- 1Abdullah Alrushaid Chair for Earth Science Remote Sensing Research, Geology and Geophysics Department, College of Science, King Saud University, Riyadh, Saudi Arabia
- 2Department of Geology, Faculty of Science, Assiut University, Assiut, Egypt
Water scarcity is becoming a growing problem in the Middle East due to urbanization, industrialization, and population growth. Saudi Arabia is one of the region’s largest consumers of water, so it is important to take immediate action to address this issue. This study used data from the Gravity Recovery and Climate Experiment (GRACE) to assess changes in groundwater storage in Wadi Fatimah and its surrounding areas. The results showed that the average annual rainfall (AAR) in Wadi Fatimah was 131 mm, while the AAR for the entire Makah province was 99.3 mm. The AAR in Makah province can be divided into three climatic periods: Period I (April 2002-December 2011): AAR of 92.8 mm; Period II (January 2012-December 2016): AAR of 101.8 mm and Period III (January 2017-December 2021): AAR of 116.4 mm. The GRACE-derived ΔTWS (time-variable gravity) variations were −0.18 ± 0.023 cm/yr in Wadi Fatimah and −0.38 ± 0.018 cm/yr in the entire Makah Province. The soil moisture storage (ΔSMS) variations were +0.039 ± 0.025 mm/yr in Wadi Fatimah and −0.008 ± 0.002 mm/yr in the entire Makah Province. The average groundwater storage (ΔGWS) variation in Wadi Fatimah was −0.18 ± 0.022 cm/yr, which indicates a slight decrease. The ΔGWS variation in the entire Makah region was −0.38 ± 0.017 cm/yr, which indicates a negative trend. The study also found that surface runoff from rainfall in the eastern section of Wadi Fatimah flows westward to join other streams that flow into the Wadi’s central and downstream areas. This runoff replenishes the shallow alluvium deposits and aquifers. Wadi Fatimah is able to partially compensate for the impact of its groundwater extraction with a recharge rate of +0.22 ± 0.22 mm/yr. The integrated method used in this study is a helpful and economical way to evaluate groundwater resource variability over Wadi Fatimah region and its surrounding province.
1 Introduction
Due to rapid population growth and groundwater exploitation, freshwater withdrawal for agricultural, industrial, and domestic uses worldwide has increased by a factor of approximately six over the past century and is expected to continue increasing at a rate of approximately one percent per year (United Nations, 2022). According to (Shiklomanov and Rodda, 2003), groundwater is the most significant source of liquid freshwater, accounting for 99 percent of the global total. 50 percent of the extracted groundwater is used for domestic purposes, while the remaining 25 percent is used for agriculture (Margat and Van der Gun, 2013). Rising water scarcity is a problem in many countries because population growth has led to a greater demand for water. Climate change influences the recharge of groundwater as a result of its impact on precipitation and surface water laquage. Groundwater can be reduced by climate change, however in the majority of cases, the reservoir was depleted due to intensive exploitation (Bierkens and Wada, 2019). As a result of increased demand and climate change, water resources will come under greater strain, particularly in arid and semiarid settings (FAO, 2020).
For the purposes of water resources development, management, and governance, constant monitoring of water storage is essential for identifying unfavorable changes such as over-pumping and reduced recharge (Mushin, 2022). Various in-situ observation techniques were utilized to evaluate the hydrological dynamics of aquifers, such as chemical and modeling techniques (de Vries and Simmers, 2002; Scanlon et al., 2002; Milewski et al., 2009; Mohamed and Gonçalvès, 2021; Mohamed et al., 2022c; Mohamed et al., 2022d). It is challenging to determine large-scale spatio-temporal changes in groundwater storage using these traditional methods due to the limited amount of data points. The absence of in-situ groundwater measurements (Frappart and Ramillien, 2018) poses the greatest difficulty to the estimate of the fluctuations in water storage in particular regions. Moreover, the outcomes of these procedures are frequently doubtful due to the enormous time and money required to produce them (Mohamed et al., 2017). According to the State of Hydrological Services in Developing Countries evaluation, just ten percent of the world’s countries have adequate water monitoring systems (Tapley et al., 2004). In addition, limited data access policies in certain regions restrict groundwater research (Tapley et al., 2004). In addition to the methods and instruments of conventional monitoring systems, the use of new technologies is required to enhance the monitoring and estimation of water resources. Groundwater management is a critical concern in regions facing water scarcity and resource depletion. In recent years, the Gravity Recovery and Climate Experiment (GRACE) satellite mission has emerged as a valuable tool for monitoring and managing groundwater resources.
