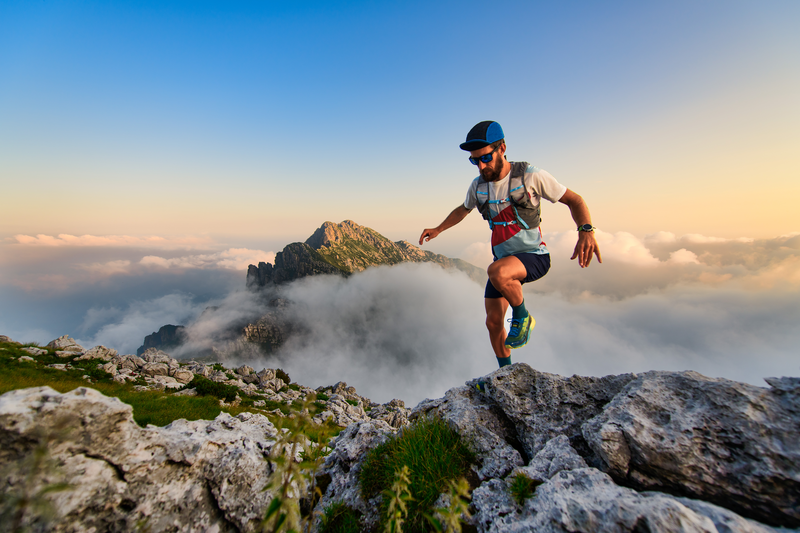
94% of researchers rate our articles as excellent or good
Learn more about the work of our research integrity team to safeguard the quality of each article we publish.
Find out more
PERSPECTIVE article
Front. Earth Sci. , 17 November 2023
Sec. Hydrosphere
Volume 11 - 2023 | https://doi.org/10.3389/feart.2023.1271547
This article is part of the Research Topic Microplastic and Nanoplastic Fate and Transport in Groundwater, Surface Water, and Soil Ecosystems: Occurrence, Methods, and Perspective View all 4 articles
Early career researchers (ECR) face a series of challenges related to the inherent difficulties of starting their careers. Microplastic (MP) research is a topical field attracting high numbers of ECRs with diverse backgrounds and expertise from a wealth of disciplines including environmental science, biology, chemistry and ecotoxicology. In this perspective the challenges that could hinder scientific, professional, or personal development are explored, as identified by an international network of ECRs, all employed in MP research, that was formed following a bilateral workshop for scientists based in the UK and China. Discussions amongst the network were grouped into four overarching themes of technical challenges: in the field, in the laboratory, in the post data collection phase, and miscellaneous. The three key areas of representativeness, access to appropriate resources, training, and clean labs, and the use of databases and comparability, as well as the overarching constraint of available time were identified as the source of the majority of challenges. A set of recommendations for pathways forward are proposed based on the principles of research openness, access to information and training, and widening collaborations. ECRs have great capacity to promote research excellence in the field of MPs and elsewhere, when provided with appropriate opportunities and suitable support.
The term Early Career Researcher (ECR), though not well defined, is typically applied to persons in the latter stages of Doctoral research, and the early stages of post-doctoral research (Bazeley, 2003). Defining the term becomes more challenging when accounting for the multiple trajectories taken within a researcher’s career, resulting from, for example, career breaks, or shifts in career focus. Despite their key research delivery role and dominance across the research landscape, ECRs are often employed on short-term contracts meaning that work (or life) commitments can be disjointed or cut short, as researchers move from place to place. Other challenges are linked to a long-hours culture of working, expectations to be mobile (i.e., move institutions frequently), and limited time in their funded workload to compete for grant applications (NERC, 2018). ECRs pursuing interdisciplinary work may face additional challenges linked to the requirement to establish, develop and use networks beyond their original field of expertise (Andrews et al., 2020). Ultimately these factors can contribute to poor mental well-being, job dissatisfaction and high staff turnover, and limit project potential where individuals have no choice but to leave precarious research programmes for more secure opportunities. Issues facing early career researchers are gaining some attention with recent papers specifically targeting the ECR audience with advice and guidance (Agathokleous, 2022; Arole et al., 2023).
One of the fields of research with a high proportion of ECRs, is microplastics (MPs), an emerging environmental contaminant of high concern. This is a relatively new area of study, whereby more experienced researchers who are already established in other (potentially related) fields, often take on PhD students or post-doctoral fellows to develop institutional or group expertise. Furthermore, MP contamination has been deemed a “hot topic” which many wishing to pursue a career in science may find enticing.
MPs are defined as plastic particles smaller than 5 mm and greater than 100 nm, although there is still ongoing debate around their definition (GESAMP, 2015; GESAMP, 2023). The lack of comparable datasets and a wide variety of potential, but not equally accessible, methodologies have been identified as major hurdles to understanding MPs in the environment (Connors et al., 2017; Nel et al., 2021). This is further complicated by the fact that MPs are a diverse suite of contaminants ranging in size, colour, shape, and chemical properties to name a few of the variable characteristics (Hartmann et al., 2019; Rochman et al., 2019). Consequently, one method does not universally detect particles across the full breadth of the spectrum. Nevertheless, like most “hot topics”, MP researchers must deal with the growing demand from the public and legislative authorities to provide timely evidence to drive decision making, despite the array of unknowns hindering current interpretations.
Understanding MP contamination in multiple dimensions across a heterogeneous matrix of natural and anthropogenic compartments is challenging and relies on comprehensive understanding of environmental scenarios. Luckily, the inherently interdisciplinary nature of MP pollution attracts a diversity of ECRs who, together, can use their varied talents, skills, and expertise to develop and advance cross-disciplinary understanding of MP sources, pathways, environmental fate, and ecotoxicology, as well as environmental psychology, management, and policy. Though this represents an exciting challenge, it can leave many ECRs feeling overwhelmed and powerless, especially when faced with a plethora of collection methodologies, validation techniques and interpretation tools that may or may not be pre-determined by their principal investigators (PIs), associated institutions and available equipment.
