- 1School of Mechanics and Civil Engineering, China University of Mining and Technology, Beijing, China
- 2China Building Technique Group Co., Ltd., Beijing, China
- 3Beijing University of Civil Engineering and Architecture, Beijing, China
- 4North Blasting Technology Co., Ltd., Beijing, China
The mining of open pit can easily cause geological disasters such as landslide and debris flow. It is widely acknowledged that communication technology could solve the existing problems in engineering practice from the aspects of disaster monitoring, deformation monitoring, landslide warning and emergency communication. This research paper introduces a fully integrated monitoring and early warning solution tailored for mining regions. By synergizing 6G and Global Navigation Satellite System (GNSS) technologies, the system effectively addresses signal transmission challenges in complex environments. The utilization of 6G’s high-speed, low-latency, and extensive connectivity capabilities enables efficient communication in these settings. The study focuses on a specific open-pit mine located in a cold region of China, utilizing it as a case study to demonstrate the system’s effectiveness in enhancing slope safety through comprehensive monitoring and early warning mechanisms. Apart from considering traditional dump characteristics and external factors, the system also introduces an innovative early warning index for detecting slope changes. Applying the Analytic Hierarchy Process (AHP) and Fuzzy comprehensive evaluation method ensures reliable evaluation results, facilitating slope assessment, monitoring, and early warning procedures in water-rich open-pit mines situated in cold areas.
1 Introduction
6G communication technology has garnered significant attention in the engineering industry due to its remarkable advantages in key technologies and performance, setting it apart from other communication technologies. Addressing existing challenges in engineering practice, such as disaster monitoring, deformation monitoring, landslide warning, and emergency communication, can be effectively achieved through the adoption of this new generation communication technology. The “14th Five-Year Plan for Mine Safety Production” in China emphasizes the prioritization of deepening “Internet + supervision and regulation” to enhance mine safety production. This supervisory approach encompasses slope stability evaluation, slope monitoring, early warning, and related aspects.
One prominent application scenario of 6G communication technology lies in slope disaster monitoring, early warning, and safety assessment. Globally, geological disasters, including landslides, have become a significant concern (Lavigne et al., 2014; Anua´rio Brasileiro de Desastres Naturais, 2012; Guadagno et al., 2001; Jafari et al., 2013; Merry et al., 2005). The demand for coal resources often leads to long-term and intensive mining practices in coal mining areas, involving large-scale operations across multiple working surfaces. Consequently, the geological environment in these mining regions suffers varying degrees of damage, resulting in occasional geological disasters (Liu et al., 2023; Zhang et al., 2023). Open-pit coal mining, in particular, can lead to large-scale mines affected by interactions between surface water and groundwater. Combined with geological structure changes and the nature of slope rock mass, these factors increase the likelihood of collapse, landslides, debris flow, and other geological disasters (Wang et al., 2021; Jiang et al., 2023). Such occurrences not only severely impact the normal production and livelihood of residents in the vicinity of mining areas but also cause economic losses while posing threats to the surrounding geological and ecological environment.
So as to better reflect the stability and safety of slopes, more and more attention has been paid to the monitoring and warning systems of slopes. Especially with the development and progress of science and technology, the main monitoring of geological disasters such as landslides has been inferred from manual observation of surface change characteristics and surrounding landform changes (Xia and Zhu, 1999; Chen, 2022; Liu and Li, 2023a; Liu et al., 2023) to some measuring tools such as total station GPS mobile phone monitoring and InSAR technology, and now it is being combined with 6G communication technology (Wang, 2020; Liu et al., 2021; Yuan and Guo, 2021; Zhang et al., 2021; Zhou, 2022). Compared with other communication technologies, 6G communication technology has great advantages in key technologies and performance, especially in the application of intelligent industrial systems. For example, 6G communication technology has significant advantages in natural disaster warning, 6G wireless communication system architecture, construction high-precision positioning, and application services based on 6G communication systems, remote control of equipment, and construction safety monitoring information collection (Ma, 2020). The effective dynamic monitoring of a mining area and timely acquisition and analysis of the mining area surface settlement law can provide a strong basis for reasonable development and planning management of the mining area in the later period (Xia et al., 2001). Based on the characteristics of mining areas, through the application of 6G communication technology, we can easily solve the existing problems in engineering practices from the aspects of disaster monitoring, deformation monitoring, landslide warning, emergency communication, and so on.
At present, landslide monitoring mainly uses displacement and deformation as early warning indicators, and the effect is not ideal for sudden and strong rock landslide early warning. Infrasound in fixed frequency bands are generated by the damage and structural changes of rock and soil in a landslide before the instability failure, which makes landslide monitoring and warning technology based on infrasound possible (in Figure 1) (Hu and Shulin, 2002; Yin et al., 2021; Wang et al., 2022; Xiong et al., 2023).