Multiple studies have been conducted to address these issues by analyzing and integrating GRACE data with other climate models and on-site observations (Wahr et al., 1998; Wahr et al., 2004; Laurent Longuevergne et al., 2010). A significant method for bridging data gaps in hydrological water monitoring is provided by the satellite GRACE-measured time-variable gravity data (Tiwari et al., 2009; Famiglietti et al., 2011). Monthly global changes in total water storage (TWS) are provided from GRACE (Wahr et al., 2004; Syed et al., 2008). GRACE data has been demonstrated to be useful in estimating water storage changes with high adequate resolution and accuracy in a number of studies (Wahr et al., 2006; Zaitchik et al., 2008; Voss et al., 2013; Mohamed et al., 2017; Alshehri and Abdelrahman, 2023a).
In March of 2002, as part of a collaborative mission between NASA and the German Aerospace Center (DLR), two satellites known as GRACE were put into orbit to track the planet’s gravity as it changed over time (Tapley et al., 2004). This data will be used to quantify the fluctuations in TWS and will also contribute to other geological and climatological studies. Generally speaking, the JPL is in charge of coordinating all missions. Several research have used GRACE data to generate regional water mass fluctuation estimates, to calculate water balances at basinal scales, to monitor the water storage changes, recharge and depletion rates, and to learn more about how aquifers’ hydrologic settings adapt to different climates (Rodell et al., 2004; Crowley et al., 2008; Xavier et al., 2010; Leblanc et al., 2009; Rodell et al., 2009; Rodell et al., 2009; Tiwari et al., 2009; Wada et al., 2010; Ferreira et al., 2012; Gonçalvès et al., 2013; Voss et al., 2013; Joodaki et al., 2014; Wouters et al., 2014;Chinnasamy et al., 2015; Al-Zyoud et al., 2015; Chinnasamy and Sunde, 2016; Lakshmi, 2016; Huo et al., 2016; Long et al., 2016; Fallatah et al., 2017; Mohamed et al., 2017; Fallatah et al., 2019; Mohamed, 2019; Mohamed, 2020a; Mohamed, 2020b; Mohamed, 2020c; Alshehri and Mohamed, 2023; Alshehri and Abdelrahman, 2023b).
Additionally, some research in the Saudi Arabia has integrated GRACE with additional data to estimate the recharge and/or depletion rates caused by climatic and/or anthropogenic activities (e.g., Fallatah et al., 2017; Fallatah et al., 2019; Taha et al., 2021; Mohamed et al., 2022a; Mohamed et al., 2022b; Alshehri et al., 2023a) given that the hydrological monitoring data gaps can be filled up with the help of GRACE (Famiglietti et al., 2011). Rodell et al. (2018) employed GRACE satellite data to assess global freshwater trends. The study highlights the utility of GRACE data in tracking changes in terrestrial water storage, including groundwater and demostrates the potential of GRACE data for better groundwater management strategies.
The lack of vertical resolution for GRACE data and its low horizontal resolution limits its usefulness for these applications (e.g., Ahmed et al., 2016). It is unclear how much of TWS can be attributed to each of the many compartments (such as groundwater, surface water, and soil moisture) and how much can be attributed to GRACE itself. Improvements in horizontal resolution and the ability to separate individual components from GRACE-derived TWS estimations have resulted from the combination of GRACE data with land surface model datasets.
Quantitative methods are used in climate model outputs to simulate the interplay of the atmosphere, oceans, land, and ice. These models have several applications, such as weather forecasting, water cycle simulation, and resource management. The Global Land Data Assimilation System is one example (GLDAS; Rodell et al., 2004; Rodell et al., 2009; Moiwo et al., 2011). Gathering data from satellites and ground sensors, GLDAS determines the most accurate fields of land surface states and fluxes.