This perspective piece explores various challenges that MP ECRs may face that could hinder project delivery, scientific advancement, and career trajectory, with the aim to better target and resolve these issues. This is not only informative for those ECRs involved in the field but also PIs and team leaders, funders, and other scientific authorities, especially as return on investment in research is underpinned by successful project delivery. The paper also offers pathways forward to these challenges, while highlighting the value of ECRs and need for investment in their careers and general wellbeing.
An international network of ECRs, all working in the field of MP pollution, was formed as a result of a successful bilateral (UK-China) workshop. The online workshop, that took place on 1–3 June 2021, was funded by the Newton Fund Researcher Links programme and the Natural Science Foundation of China (NSFC), and focused on analytical techniques to isolate and characterize MPs from environmental and biological samples. Formal and informal networks (e.g., journal clubs, mailing lists, recorded webinars, social media) allow for more open and transparent sharing of data, outcomes and lessons learned. These networks are also an opportunity for researchers to collaborate and bid for grants together, resulting in potentially increased exposure and chances of success. Due to the Covid-19 pandemic, an increase in remote working has also resulted in more virtual spaces for international sharing and communication. Although there is a huge benefit to in-person meetings, virtual spaces allow for increased accessibility especially between colleagues who may not always be able to travel. These platforms give researchers the opportunity to candidly discuss advancements that are not yet published, challenges that are not often shared, and the opportunity to form communities that will hopefully strengthen collaboration beyond inner circles. Overall, this workshop facilitated the development of a collaborative network between British and Chinese ECRs which would not otherwise have been possible during the Covid-19 pandemic.
Twenty-nine participants from the UK (De Frond et al., 2023) and China (Horton et al., 2021) took part in the workshop. ECRs were defined by the funding body as holding a PhD (or having equivalent research involvement) or having up to 10 years post-PhD research experience. At the time of the workshop, participants also must have held a research or academic position (a permanent post, research contract, fellowship, PhD studentship, etc.) at a recognised research institution either in the UK or China. It must be noted that no socio-economic and demographic data was gathered for participants, however these factors would have an impact on the types of challenges individuals would face in research/academic positions.
Most of the participants were affiliated with universities as fellows, research associates and lecturers while others worked for research centres or environmental institutes. Almost half of the participants were awarded their PhD less than 5 years prior to the workshop (N = 15; Figure 1) but there was a good balance of experience ranging from participants that were in the final stages of completing their doctoral projects, to well-established scientists. It must be noted that experience in the field of MPs is not necessarily positively correlated with years since PhD award, in fact some well-established scientists were new to the field. The background of the participants was truly multidisciplinary, encompassing environmental science, biology, marine science/biology, oceanography, analytical/environmental/physical chemistry, ecology, nanotechnology, risk assessment, civil engineering, geology, hydrogeology, ecotoxicology and sociology to state a few of the expertise represented. In terms of MP related research, projects covered a diversity of environmental compartments (freshwater, estuarine, marine, mangrove, air, soil, glacial) as well as biota. Projects focused not only on sampling and analysis but also the development of new methods, technology and monitoring systems, meta-analysis of data, evaluation of current practises, investigation of MP transport, fate and deposition, and investigation of MP toxicological effects. From an outreach and stakeholder engagement perspective, implementation of citizen science projects and development of policies and legal frameworks were also discussed.
FIGURE 1. Participant numbers and associated years since PhD award for attendees of the bilateral workshop (UK and China) on analytical techniques to isolate and characterize microplastics from environmental and biological samples. Dashed line shows average time (years) since PhD award.
The challenges faced by the ECR community in MP research was a recurring theme throughout this workshop. These discussions continued after the workshop through an online community of the workshop attendees. To guide discussions amongst the network, an anonymous platform was used in which participants could share challenges related to four (primarily technical) overarching themes. These were: 1) Technical challenges in the field; 2) Technical challenges in the laboratory; 3) Technical challenges in the post data collection phase; and 4) Technical challenges in general. Thereafter the ECR network entered further discussions through online meetings. Two 1-h meetings were held for each topic listed above. Although many of these issues raised are universal in the field of MPs, and do not affect ECRs in isolation, the risks associated with these challenges disproportionately impact the research and career trajectory of ECRs over established researchers, especially where appropriate support is lacking.
The insights from this diverse community were consolidated following these semi-structured meetings to identify challenges shared by the participants. Whilst it is not the purpose of this article to identify, for example, the appropriate sampling design for individual matrices, some recommendations and considerations are summarised below. Topics focused on here are 1) Representativeness, 2) Access to appropriate resources, training, and clean labs, 3) Databases and comparability, and 4) Time as an overarching constraint.
A recurring theme was “representativeness” and what this means in MP research specifically focusing on field studies. Site, matrix, collection method, sample size and number, processing technique, identification procedure and study duration may all influence the type and quantity of MP particles detected (Green et al., 2018; Nel et al., 2018; De Frond et al., 2023). Some of these collection and processing decisions will be governed by the chosen research questions—for example, sampling particular sources (i.e., wastewater treatment plant) to understand input into a system (Horton et al., 2021; Margenat et al., 2021) or an entire river basin to understand variation in MP concentrations associated with differing land-uses (Mani et al., 2015; Weideman et al., 2020). Choices, however, are complicated by issues associated with availability of resources (discussed later), which is often out of the hands of the ECRs. A few examples expressed by the network attendees that may affect study design and simultaneously representativeness were funding constraints, short timeframes, fitting in with a larger predefined project, whether working domestically or with partners located abroad, equipment/infrastructure availability and accessibility, and the size of the team which will influence how much support is available for sampling. Another issue, brought about by the extremely fast-paced development of new methods, is that by the time a project is finished, when entering the peer-review process, the chosen approach may already have become outdated and superseded by more robust procedures, bringing the study design into question. Overall, it is important to have a clear understanding of what it is one wishes to represent, and how to appropriately disseminate the data considering inherent limitations.