The emergency communication technology and systems for mine disaster rescue scenarios generally adopt Wi-Fi as the relay and coverage scheme. Due to the legal restrictions of continuous channel occupancy time and the legal requirements of pre-access monitoring in unauthorized frequency bands, it is difficult to achieve a communication index with extremely low delay. Simultaneous relay networking of multiple emergency communication devices in the same frequency band has the same frequency interference, which will affect the communication quality. For public safety and vehicle networking application scenarios, 6G technology defines Sidelink direct link communication technology, which can achieve low time delay and high reliability direct communication between terminal devices (Wu and Tu, 2002). Aiming at the technical requirements of mine emergency communication systems for low delay and high reliable communication, a mine emergency communication system architecture based on 6G direct communication is proposed, and a mapping method of equipment identification and time-frequency resource location of the direct communication link is designed (Figure 2). The device transmits resource locations through pre-configured forward and back links, and can communicate with adjacent front-end and back-end devices without establishing routing connections. The device uses time-domain resource cyclic multiplexing to realize resource space division multiplexing and same-frequency interference avoidance between neighboring relay devices. The proposed method can realize the relay network mechanism without scheduling permission and minimal routing to avoid the same-frequency interference under the space division multiplexing of transmission resources, effectively improve the utilization efficiency of transmission resources, and the average air interface delay under conventional baseband parameters and network configuration can reach 25 ms, significantly reducing the transmission delay.
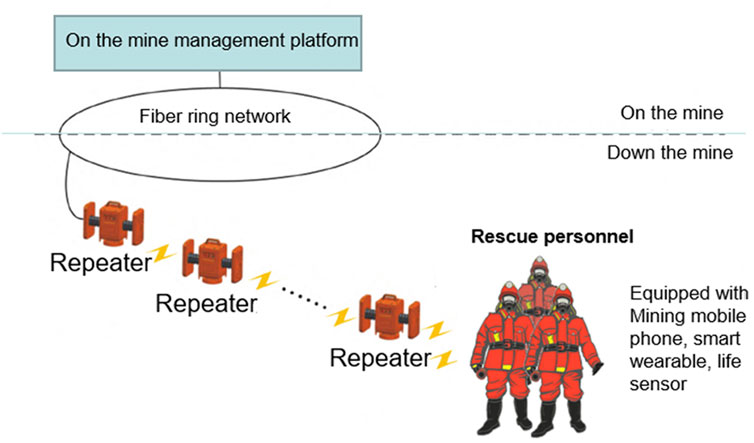
FIGURE 2. Structure diagram of the mine emergency communication system based on 6G direct communication.
In this study, 6G transmission technology is applied to the field of open mine safety, and high-speed and low-delay data transmission and communication are realized. Through 6G technology, real-time monitoring and data transmission of various locations in mining areas can be realized, improving the efficiency and accuracy of mine safety monitoring. The research team has developed a set of intelligent assessment and monitoring systems to conduct the intelligent assessment and monitoring of mine safety through comprehensive analysis of real-time data and monitoring results of the mine. The system can detect abnormal situations quickly and give early warning in time, which improves the level of mine safety management.
The eastern part of the Inner Mongolia Autonomous Region, located in an extremely cold region, has the largest number of open-pit coal mines and the largest output in China. The slope stability of open-pit mines in this region is greatly affected by water because the precipitation is very concentrated in summer and winter, and the slopes are also hit by meltwater in spring, which can easily cause flooding. Therefore, wise evaluation, monitoring, and early warning are urgently needed regarding the slope stability of open-pit mines. Taking the Dongming open-pit mine as an example in the first section, this paper constructs the stability evaluation–monitoring–early-warning system of the inner dump. The second part introduces the geometeorological and hydrogeological conditions of the Dongming open-pit mine. The third part uses AHP-fuzzy comprehensive evaluation to evaluate the stability of the inner dump from 19 indexes in three aspects: characteristics of the inner dump, external influences, and early warning of slope change. The fourth part introduces the landslide monitoring and warning system based on 6G Internet of things technology in detail. The fifth part summarizes the thesis briefly.
2 Research background
In order to achieve high quality mine emergency communication with low delay and no co-frequency interference, the repeater adopts intelligent transport systems (ITS) dedicated frequency band 5,855–5,925 MHz (band 47) and 6G direct communication technology to establish and transmit wireless links. The diagram of the 6G direct connection communication is shown in Figure 3, where N is the number of interval devices that can reuse the same transmission resources.
In addition to the early warning of mining landslides and rescue, etc., as well as the early warning of some electrical fires, 6G technology (Wan et al., 2021) designed an electrical fire monitoring system based on wireless transmission technology. The system uses the heat (temperature) and threshold (current) generated by the monitoring line, adopts wireless communication technology to transmit the alarm information to the monitoring host, and the monitoring host issues instructions, and the information is transmitted to the personnel on duty, so as to prevent the occurrence of electrical fires. Through the test, the system has good stability, a strong ability to penetrate walls, and far-reaching transmission. In addition, the new generation of communication technology has further improved the application of unmanned driving in various environments (Wu and Tu, 2001) and emergency communication in rescue and disaster relief (Zhou et al., 2004).
