- 1Abdullah Alrushaid Chair for Earth Science Remote Sensing Research, Geology and Geophysics Department, College of Science, King Saud University, Riyadh, Saudi Arabia
- 2Geology Department, Faculty of Science, Assiut University, Assiut, Egypt
Water scarcity is developing in the Middle East as a result of the region’s growing population and tremendously advanced agricultural and industrial sectors. Saudi Arabia is the Middle East country with the highest water consumption, so there is an urgent need to take action, and new technology advancements in geophysical measurements allow for the monitoring of groundwater. Wadi Ar-Ramah is one region that has witnessed significant agricultural expansion as well as a serious over-exploitation of the groundwater resources that are available there. Depletion rate in groundwater of the Wadi Ar-Ramah basin at eastern Saudi Arabia was determined for the time interval of 04/2002 to 12/2021 using a combination of gravity data from the Gravity Recovery and Climate Experiment (GRACE) mission and results of land surface models. The findings are: 1) the average yearly rainfall rate was computed at 87.7 mm yr−1 over the Wadi Ar-Ramah; 2) the terrestrial water storage variation (ΔTWS) was computed at −1.216 ± 0.013 cm yr−1; 3) the GLDAS-derived soil moisture (ΔSMS) was minimal at −0.32 ± 0.025 mm yr−1; 4) the GRACE-derievd groundwater decreasing rate was calculated at 1.212 ± 0.012 cm yr−1; 5) the relief of the ground surface is producing northeasterly streams that carry the minimal surface water to the east; 6) our integrated method provides a repeatable and cost-effective approach.
1 Introduction
At least 2 billion people rely on groundwater as the world’s largest and most dependable source of freshwater, with the majority of them living in arid and dry zones (Taylor et al., 2013; Jasechko et al., 2017; Sun et al., 2022). Globally, groundwater is the source of one-third of all freshwater exploitation, supplying an estimated 36%, 42%, and 27% of the water used for domestic, agricultural, and industrial purposes, respectively (Döll et al., 2012). In recent decades, the increased use of groundwater for human consumption and agriculture has led to a decline in groundwater levels in many regions of the globe (Castellazzi et al., 2016; Narany et al., 2017; Cui et al., 2020). As a result, aquifers have been depleting, which has caused a number of environmental and geological problems, including land subsidence and the destruction of wetlands (Dalin et al., 2017). Improving groundwater management is necessary to ensure its sustainable usage and reasonable expansion. Saudi Arabia is regarded as one of the driest countries in the world, despite the fact that 99.84% of the country’s population have access to drinkable water (General Authority of Statistics, 2019). Saudi Arabia only has 89 m3/cap./year of absolute water scarcity, compared to 500 m3/cap./year worldwide. (Water Challenges in KSA, 2021).
In arid and semiarid regions, it is crucial to evaluate groundwater resources, hydrogeologic settings of aquifers, and recharge and/or discharge (natural or anthropogenic) of these systems in order to establish effective management scenarios for these resources and the economic development of such locations (Simmers, 1997). Much of the groundwater in dry regions is used for irrigation, which accounts for roughly 70% of the world’s water withdrawal and 90% of its consumption (Siebert et al., 2010; Gerten et al., 2020).
Groundwater, surface water (dams, reservoirs, rivers, and lakes), snow, soil moisture, plant canopy water, and glaciers all contribute to the total amount of water stored on and below the land’s surface, which is referred to as “terrestrial water storage” (TWS) (Zhu et al., 2021). Changes in the TWS can efficiently indicate the equilibrium or imbalance of water fluxes that are substantially impacted by regional climate conditions (Zhu et al., 2021). Recent global warming has increased TWS variability in many dry and semi arid settings (Wang et al., 2018; Chang et al., 2020; Shugar et al., 2021; Yang, et al., 2022). As a result, correct TWS calculation is critical for understanding regional hydrological processes, which allow effective basin-scale water resource allocation and management.
The goal of groundwater management (Ahamed, et al., 2021) is to develop methods for accurately estimating and monitoring changes in GWS across temporal and spatial scales. Several huge aquifers have been significantly depleted in several regions throughout the world as a result of human activity; these regions include North Africa, South and Central Asia, the Middle East, North China, Australia, and North America (Rodell, et al., 2009; Sun et al., 2022). Historically, scientists have attempted to predict GWS changes using in situ observation (such as monitoring changes in groundwater level), the water balance approach, and hydrological modeling techniques (Ahamed et al., 2021; Sun et al., 2022). Although these techniques have been employed in a number of earlier research, they are constrained by real-world constraints including the dispersed nature of observation wells. Therefore, it remains a significant problem on a global scale to manage refined groundwater resources in a reliable and timely manner, as required.
Several strategies, including physical and chemical methods, in situ measuring, satellite remote sensing, and modeling techniques, have been used to estimate the TWS and assess the recharge, discharge, and depletion rates of aquifer systems, as well as to investigate the connectivity of their subbasins (Cox and Chao, 2002; de Vries and Simmers, 2002; Cazenave and Nerem, 2002; Scanlon et al., 2016; Ramillien et al., 2005; Rodell et al., 2009). These methods are difficult to use on a regional scale, and their results are sometimes suspicious due to the scarcity of datasets required for implementation, as well as the substantial efforts and resources required to obtain them. Satellite remote sensing only captures information on surface water bodies, and the precision of model parameters influences numerical modeling. Fortunately, the implementation of the Gravity Recovery and Climate Experiment (GRACE) and GRACE Follow-On (GRACE-FO) can address these shortcomings and limitations by providing a new remote sensing technique for monitoring monthly TWS (Chen et al., 2016).
As a twin satellite-to-satellite monitoring mission, the GRACE mission started in March 2002 enables us to compute the average and time-varying components of the Earth’s gravitational field (Tapley et al., 2004). Hydrological signals dominate the gravitational field’s change, hence GRACE data can be used to correctly quantify TWS fluctuations (Ahmed, et al., 2014; Tapley et al., 2019). GRACE can detect water storage changes to within 1–1.5 cm worldwide (Wahr et al., 2004), despite having a poorer spatial resolution (300 km). The GRACE mission terminated in October of 2017, however the mission that replaced it, which began operations in May 2018, is still delivering consistent data for hydrological uses (Landerer et al., 2020).