In order to generate representative environmental data, sample collection must be considered over multiple dimensions: latitude, longitude, depth, and time. The relative importance of these dimensions varies between environmental matrices and is guided by the aims and the objectives of the project. Solid environmental matrices can be relatively static, such as soils and lake sediments, and in the absence of anthropogenic interferences such as dredging and ploughing, can encompass temporal trends within their depth profile (Zubris and Richards, 2005). This is not true of all sediments though. River sediments are highly variable in their form, necessitating different sampling procedures. They are susceptible to changes in flow and zoogeomorphic processes (Johnson et al., 2020; Wazne et al., 2023) that alter sediment mobility at short (diurnal), long (seasonal), and event-specific (e.g., flooding and drought) time scales (Hurley et al., 2018).
Quantification of MPs in waterbodies requires considerations of flow and mixing. Flowing water will carry particles in suspension and at its surface, and will be influenced by characteristics of a rivers reach including discharge, bed topography and course. In lentic environments, stratified systems will influence the detectability of some polymers (Zobkov et al., 2019; Uurasjärvi et al., 2021) requiring a systematic sampling campaign throughout the stratified zones. In a well-mixed system, a more randomised approach to sampling at depth may be better suited. For estuaries, tides and mixing of marine and riverine water further play a role in MP distribution (Vermeiren et al., 2016). For groundwater more research is needed to define how sampling should occur (Re, 2019; Viaroli et al., 2022). Representative MP sampling can therefore require expensive sampling programmes that require utilisation of boats and equipment capable of sampling at depth and on multiple occasions.
Once an appropriate sampling regime has been determined, MP researchers must consider their sampling method, and the volume and replicate number of samples they wish to collect to ensure representativeness. In locations or for sample types where MP concentrations are expected to be low, far greater volumes and numbers of samples are required in order to detect even small numbers, compared to highly contaminated sites where high concentrations could be found even by sampling small volumes. Therefore, we can never infer that simply because MPs were not detected, that they are absent from a site.
Though time consuming, pilot work that explores not just the feasibility of MP sampling, but also the variability within it, is key to ensuring that sampling procedures are able to account for the heterogeneity of this contaminant, or at minimum provide additional information on how to accurately interpret data collected. Each piece of MP research must determine its own limitations and demonstrate an awareness of these in the reporting of its findings. If provided with the opportunities and resources, ECRs are better placed than most to dedicate the time that is needed to this element of MP research.
The limitations outlined above mean that it is difficult to generate a truly representative piece of MP research. This is influenced by the variability of methods available to researchers, the diversity of the environmental systems these individuals work in, and the heterogeneity of MPs themselves (Zarfl, 2019). Despite this, MP researchers continue to present their findings with definitive concentrations and fluxes that are beyond the scope of their experimental design.
Appropriate dissemination of MP research, that is aware of the limitations of its sample collection, is therefore perhaps the most important element of representative MP research. This is not to undermine the value of studies that are unable to sample in a representative manner, but rather to encourage the drawing of appropriate conclusions from the data they generate. This appropriate dissemination should ideally encompass platforms beyond academic journals, such as the dissemination of research to non-expert audiences by means of outreach, policy documentation, and stakeholder engagement events. Incorporating this type of dissemination to non-academic audiences into the role of an ECR has additional benefits for the development of the researcher, broadening their skillset, network building, and providing them with the greatest opportunity for success in a highly competitive and precarious industry. However, such dissemination is not always available or valued by funders or PIs, and ECRs do not always have the power to incorporate this into their research within their contracted time.
The requirements of representative sampling of some (particularly aquatic) environments necessitates access to equipment and training that is not always available to ECRs. It is the responsibility of PIs to ensure that ECRs are suitably equipped to meet their potential and deliver robust contributions to a research project and research team. However, ECRs should be proactive in finding alternatives such as collaborating with external facilities. Budgeting time (discussed later) for the development of field procedures and associated problem solving, and the development of individuals should be included into project planning. Everyone involved should recognise these as vital steps in the research process despite the fact they are unlikely to yield the common deliverables (publications, conference presentations, funding applications) against which research success is too often measured in isolation.
Access to all the required analytical equipment was highlighted as a major challenge, as necessary infrastructure may not be available or may sit in somebody else’s laboratory/institution. The rules and regulations of different research institutions (both internal and external) were discussed and it was agreed that ECRs are relatively powerless to formalise these relationships, but are most affected by their absence. This is confounded by the precarity of the early career stage, when ECRs can use the kit they have access to, but do not always have the authority to allow, or time to train/supervise, fellow ECRs who would benefit from access to the equipment they use. It is highly recommended to develop networks or platforms for ECRs to communicate their needs or share their available information and accessibility to resources, analytical methods, and equipment. PIs can greatly assist by discussing what barriers may be in place early on when before the ECR is appointed so that the right tools are available, or networks and relationships are in place before the start of appointment. If these are not in place the ECR needs to be made aware of this early, so that appropriate steps can be taken to tackle these issues.
Another issue raised was what is the definition of a lab “space”. Some ECRs do not have their own labs, but rather a “space” in a shared area. This was especially challenging during the Covid-19 pandemic lockdowns, where access to labs was limited. This also has consequences for contamination control.
Contamination is a common challenge facing all MP researchers (regardless of career stage). It is known that MPs are widespread within indoor and outdoor air (Dris et al., 2017; Zhang et al., 2020; Jenner et al., 2021), lending the potential for them to become easily deposited onto samples during collection, processing, and analysis. Especially where environmental samples are expected to be relatively “clean”, i.e., have low concentrations of MPs, any inadvertently introduced contamination can have a significant effect on the number or mass of MPs reported. For this reason, it is essential, where possible, to eliminate or significantly reduce the amount of plastic material that the sample is likely to come into contact with at any stage (Prata et al., 2021). This can be done by having dedicated rooms to conduct MP research, when space and demand allows.
