC, significant differences exist between the curves in the drying (increased matric suction) and wetting (decreased matric suction) phases (Qi et al., 2019; Yao et al., 2021). These phenomena implying that besides water content magnitude, the change path of water content variation also influences the mechanical properties of the soil and the compaction effect (Zeng et al., 2021; Li et al., 2022).
However, in the field, due to the lack of the knowledge of the differences between the dry compaction and the wet compaction, the dry compaction testing results from the laboratory, which are more familiar to engineers, and often used as the construction quality controlling standard for both type of compaction work (Jamsawang et al., 2017). Apparently, errors may be induced if the wet compaction testing results from laboratory are used as the construction quality control standard for the dry compaction in the field. In this paper, to understand the effect of the water content change path, the results of dry compaction tests and wet compaction tests from the laboratory were analyzed, and results were compared with the field rolling compaction data in the Huai’an-Nanjing Highway project in China.
2 Material and methods
2.1 Specimen preparation
Field expansive soils were collected from No.6 and No.7 borrow pits in the Huai’an-Nanjing Highway project in China. The soil was crushed and screened, and the basic physical property tests were carried out. It can be seen in Figure 1. Particle size distribution of the soil samples that the proportion is 36.1% in the range of 0.075 mm–0.005 mm and 63% for the particle size smaller than 0.005 mm. The liquid limit of the sample is 47.0%, the plastic limit is 19.1%, and the plasticity index is 28, one-dimensional swell test was conducted according to ASTM D4546. The basic parameters of expansive soil are shown in Table 1.
The initial water content of the soil in these borrow pits were high due to a high groundwater level, and the soils were mostly expansive clay. This kind of soil collected directly from the site does not meet the requirements of the soil material for the indoor compaction test, so the unused soil collected from the site needs to be treated (Lu et al., 2020; Okeke, 2020). Lime was added to improve the field expansive soil. In laboratory compaction test, the content of lime was 5% to keep it consistent with the field test. Nalbantoglu and Tuncer (2001) mixed expansive soil with 3%, 5%, and 7% lime, and the results stated that when the lime content is higher than 3%, the swelling potential was effectively reduced.
In this paper, we took two steps to add lime: (1) 2% lime (2% weight of dry soil) was added, and mixed. The soil mixture was placed into a large plastic bucket to cure and was mixed once per day for 3 days. (2) On the first day after the first step, the soil mixture was poured out from the bucket and mixed evenly. Then, the slaked 3% lime (3% weight of dry field soil) was mixed evenly with the soil mixture, and Kumar et al. (2007) stated that time of curing for lime soil mixtures did not increase very much strength, so the mixture was only cured for 24 h.
2.2 Laboratory compaction test
Relevant studies indicate that there is minimal difference between the optimum water content and maximum dry density obtained from the modified Proctor test and those from the standard Proctor test (Shaivan and Sridharan, 2020). However, in case of the unique characteristics of the soil samples employed in this study, the standard Proctor test was conducted in this paper in order to avoid introducing unnecessary errors. The standard proctor compaction test apparatus is shown in Figure 2. In the dry compaction test, the soil mixtures from both borrow pits were air-dried until the water content was smaller than 10%, and then soil mixture was sieved with a sieve with 5 mm openings. Then, 7 specimens for each mixture from No. 6 and No. 7 borrow pits with different water contents were prepared by adding different amount of water in each specimen, ensuring that there were at least 2 specimens wetter and 2 specimens drier than the soil with the optimum water content. The water content increment between two specimens was about 2%, with indoor air-drying within 1 day. Water contents were individually in the ranges of 21–23%, 19–21%, 17–19%, 15–17%, 13–15%, 11–13%, 9–11%. In the wet compaction test, after being mixed with lime, 6 specimens were prepared for each soil from No. 6 and No. 7 borrow pits individually by gradually air-drying to make sure that they had a 2% water content reduction between two individual specimens. After achieving the target water content, the samples are then placed into molds and compacted to ensure that each sample has the same dry density before testing. Tests were performed following the ASTM D698–12 standard.