This study addresses the pressing issue of extreme water scarcity in the Middle East, primarily driven by urbanization, industrialization, and population growth. Given that Saudi Arabia is a major water consumer in the region, immediate action is imperative. Assessing fluctuations in groundwater storage in Wadi Fatimah and the entire Makah province, particularly by combining data from the Gravity Recovery and Climate Experiment (GRACE) with other relevant data, provides critical insights into managing and mitigating the water scarcity problem. We demonstrate how GRACE data and outputs from land surface models with the Tropical Rainfall Measuring Mission (TRMM) datasets may be utilized to calculate the changes in groundwater resources and investigate the response of the groundwater aquifer to climatic changes and/or anthropogenic activities for the Wadi Fatma in the southwestern region of Saudi Arabia. Overall the framework of this study is built upon the urgency of addressing extreme water scarcity in the Middle East, with a specific focus on the Wadi Fatimah region in the Makah province of Saudi Arabia. The integration of multiple data sources, climatic and hydrological analyses, and a focus on groundwater dynamics forms the basis of this study, aimed at providing valuable insights into the management of this critical natural resource.
2 Geology and hydrogeology
The study area is located in the Makkah quadrangle on Sheet 21D of the Saudi Arabian (Figure 1). This quadrangle is situated in the Arabian Shield at the west-central portion of the Arabian Peninsula, which borders the Red Sea at the west. It extends between latitude 21° 00’ and 22° 00’ N and longitude 38° 55’ and 40° 30’ E. The western section of the basin is made up of Quaternary coastal deposits and the eastern part is made up mostly of the basement rocks of the Arabian Shield, and the geology of the basin reflects these distinct differences in topography (Figure 1). Wadi Fatimah is located on the Shield’s rifted western border, a massive fault zone heading southwest that has been active since the Precambrian time (Grainger, 1992). Multiphase deformation, metamorphism, and igneous intrusions have affected the late Proterozoic volcanic and volcaniclastic rocks that make up much of the wadi. Harrat Rahat Quaternary basalts fill in the northeastern portion of the basin. The Tertiary Shumaysi sediments are covered by surface loose sediments of alluvian fan, eolisn sands, and terrace deposits along the main channel of the Wadi and the coast (Al-Garni, 2009; Alshehri et al., 2023b). The northwest region of the wadi, north of its main channel, contains the Fatimah Formation that is represented by a Precambrian clastic sedimentary rock unit (Figure 1).
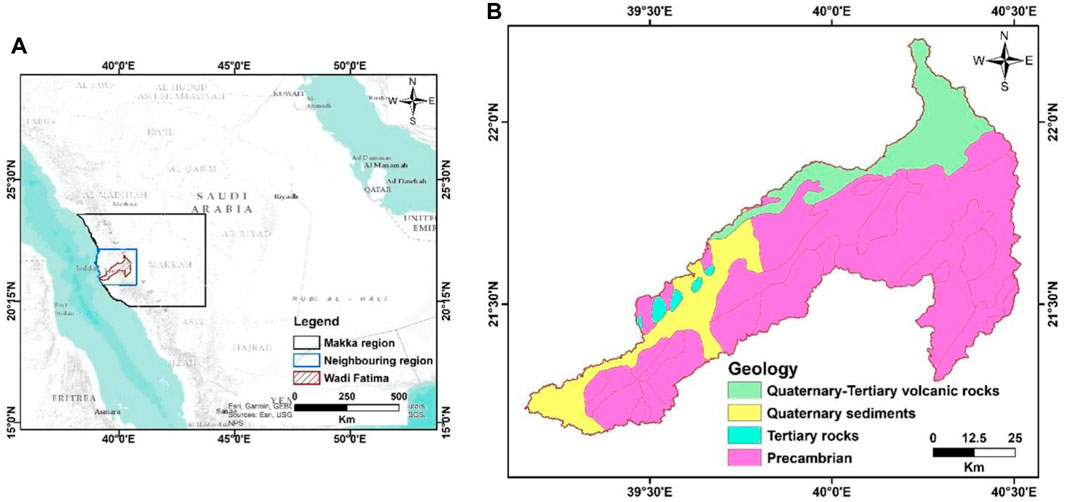
FIGURE 1. A location map of Wadi Fatimah, surrounding, and the entire Makah region (A) and the geology OF Wadi Fatimah (B).