Because GRACE can be utilized to fill in the gaps in hydrological monitoring data, some studies have combined GRACE with other data to estimate depletion and/or recharge rates caused by climate and/or human activities in Saudi Arabia and other regions (e.g., Fallatah et al., 2017; 2019; Mohamed, 2019; Mohamed, 2020a; Mohamed, 2020b; Mohamed, 2020c; Mohamed et al., 2021; Mohamed and Gonçalvès, 2021; Taha et al., 2021; Mohamed, 2022a; Mohamed, 2022b; Mohamed, 2022c; Mohamed, 2023a; Mohamed, 2023b; Othman et al., 2022; Alshehri and Mohamed, 2023). Other satellite and aerial geophysical field datasets have been utilized to explore continental crustal properties (Mohamed and Al Deep. 2021), Curie point depth and magma chamber geometry (Mohamed et al., 2022d), and subsurface structures controlling the mineralization zones (Mohamed et al., 2022e; Mohamed et al., 2023).
More accurate estimate of the depletion rate of the Wadi Ar-Ramah basin was obtained in this work by combining GRACE data with results from land surface models (LSMs) for the time period of 04/2002 to 12/2021. The objectives of this study are to: 1) use improved state-of-the-art solutions, the global mass concentration solutions (mascons) covering the time of April 2002 to December 2021; 2) using outputs from three versions of the Global Land Data Assimilation System (GLDAS) to estimate the groundwater storage (ΔGWS) from the GARCE-derived ΔTWS, and define its controlling factors; and 3) assess if the basin is in steady-state conditions or is being exhausted, and, if the latter, whether this depletion is due to natural and/or anthropogenic factors.
2 Study area
With a length of about 600 km, Wadi Ar-Ramah is a long river valley on the Arabian Peninsula (370 mi; Figure 1). The wadi begins near Medina in Jibl al Abyad (the White Mountain), where it is mostly dry and partially dammed by expanding sand dunes. A number of smaller wadis, including Murghala Wadi, Mohalla Wadi, Jarir Wadi, and Jifn Wadi, are connected to it as it flows towards the north-east. In Al-Qassim Province close to Buraidah, it comes to an end in the Thuayrat Dunes of the ad-Dahna Desert. After there, the wadi disappears beneath the sand dunes, where it is known as Mistewy Wadi. It appears as Wadi al-Batin on the other side of the sand dunes, which runs about 425 km north to define Kuwait’s western boundary. It finally drains into the Arabiani Gulf (Edwards, et al., 1970; Helen Chapin Metz, 1992; Wadi al-Ramah, 2011).
The valley is wide because it was previously a prominent river valley. It was originally a 1,200-km-long river that flowed from Medina to the Arabian Gulf roughly 10,000 years ago (according to Dr. Abdullah Al-Musnad). Drought conditions and sand migration at Althwairat and Dahna caused the valley’s route to be divided into three sections: Wadi al-Ramah, the longest at 600 km, Wadi Aloddi, at 45 km, and Wadi al-Batin, at 450 km. According to geological studies, the Wadi Ar-Ramah flows fully three times per hundred years. Recently, it flowed in the years of (1945, 1982, 1987, 2004, 2008, and 2018). The river valley flooded for afew days in 1818, 1838, 1987, and 2008. The wadi overflowed in 1838, generating a lake with an area of 520 km2 that continued 2 years and attracted rare water birds to the region (alriyadh.com; Wadi al-Ramah, 2011).
The research region contains two surface rock units representing different geologic time periods, namely, the Cambro-Ordovician and Quaternary. Except for the eastern and northeastern parts of the study area, which are covered by sand dunes, the Saq Sandstone aquifer outcrops on both sides of the wadi. This Cambrian-Ordovician sandstone has several colors and is interbedded with jointed quartz (Bramkamp et al., 1963; Sowayan and Allayla, 1989). This Sandstone outcrop stretches 170 kilometers south of the wadi and north across the Syrina border. It has a surface area of around 65,000 km2. The Saq Sandstone is the country’s oldest sedimantary unit, and it unconfromably overlies the underlying crystalline rocks. The Tabuk Formation, which is represented by alternating sandstone and shale elements, is likewise an important water-bearing reservoir. Eolian and fluvial deposits make up the more recent Quaternary sediments. Eolian sediments are made up of many forms of undulating sand sheets. These sands impact the width of the wadi, which is around 1 km in the eastern part near the downstrem compared to nearly 14 km in the western poerion of the study area (Sowayan and Allayla, 1989). The wadi channed and its floodplain are occupied by fluvial deposits. The Saq Sandstone is the main imprtnat aquifer in the research area, spreading for long distances north and south. This aquifer becomes cofined to the east with good water quality, however higher salinity values occur locally in the study region (Sowayan and Allayla, 1989).
3 Data and methods
3.1 GRACE mascon data
After the success of the GRACE mission, the National Aeronautics and Space Administration (NASA) and the German Research Centre for Geosciences (GFZ) decided to launch a new project to continue the research (Watkins et al., 2015). This new mission is called GRACE Follow-On (GRACE-FO). It was the follow-up to the original mission, which was in orbit from 2002 to 2017. It refines the previous model’s successes and pilots cutting-edge technology that significantly enhances the accuracy of the measuring apparatus. High-resolution, monthly global models of the Earth’s gravitational field were initiated during GRACE, and this adds data to those models.