At present, there are roughly four analysis methods for slope stability, which are the limit equilibrium method, engineering geological analysis method, numerical analysis method, and modern mathematical analysis method (Perski et al., 2009). The research object of slope engineering in inner dump is rock and soil mass in complex geological environment. Because the working face is advancing all the time, the mechanical properties of rock and soil mass are jointly affected by various complex factors such as the cause of stacking part, structure, and the environment in which it is located. The rock and soil mass in the inner dump shows characteristics such as non-uniformity, discontinuity, and time variation. The evaluation of slope stability of the inner dump is inevitably fuzzy. The fuzzy comprehensive evaluation method based on fuzzy set theory can better evaluate the hierarchical and fuzzy problems of influencing factors such as slope stability (Zhang, 2008a), such as rock slope stability evaluation (Wang and Tong, 2007a), two-level comprehensive evaluation model of slope stability (Li, 1992a), and multi-level fuzzy comprehensive evaluation model of rock slope stability (Li, 1997a). At present, the evaluation indexes of stability tend to focus more on the mechanical characteristics and external influences of the inner dump (Li, 1992b; Zhou et al., 1995; Li, 1997b; Zhang and Shi, 2000a; Zhang and Shi, 2000b; Wang and Tong, 2007b; Zhang, 2008b; Liu and Li, 2023b) and lack slope change warning.
3 Case situation
The Dongming open-pit mine is located in the eastern part of the Inner Mongolia Autonomous Region in China (Figure 4; Figure 5), located on the Hulunbuir High Plain, with Hailar City to the southeast and Chenbahu Banner to the west. The administrative division is subordinate to Chenbahu Banner. It is 20 km from Hyrule. The geographical coordinates of the mining area are 119°38′27″-119° 40′32″east longitude, 49°24′22″-49°25′37″north latitude, and the area of the mining area is 4
The Dongming open-pit coal mine is located on the north bank of the Hailar River on the western slope of the Great Khingan Mountains. It is a slightly undulating high plain, connected with low mountains and hills in the north and northeast, with an elevation of
This region is a subcold continental climate, winter cold and long snowy; Summer is hot and short, seasonal rainy, spring and autumn monsoon drought; The average annual temperature is
According to the geological engineering characteristics of the Dongming open-pit mine, the slope instability mode, the type of landslide, as well as the overall analysis of mining engineering conditions, it is necessary to have inner dump slope safety evaluation and monitoring to ensure safe production, considering that rain water is prone to producing gullies in spring and summer.
4 Stability evaluation of inner dump
The occurrence of landslide accidents in dump sites is a gradual process. The change is usually slow at the beginning, but with the passage of time, the deformation of slopes accelerate gradually, and the development speed is faster when closed to landslide accident. According to the landslide mechanism of dumps, the stability evaluation of the inner dump is divided into three aspects, namely, the characteristics of the inner dump, the external influences, and the early warning of slope changes.
4.1 Establishment of evaluation model
Based on the previous monitoring data, research reports, and characteristics of the Dongming open-pit slope stability in water-rich mining areas in cold areas, comprehensive research and analysis of various conditions, the stability evaluation of the inner dump is formed, and the system is divided into three levels. On the basis of the preliminary evaluation system framework, 30 geoscience and geotechnical engineering experts were invited to refine and determine the evaluation system structure and index selection. Finally, the system was divided into three levels with 19 indicators.
The first layer is the objective layer (A), which is the stability evaluation of the inner dump and the top level and ultimate goal of the system.
The second layer is the criterion layer (B), which mainly focuses on the comprehensive evaluation of the stability of the internal dump from three aspects: characteristics of the internal dump (B1), external influences (B2), and slope changes (B3).
The third layer is the index layer (C), which is the concrete expansion of the criterion layer.
To sum up, the stability evaluation system of the inner dump consists of one target layer, three criterion layers, and 19 indicator layers, as shown in Figure 6.
In terms of the characteristics of the inner dump, various factors that may affect the characteristics of the dump in the open pit are comprehensively considered in the inner dump characteristics’ part, including the foundation bed, basement bedrock, internal friction angle, slope, height, step height, platform width as inner dump features, and cohesive force moisture content, permeability coefficient and porosity as stacking material. For the external part, rain is the main factor that causes landslide disasters in open pit mines in cold areas. Therefore, two indexes of rainfall intensity and rainfall duration, as well as the maximum seismic intensity related to earthquakes and the weathering alterations related to soil discharge were selected. In the newly added change of side slope section, indicators that can be measured in real time were mainly added: surface displacement, internal displacement, pore water pressure, and the internal stress of rock and soil.
Index quantification and grade are determined by Expert ratings based on Dongming Open-pit geoscience and geotechnical engineering feature, Engineering rock mass classification standard and Codes such as Code for waste dump design of metal mine (GB 51119-2015).
where P is the void water pressure and K is the calibration coefficient of the measured pore water pressure gauge.