Wadi Fatimah’s unconsolidated alluvial deposits vary in thickness from 10 to 20 m in the Arabian Shield to 80 m in the coastal plains. Conglomerate, sandstone, and mudstone make up the bulk of the sediments (Sharaf, 2013). The shallow aquifers rely on the basement rocks’ secondary porosity to provide hydraulic support in the shield (Sharaf et al., 2004). Transmission coefficients for the unconfined aquifer fall between 300 and 1800 m2/day, while specific yields are between 0.12 and 0.20. These rare runoff occurrences feed the alluvial sediments above the eroded basement rocks immediately after rainfall, which in turn recharges the shallow aquifer. However, due to evaporation and water infiltration into the underlying weathered and fractured basement, the water table quickly drops (Alyamani and Hussein, 1995).
3 Data and methods
3.1 GRACE mascon data
The GRACE satellites have been monitoring the Earth’s gravitational pull since their 2002 launch to detect variations in mass. GRACE is comprised of a pair of satellites that orbit the Earth at 450–500 km apart and share an identical orbit. When Earth’s gravity field shifts, the satellites’ velocities adjust accordingly. As the pair of satellites reaches a positive mass anomaly, such a mountainous region, the gravitational pull increases, causing the leading satellite to speed up and increase the gap between them (Houborg et al., 2012). It has been found that variations in TWS are the primary cause of mass anomalies in the absence of tectonic activity (Swenson et al., 2008).
This research made use of three different time-variable gravity mascon solutions from the GRACE processing center. Mascon products include datasets from the Jet Propulsion Laboratory (JPL), the Center for Space Research (CSR) at University of Texas, and the Goddard Space Flight Center (GSFC) at NASA all comprise gravity solutions. When compared to spherical harmonic solutions, the spatial resolution and accuracy of these mascon solutions are superior; they are able to detect all signals below the GRACE noise threshold. Not even a little de-striping or smoothing is necessary. These products may not even need the scaling factor (Watkins et al., 2015; Luthcke et al., 2013; Save et al., 2016; Wiese et al., 2016).
In the JPL-RL06M v02 solution, the TWS anomalies were determined for each of the three spherical cap mascon blocks with the same area (Watkins et al., 2015; Wiese et al., 2016). The TWS fluctuations are sampled in the final data set to 0.5° × 0.5° grids. By employing the scaling factors provided by JPL, leakage signals caused by 3° mascon blocks can be recovered, presuming the JPL-RL06M v02 has a real 3° resolution. The new JPL-RL06M v02 data improves upon the original by allowing for greater differentiation between land and sea mascons (JPL-RL05M). For each mascon that crosses a coastline, the Coastal Resolution Improvement filter was applied as a last step in the process (Watkins et al., 2015).
The CSR RL06M v02 solution that was computed from an equal region at a resolution of 1, displays TWS variations in 0.25° × 0.25° grids (Save et al., 2016; Sultan and Save, 2020). This new grid separates the shoreline’s hexagonal tiles into ocean and land sections to reduce interference between the two. The scaling factor is unnecessary for such tiny mascons. We also utilized the mascon solution produced by the GSFC-RL06v1.0 for each 0.5° equal-area square mascon.
The missing monthly data was interpolated using the cubic-spine approach. In this work, the mascon solutions were used significantly. We determined the slope values of the TWS trends. In the next step, we estimated the errors in the trend values.
The choice of using GRACE mascon data in this research is well-justified based on its unique capabilities and advantages. GRACE, consisting of two satellites orbiting the Earth, is designed to monitor variations in Earth’s gravitational field caused by changes in mass. These variations can result from factors like total water storage (TWS) changes, making GRACE a valuable tool for assessing groundwater fluctuations.
3.2 GLDAS total soil moisture (TSM)
Since data from gauge stations in the research area were unavailable or insufficient during the time period under study, we rely on estimates of soil moisture, extracted from the Global Land Data Assimilation System (GLDAS) model (Rodell et al., 2004). Those results were put to use in order to break down the TWS into its constituent parts. This is because GRACE does not understand the various TWS parts. In this research, we compared two different NSAS GLDAS versions (VIC and CLM). The same time period as GRACE data was covered by the monthly data, which has a spatial resolution of one degree. The use of GLDAS data can be justified due to the absence of gauge station data, the need to dissect TWS into its components, the reliability of GLDAS, the appropriate spatial resolution, and the temporal consistency with GRACE data. This choice enables a more comprehensive and accurate analysis of groundwater dynamics and their contributing factors in the study area.