2.3 Soil water character analysis
The water content significantly affects the suction of unsaturated soils, thus soil water characteristic analysis was conducted to evaluate the influence of matric suction on compaction tests. The soil water characteristic curves were measured for the soil specimens from No. 7 borrow pit for both the drying and the wetting processes using pressure membrane apparatus. The soil with 8% lime was also measured in order to evaluate the effect of lime content on matric suction. Dew point potential meter was used to measure matric suction. The test steps were as follows:
(1) To obtain the soil water characteristic curve using the air-drying method, an appropriate amount of air-dried soil was saturated with water and allowed to stand for 4–5 h. The saturated soil was then transferred to a stainless steel sample cup (with a diameter of 4 cm and height of 1 cm) in a uniform layer at the bottom of the cup. The soil was continuously mixed using a spoon to ensure uniform moisture conditions for all soil samples. When the water content approaches the predetermined value through natural evaporation (at room temperature of 25°C), the matric suction was measured, and the mass of the sample at that was measured using a balance. The measurements were repeated at the same time intervals until the water content becomes extremely low. The experiment is stopped at this point.
(2) To obtain the soil water characteristic curve using the wetting method, air-dried soil and place was took in a stainless steel sample cup, evenly spread at the bottom, then using a small spray bottle to apply a certain amount of distilled water to the soil and mix it continuously with a spoon to achieve the desired soil water content. Measured the soil water potential and soil mass using a dew point water potential meter and a balance, respectively, at the specific water content. Repeat the process with different water contents to obtain multiple data points and plot the data points to create the soil water characteristic curve, representing the relationship between matric suction and water content during the wetting process.
Two set of specimens were tested with lime contents of 5% and 8% to confirm the data trend reliability for specimens with different lime content.
3 Results and discussion
3.1 Effect of water content change path
Both the dry and wet compaction testing results for the soils from No. 6 and No. 7 borrow pits are shown in Figure 3. It can be found that the dry compaction curves are above the wet compaction curves for soils from both No.6 and No. 7 borrow pits. For pit 6 soil, the maximum dry density of dry compaction test is 1.69 g/cm3, the maximum dry density of wet compaction test is 1.613 g/cm3, for pit 7 soil, the maximum dry density of dry compaction test is 1.72 g/cm3, and the maximum dry density of wet compaction test is 1.65 g/cm3. It means that the maximum dry density from dry compaction tests are higher than those from wet compaction tests.
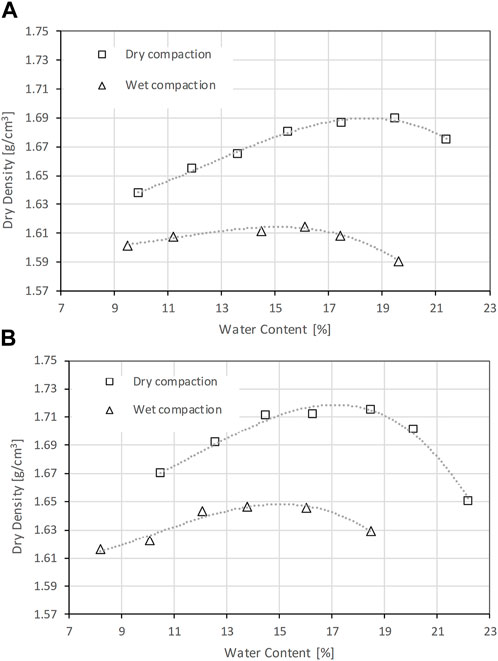
FIGURE 3. Laboratory compaction test results: (A) soil collected from the No.6 borrow pit; (B) soil collected from the No.7 borrow pit.
For the soil of pit 6, the optimum water content for the dry compaction test is 19% and the optimum water content for the wet compaction test is 15%. For the soil of pit 7, the optimum water content for the dry compaction test is 17% and the optimum water content for the wet compaction test is 15%. Hence, we can draw conclusions that the optimum water content of dry compaction tests is relatively high compared to wet compaction tests.