However, besides mass concentration (Mascon) solutions, spherical harmonics (SH) solutions are also available for the GRACE/GRACE-FO data (Soltani et al., 2021). For the first 10 years of GRACE data, the SH solutions were the gold standard since they were used to parameterize the earth’s gravity field model using global SH basis functions (Landerer and Swenson, 2012; Wei et al., 2021). The GRACE/GRACE-FO SH solutions are made public every month by the Scientific Data System, which is made up of three centers: the Jet Propulsion Laboratory (JPL), the Center for Space Research at the University of Texas (CSR), and the GFZ. Parameterizing the earth gravity field model using mass concentration blocks in mascon solutions makes it much simpler to incorporate geophysical constraints and has the potential to enhance the gravity information for research into Earth’s surface processes (Scanlon et al., 2016; Save et al., 2016; Yang et al., 2022). This research makes use of three different time-variable gravity mascon solutions from the three processing centers. Datasets from the JPL, the CSR, and the Goddard Space Flight Center (GSFC) at NASA feature mascon products. All these mascon products were utilized to calculate the changes in TWS for the Wadi Ar-Ramah basin. Data was available from April 2002 through December 2021. This included 175 months from GRACE (01/2002 through 06/2017) and 41 months from GRACE-FO (06/2018 to 12/2021). Data gap between GRACE and GRACE-FO of 11 months could not be filled because of the satellites’ termination. This research evaluated the TWSA measurements taken between 2002 and 2021 using data from the mascon CSR, GSFC, and JPL solutions. The spatial averaging was used to create a monthly TWS time series of within the basin. By averaging the TWSA from three solutions to generate the TWSA, the signal-to-noise ratio was improved (Sakumura et al., 2014; Wang et al., 2021). The TWS trend were determined using the slope. In the next step, the errors in the trend values were then estimated.
3.2 GLDAS
We have used the most recent GLDAS-2.1 (Rodell et al., 2004) Community Land Model (CLM), the Variable Infiltration Capacity, and Noah model results (https://disc.gsfc.nasa.gov) to determine SWE, CWS, and SMS for the period of 2002–2021 with a spatial resolution of 1° degree. The SMS refers to the total amount of water present in all soil profiles, including those amount in 0–0.1 m, 0.1–0.4 cm, 0.4–1 m, and 1-2 m underground, and root zone SM. Based on the deviation from the 2004–2009 mean, we converted the GLDAS data into an anomalous format.
3.3 Rainfall data
The mass fluctuations within geological formations are influenced by a number of reasons, one of which is rainfall. Precise point rainfall readings can be obtained using rain gauges, but there is a lack of continuous monitoring stations in the sparsely populated desert regions of Wadi Ar-Ramah. So, this research made use of satellite monitoring of the rainfall. The Tropical Rainfall Measuring Project (TRMM) is a cooperative space mission designed to measure rainfall for climate research (Kummerow, 1998; Huffman et al., 2007).
Using precipitation records, monthly total precipitation images were generated. Second, we averaged the rainfall rates at each grid point within the research area to produce a monthly rainfall time series. Finally, the region’s AAR (average annual rainfall; see Figure 3) was calculated. Finally, the impact of rainfall on GWS variations over the research area was analyzed using the TRMM data.
3.4 Landsat images
Landsat 4, 5, 7, 8, and 9 multi-temporal satellite images were downloaded and processed for the study region to assess land-use changes caused by human activities across the research period. The images were also used to demonstrate agricultural expansion and community growth in the Wadi Ar-Ramah.
4 Result and discussion
In this study, we integrate GRACE and GLDAS data to get a comprehensive understanding of the groundwater potentialities of Wadi Ar-Ramah in Saudi Arabia’s eastern region.
4.1 Water storage changes from GRACE
An examination of Figure 2 reveals a decline in AAR started in 2006 and continues till 2017 with AAR values varying between 48.38 and 93.3 mm/yr and a general decrease trend of −0.89 mm/yr throughout the time interval 2006–2017. The AAR rate increases to 193.3 mm/yr withing the year 2018 and decreases to 83.89 mm/yr in the year 2019. The average spatial distribution of the AAR-derived TRMM data over the study region shows increasing in the rainfall from low values of ∼40.6 mm/yr at the southwestern part of the Wadi to higher values at the eastern part of it reaching up to 147.3 at its downstream. The study area has an AAR rate of 87.7 mm/yr throughout the study period. The decreasing trend of rainfall between the years of 2006 and 2017 might be one of the causes of the higher TWS depletion for the Wadi. We cannot compare 2018 due to the absence of TWS anomalies induced by the termination of GRACE observations between July 2017 and May 2018.
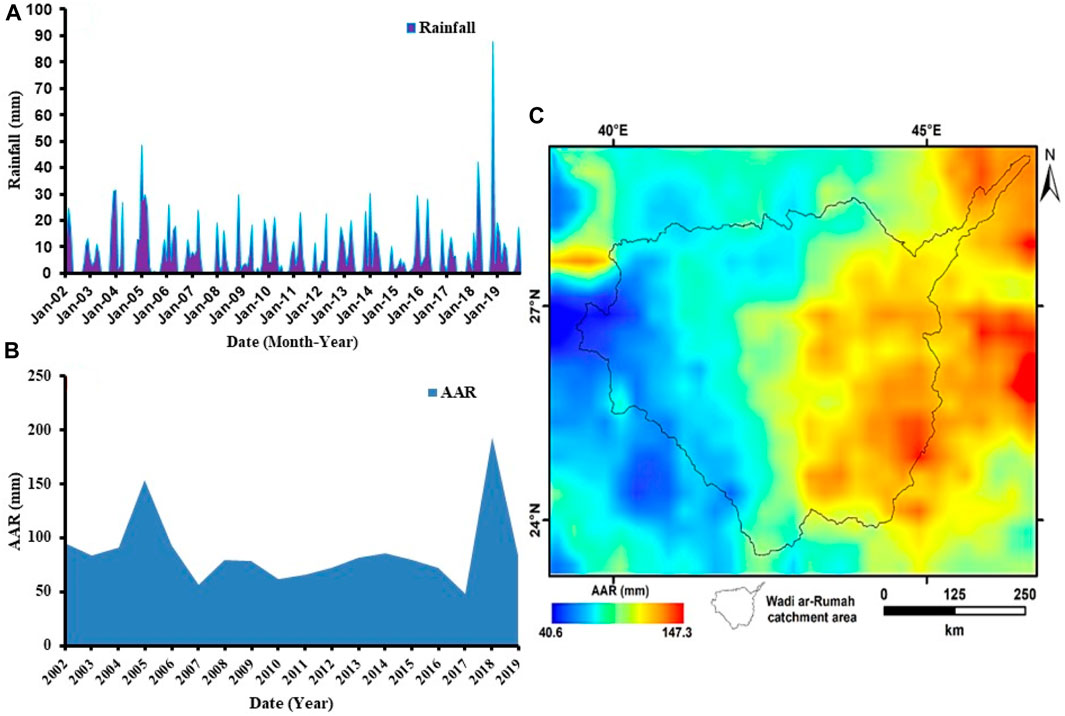
FIGURE 2. Monthly rainfall time series (A), Temporal AAR variation time series (B), and the spatial variation of the AAR (C) over the study area.