3.3 TRMM rainfall data
Due to a lack of available rainfall data from the ground, the TRMM (Kummerow et al., 1998) data was used instead. The time span covered by TRMM data, which has a spatial resolution of 0.25° × 0.25°, overlaps with that of GRACE and GLDAS. Through yearly averaging of all monthly rainfall, TRMMdata was utilized to create a map of the average annual rainfall (AAR) (Figure 3).
Based on the weather patterns and the beginning of the 2007 drought, two separate time periods have been determined (Trigo et al., 2010). The first period, from 04/2002 to 12/2006, experienced a higher than average rate of precipitation, whereas the second period, from January 2007 to July 2017, experienced a lower than average rate of precipitation. Each period’s AAP rate was determined by averaging that period’s monthly rainfall data over a full year. For this study, we used the AAP to look into how precipitation affected GWS in various time periods. The use of TRMM data not only fills a critical data gap resulting from the lack of ground-based rainfall measurements but also provides valuable information on long-term precipitation trends. This data source enabled to explore the connections between precipitation and groundwater storage variations over specific time periods, which is essential for the study’s objectives and analyses.
The period of study in this investigation spans from the launch of the GRACE satellites in 2002 to the conclusion of data analysis in 2022. The integration of GRACE, GLDAS, and TRMM data sources, along with various processing steps, allowed for a comprehensive examination of groundwater dynamics and their relationship to precipitation patterns over these years. ArcGIS 10.6.1 was used to prepare all the maps in this study.
4 Result and discussion
In this study, we integrate GRACE and GLDAS satellite data to get a comprehensive look at the groundwater potentialities of Wadi Fatma and its surroundings in Saudi Arabia’s southwestern region.