Given that all the compaction energy in each test is the same, these differences might be due to the different amount of energy needed to change the soil fabric. For the soil specimens from the same borrow pit, the only difference would be in the soil specimen preparation (drying and wetting), associated with great matric suction change. The determined soil water characteristic curves are shown in Figure 4.
Some scholars have measured the moisture characteristic curve of clay in two different processes of wetting and drying found that under the same water content, the matric suction in the drying process is always greater than that in the wetting process (Kholghifard, 2020; Ding et al., 2022; Sarker and Wang, 2022). Figure 4 shows that the drying curves are above the wetting curves for specimens with both 5% lime and 8% lime contents. It reveals that with the same water content, the matric suction on the drying curve is higher than that on the wetting curve. This phenomenon is caused by “ink-bottle” effect and “rain-drop” effect caused by the non-homogenous pore size distribution and difference between contact angle advancing interface and receding interface, respectively (Zhai et al., 2020). On the other hand, it can be seen from Figure 4 that the matric suction increases with increase limes content, this is because the cations provided by the dissolved limes decrease the repulsive force between diffuse double layers, thereby the matric suction increases (Liu et al., 2009).
In the process of compaction energy transfer, a portion of energy is dissipated by generating irreversible deformation of soil skeleton and the remaining part is dissipated through mechanisms such as viscous dissipation (Bai et al., 2021). The interaction forces between particles, comprising normal and tangential contact forces can indicate whether the soil skeleton is at a critical state for generating irreversible deformation. The normal contact forces can be expressed in terms of matric suction according to the principle of effective stress.
where
Coulomb’s Law of Friction is widely used to calculate friction
It can be inferred from Eqs 1 and 2 that for specimens with the same effective stress and strength parameters, the shear strength is a linear function of matric suction. From Figure 4, it can be concluded that the strength of the wet compaction specimens was greater than that of the dry compaction specimens with the same water content. Thus, with the same compaction energy, the amount of volume change due to compaction in wet compaction tests should be smaller than that in dry compaction test, and therefore, the maximum dry density of wet compaction samples should be smaller than that of dry compaction samples as that shown in Figure 3.
3.2 Field test validation
Standard proctor compaction test generated weaker vibrations than those produced during compaction by a vibratory roller. Zvonarić et al. (2021) preformed a series of standard proctor tests and vibratory hammer tests and pointed out that significant differences in results may exist between the two methods for some soil types. Additionally, the boundary conditions of laboratory tests and field tests are not entirely consistent. Therefore, in order to enhance the reliability of laboratory compaction test results and depict the influence of water content change paths on compaction effects, several field compaction tests were conducted.
In the field tests, 5% limes were mixed with expansive soil by experts’ recommendations, the subgrade fill was continually compacted until the degree of compaction (defined as the ratio of the dry unit weight of compacted soil to the maximum dry unit weight) remained unchanged in order to determine the relationship between the compaction times and the degree of compaction. Because the natural water content was really high in the field testing site, the fill material was dried after each compaction which was similar to what happened in the laboratory wet compacting tests. Different compaction energy input will significantly affect the compaction results in the field (Gurtug and Sridharan, 2002), so the same set of compaction equipment is used in one set of tests.
Two field compaction methods were compared to analyze the effect of compaction method. With the compaction method No.1, the subgrade fill was compacted 4 times with Vibratory roller XS190, before being compacted with the Macadam roller 3Y 18/21 until the degree of compaction remained unchanged. With the compaction method No.2, the subgrade fill was compacted twice with Vibratory roller XS190, before being compacted with Macadam roller 3Y 18/21 until the degree of compaction remained unchanged.
Dynamic stress during the compaction process was monitored using soil pressure gauge, with measurement points placed at depths of 0.3, 0.6, 1.2, and 2.4 m from the subgrade surface. PauTa criterion which is a popular method to eliminate outlier data points typically requires a sufficient amount of information. However, due to the limited quantity of recorded data, the Dixon criterion was utilized in this paper to the discard outlier data points following the method outlined below.
where
As shown in Figure 5, the dynamic stress during the compaction process continuously decreases along the depth direction, with a peak value of 221.5 kPa. It can be seen that the compaction primarily affecting the soil layers within a thickness of 1 m.