Nonetheless, despite all the measures that can be taken, it should be recognised that it is almost impossible to remove or prevent 100% contamination (Mintenig et al., 2019; Su et al., 2019; Horton et al., 2021). Knowing that even the most stringent measures to prevent plastic contamination can leave some particles, steps must also be taken to measure contamination through the use of procedural blank samples, and prevent inclusion of these false positives when reporting data. The simplest way to do this is to take an average of the particles present in the blank, and subtract this value from each environmental sample, thus constituting a blank-correction (Miller et al., 2017). To add weight to this approach, blank-correction can also be done by polymer type, particle size or particle shape (e.g., fibre, fragment, bead) (Lusher et al., 2017; Adams et al., 2021). For an even more rigorous approach to blank-correction, limit of detection (LOD) or limit of quantification (LOQ) may be used (Johnson et al., 2020; Horton et al., 2021). The chance of reporting false positives using this method is far reduced but does have the potential to eliminate real data, if samples are very clean. The most appropriate approach should therefore be selected based on the types of samples being analysed (clean/dirty) and the likelihood of contamination, which will vary for each study.
Reporting of data correctly, which is especially relevant in the field of MPs as comparability across studies often comes into question, is essential for accurate interpretations of individual case studies in global assessments. There have been recommendations of what information is necessary for transparent reporting to allow for this comparison and reproducibility, which include but are not limited to specifics on sample design and chemical identification (Cowger et al., 2020). These articles are extremely useful for ECRs and other researchers new to the field.
Not only do researchers need to report their finding appropriately, but also put the results and interpretations into the wider context. To get a holistic overview about the field, ECRs need access to journals through a subscription, which for many universities comes with a high price tag. This has in part been eased by social media and other networking platforms that allow researchers to contact authors more readily to request access or personal prints (Asyyed et al., 2019). In addition to the costs of journal subscriptions, the cost of open access (OA) publication is a further hurdle for ECRs. More than 14,000 journals have at least one gold OA publication, and around one third are fully OA (Collier, 2021). ECRs are in favour of the concept of OA, because it gives a study more visibility than traditional publications, and OA publications are available to readers for free (Pisoschi and Pisoschi, 2016). However, for most researchers, especially ECRs, their funds to cover the usually expensive OA publications fees are often inadequate, leading to a decline in ability to submit to these journals. In the long run, if the prices of OA journals decreased, or funding for publication increased, the problem of limited access to journals would be alleviated and ECRs would benefit.
Comparability is an overarching theme applicable to ECRs in the field of MPs as discussed under the reporting criteria topic. However, here, we have chosen to focus on spectroscopic databases as attendees felt this was one of the major factors affecting comparability.
Spectroscopic characterization is routinely conducted in MP analysis, with Raman and Fourier transform infrared spectroscopy (FTIR) being regularly employed (Cabernard et al., 2018). The specific analytical protocols vary due to the type of instrument and the level of resource available. For example, a researcher may only have access to a FTIR spectrometer without automated imaging capabilities (i.e., Attenuated-total-reflectance (ATR) FTIR), requiring selected particles to be analysed one at a time, hence time becomes a limiting factor (discussed below). This may subsequently lead to a lower proportion of the sample being analysed (Song et al., 2021). This ECR working group found there to be significant financial and access-related barriers to performing spectroscopic analysis on particulates, especially as numbers increase and sizes decrease. Additionally, when conducting research in low-to middle-income countries availability and accessibility of the necessary equipment becomes more challenging (Nel et al., 2021). More work needs to be done to understand what the minimum proportion of spectroscopic analysis is needed, along with how this proportion may vary depending on the matrix, and size and shape of MPs targeted, for example.
Chemometric analysis of spectral datasets is a complex task, one which commercial libraries have attempted to simplify for the end-user. Such commercial spectral libraries typically include a wide variety of material spectra, though how extensive such libraries are, depend upon the researcher’s commercial licence. The cost of commercial libraries increases the financial pressure on MP researchers. Furthermore, reliance on spectral libraries containing exclusively polymer only spectral datasets, without appropriate enforcement of Hit Quality Index (HQI) thresholding or operator evaluation, only increases the probability of false-positive classifications. To address such limited access, facilitate inclusive research, and ensure studies are comparable, several open-source online spectral databases have been established, i.e., OpenSpecy (Cowger et al., 2021), The Open Spectral Database (Chalk, 2016), FLOPP-e (De Frond et al., 2021) and many others. With the OpenSpecy providing basic chemometric functionality, while the latter two require the user to implement their own chemometric framework.
During this ECR working group it was identified that inappropriate usage of spectral pre-processing and classification tools can often result in spectral matches that bear little similarity, thereby necessitating aptitude in the evaluation of chemometric classification. Chemometric software, i.e., siMPle (Primpke et al., 2020), Polypython (Levermore et al., 2020), and complex statistical approaches, i.e., deep learning (Brandt et al., 2021), and spectral clustering (Kedzierski et al., 2019), have made significant contributions to the field’s chemometric capabilities. However, the need for further development of chemometric software, with increased statistical certainty and a focus upon inclusivity, remains.
An increasing number of statistical software and packages are used for data analysis (R, Stata, GraphPad, SPSS, etc.), adding to the skillset an ECR is expected to master in a short period of time. Although an understanding of fundamental statistical principles is arguably required for executing any environmental research, the high prevalence of 0s in MP datasets and as count data it often can’t be transformed implies that some general courses for environmental science statistics might not be as useful to MP specialists.