The internal stress of rock and soil takes the formula for calculating the original rock stress given by Kinnick in 1925 in the hypothesis of homogeneous isotropic linear elastomers: the vertical principal stress is equal to the weight of the rock and soil body on the unit base area, that is,
where α is the average volume weight of the overlying rock and Z is the depth.
There are also two horizontal principal stress σx and σy, which is equal to side stress coefficient λ multiply vertical principal stress, that is,
while side stress coefficient is related to Poisson ration, that is
General speaking μ≈0.2–0.3 as μ is Poisson ratio if object is rock mass, and then λ≈0.25 here.
4.2 AHP weight calculation and consistency test
4.2.1 Single hierarchical sorting
Single hierarchical ranking refers to the ranking of the importance of each factor at this level relative to the factor at the previous level.
The method of determining the maximum eigenroot of a matrix and corresponding eigenvectors is.
1) The matrix is normalized by column
2) Sum the normalized matrix by row to get
3) Normalize the vector
4) Calculate the maximum feature root
5) Get the weight vector
6) Questionnaires were sent to experts in civil engineering, who judged the relative importance of factors under the same index layer by pairwise comparison between factors, and scored the importance of factors in the questionnaire using the form of 1-9 and its reciprocal. Therefore, it is easy to get the importance rankings of criterion layer: B1 internal characteristics >B2 External influences>>B3 Slope variations. Among criterion layers, the importance rankings of index layer of B1 layer show that C3 Internal friction angle>C8 Cohesive force>C1 Foundation bed>C9 Moisture content>C4 Slope>C5 Height>C6 Step height>C7 Platform width>C11 Porosity>C10 Permeability coefficient>C2 Basement bedrock. Moreover, the importance rankings of B2 layer are C13 Rainfall intensity>C14 Duration of rainfall>C12 Maximum seismic intensity>C15 Weathering alterations; and C17 Internal displacement>C18 Pore water pressure>C16 Surface displacement>C19 Internal stress of rock and soil in B3 layer.
4.2.2 Determine the index membership degree
To determine the membership degree relationships related to evaluation, questionnaire surveys were sent to experts in related fields, and the data results of the questionnaire were analyzed after evaluation and scoring. Membership was represented by R, and the absolute value reduction method was used to calculate:
According to the value
The i th index of the evaluation factor U corresponds to the evaluation value generated by the j item in the index set V, which is represented by rij.
For example, the proportion of C1 with high stability, average stability, and low stability is 0.2, 0.7, and 0.1, respectively. Therefore, the C1 membership degree is
4.2.3 Consistency check
where CI is the consistency index and λmax is the largest characteristic root. [CW]i is the Ith component of the matrix [CW]. Define conformance metrics
RI is the value of the random consistency index, as shown in Figure 7.
CR is the consistency ratio; RI is a random consistency index.
Criterion layer and index layers all passed the conformance test, with the judgment matrix shown in Tables 1–5.
4.3 Fuzzy comprehensive evaluation
Fuzzy comprehensive evaluation is similar to analytic hierarchy process (AHP), but it does not evaluate which specific plan is better, but which of the ratings of very bad, bad, good, and very good is more consistent with the overall situation of medium our overall index A. In this project, it is of great significance to know the grade of slope safety in inner dump, and evaluating grades of each indicator is shown in Table 6.
4.3.1 Membership degree of the qualitative index
The standard of the qualitative index membership degree can be determined by statistical method. The calculation formula is adopted:
where i is the index and k is the evaluation level (
4.3.2 Membership degree of quantitative indicators
The membership degree of quantitative indicators can be determined thanks to the grade standard, that is to say, if the obtained survey results are in a certain standard value or within a certain interval, the membership degree is 1, and the others are 0. Get the index layer weight. Among them.
B1-B11; B12-B15;
B16-B19.
4.3.3 Membership matrix
The membership matrices of the characteristics of B1 inner dump, B2 external influences, and B3 slope changes are presented in Figures 8-10, respectively.
Firstly, the weight of the index layer is calculated, and then the first-order fuzzy evaluation can be obtained by multiplying the right weight vector with the membership matrix, and the following formula is used:
For example,:
By the same token, you get k1, k2, k3, and you get K as follows, as shown in Figure 11.
The weight of the criterion layer is written as follows:
Then the weight of criterion layer is used to multiply the membership matrix:
The second-order fuzzy evaluation can be obtained as follows:
The five indexes respectively correspond to high suitability, high suitability, average suitability, low suitability, and low suitability. The highest value of fuzzy evaluation was 0.8411, indicating that it is in the high suitability rating on the whole. In order to obtain the final score, the evaluation set is assigned 1 to 5 points, by the formula:
The total score was 4.7853, which belongs to the high security level. This is mainly attributed to internal characteristics, including the current stacking material and stacking methods, but the impact of rain intensity and duration as well as ground water welling cause tremendous impact as the main disaster-inducing factors. With the advance of the working surface, the inner dump characteristics change, which affect stability. Therefore, it is necessary to monitor rainfall and surface deformation for early warning. Based on the 6G Internet of things, real-time monitoring and transmission of rainfall and displacement in various locations on the slope can be realized, improving the efficiency and accuracy of mine safety monitoring.