4.1 Average annual rainfall
Figure 2 presents a graph displaying the monthly rainfall rates, revealing a pattern of higher values from December to May and lower values from June to November. This climatic data facilitates the identification of three distinct time periods for the entire Makkah region, attributed to the substantial area with varying precipitation levels. Precipitation diminishes as we move from the coastal zone towards the central part of Saudi Arabia, as depicted in Figure 3. These three time intervals were determined based on the typical annual rainfall pattern, as shown in Figure 4. The Average Annual Precipitation (AAP) ranged from a minimum of 59.7 mm in 2008 to a maximum of 157.8 mm in 2018. Period I (2002–2011) had the lowest AAP at 92.8 mm, while period III (2017–2019) recorded the highest AAP at 101.8 mm. Period II (2012–2016), falling between the other two, had an AAP of 48.7 mm (refer to Table 1). Focusing on Wadi Fatimah and its nearby coastal area, the AAP exhibited slightly higher values, ranging from 130.7 to 131.1 mm throughout the study period, compared to the lower AAP of 99.3 mm for the entire Makkah region. Given that Wadi Fatma and its surrounding area represent the lowlands near the coast within the study area, the AAP for Wadi Fatma displayed a slightly higher positive trend, calculated at 3.20 mm. This is in contrast to the lower positive trend of 1.07 mm observed over the entire region during the entire study period. These precipitation rates continue to underscore the arid nature of the study area, with most rainfall events and storms occurring between the months of November to May.
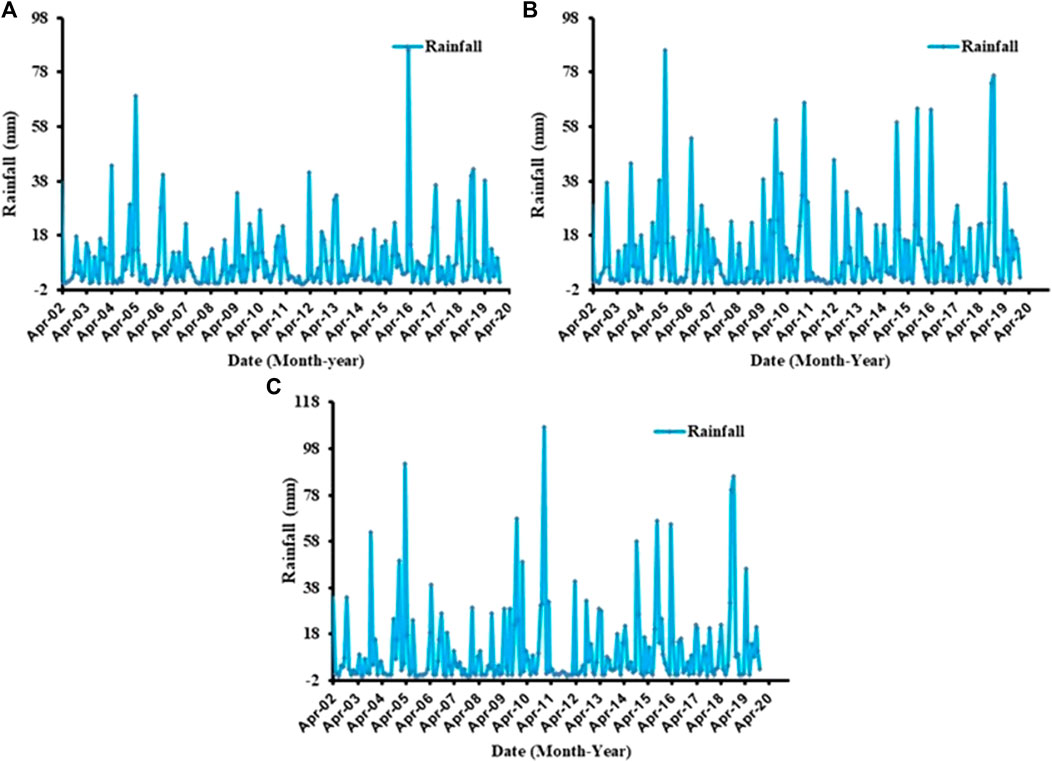
FIGURE 2. Monthly rainfall timeseries over the Makah (A), surrounding zone of Wadi Fatimah (B), and the Wadi Fatimah (C) extracted from the TRMM datasets.
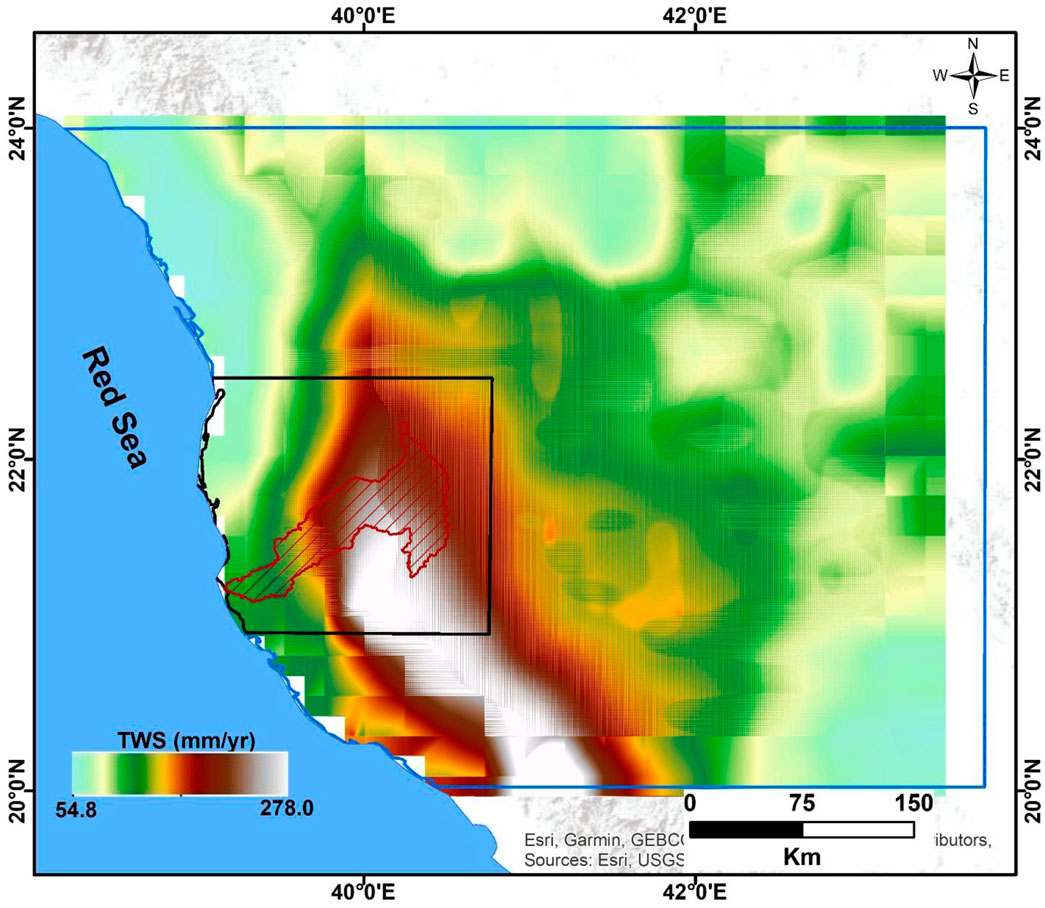
FIGURE 3. Average annual precipitation rate over the study region extracted from the TRMM over the entire period.