The field test results are presented in Figure 6, where the degree of compaction using various field compaction methods is assessed against both the dry compaction and wet compaction test data obtained from laboratory tests.
From the results of the field rolling compaction in Figure 6, it can be seen that the degree of compaction of the wet compaction test is greater than that of the dry compaction test whether method 1 or method 2 is adopted. And the compaction degree of the second compaction method is greater than that of the first compaction method in the dry compaction test and the wet compaction test. The overall compaction law is manifested that the degree of compaction reached the peak value after the fourth rolling compaction with Macadam roller. However, after the peak value, the degree of compaction fell down with continuing compaction. For method 2, the compaction gradually stabilizes after the 6th compaction, but for Method 1, the compaction increases to a certain extent after the 6th compaction. The reason for the analysis may be because for method 1the subgrade fill was compacted 4 times with Vibratory roller XS190, but the method 2 subgrade fill was compacted 2 times with Vibratory roller XS190. Meanwhile, there were “peeling” and “cracking” on the soil surface after the fifth rolling compaction. The soil in the compaction area forms a conical core in which the soil particles undergo vertical compression, while shear band forms around the conical core and moves upward to the shallow soil due to the wedging effect (Jia et al., 2018). This implies that the “peeling” and “cracking” phenomenon are attributed to shear failure on the surface soil. It can also be concluded that the degrees of compaction in the field tests with method No.1 are lower than those in the field tests with method No.2 in which less Vibratory roller compaction was applied.
According to the above analysis, it can be inferred that the degrees of compaction calculated with the dry compaction laboratory test data are smaller than those with the wet compaction laboratory test data. Therefore, there might be a risk of under-compacting soils during construction caused by different moisture conditions in actual field conditions. Under-compacted soils can lead to several issues such as settlement problems, reduced load-bearing capacity, and decreased stability over time. Therefore, it becomes essential for engineers and contractors involved in construction projects to carefully consider these variations when determining appropriate levels of effort required for achieving target degrees of soil compactions. In conclusion, understanding how different testing methods impact measured degrees of soil compactness is vital for effective quality control management in construction projects.
4 Conclusion
The dry compaction laboratory tests and wet compaction laboratory tests are both performed and compared to investigate the effect of water content change path on compaction results.
Field tests were performed to show the effect of water content change path on the field compaction quality control. With the testing results, the following conclusions can be drawn:
(1) The maximum dry density and optimum water content from wet compaction tests are smaller than that from dry compaction tests with the same compaction energy due to the hysteresis behavior of unsaturated soil.
(2) At the same water content the matric suction in the dry compaction test is smaller than that in the wet compaction test regardless of the lime contents.
(3) The field tests show a peak value of the degree of compaction, corresponding to the “peeling” and “cracking” on the soil surface during construction, indicating the destruction of soil fabric with continuous compaction effort after the peak.
Therefore, with all the results and conclusions above, some recommendations can be made. If the initial water content of the filling material is higher than the objective water content during the construction, the water content may be reduced gradually during the compaction. This water content change process is consistent with the laboratory wet compaction test process. The wet compaction testing results should be used as field compaction quality control standards in this situation. If the initial water content is lower than the objective water content, the water content may be increased gradually during the construction. The water content change process is consistent with that in the laboratory dry compaction tests. Therefore, the dry compaction testing results should be used as field compaction quality control standards in this case.
Data availability statement
The original contributions presented in the study are included in the article/Supplementary material, further inquiries can be directed to the corresponding author.
Author contributions
YG: Data curation, Formal Analysis, Investigation, Writing–original draft, Writing–review and editing, Conceptualization. FZ: Conceptualization, Investigation, Methodology, Validation, Writing–original draft. ZL: Data curation, Formal Analysis, Investigation, Supervision, Writing–review and editing. QM: Conceptualization, Formal Analysis, Investigation, Methodology, Validation, Writing–review and editing.