Here, we describe the spatial distributions of GRACE’s detected signals of changes in ΔTWS. The spatial distribution of ΔTWS trends between 2002 and 2021, as computed from CSR and GSFC solutions, is shown in Figure 3. Witnessing of this Figure 3 shows that high decreasing trends of TWS to about −2.05 cm/yr using CSR mascon solution; and −2.68 cm/yr using GSFC mascon solution were mainly found in the middle and northwestern regions of the Wadi. The TWS trend values increase northeast and southwestwards, attaining values ranging from −0.07 to + 0.009 cm/yr when employing the GSFC and CSR mascon solutions, respectively.
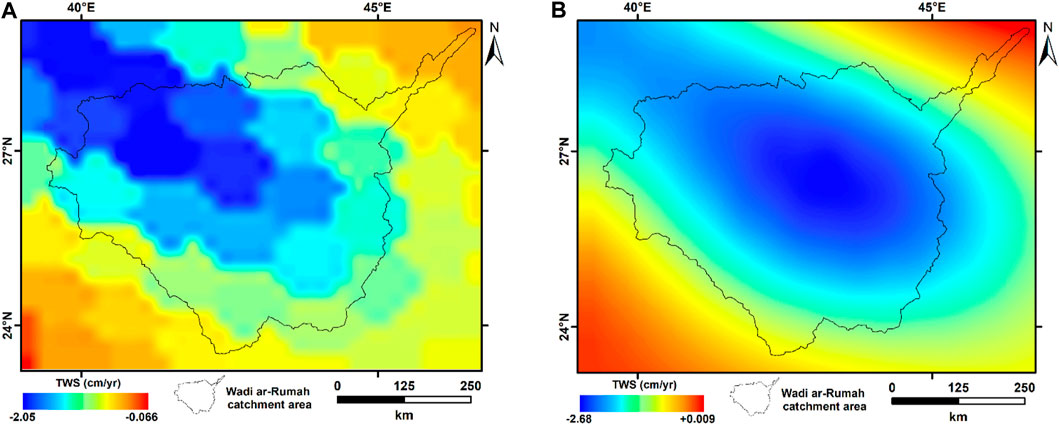
FIGURE 3. GRACE-derived TWS spatiotemporal fluctuations for the research area utilizing CSR (A) and GSFC (B) mascon solutions.
Figure 4 depicts the variability in monthly average storage anomalies of GRACE-estimated ΔTWS over the Wadi. The degree to which two variables are linearly related is measured by a statistic called the correlation coefficient. The higher the correlation coefficient R among the different three solutions, the stronger the averaging will be. The correlation coefficients for the three mascon solutions range from 0.97 to 0.99, indicating that they share similar patterns (Figure 4). Based on the monthly TWS estimates, the annual mean ΔTWS fluctuations were examined in further detail. The ΔTWS was decreased at rate of −1.216 ± 0.013 cm/year in equivalent water height over the entire Wadi Ar-Ramah between 2002 and 2021.
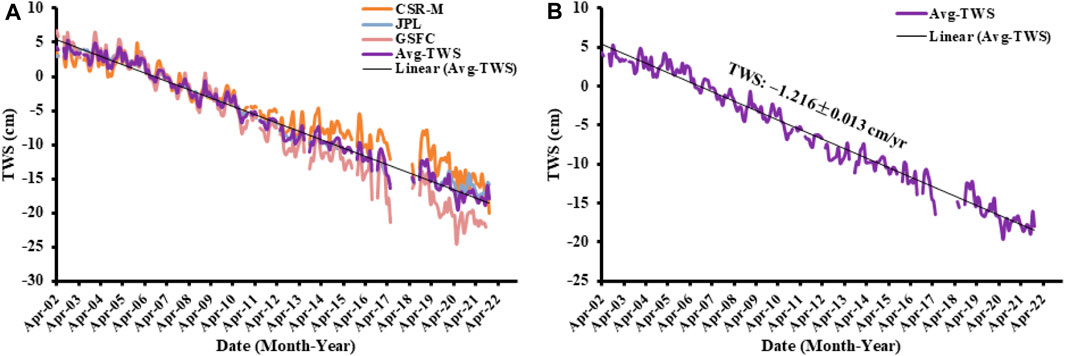
FIGURE 4. Time series of the TWS (A) and averaging TWS trend (B) from 04/2002 to 12/2021 for the Wadi.
Three different GLDAS models were utilized to calculate the ΔSMS; Figure 5 displays the average of these estimates for Wadi Ar-Ramah. The amplitude of seasonal SM anomalies is around 10 cm. SMS values from the three models vary from 0.2 to 0.5 mm/yr, with an average of −0.32 ± 0.025 mm/yr due to the relatively low rainfall rates experienced by the Wadi Ar-Ramah over the research period. Consequently, the contribution of SMS to the variability of terrestrial water storage is often minimal on a regional scale.
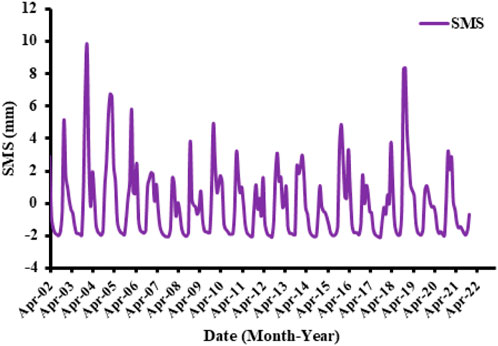
FIGURE 5. Temporal variations of the GLDAS-derived SMS for the Wadi Ar-Ramah from 04/2002 to 12/2021.
As was already mentioned, however, GRACE can’t tell the difference between anomalies brought on by distinct TWS compartments. Consequently, GLDAS models were used to determine the non-groundwater components reflected in SMS fluctuations.