ECRs, who find themselves under pressure to understand and implement complex statistical methods should receive the necessary support and training to do so. These could be run by other MP researchers, with experience and knowledge to share. ECRs across the globe would benefit from peer-to-peer workshops on statistical analyses of real-world MP data. Training can be addressed early on if a discussion on statistical needs is had at the beginning of the appointment. Additionally, ECRs should not rely solely on published articles to inform which statistical approach is preferred, as in many cases the statistical methods are not reported in detail, adding ambiguity, and holding back an invaluable source of scientific knowledge.
Time is a major barrier for ECRs whose research contracts may vary from 6 months to ∼4 years. This barrier may be greater for researchers joining groups without established MP practices, or groups that are not already operating at a particular field location. Obtaining samples from new locations may require obtaining permission for collection and transportation; this can be particularly difficult if the project is abroad. Locations abroad require collaboration with researchers in-country and may need additional consideration with regards to site access, mobility between sites, sample size, shipping, and follow-up campaigns. New relationships take time to establish therefore it is important that these partnerships are already in place before an ECR is employed if such research is to form the core of their appointment.
Time constraints are reflected in the fewer long-term studies looking at the temporal changes in MP contamination across various environments, despite their obvious benefits (such as improved representativeness). This is further confounded by the appropriate frequency to accurately detect these temporal changes.
Spatial and temporal variability in MP contamination has been linked to drivers that affect how particles move through the surrounding environment (Turner et al., 2019). In rivers, for example, this can depend on factors such as increased rainfall or changes in wind strength and direction (Rodrigues et al., 2018). Depending on the study focus, researchers now require access to additional data, e.g., complementary meteorological data, to move beyond presence vs. absence understanding, and towards a more holistic interpretation. This may be difficult, especially as available metadata may need reformatting before its use. Furthermore, data analysis and data management are time intensive, especially while handling short and long-term projects which often extend beyond the ECR’s contract. Notwithstanding the additional consideration that papers from previous employments often need to be written after accepting new contracts, despite no additional time allocated to do so.
Overall, early communication is essential, with PIs discussing where an ECR would need to focus their attention, whether it is in setting up a lab/establishing a piece of equipment or designing an appropriate and representative sampling campaign and subsequently support the ECR in this task as necessary.
Barriers to project delivery such as those highlighted in this article should be discussed on a regular basis not just amongst team members but across research landscapes. Utilising a risk register where ECRs document the types of hurdles they may encounter and how identified risks may impact a projects critical path is recommended. ECRs and PIs can then look at mitigation efforts to reduce the impact of the identified risk, these mitigation interventions can be ECR-led, PI-led or both. Risk registers can be utilised by others in the team without being lost when individual researcher move on. Below are suggested solutions/pathways forwards to further aid discussions and the development of a risk register (Figure 2).
1. Discussions need to be had at various stages of a project regarding the necessary equipment needed to ensure delivery and success. These stages should be when a project is being developed by the PIs, once a project has been awarded, once researchers have been appointed and then at various stages throughout the life span of a project. For example, if access to infrastructure is limited, appropriate mitigation strategies need to be initiated such as visiting equipped labs, collaboration, funding bids, outsourcing to commercial labs etc.
2. Clear training requirements for ECRs early on in their projects could be captured in their risk register—this may involve grant writing courses, paper writing courses. Training can also be through visiting external labs or collaborating with other researchers for mutually beneficial project delivery where both labs benefit from access to infrastructure/facilities/expertise.
3. In order to address the ambiguity and heterogeneity in the gathering, handling, analysing and reporting of data, workshops on statistical methods and the use of specialized software tailored to MP research would be extremely beneficial. These need to be run by more experienced researchers or the developers themselves, however peer-to-peer workshops among ECR networks would also go a long way to supporting knowledge tranfer. Reporting of detailed statistical methods as well as the settings, packages or code used in software and software environments, for example, in a more focused methods appendix, could assist future researchers and promote reproducibility and transparency in research.
4. Reporting of limitations and challenges surrounding specific research activities. This can often be omitted in favour of reporting successes but is crucial to enable a better understanding of where challenges may be encountered, and how these have previously been circumnavigated, to prevent repeated efforts wasting time. This can also be done through networks, workshops, and conferences.
5. Researchers should strive to report their findings in the highest possible level of detail. Even if different metrics for MP concentrations cannot be presented by the authors, available raw data could be used in the future to that end. The current widespread use of supplementary material by many journals can be used for the presentation of large datasets that could not have been reported in the past.
FIGURE 2. Workflow to be used by ECRs, PIs and team members to guide project planning to ensure quality research is delivered and individuals are supported.
Compared with established academics and ECRs in teaching-focussed positions, ECRs in research-focussed positions (PhD students, Research Assistants, Postdoctoral Researchers) have a greater opportunity to 1) ensure that the research of MP groups is able to account for inherent limitations within their aims and objectives, 2) conduct fieldwork that is able to account for variation across the four dimensions outlined previously, and 3) inform “work culture” change beyond the progression of academic knowledge. Especially in such a fast-moving field as MPs, ECRs should be given the opportunity to develop ideas, protocols and networks, instead of simply following existing procedures to enable both their personal and professional development, and also to progress the research field, setting new standards for future research.
The original contributions presented in the study are included in the article/Supplementary Material, further inquiries can be directed to the corresponding authors.
ED: Conceptualization, Project administration, Visualization, Writing–original draft, Writing–review and editing. TS: Writing–original draft, Writing–review and editing, Project administration. YM: Writing–review and editing. AH: Writing–review and editing. QC: Writing–review and editing. JLe: Validation, Writing–review and editing. JLi: Writing–review and editing. AM: Visualization, Writing–original draft, Writing–review and editing. YL: Writing–review and editing. SN: Writing–review and editing. RL: Writing–review and editing. LA: Writing–review and editing. CT: Writing–review and editing. HB: Writing–review and editing. BW: Writing–review and editing. MH: Conceptualization, Funding acquisition, Writing–review and editing. HS: Conceptualization, Funding acquisition, Writing–review and editing. JR: Conceptualization, Funding acquisition, Writing–review and editing. HN: Visualization, Writing–original draft, Writing–review and editing.