Funding
The author(s) declare financial support was received for the research, authorship, and/or publication of this article. Financial support from the National Natural Science Foundation of China (NSFC No. 51778211), Natural Science Foundation of the Higher Education Institutions of Jiangsu Province, China (No. BK20171434).
Conflict of interest
The authors declare that the research was conducted in the absence of any commercial or financial relationships that could be construed as a potential conflict of interest.
Publisher’s note
All claims expressed in this article are solely those of the authors and do not necessarily represent those of their affiliated organizations, or those of the publisher, the editors and the reviewers. Any product that may be evaluated in this article, or claim that may be made by its manufacturer, is not guaranteed or endorsed by the publisher.
References
Al-Rawas, A. A., Hago, A., and Al-Sarmi, H. (2005). Effect of lime, cement and Sarooj (artificial pozzolan) on the swelling potential of an expansive soil from Oman. Build. Environ. 40 (5), 681–687. doi:10.1016/j.buildenv.2004.08.028
Bai, B., Rao, D., Chang, T., and Guo, Z. (2019). A nonlinear attachment-detachment model with adsorption hysteresis for suspension-colloidal transport in porous media. J. Hydrology 578, 124080. doi:10.1016/j.jhydrol.2019.124080
Bai, B., Zhou, R., Cai, G., Hu, W., and Yang, G. (2021). Coupled thermo-hydro-mechanical mechanism in view of the soil particle rearrangement of granular thermodynamics. Comput. Geotechnics 137, 104272. doi:10.1016/j.compgeo.2021.104272
Baker, R., and Frydman, S. (2009). Unsaturated soil mechanics: critical review of physical foundations. Eng. Geol. 106 (1-2), 26–39. doi:10.1016/j.enggeo.2009.02.010
Dexter, A., and Chant, K. (1991). Soil mechanical properties as influenced by exchangeable cations. J. Soil Sci. 42 (2), 219–226. doi:10.1111/j.1365-2389.1991.tb00403.x
Ding, X., Ma, L., Zhang, R., Li, J., Lin, W., and Jin, Y. (2022). Experimental study on microstructure and soil-water characteristic curve of unsaturated remolded weak expansive soil. J. Cent. South Univ.(Sci. Technol.) 53, 350–360. doi:10.11817/j.issn.1672-7207.2022.01.029
Gallage, C. P. K., and Uchimura, T. (2010). Effects of dry density and grain size distribution on soil-water characteristic curves of sandy soils. Soils Found. 50 (1), 161–172. doi:10.3208/sandf.50.161
Gaspar, T. A. V., Jacobsz, S. W., Heymann, G., Toll, D., Gens, A., and Osman, A. (2022). The mechanical properties of a high plasticity expansive clay. Eng. Geol. 303, 106647. doi:10.1016/j.enggeo.2022.106647
Gurtug, Y., and Sridharan, A. (2002). Prediction of compaction characteristics of fine-grained soils. Géotechnique 52 (10), 761–763. doi:10.1680/geot.2002.52.10.761
Hirebelaguly Shivaprakash, S., and Sridharan, A. (2021). Correlation of compaction characteristics of standard and reduced Proctor tests. Proc. Institution Civ. Engineers-Geotechnical Eng. 174 (2), 170–180. doi:10.1680/jgeen.20.00060
Jamsawang, P., Nuansrithong, N., Voottipruex, P., Songpiriyakij, S., and Jongpradist, P. (2017). Laboratory investigations on the swelling behavior of composite expansive clays stabilized with shallow and deep clay-cement mixing methods. Appl. Clay Sci. 148, 83–94. doi:10.1016/j.clay.2017.08.013
Jia, M., Yang, Y., Liu, B., and Wu, S. (2018). PFC/FLAC coupled simulation of dynamic compaction in granular soils. Granul. Matter 20, 76–15. doi:10.1007/s10035-018-0841-y