Several authors have used Eq. 1 to determine groundwater storage fluctuations (e.g., Rodell et al., 2009; Tiwari et al., 2009; Mohamed et al., 2017). Estimates ΔGWS were obtained by deducting the variations in ΔSMS, from GRACE-derived ΔTWS.
As can be seen in the following formula, TWS is comprised of two distinct parts:
Variations in the other components represnted by CWS, SWS, and SWE are ignored due to the absence of trends in their respective time series.
The time series of GRACE-derived GWS reach their peaks in May and June, and their troughs in October and November. Their temporal variations show similar patterns as those of the TWS, due to the minimal contribution from the non-groundwater components in Wari Ramah (Figure 6). The calculated groundwater depletion of Wadi Ar-Ramah varying between −1.418 ± 0.019 and −0.978 ± 0.018 cm/yr using the three mascon solutions with an average value of −1.212 ± 0.012 cm/yr during the entire period (Table 1).
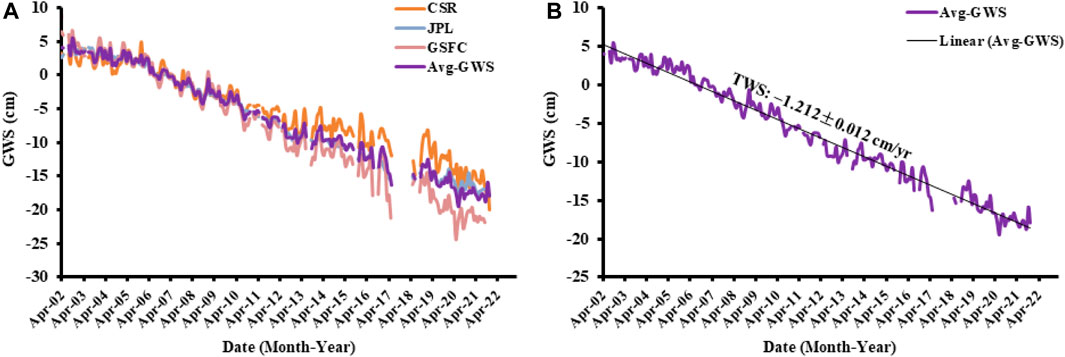
FIGURE 6. Time series of the GWS (A) and averaging GWS trend (B) from 04/2002 to 12/2021 for the Wadi.
4.2 Sediment thickness
NOAA’s National Geophysical Data Center provided data on sediment thickness (Divins, 2003). In the southwestern part of the Wadi Ar-Ramah, the sedimentary cover reaches a thickness of less than 10 m (Figure 7) in the lowlands of the streams that dissect the basement crystalline and volcanic rocks. Northeastwards, the sediment thickness increases to reach higher sediment thickness of more than 3,500 m at the confluence of the streams that continues to more 4,000–6,000 m at the main channel in the downstream of the Wadi. This may provide evidence for the existence of a large fluid reserve in the Wadi around its main long channel.
4.3 Watershed analysis
Figure 8 is a topographic map generated from the ETOPO1 Global Relief Model depicting the stream networks of Wadi Ar-Ramah. The elevation varies dramatically from more than 1,000 m at the southwestern parts of the Wadi over the mountainous region of the Arabian Nubian Shield to less than 500 m at the beginning of the sedimentary region close to the shield. It continues down to less than 300 m northeast of the Hafar Al Batin region, close to the mainstream. Streams arise in the southwestern portion of the Wadi and flow downstream toward the east. Given the Wadi’s low average annual precipitation rate of 87.8 mm, the Wadi may receive a minimum recharge rate. This is demonstrated by the Wadi’s heavy groundwater extraction rate of −1.216 ± 0.013 cm/year during the study period.
4.4 Anthropogenic activities
Human activity has been found to induce changes in land/land cover, as shown by the outputs of Landsat datasets that contained satellite imagery from multiple historical periods (Figure 9). Landsat’s 1984 view of the Wadi Ar-Ramah region shows signs of farming and aqricultural activities that increses across the time to large areas occupied by the these activities. In addition, the building of new communities has consumed more water and adding a stress on the groundwater potential. Deserts of the wadi are gradually becoming dotted with green in later images. A center-pivot irrigation system waters each green dot, which represents a farm field. For the purpose of watering the fruit, vegetable, and wheat crops, water is piped up from a deep underground reservoir. The reservoir is known as Al Sag. Water in this aquifer is estimated to have been gathered between 12,000 and 30,000 years ago, with just a small amount being replenished in the present day. Mohamed et al. (2022a) assessed the groundwater depletion of the Saq aquifer to be −10.16 ± 0.39 mm/yr between 04/2006 and 06/2016 as a result of intensive groundwater withdrawal for residential and agricultural purposes.
5 Conclusion
Based on an analysis of rainfall data for the Wadi Ar-Ramah between April 2002 and December 2021, the annual precipitation rate is 87.7 mm. Wadi Ar-Ramah Basin has been subjected to extensive groundwater extraction since 1990. The integrated results from GRACE and GLDAS indicate that the groundwater resource is declining at a rate of −1.212 ± 0.012 cm/year. The identification of change from Landsat images has revealed significant land use/land cover changes since 1985. The substantial rate of groundwater extraction is not mitigated by the small amount of surface water derived from precipitation. In the aquifer’s downstream section, the higher sediment succession of 4,000–6,000 m is preserving vast quantities of groundwater. Using the Wadi Ar-Ramah Basin, we demonstrated that GRACE data can be used to estimate mass variations across enormous hydrologic basins, a normally challenging endeavor. Despite the unavoidable challenges, the current research advances our knowledge of the groundwater variability from both a present and a future perspective. Policymakers may use this information to increase groundwater-dependent agriculture and community development. A continuous monitoring program using the most recent GARCE data, meteorological models, and ground-based data should be carried out to evaluate the water storage dynamics in the future.
Data availability statement
The raw data supporting the conclusion of this article will be made available by the authors, without undue reservation.
Author contributions
All authors listed have made a substantial, direct, and intellectual contribution to the work and approved it for publication.