The authors declare financial support was received for the research, authorship, and/or publication of this article. This work was funded by a Newton Fund Researcher Links grant ID 2019-RLWK11-10166 under the UK-China partnership. The grant is funded by the UK Department for Business, Energy and Industrial Strategy and the National Natural Science Foundation of China (4191101382) and delivered by the British Council.
Xinghong Wang, Chenxi Wu, Xiong Xiong, Jingxi Li, Maowei Ju, Lang Lin, Lei Mai, Lingling Hu, Shan Zheng, Guang Gao, Yanan Di, Junjie Zhang, and Amy L. Lusher participated in the workshop and contributed in crystallizing the key areas of MP research where ECRs face challenges.
The authors declare that the research was conducted in the absence of any commercial or financial relationships that could be construed as a potential conflict of interest.
All claims expressed in this article are solely those of the authors and do not necessarily represent those of their affiliated organizations, or those of the publisher, the editors and the reviewers. Any product that may be evaluated in this article, or claim that may be made by its manufacturer, is not guaranteed or endorsed by the publisher.
ATR, attenuated-total-reflectance; ECR, early career researchers; FTIR, Fourier transform infrared spectroscopy; HQI, Hit Quality Index; LOD, limit of detection; LOQ, limit of quantification; MP, microplastic; NSFC, Natural Science Foundation of China; OA, open access; PI, principal investigators.
Adams, J. K., Dean, B. Y., Athey, S. N., Jantunen, L. M., Bernstein, S., Stern, G., et al. (2021). Anthropogenic particles (including microfibers and microplastics) in marine sediments of the Canadian Arctic. Sci. Total Environ. 784, 147155. doi:10.1016/j.scitotenv.2021.147155
Agathokleous, E. (2022). Mastering the scientific peer review process: tips for young authors from a young senior editor. J. For. Res. 33 (1), 1–20. doi:10.1007/s11676-021-01388-8
Andrews, E. J., Harper, S., Cashion, T., Palacios-Abrantes, J., Blythe, J., Daly, J., et al. (2020). Supporting early career researchers: insights from interdisciplinary marine scientists. ICES J. Mar. Sci. 77 (2), 476–485. doi:10.1093/icesjms/fsz247
Arole, K., Velhal, M., Tajedini, M., Xavier, P. G., Bardasz, E., Green, M. J., et al. (2023). Impacts of particles released from vehicles on environment and health. Tribol. Int. 184, 108417. doi:10.1016/j.triboint.2023.108417
Asyyed, Z., McGuire, C., Samargandi, O., Al-Youha, S., and Williams, J. G. (2019). The use of Twitter by plastic surgery journals. Plastic Reconstr. Surg. 143 (5), 1092e–8e. doi:10.1097/prs.0000000000005535
Bazeley, P. (2003). Defining 'early career' in research. High. Educ. 45 (3), 257–279. doi:10.1023/a:1022698529612
Brandt, J., Mattsson, K., and Hassellöv, M. (2021). Deep learning for reconstructing low-quality FTIR and Raman Spectra─A case study in microplastic analyses. Anal. Chem. 93 (49), 16360–16368. doi:10.1021/acs.analchem.1c02618
Cabernard, L., Roscher, L., Lorenz, C., Gerdts, G., and Primpke, S. (2018). Comparison of Raman and fourier transform infrared spectroscopy for the quantification of microplastics in the aquatic environment. Environ. Sci. Technol. 52 (22), 13279–13288. doi:10.1021/acs.est.8b03438
Chalk, S. J. (2016). The Open Spectral Database: an open platform for sharing and searching spectral data. J. Cheminformatics 8 (1), 55. doi:10.1186/s13321-016-0170-2
Collier, K. (2021). The 2021 journal citation reports: a continuing evolution in journal intelligence. Clarivate, web of science. Available at: https://clarivate.com/blog/the-2021-journal-citation-reports-a-continuing-evolution-in-journal-intelligence.
Connors, K. A., Dyer, S. D., and Belanger, S. E. (2017). Advancing the quality of environmental microplastic research. Environ. Toxicol. Chem. 36 (7), 1697–1703. doi:10.1002/etc.3829
Cowger, W., Booth, A. M., Hamilton, B. M., Thaysen, C., Primpke, S., Munno, K., et al. (2020). Reporting guidelines to increase the reproducibility and comparability of research on microplastics. Appl. Spectrosc. 74 (9), 1066–1077. doi:10.1177/0003702820930292
Cowger, W., Steinmetz, Z., Gray, A., Munno, K., Lynch, J., Hapich, H., et al. (2021). Microplastic spectral classification needs an open source community: open specy to the rescue. Anal. Chem. 93 (21), 7543–7548. doi:10.1021/acs.analchem.1c00123
De Frond, H., O'Brien, A. M., and Rochman, C. M. (2023). Representative subsampling methods for the chemical identification of microplastic particles in environmental samples. Chemosphere 310, 136772. doi:10.1016/j.chemosphere.2022.136772
De Frond, H., Rubinovitz, R., and Rochman, C. M. (2021). Μatr-FTIR spectral libraries of plastic particles (FLOPP and FLOPP-e) for the analysis of microplastics. Anal. Chem. 93 (48), 15878–15885. doi:10.1021/acs.analchem.1c02549
Dris, R., Gasperi, J., Mirande, C., Mandin, C., Guerrouache, M., Langlois, V., et al. (2017). A first overview of textile fibers, including microplastics, in indoor and outdoor environments. Environ. Pollut. 221, 453–458. doi:10.1016/j.envpol.2016.12.013
Gesamp, (2015). Sources, fate and effects of microplastics in the marine environment: a global assessment. London, UK: Group of Experts on Scientific Aspects of Marine Environmental Protection.