5 Application of monitoring and warning system based on the 6G internet of things
Combined with the latest 6G Internet of things technology, a remote landslide monitoring and early warning system can be established for long-term monitoring and early warning, and real-time tracking of the deformation and failure trend of landslides, timely discovery and prediction of dangerous situations, and corresponding measures are taken to prevent the loss of life and property caused by sudden disasters.
The main difference between monitoring and early warning is to judge monitoring data due to early warning criteria, and to issue timely early warning and risk response, as shown in Figure 12. This requires the landslide monitoring and early warning system to have an automatic identification and judgment function thanks to the early warning criteria, and send out early warning information. The monitoring and early warning system organically combines 6G, the Internet of things, and landslide early warning technology. Taking rainfall and surface deformation as the key monitoring objects, data of rainfall, surface cracks, and GNSS surface displacement through monitoring stations are transmitted through 6G technology and analyzed and processed on the server, and finally advanced warning is conducted thanks to the effective early warning criteria.
Using the latest 6G Internet of things technology, a remote landslide monitoring and early warning system can be established for long-term monitoring and early warning of landslides. The system can track the deformation and failure trends of landslides in real time, judge the monitoring data according to the early warning criteria, and issue early warning and hedge responses in time to prevent the loss of life and property caused by sudden disasters. The system uses 6G technology to transmit monitoring data. Since the main cause of disasters in the inner dump is precipitation, data are collected by sensing and sensing monitoring stations such as rainfall, surface cracks, and GNSS surface displacement, and the data are analyzed and processed on a server to realize automatic identification and judgment functions, and advance warning is carried out depending on effective warning criteria. Therefore, the monitoring and early warning system plays an important role in landslide early warning technology, which can improve the efficiency of monitoring and management and contribute to the sustainable development of society.
5.1 Data collection
Data acquisition is one of the core steps of the monitoring and early warning system. Since water is the main disaster factor of water-rich open-pit mines in cold regions, landslide disaster field monitoring stations are arranged, equipped with a combination of machine vision monitoring systems, GNSS monitoring systems, rainfall gauges, groundwater level monitoring equipment, second-order work monitoring equipment, and other related monitoring equipment to perceive the slope and environmental state data depending on the specific slope monitoring needs. These devices collect monitoring data periodically, communicate directly with the server on the device side, and transmit the collected data to the server on the device side over the network.
5.1.1 GNSS
GNSS mobile stations and self-calibration cooperative targets are installed on the rods of monitoring equipment. GNSS data acquired by receivers’ collecting data, and temporary storage and fixed time interval transmission are realized by transmission control boxes. The displacement observation platforms can simultaneously obtain the surface displacement data calculated by real-time difference decomposition of the difference processing program of GNSS mobile station data and the three-dimensional displacement data obtained by the machine vision surface displacement monitoring system. By comparing the two sets of displacement data, the accuracy analysis function of the field monitoring test system of slope surface displacement monitoring based on binocular vision can be realized.
A rainfall monitoring point should be set up every 20 km2 catchment area in the monitoring area, and the measuring point should be arranged in an open position away from people. The rainfall monitor can be installed next to the GNSS reference station to facilitate site construction and maintenance.
The point layout density of the integrated surface displacement observation station should refer to the layout requirements of the GNSS displacement monitoring system in the Survey Code for Landslide Prevention and Control Engineering. According to the geological conditions of the monitoring points, a monitoring section should be arranged along the vertical and horizontal axis of the the potential landslide area, and each section should have no less than three measuring points.
5.1.2 Rainfall monitoring subsystem
Rainfall is an important inducing factor of landslide disasters in this study area. The selection of rainfall monitoring points should be based on the “watershed control principle, elevation control principle, communication smooth principle, terrain open principle, and area control principle” in order to study the rainfall characteristics of landslides induced.
The initial threshold is divided according to the corresponding rainfall level of the National Meteorological Administration (after the monitoring system runs steadily, the early warning parameters can be optimized and adjusted due to the local hydrological rainfall data and actual monitoring data), in which: the first-level warning value consists of a rainfall rate of 1.0 mm/min, an hourly rainfall of 15 mm, and a daily rainfall of 50 mm; the second-level warning value is a rainfall rate of 2.0 mm/min, an hourly rainfall of 30 mm, and a daily rainfall of 100 mm; and the third-level warning value is a rainfall rate of 4.0 mm/min, an hourly rainfall of 60 mm, and a daily rainfall of 200 mm.