Funding
The authors extend their appreciation to Abdullah Alrushaid Chair for Earth Science Remote Sensing Research for the funding.
Conflict of interest
The authors declare that the research was conducted in the absence of any commercial or financial relationships that could be construed as a potential conflict of interest.
Publisher’s note
All claims expressed in this article are solely those of the authors and do not necessarily represent those of their affiliated organizations, or those of the publisher, the editors and the reviewers. Any product that may be evaluated in this article, or claim that may be made by its manufacturer, is not guaranteed or endorsed by the publisher.
References
Ahamed, A., Knight, R., Alam, S., Pauloo, R., and Melton, F. (2021). Assessing the utility of remote sensing data to accurately estimate changes in groundwater storage. Sci. Total Environ. 807, 150635. doi:10.1016/j.scitotenv.2021.150635
Ahmed, M., Sultan, M., Wahr, J., and Yan, E. (2014). The use of GRACE data to monitor natural and anthropogenic induced variations in water availability across Africa. Earth-Science Rev. 136, 289–300. doi:10.1016/j.earscirev.2014.05.009
Alshehri, F., and Mohamed, A. (2023). Analysis of groundwater storage fluctuations using GRACE and remote sensing data in wadi as-sirhan, northern Saudi Arabia. Water 15, 282. doi:10.3390/w15020282
Bramkamp, R. A., Ramirez, L. F., Brown, G. F., and Pocock, A. E. (1963). Geology of the wadi as-rumah quadrangle U.S. Geological survey. Washington, DC, USA: Miscellaneous Geologic Investigations.
Castellazzi, P., Martel, R., Galloway, D., Longuevergne, L., and Rivera, A. (2016). Assessing groundwater depletion and dynamics using GRACE and InSAR: potential and limitations. Ground Water 54, 768–780. doi:10.1111/gwat.12453
Cazenave, A., and Nerem, R. S. (2002). Redistributing Earth’s mass. Science 297, 783–784. doi:10.1126/science.1074593
Chang, L. L., Yuan, R. Q., Gupta, H. V., Winter, C., and Niu, G. Y. (2020). Why is the TerrestrialWater storage in dryland regions declining? A perspective based on gravity Recovery and climate experiment satellite observations and Noah land surface model with multiparameterization schemes model simulations. Water Resour. Res. 56, e2020WR027102. doi:10.1029/2020wr027102
Chen, J., Famiglietti, J. S., Scanlon, B. R., and Rodell, M. (2016). Groundwater storage changes: present status from GRACE observations. Surv. Geophys 37, 397–417. doi:10.1007/s10712-015-9332-4
Cox, C. M., and Chao, B. F. (2002). Detection of a large-scale mass redistribution in the terrestrial system since 1998. Science 297, 831–833. doi:10.1126/science.1072188
Cui, Y., Liao, Z., Wei, Y., Xu, X., Song, Y., and Liu, H. (2020). The response of groundwater level to climate change and human activities in baotou city, China. Water 12, 1078. doi:10.3390/w12041078
Dalin, C., Wada, Y., Kastner, T., and Puma, M. J. (2017). Groundwater depletion embedded in international food trade. Nature 543, 700–704. doi:10.1038/nature21403
de Vries, J. J., and Simmers, I. (2002). Groundwater recharge: an overview of process and challenges. Hydrogeology Journal 10, 5–17. doi:10.1007/s10040-001-0171-7
Divins, D. (2003). Total sediment thickness of the world’s oceans and marginal seas. Boulder, CO, USA: NOAA National Geophysical Data Center.
Döll, P., Hoffmann-Dobrev, H., Portmann, F., Siebert, S., Eicker, A., Rodell, M., et al. (2012). Impact of water withdrawals from groundwater and surface water on continental water storage variations. J. Geodyn. 59, 143–156. doi:10.1016/j.jog.2011.05.001
Edwards, I. E. S., Gadd, C. J., and Hammond, N. G. L. (1970). The cambridge ancient history. Cambridge: Cambridge University Press, 62.
Fallatah, O. A., Ahmed, M., Cardace, D., Boving, T., and Kanda, A. S. (2019). Assessment of modern recharge to arid region aquifers using an integrated geophysical, geochemical, and remote sensing approach. J. Hydrol. 569, 600–611. doi:10.1016/j.jhydrol.2018.09.061
Fallatah, O. A., Ahmed, M., Save, H., and Akanda, A. S. (2017). Quantifying temporal variations in water resources of a vulnerable middle eastern transboundary aquifer system. Hydrol. Process 31, 4081–4091. doi:10.1002/hyp.11285
General Authority of Statistics (2019). Available at: https://www.stats.gov.sa/en
Gerten, D., Heck, V., Jägermeyr, J., Bodirsky, B. L., Fetzer, I., Jalava, M., et al. (2020). Feeding ten billion people is possible within four terrestrial planetary boundaries. Nat. Sustain. 3 (3), 200–208. doi:10.1038/s41893-019-0465-1
Helen Chapin Metz (1992). “"Topography and natural regions",” in Saudi Arabia: a country study (Saudi Arabia: U.S. Library of Congress).