Gesamp, (2023). Sources fate and effects of microplastics in the marine environment: part two of a global assessment. London, UK: The Joint Group of Experts on Scientific Aspects of Marine Environmental Protection.
Green, D. S., Kregting, L., Boots, B., Blockley, D. J., Brickle, P., da Costa, M., et al. (2018). A comparison of sampling methods for seawater microplastics and a first report of the microplastic litter in coastal waters of Ascension and Falkland Islands. Mar. Pollut. Bull. 137, 695–701. doi:10.1016/j.marpolbul.2018.11.004
Hartmann, N. B., Huffer, T., Thompson, R. C., Hassellov, M., Verschoor, A., Daugaard, A. E., et al. (2019). Are we speaking the same language? Recommendations for a definition and categorization framework for plastic debris. Environ. Sci. Technol. 53 (3), 1039–1047. doi:10.1021/acs.est.8b05297
Horton, A. A., Cross, R. K., Read, D. S., Jürgens, M. D., Ball, H. L., Svendsen, C., et al. (2021). Semi-automated analysis of microplastics in complex wastewater samples. Environ. Pollut. 268, 115841. doi:10.1016/j.envpol.2020.115841
Hurley, R., Woodward, J., and Rothwell, J. J. (2018). Microplastic contamination of river beds significantly reduced by catchment-wide flooding. Nat. Geosci. 11 (4), 251–257. doi:10.1038/s41561-018-0080-1
Jenner, L. C., Sadofsky, L. R., Danopoulos, E., and Rotchell, J. M. (2021). Household indoor microplastics within the Humber region (United Kingdom): quantification and chemical characterisation of particles present. Atmos. Environ. 259, 118512. doi:10.1016/j.atmosenv.2021.118512
Johnson, W. P., Rasmuson, A., Ron, C., Erickson, B., VanNess, K., Bolster, D., et al. (2020). Anionic nanoparticle and microplastic non-exponential distributions from source scale with grain size in environmental granular media. Water Res. 182, 116012. doi:10.1016/j.watres.2020.116012
Kedzierski, M., Falcou-Préfol, M., Kerros, M. E., Henry, M., Pedrotti, M. L., and Bruzaud, S. (2019). A machine learning algorithm for high throughput identification of FTIR spectra: application on microplastics collected in the Mediterranean Sea. Chemosphere 234, 242–251. doi:10.1016/j.chemosphere.2019.05.113
Levermore, J. M., Smith, T. E. L., Kelly, F. J., and Wright, S. L. (2020). Detection of microplastics in ambient particulate matter using Raman spectral imaging and chemometric analysis. Anal. Chem. 92 (13), 8732–8740. doi:10.1021/acs.analchem.9b05445
Lusher, A., Bråte, I. L. N., Hurley, R., Iversen, K., and Olsen, M. (2017). Testing of methodology for measuring microplastics in blue mussels (Mytilus spp) and sediments, and recommendations for future monitoring of microplastics (R & D-project). Oslo, Norway: Norwegian Institute for Water Research.
Mani, T., Hauk, A., Walter, U., and Burkhardt-Holm, P. (2015). Microplastics profile along the rhine river. Sci. Rep. 5 (1), 17988. doi:10.1038/srep17988
Margenat, H., Nel, H. A., Stonedahl, S. H., Krause, S., Sabater, F., and Drummond, J. D. (2021). Hydrologic controls on the accumulation of different sized microplastics in the streambed sediments downstream of a wastewater treatment plant (Catalonia, Spain). Environ. Res. Lett. 16 (11), 115012. doi:10.1088/1748-9326/ac3179
Miller, M. E., Kroon, F. J., and Motti, C. A. (2017). Recovering microplastics from marine samples: a review of current practices. Mar. Pollut. Bull. 123 (1-2), 6–18. doi:10.1016/j.marpolbul.2017.08.058
Mintenig, S. M., Löder, M. G. J., Primpke, S., and Gerdts, G. (2019). Low numbers of microplastics detected in drinking water from ground water sources. Sci. total Environ. 648, 631–635. doi:10.1016/j.scitotenv.2018.08.178
Nel, H. A., Dalu, T., and Wasserman, R. J. (2018). Sinks and sources: assessing microplastic abundance in river sediment and deposit feeders in an Austral temperate urban river system. Sci. Total Environ. 612, 950–956. doi:10.1016/j.scitotenv.2017.08.298
Nel, H. A., Naidoo, T., Akindele, E. O., Nhiwatiwa, T., Fadare, O. O., and Krause, S. (2021). Collaboration and infrastructure is needed to develop an African perspective on micro (nano) plastic pollution. Environ. Res. Lett. 16 (2), 021002. doi:10.1088/1748-9326/abdaeb
Nerc, (2018). Evaluation of support to ECRs. Stockport Cheshire, UK: Natural Environment Research Council.