5.2 Data storage
To facilitate data exchange with the application server, the server on the device uses a cache database and periodically pushes data to the application server due to the data request specifications of the application server. After receiving the data pushed by the device server, the application server preprocesses the data before storing it, and then stores the data in the database of the storage center. It can realize the real-time monitoring and early warning of landslides. The collected data can be directly transmitted to the server for analysis and processing, and advance warning can be carried out thanks to effective warning criteria. Thus, the accuracy and timeliness of early warnings are greatly improved, and the reliability and precision of landslide early warning are guaranteed. At the same time, the cache database and data push technology can realize the rapid transmission and processing of data and improve the efficiency and stability of the system.
5.3 Data storage layer
The built-in data parsing function is used to analyze and store the data on the database according to the professional data protocol of the data acquisition layer. The original database and backup database are used to store the monitoring perceived data values.
5.4 Data transmission
In the Internet of things, 6G communication, as a high-quality and real-time monitoring data transmission system, has many advantages, such as high speed, large capacity, and low delay. In the Dongming inner dump detection project, the corresponding Internet of things terminal is used to monitor rainfall, ground cracks, and surface deformation in real time, and then the 6G communication channel is selected for data transmission.
Based on the data output interface and communication protocol of high-precision mechanical sensors, an automatic data acquisition device was developed. The equipment includes a data acquisition module, data storage module, and data transmission module, and is equipped with solar power supply equipment. The data acquisition device is connected with the sensor and the solar power supply system through shielded cables to form a complete data acquisition system. The system adopts the Beidou satellite and GPRS dual communication mode (BD/GPRS mode) after data acquisition to transfer the data from the site to the indoor monitoring center, which can realize the rapid transmission and processing of data. At the same time, the system collects the data of the monitoring points in two modes: network networking and line networking, and can select the appropriate data transmission mode according to different monitoring areas and transmission distances, which improves the flexibility and adaptability of the system.
5.5 Data monitoring and early warning
The monitoring and early warning platform can provide comprehensive analysis of monitoring data. Users can specify the time range of data generation and visually display the data of all monitoring points monitoring landslides. This can help users better understand the change trends and stability of the slope, and take appropriate measures in time. The method of Li et al. (2021) was adopted to improve the tangent Angle warning criterion for landslide warning. By analyzing real-time monitoring data and warnings, landslide velocity and deformation trends could be judged more accurately, and corresponding measures could be taken in time to ensure slope safety (Figure 13).
6 Conclusion
This research paper introduces an advanced integrated monitoring and early warning system designed for mining areas, utilizing cutting-edge 6G communication technology. The system employs a novel AHP-fuzzy comprehensive evaluation method with a three-layer model and 19 indicators to assess and monitor the stability of water-rich inner dump slopes in open-pit mines. The evaluation results indicate a high safety level score of 4.7853. To ensure the long-term safety of slopes, a geological disaster monitoring and early warning system is implemented, leveraging the capabilities of the 6G communication network. This system takes advantage of low-power consumption, long-distance communication, and multi-channel data return facilitated by differential chirp modulation technology. With its broad coverage, the system effectively guarantees slope stability throughout the entire process of evaluation, monitoring, and warning. The research outcomes offer valuable insights for slope assessment and monitoring in cold regions housing water-rich open-pit mines.
Data availability statement
The datasets presented in this study can be found in online repositories. The names of the repository/repositories and accession number(s) can be found in the article/Supplementary material.
Author contributions
XS: Writing–original draft, Writing–review and editing. HS: Data curation, Writing–original draft, Conceptualization. TJ: Formal Analysis, Supervision, Conceptualization, Writing–original draft. PZ: Visualization, Conceptualization, Writing–original draft. SP: Investigation, Data curation, Validation, Writing–original draft. SZ: Project administration, Resources, Writing–original draft.
Funding
The author(s) declare financial support was received for the research, authorship, and/or publication of this article. This work was supported by the National Natural Science Foundation of China (Grant number: 41772335).
Conflict of interest
Authors HS, TJ, and PZ were employed by the China Building Technique Group Co., Ltd. Author SZ was employed by the North Blasting Technology Co Ltd.
The remaining authors declare that the research was conducted in the absence of any commercial or financial relationships that could be construed as a potential conflict of interest.
Publisher’s note
All claims expressed in this article are solely those of the authors and do not necessarily represent those of their affiliated organizations, or those of the publisher, the editors and the reviewers. Any product that may be evaluated in this article, or claim that may be made by its manufacturer, is not guaranteed or endorsed by the publisher.
References
Anua´rio Brasileiro de Desastres Naturais, (2012). Ministery of national integration. Brası´lia: National Center for Management of Risks and Disasters, 78.
Chen, P. (2022). Stability analysis and safety evaluation of west Dump slope in Anjialing open-pit Mine. Open-pit Min. Technol. 37 (01), 36–38. (in Chinese).