Khattab, S., Al-Mukhtar, M., and Fleureau, J.-M. (2007). Long-term stability characteristics of a lime-treated plastic soil. J. Mater. Civ. Eng. 19 (4), 358–366. doi:10.1061/(asce)0899-1561(2007)19:4(358)
Kholghifard, M. (2020). Effective stress and compressibility of unsaturated clayey soil under drying and wetting cycles. Period. Polytech. Civ. Eng. 64 (4), 999–1006. doi:10.3311/ppci.16166
Kumar, A., Walia, B. S., and Bajaj, A. (2007). Influence of fly ash, lime, and polyester fibers on compaction and strength properties of expansive soil. J. Mater. Civ. Eng. 19 (3), 242–248. doi:10.1061/(asce)0899-1561(2007)19:3(242)
Lachgueur, K., Abou-Bekr, N., Taibi, S., and Fleureau, J.-M. (2021). Effects of compaction and suction on the hydromechanical properties of a dam core clay. Transp. Geotech. 27, 100498. doi:10.1016/j.trgeo.2020.100498
Li, J., Xu, H., Chen, L., Li, B., Liang, D., Ren, S., et al. (2022). Effect of saturation degree on mechanical behaviors of shallow unsaturated expansive soils. Sustainability 14 (21), 14617. doi:10.3390/su142114617
Li, K., Nowamooz, H., Chazallon, C., and Migualt, B. (2021). Mechanical behaviour of densely compacted expansive soils during wetting and drying cycles: an analytical model based on shakedown concept. Eur. J. Environ. Civ. Eng. 25 (6), 1065–1079. doi:10.1080/19648189.2019.1568307
Liu, L., Moreno, L., and Neretnieks, I. (2009). A dynamic force balance model for colloidal expansion and its DLVO-based application. Langmuir 25 (2), 679–687. doi:10.1021/la8026573
Lu, Z., Zhao, Y., Xian, S., and Yao, H. (2020). Experimental study on dynamic resilient modulus of lime-treated expansive soil. Adv. Mater. Sci. Eng. 2020, 1–10. doi:10.1155/2020/3272681
Mather, B., and Etris, S. F. (2013). American society for testing and materials. Germany, Springer US. doi:10.1007/0-387-30720-6_5
Nalbantoglu, Z., and Tuncer, E. R. (2001). Compressibility and hydraulic conductivity of a chemically treated expansive clay. Can. Geotechnical J. 38 (1), 154–160. doi:10.1139/t00-076
Okeke, C. A. (2020). Engineering behaviour of lime-and waste ceramic dust-stabilized expansive soil under continuous leaching. Bull. Eng. Geol. Environ. 79 (4), 2169–2185. doi:10.1007/s10064-019-01648-2
Popova, E., and Popov, V. L. (2015). The research works of Coulomb and Amontons and generalized laws of friction. Friction 3, 183–190. doi:10.1007/s40544-015-0074-6
Qi, S., Vanapalli, S. K., Yang, X.-g., and Zhou, J.-w. (2019). Stability analysis of an unsaturated expansive soil slope subjected to rainfall infiltration. Geomechanics Eng. 19 (1), 1–9. doi:10.12989/gae.2019.19.1.001
Qian, G., Hu, K., Li, J., Bai, X., and Li, N. (2020). Compaction process tracking for asphalt mixture using discrete element method. Constr. Build. Mater. 235, 117478. doi:10.1016/j.conbuildmat.2019.117478
Salahudeen, A., Eberemu, A. O., and Osinubi, K. J. (2014). Assessment of cement kiln dust-treated expansive soil for the construction of flexible pavements. Geotechnical Geol. Eng. 32, 923–931. doi:10.1007/s10706-014-9769-0
Sarker, D., and Wang, J. X. (2022). Experimental study on soil–water retention properties of compacted expansive clay. Adv. Transp. Geotechnics IV Proc. 4th Int. Conf. Transp. Geotechnics 3, 433–445. doi:10.1007/978-3-030-77238-3_33
Shaivan, H., and Sridharan, A. (2020). Comparison of reduced modified proctor vs modified proctor. Geotechnical Geol. Eng. 38, 6891–6897. doi:10.1007/s10706-020-01405-3
Sridharan, A. (1985). Free swell index of soils, a need for redefinition. Indian Geotechnical J. 15 (2), 94–99.