Pisoschi, A. M., and Pisoschi, C. G. (2016). Is open access the solution to increase the impact of scientific journals? Scientometrics 109 (2), 1075–1095. doi:10.1007/s11192-016-2088-x
Prata, J. C., Reis, V., da Costa, J. P., Mouneyrac, C., Duarte, A. C., and Rocha-Santos, T. (2021). Contamination issues as a challenge in quality control and quality assurance in microplastics analytics. J. Hazard. Mater. 403, 123660. doi:10.1016/j.jhazmat.2020.123660
Primpke, S., Cross, R. K., Mintenig, S. M., Simon, M., Vianello, A., Gerdts, G., et al. (2020). Toward the systematic identification of microplastics in the environment: evaluation of a new independent software tool (siMPle) for spectroscopic analysis. Appl. Spectrosc. 74 (9), 1127–1138. doi:10.1177/0003702820917760
Re, V. (2019). Shedding light on the invisible: addressing the potential for groundwater contamination by plastic microfibers. Hydrogeology J. 27 (7), 2719–2727. doi:10.1007/s10040-019-01998-x
Rochman, C. M., Brookson, C., Bikker, J., Djuric, N., Earn, A., Bucci, K., et al. (2019). Rethinking microplastics as a diverse contaminant suite. Environ. Toxicol. Chem. 38 (4), 703–711. doi:10.1002/etc.4371
Rodrigues, M. O., Abrantes, N., Gonçalves, F. J. M., Nogueira, H., Marques, J. C., and Gonçalves, A. M. M. (2018). Spatial and temporal distribution of microplastics in water and sediments of a freshwater system (Antuã River, Portugal). Sci. total Environ. 633, 1549–1559. doi:10.1016/j.scitotenv.2018.03.233
Song, Y. K., Hong, S. H., Eo, S., and Shim, W. J. (2021). A comparison of spectroscopic analysis methods for microplastics: manual, semi-automated, and automated Fourier transform infrared and Raman techniques. Mar. Pollut. Bull. 173, 113101. doi:10.1016/j.marpolbul.2021.113101
Su, L., Deng, H., Li, B., Chen, Q., Pettigrove, V., Wu, C., et al. (2019). The occurrence of microplastic in specific organs in commercially caught fishes from coast and estuary area of east China. J. Hazard. Mater. 365, 716–724. doi:10.1016/j.jhazmat.2018.11.024
Turner, S., Horton, A. A., Rose, N. L., and Hall, C. (2019). A temporal sediment record of microplastics in an urban lake, London, UK. J. Paleolimnol. 61 (4), 449–462. doi:10.1007/s10933-019-00071-7
Uurasjärvi, E., Pääkkönen, M., Setälä, O., Koistinen, A., and Lehtiniemi, M. (2021). Microplastics accumulate to thin layers in the stratified Baltic Sea. Environ. Pollut. 268, 115700. doi:10.1016/j.envpol.2020.115700
Vermeiren, P., Muñoz, C. C., and Ikejima, K. (2016). Sources and sinks of plastic debris in estuaries: a conceptual model integrating biological, physical and chemical distribution mechanisms. Mar. Pollut. Bull. 113 (1-2), 7–16. doi:10.1016/j.marpolbul.2016.10.002
Viaroli, S., Lancia, M., and Re, V. (2022). Microplastics contamination of groundwater: current evidence and future perspectives. A review. Sci. Total Environ. 824, 153851. doi:10.1016/j.scitotenv.2022.153851
Wazne, M., Mermillod-Blondin, F., Vallier, M., Hervant, F., Dumet, A., Nel, H. A., et al. (2023). Microplastics in freshwater sediments impact the role of a main bioturbator in ecosystem functioning. Environ. Sci. Technol. 57 (8), 3042–3052. doi:10.1021/acs.est.2c05662
Weideman, E. A., Perold, V., and Ryan, P. G. (2020). Limited long-distance transport of plastic pollution by the Orange-Vaal River system, South Africa. Sci. Total Environ. 727, 138653. doi:10.1016/j.scitotenv.2020.138653
Zarfl, C. (2019). Promising techniques and open challenges for microplastic identification and quantification in environmental matrices. Anal. Bioanal. Chem. 411 (17), 3743–3756. doi:10.1007/s00216-019-01763-9
Zhang, Q., Zhao, Y., Du, F., Cai, H., Wang, G., and Shi, H. (2020). Microplastic fallout in different indoor environments. Environ. Sci. Technol. 54 (11), 6530–6539. doi:10.1021/acs.est.0c00087
Zobkov, M. B., Esiukova, E. E., Zyubin, A. Y., and Samusev, I. G. (2019). Microplastic content variation in water column: the observations employing a novel sampling tool in stratified Baltic Sea. Mar. Pollut. Bull. 138, 193–205. doi:10.1016/j.marpolbul.2018.11.047
Keywords: representativeness, contamination, comparability, time constraints, networking, limitations
Citation: Danopoulos E, Stanton T, Ma Y, Horton AA, Chen Q, Levermore JM, Li J, McGoran AR, Lai Y, Nolte S, Li R, Alvarez Barrantes L, Tu C, Beaumont H, Williams B, Hartl MGJ, Shi H, Rotchell JM and Nel HA (2023) Insights into technical challenges in the field of microplastic pollution through the lens of early career researchers (ECRs) and a proposed pathway forward. Front. Earth Sci. 11:1271547. doi: 10.3389/feart.2023.1271547
Received: 02 August 2023; Accepted: 01 November 2023;
Published: 17 November 2023.
Edited by:
Stefano Viaroli, University of Pisa, ItalyCopyright © 2023 Danopoulos, Stanton, Ma, Horton, Chen, Levermore, Li, McGoran, Lai, Nolte, Li, Alvarez Barrantes, Tu, Beaumont, Williams, Hartl, Shi, Rotchell and Nel. This is an open-access article distributed under the terms of the Creative Commons Attribution License (CC BY). The use, distribution or reproduction in other forums is permitted, provided the original author(s) and the copyright owner(s) are credited and that the original publication in this journal is cited, in accordance with accepted academic practice. No use, distribution or reproduction is permitted which does not comply with these terms.
*Correspondence: Evangelos Danopoulos, ZXYubnRhbm9wb3Vsb3NAZ21haWwuY29t; Chen Tu, Y3R1QGlzc2FzLmFjLmNu
‡Present Address: Chen Tu, Institute of Soil Science, Chinese Academy of Sciences, Nanjing, China
Disclaimer: All claims expressed in this article are solely those of the authors and do not necessarily represent those of their affiliated organizations, or those of the publisher, the editors and the reviewers. Any product that may be evaluated in this article or claim that may be made by its manufacturer is not guaranteed or endorsed by the publisher.
Research integrity at Frontiers
Learn more about the work of our research integrity team to safeguard the quality of each article we publish.