Huffman, G. J., Bolvin, D. T., Nelkin, E. J., Wolff, D. B., Adler, R. F., Gu, G., et al. (2007). The TRMM Multisatellite Precipitation Analysis (TMPA): quasi-global, multiyear, combined-sensor precipitation estimates at fine scales. J. Hydrometeorol. 8, 38–55. doi:10.1175/JHM560.1
Jasechko, S., Perrone, D., Befus, K. M., Bayani Cardenas, M., Ferguson, G., Gleeson, T., et al. (2017). Global aquifers dominated by fossil groundwaters but wells vulnerable to modern contamination. Nat. Geosci. 10, 425–429. doi:10.1038/ngeo2943
Kummerow, C. (1998). Beamfilling errors in passive microwave rainfall retrievals. J. Appl. Meteorol. 37, 356–370. doi:10.1175/1520-0450(1998)037<0356:BEIPMR>2.0.CO;2
Landerer, F. W., Flechtner, F. M., Save, H., Webb, F. H., Bandikova, T., Bertiger, W. I., et al. (2020). Extending the global mass change data record: GRACE follow-on instrument and science data performance. Geophys. Res. Lett. 47, 12. doi:10.1029/2020gl088306
Landerer, F. W., and Swenson, S. C. (2012). Accuracy of scaled GRACE terrestrial water storage estimates. Water Resour. Res. 48, 4531. doi:10.1029/2011wr011453
Mohamed, A. (2019). Hydro-geophysical study of the groundwater storage variations over the Libyan area and its connection to the Dakhla basin in Egypt. J. Afr. Earth Sci. 157, 103508. doi:10.1016/j.jafrearsci.2019.05.016
Mohamed, A., Abdelrady, A., Alarifi, S. S., and Othman, A. (2023a). Geophysical and remote sensing assessment of Chad’s groundwater resources. Remote Sens. 15, 560. doi:10.3390/rs15030560
Mohamed, A., Abdelrady, M., Alshehri, F., Mohammed, M. A., and Abdelrady, A. (2022e). Detection of mineralization zones using aeromagnetic data. Appl. Sci. 12, 9078. doi:10.3390/app12189078
Mohamed, A., Abdelrahman, K., and Abdelrady, A. (2022a). Application of time-variable gravity to groundwater storage fluctuations in Saudi Arabia. Front. Earth Sci. 10, 873352. doi:10.3389/feart.2022.873352
Mohamed, A., Al Deep, M., Abdelrahman, K., and Abdelrady, A. (2022d). Geometry of the magma chamber and curie point depth beneath Hawaii Island: inferences from magnetic and gravity data. Front. Earth Sci. 10, 847984. doi:10.3389/feart.2022.847984
Mohamed, A., and Al Deep, M. (2021). Depth to the bottom of the magnetic layer, crustal thickness, and heat flow in Africa: inferences from gravity and magnetic data. J. Afr. Earth Sci. 179, 104204. doi:10.1016/j.jafrearsci.2021.104204
Mohamed, A., Al Deep, M., Othman, A., Taha, A. L., Alshehri, F., and Abdelrady, A. (2022b). Integrated geophysical assessment of groundwater potential in southwestern Saudi Arabia. Front. Earth Sci. 10, 937402. doi:10.3389/feart.2022.937402
Mohamed, A., Faye, C., Othman, A., and Abdelrady, A. (2022c). Hydro-geophysical evaluation of the regional variability of Senegal’s terrestrial water storage using time-variable gravity data. Remote Sens. 14, 4059. doi:10.3390/rs14164059
Mohamed, A., and Gonçalvès, J. (2021). Hydro-geophysical monitoring of the North Western Sahara Aquifer System’s groundwater resources using gravity data. J. Afr. Earth Sci. 178, 104188.
Mohamed, A. (2020c). Gravity applications in estimating the mass variations in the Middle East: a case study from Iran. Arab. J. Geosci. 13, 364.
Mohamed, A. (2020b). Gravity applications to groundwater storage variations of the Nile Delta Aquifer. J. Appl. Geophys. 182, 104177.
Mohamed, A. (2020a). Gravity based estimates of modern recharge of the Sudanese area. J. Afr. Earth Sci. 163, 103740.
Mohamed, A., Othman, A., Galal, W. F., and Abdelrady, A. (2023b). Integrated geophysical approach of groundwater potential in wadi ranyah, Saudi Arabia, using gravity, electrical resistivity, and remote-sensing techniques. Remote Sens. 15, 1808. doi:10.3390/rs15071808
Mohamed, A., Ragaa Eldeen, E., and Abdelmalik, K. (2021). Gravity based assessment of spatio-temporal mass variations of the groundwater resources in the Eastern Desert, Egypt. Arab. J. Geosci. 14, 500.
Mohamed, A., Sultan, M., Ahmed, M., Yan, E., and Ahmed, E. (2017). Aquifer recharge, depletion, and connectivity: inferences from GRACE, land surface models, and geochemical and geophysical data. Bull. Geol. Soc. Am. 129, 534–546. doi:10.1130/B31460.1
Mohamed, M., Mohamed, A., Alarifi, S. S., Othman, A., Ahmed, A. A., Mohammed, M. A. A., et al. (2023c). Geophysical investigations for the identification of subsurface features influencing mineralization zones. J. King Saud Univ. – Sci. 2023, 102809. doi:10.1016/j.jksus.2023.102809
Narany, T. S., Aris, A. Z., Sefie, A., and Keesstra, S. (2017). Detecting and predicting the impact of land use changes on groundwater quality, a case study in Northern Kelantan, Malaysia. Sci. Total. Environ. 599, 844–853. doi:10.1016/j.scitotenv.2017.04.171
Othman, A., Abdelrady, A., and Mohamed, A. (2022). Monitoring mass variations in Iraq using time-variable gravity data. Remote Sens. 14, 3346. doi:10.3390/rs14143346
Ramillien, G., Frappart, F., Cazenave, A., and Guntner, A. (2005). Time variations of land water storage from an inversion of 2 years of GRACE geoids. Earth Planet. Sci. Lett. 235, 283–301. doi:10.1016/j.epsl.2005.04.005
Rodell, M., Famiglietti, J. S., Chen, J., Seneviratne, S. I., Viterbo, P., Holl, S., et al. (2004). Basin scale estimates of evapotranspiration using GRACE and other observations. Geophys. Res. Lett. 31, L20504. doi:10.1029/2004gl020873
Rodell, M., Houser, P. R., Jambor, U., Gottschalck, J., Mitchell, K., Meng, C. J., et al. (2009). Satellite-based estimates of groundwater depletion in India. Nature 460, 999–1002. doi:10.1038/nature08238
Sakumura, C., Bettadpur, S., and Bruinsma, S. (2014). Ensemble prediction and intercomparison analysis of GRACE time-variable gravity field models. Geophys. Res. Lett. 41, 1389–1397. doi:10.1002/2013gl058632
Save, H., Bettadpur, S., and Tapley, B. D. (2016). High-resolution CSR GRACE RL05 mascons. J. Geophys. Res. Solid Earth 121, 7547–7569. doi:10.1002/2016jb013007
Scanlon, B. R., Zhang, Z., Save, H., Wiese, D. N., Landerer, F. W., Long, D., et al. (2016). Global evaluation of new GRACE mascon products for hydrologic applications. Water Resour. Res. 52, 9412–9429. doi:10.1002/2016wr019494
Shugar, D. H., Jacquemart, M., Shean, D., Bhushan, S., Upadhyay, K., Sattar, A., et al. (2021). A massive rock and ice avalanche caused the 2021 disaster at Chamoli, Indian Himalaya. Science 373, 300–306. doi:10.1126/science.abh4455
Siebert, S., Burke, J., Faures, J. M., Frenken, K., Hoogeveen, J., Döll, P., et al. (2010). Groundwater use for irrigation - a global inventory. Hydrol. Earth Syst. Sci. 14, 1863–1880. doi:10.5194/hess-14-1863-2010
Simmers, I. (1997). Recharge of phreatic aquifers in (semi-) arid areas: international association of hydrogeologists (IAH) international contributions to hydrogeology 19. Boca Raton, FL: CRC Press, 240.