Guadagno, F. M., Revellino, P., and Grelle, G. (2001). “The 1998 Sarnolandslides: conflicting interpretations of a natural event,” in International conference on debris-flow hazards mitigation: mechanics. doi:10.4408/IJEGE.2011-03.B-009
Hu, W., and Shulin, L. (2002). A study on the acoustic emission technology in the analysis of rock slope stability. Min. Res. Dev. 22 (3), 9–11. (in Chinese).
Jafari, N. H., Stark, T. D., and Merry, S. (2013). The July 10, 2000 Payatas landfill slope failure. Int. J. Geoeng Case Hist. 2 (3), 208–228. doi:10.4417/IJGCH-02-03-03
Jiang, B., Xin, Z., Zhang, X., Deng, Y., Wang, M., Li, S., et al. (2023). Mechanical properties and influence mechanism of confined concrete arches in high-stress tunnels. Int. J. Min. Sci. Technol. 33, 829–841. doi:10.1016/j.ijmst.2023.03.008
Lavigne, F., Wassmer, P., Gomez, C., Davies, T. A., Hadoko, D. S., Yan, T., et al. (2014). The 21 February 2005, catastrophic waste avalanche at Leuwigajah dumpsite, Bandung, Indonesia. Geoenviron Disasters 1 (1), 10. doi:10.1186/s40677-014-0010-5
Li, J. (1992a). Hierarchical fuzzy comprehensive evaluation method for rock slope stability. J. Guizhou Inst. Technol. 21 (4), 27–35. (in Chinese).
Li, J. (1992b). Hierarchical fuzzy comprehensive evaluation method for rock slope stability. J. Guizhou Inst. Technol. 21 (4), 27–35. (in Chinese).
Li, Z. (1997a). Application of fuzzy analysis in slope stability evaluation. Chin. J. Rock Mech. Eng. 16 (5), 490–495. (in Chinese).
Li, Z. (1997b). Application of fuzzy analysis in slope stability evaluation. Chin. J. Rock Mech. Eng. 16 (5), 490–495. (in Chinese).
Li, Z., Fang, L., Sun, X., and Peng, W. (2021). 5G IoT-based geohazard monitoring and early warning system and its application. EURASIP J. Wirel. Commun. Netw. 2021 (1), 160. doi:10.1186/s13638-021-02033-y
Liu, H. M., Li, X. L., and Yu, Z. Y. (2023a). Influence of hole diameter on mechanical properties and stability of granite rock surrounding tunnels. Phys. Fluids 35 (6), 064121.
Liu, J., Wang, X., and Guobin, S. (2021). Study on slope disaster mechanism of inner dump in Yimin open-pit Mine. Saf. Coal Mine 52 (03), 234–236.
Liu, S. M., and Li, X. L. (2023a). Experimental study on the effect of cold soaking with liquid nitrogen on the coal chemical and microstructural characteristics. Environ. Sci. Pollut. Res. 30 (3), 36080–36097. doi:10.1007/s11356-022-24821-9
Liu, S. M., and Li, X. L. (2023b). Experimental study on the effect of cold soaking with liquid nitrogen on the coal chemical and microstructural characteristics. Environ. Sci. Pollut. Res. 30 (3), 36080–36097. doi:10.1007/s11356-022-24821-9
Liu, S. M., Sun, H. T., Zhang, D. M., Yang, K., Li, X., Wang, D., et al. (2023b). Experimental study of effect of liquid nitrogen cold soaking on coal pore structure and fractal characteristics. Energy 275 (7), 127470. doi:10.1016/j.energy.2023.127470
Ma, Y. (2020). Stability analysis and Safety evaluation analysis of dump slope. World Nonferrous Met. (05), 193–194. (in Chinese).
Merry, S., Kavazanjian, E., and Fritz, W. U. (2005). Reconnaissance of the july 10, 2000, payatas landfill failure. J. Perform. Constr. Facil. 19 (2), 100–107. doi:10.1061/(asce)0887-3828(2005)19:2(100)
Perski, Z., Ramon, H., Wojcik, A., and Wojciechowski, T. (2009). InSAR analyses of terrain deformation near the Wieliczka Salt Mine,Poland. Eng. Geol. 106, 58–67. doi:10.1016/j.enggeo.2009.02.014
Wan, H., Cai, D., He, X., et al. (2021). Experimental research on monitoring deep deformation of high-steep slopes by using optical fiber sensing. J. China Three Gorges Univ. Sci. 23 (1), 20–23. (in Chinese).
Wang, F. N., Guo, Z. B., Qiao, X. B., Fan, J., Li, W., Mi, M., et al. (2021). Large deformation mechanism of thin-layered carbonaceous slate and energy coupling support technology of NPR anchor cable in Minxian Tunnel: a case study. Tunn. Undergr. Space Technol. 117, 104151. doi:10.1016/j.tust.2021.104151
Wang, Q., Xu, S., Xin, Z., He, M., Huayong, W., and Jiang, B. (2022). Mechanical properties and field application of constant resistance energy-absorbing anchor cable. Tunn. Undergr. Space Technol. 125, 104526. doi:10.1016/j.tust.2022.104526
Wang, Q., and Tong, R. (2007a). Analysis and application of fuzzy comprehensive evaluation method in Enterprise safety evaluation. J. North China Inst. Sci. Technol. 3 (4), 26–31. (in Chinese).