Sridharan, A., Rao, S. M., and Murthy, N. S. (1986). A rapid method to identify clay type in soils by the free-swell technique. Geotechnical Test. J. 9 (4), 198–203. doi:10.1520/gtj10630j
Vanapalli, S. K., Fredlund, D. G., and Pufahl, D. E. (1999). The influence of soil structure and stress history on the soil–water characteristics of a compacted till. Géotechnique 49 (2), 143–159. doi:10.1680/geot.1999.49.2.143
Yao, Y., Li, J., Xiao, Z., and Xiao, H. (2021). Soil-Water characteristics and creep deformation of unsaturated expansive subgrade soil: experimental test and simulation. Front. Earth Sci. 9, 783273. doi:10.3389/feart.2021.783273
Ye, C., Guo, Z., Cai, C., Wang, J., and Deng, J. (2017). Effect of water content, bulk density, and aggregate size on mechanical characteristics of Aquults soil blocks and aggregates from subtropical China. J. soils sediments 17, 210–219. doi:10.1007/s11368-016-1480-8
Zeng, L., Yu, H.-C., Liu, J., Gao, Q.-F., and Bian, H.-B. (2021). Mechanical behaviour of disintegrated carbonaceous mudstone under stress and cyclic drying/wetting. Constr. Build. Mater. 282, 122656. doi:10.1016/j.conbuildmat.2021.122656
Zhai, Q., Rahardjo, H., Satyanaga, A., Dai, G., and Du, Y. (2020). Estimation of the wetting scanning curves for sandy soils. Eng. Geol. 272, 105635. doi:10.1016/j.enggeo.2020.105635
Zhang, J., Niu, G., Li, X., and Sun, D. a. (2020). Hydro-mechanical behavior of expansive soils with different dry densities over a wide suction range. Acta Geotech. 15, 265–278. doi:10.1007/s11440-019-00874-y
Zhang, R., Liu, Z., Zheng, J., and Lei, G. (2022). Utilisation of expansive soils as highway embankment materials in humid environments. Int. J. Pavement Eng. 23 (7), 2176–2190. doi:10.1080/10298436.2020.1849685
Zhao, G.-t., Zou, W.-l., Han, Z., Wang, D.-x., and Wang, X.-q. (2021). Evolution of soil-water and shrinkage characteristics of an expansive clay during freeze-thaw and drying-wetting cycles. Cold Regions Sci. Technol. 186, 103275. doi:10.1016/j.coldregions.2021.103275
Zhao, Y., Krzic, M., Bulmer, C. E., and Schmidt, M. G. (2008). Maximum bulk density of British Columbia forest soils from the proctor test: relationships with selected physical and chemical properties. Soil Sci. Soc. Am. J. 72 (2), 442–452. doi:10.2136/sssaj2007.0075
Keywords: dry compaction test, wet compaction test, maximum dry density, optimum water content, matric suction
Citation: Guo Y, Zhang F, Liu Z and Meng Q (2023) Effects of water content change path on laboratory and field compaction of lime stabilized expansive soil. Front. Earth Sci. 11:1282044. doi: 10.3389/feart.2023.1282044
Received: 23 August 2023; Accepted: 20 November 2023;
Published: 29 December 2023.
Edited by:
Zhuo Chen, Sichuan Agricultural University, ChinaReviewed by:
Sujatha Evangelin Ramani, SASTRA University, IndiaBing Bai, Beijing Jiaotong University, China
Zhi Ding, Hangzhou City University, China
Copyright © 2023 Guo, Zhang, Liu and Meng. This is an open-access article distributed under the terms of the Creative Commons Attribution License (CC BY). The use, distribution or reproduction in other forums is permitted, provided the original author(s) and the copyright owner(s) are credited and that the original publication in this journal is cited, in accordance with accepted academic practice. No use, distribution or reproduction is permitted which does not comply with these terms.
*Correspondence: Fuhai Zhang, fhzhang@hhu.edu.cn