Soltani, S. S., Ashtiani, B. A., and Simmons, C. T. (2021). Review of assimilating GRACE terrestrial water storage data into hydrological models: advances, challenges and opportunities. Earth-Sci. Rev. 213, 103487. doi:10.1016/j.earscirev.2020.103487
Sowayan, A. M., and Allayla, R. (1989). Origin of the saline ground water in wadi Ar-rumah, Saudi Arabia. Groundwater 27, 481–490. doi:10.1111/j.1745-6584.1989.tb01968.x
Sun, J., Hu, L., Liu, X., and Sun, K. (2022). Enhanced understanding ofGroundwater storage changes underthe influence of river basin governance using GRACE data and downscaling model. Remote Sens. 14, 4719. doi:10.3390/rs14194719
Taha, A. I., Al Deep, M., and Mohamed, A. (2021). Investigation of groundwater occurrence using gravity and electrical resistivity methods: a case study from wadi sar, hijaz mountains, Saudi Arabia. Arab. J. Geosci. 14, 334. doi:10.1007/s12517-021-06628-z
Tapley, B. D., Bettadpur, S., Ries, J. C., Thompson, P. F., and Watkins, M. M. (2004). GRACE measurements of mass variability in the Earth system. Science 305, 503–505. doi:10.1126/science.1099192
Tapley, B. D., Watkins, M. M., Flechtner, F., Reigber, C., Bettadpur, S., Rodell, M., et al. (2019). Contributions of GRACE to understanding climate change. Nat. Clim. Chang. 9, 358–369. doi:10.1038/s41558-019-0456-2
Taylor, R. G., Scanlon, B., Döll, P., Rodell, M., Van Beek, R., Wada, Y., et al. (2013). Ground water and climate change. Nat. Clim. Chang. 3, 322–329. doi:10.1038/nclimate1744
Tiwari, V. M., Wahr, J., and Swenson, S. (2009). Dwindling groundwater resources in northern India, from satellite gravity observations. Geophys. Res. Lett. 36, L18401. doi:10.1029/2009GL039401
Wadi al-Rummah, R. (2011). Wadi al-rummah - wikipedia. Avaliable At: wikipedia.org/wiki/Wadi_al-Rummah.
Wahr, J., Swenson, S., Zlotnicki, V., and Velicogna, I. (2004). Time-variable gravity from GRACE: first results. Geophys Res. Lett. 31, L11501. doi:10.1029/2004GL019779
Wang, F., Shen, Y., Chen, Q., and Wang, W. (2021). Bridging the gap between GRACE and GRACE follow-on monthly gravity field solutions using improved multichannel singular spectrum analysis. J. Hydrology 594, 125972. doi:10.1016/j.jhydrol.2021.125972
Wang, J. D., Song, C. Q., Reager, J. T., Yao, F. F., Famiglietti, J. S., Sheng, Y. W., et al. (2018). Recent global decline in endorheic basin water storages. Nat. Geosci. 11, 926–932. doi:10.1038/s41561-018-0265-7
Watkins, M. M., Wiese, D. N., Yuan, D. N., Boening, C., and Landerer, F. W. (2015). Improved methods for observing Earth’s time variable mass distribution with GRACE using spherical cap mascons. J. Geophys. Res. Solid Earth 120, 2648–2671. doi:10.1002/2014JB011547
Wei, L. Y., Jiang, S. H., Ren, L. L., Tan, H. B., Ta, W. Q., Liu, Y., et al. (2021). Spatiotemporal changes of terrestrial water storage and possible causes in the closed Qaidam Basin, China using GRACE and GRACE Follow-On data. J. Hydrol. 598, 126274. doi:10.1016/j.jhydrol.2021.126274
Yang, X., Wang, N., Chen, A., Li, Z., Liang, Q., and Zhang, Y. (2022). Impacts of climate change, glacier mass loss and human activities on spatiotemporal variations in TerrestrialWater storage of the qaidam basin, China. Remote Sens. 14, 2186. doi:10.3390/rs14092186
Keywords: geophysical data, GLDAS, groundwater depletion, Wadi Ar-Ramah, Saudi Arabia
Citation: Mohamed A and Alshehri F (2023) Application of gravity and remote sensing data to groundwater potential in Wadi Ar-Ramah, Saudi Arabia. Front. Earth Sci. 11:1227691. doi: 10.3389/feart.2023.1227691
Received: 23 May 2023; Accepted: 01 August 2023;
Published: 16 August 2023.
Edited by:
Ahmed M. Eldosouky, Suez University, EgyptReviewed by:
Cheikh Faye, Ziguinchor University, SenegalSwapan Talukdar, Jamia Millia Islamia, India
Copyright © 2023 Mohamed and Alshehri. This is an open-access article distributed under the terms of the Creative Commons Attribution License (CC BY). The use, distribution or reproduction in other forums is permitted, provided the original author(s) and the copyright owner(s) are credited and that the original publication in this journal is cited, in accordance with accepted academic practice. No use, distribution or reproduction is permitted which does not comply with these terms.
*Correspondence: Ahmed Mohamed, ahmedmohamed@aun.edu.eg; Fahad Alshehri, falshehria@ksu.edu.sa