Wang, Q., and Tong, R. (2007b). Analysis and application of fuzzy comprehensive evaluation method in Enterprise safety evaluation. J. North China Inst. Sci. Technol. 3 (4), 26–31. (in Chinese).
Wang, T. (2020). An open mined-out area in mine slope stability analysis. Chin. Min. Eng. 49 (02), 9–11. (in Chinese).
Wu, X., and Tu, Y. (2001). Methods and new development of landslide activity monitoring. China Instrum. (1), 10–12. (in Chinese).
Wu, X., and Tu, Y. (2002). New approach of landslide activity monitoring—probing into TDR technology. Chin. J. Rock Mech. Eng. 21 (5), 740–744. (in Chinese).
Xia, B., Zhang, Y., Yu, L., et al. (2001). Monitoring and treatment technology of landslide geological disasters in China. Explor. Eng. Tunneling, 87–90. (in Chinese).
Xia, Y., and Zhu, R. (1999). Multilevel Fuzzy Comprehensive evaluation System for rock slope stability. J. Eng. Geol. 7 (1).
Xiong, F., Zhu, C., Feng, G., Zheng, J., and Sun, H. (2023). A three-dimensional coupled thermo-hydro model for geothermal development in discrete fracture networks of hot dry rock reservoirs. Gondwana Res. 122, 331–347. doi:10.1016/j.gr.2022.12.002
Yin, Q., Wu, J. Y., Zhu, C., He, M. C., Meng, Q. X., and Jing, H. W. (2021). Shear mechanical responses of sandstone exposed to high temperature under constant normal stiffness boundary conditions. Geomechanics Geophys. Geo-Energy Geo-Resources 7, 35. doi:10.1007/s40948-021-00234-9
Yuan, Y., and Guo, X. (2021). Analysis on slope stability of Antaibao open-pit Mine. Open-pit Min. Technol. 36 (01), 76–78+82. (in Chinese).
Zhang, J. C., Li, X. L., Qin, Q. Z., Wang, Y., and Gao, X. (2023). Study on overlying strata movement patterns and mechanisms in super-large mining height stopes. Bull. Eng. Geol. Environ. 82 (3), 142. doi:10.1007/s10064-023-03185-5
Zhang, L., Lu, Z., Tang, S., Zhang, M., Zhang, R., Huang, Y., et al. (2021). Vegetation composition and community stability evaluation of open-pit coal mine slope. Acta Ecol. Sin. 41 (14), 5764–5774.
Zhang, Y., and Shi, H. (2000a). Two-stage fuzzy comprehensive evaluation of slope stability. J. Eng. Geol. 8 (1), 31–34.
Zhang, Y., and Shi, H. (2000b). Two-stage fuzzy comprehensive evaluation of slope stability. J. Eng. Geol. 8 (1), 31–34.
Zhang, Y. (2008a). Slope engineering. Beijing: China Architecture and Building Press, 4–8. (in Chinese).
Zhang, Y. (2008b). Slope engineering. Beijing: China Architecture and Building Press, 4–8. (in Chinese).
Zhou, C., Chen, W., and Guoqi, T. (2004). Study on rock body thrust monitoring system with OTDR distributing optical fiber. Explor. Eng. Drill. Tunneling (1), 43–46. (in Chinese).
Zhou, J. (2022). Study on slope stability of dump in the first mining area of huolin hebei open-pit mine. Liaoning Technical University. (in Chinese).
Keywords: AHP-fuzzy comprehensive evaluation, 6G, GNSS, mine monitoring and warning, open-pit mine
Citation: Sun X, Shen H, Jiang T, Zhang P, Peng S and Zhang S (2023) 6G-enabled open-pit mine security: toward wise evaluation, monitoring, and early warning. Front. Earth Sci. 11:1278308. doi: 10.3389/feart.2023.1278308
Received: 16 August 2023; Accepted: 28 September 2023;
Published: 26 October 2023.
Edited by:
Xuelong Li, Shandong University of Science and Technology, ChinaReviewed by:
Qiguang Di, China Railway 12th Bureau Group Co., Ltd., ChinaYanpeng He, Xi’an University of Science and Technology, China
Jinlong Zhou, China Coal Research Institute, China
Copyright © 2023 Sun, Shen, Jiang, Zhang, Peng and Zhang. This is an open-access article distributed under the terms of the Creative Commons Attribution License (CC BY). The use, distribution or reproduction in other forums is permitted, provided the original author(s) and the copyright owner(s) are credited and that the original publication in this journal is cited, in accordance with accepted academic practice. No use, distribution or reproduction is permitted which does not comply with these terms.
*Correspondence: Honghao Shen, shenhonghao@cbtgc.com