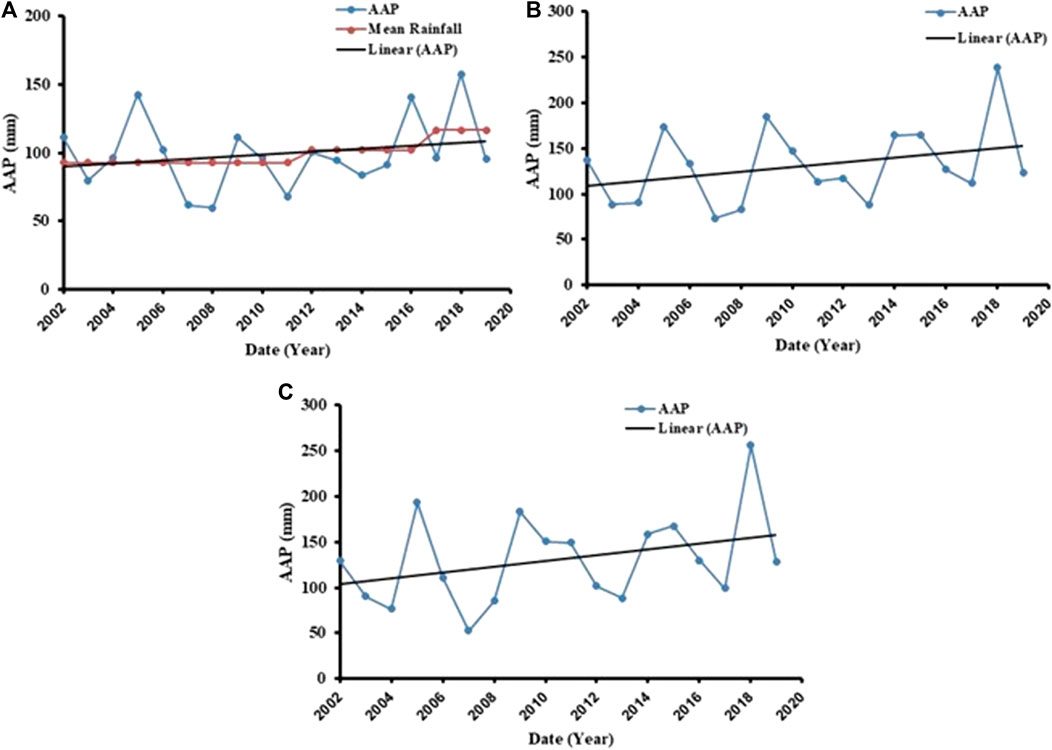
FIGURE 4. AAP (mm) data for the Makah (A), surrounding zone of Wadi Fatimah (B), and the Wadi Fatimah (C) extracted from the TRMM datasets.
4.2 Temporal variations in ΔTWS
Time-series plots depicting the variations in ΔTWS across the study area are displayed in Figure 5, combining data from two different solutions and their average. Notably, a strong correlation of 0.82 was observed between the two mascon datasets, encompassing the entire region. Analyzing the average trends in TWS from CSR and GSFC, positive values are evident in the southwestern portions of the research area, while negative trends prevail in the northeastern regions (Figure 6). Conducting a linear regression analysis on the averaged ΔTWS solutions illustrated in Figure 5 reveals three distinct trends across the entirety of the Makkah region throughout the study period (Figure 7; Table 1). The first trend (period I) exhibits a slightly negative slope, estimated at −0.49 ± 0.051 cm/yr (Table 1). Period II displays a very slight positive trend, estimated at −0.012 ± 0.115 cm/yr. The third trend (period III) takes a notably steep descent, with a slope of −0.64 ± 0.15 cm/yr. Interestingly, these trend variations do not align with the Average Annual Precipitation (AAP) time series, which displays a positive increase from 92.8 mm in Period I to 116.4 mm in Period III (Table 1; Figure 4).
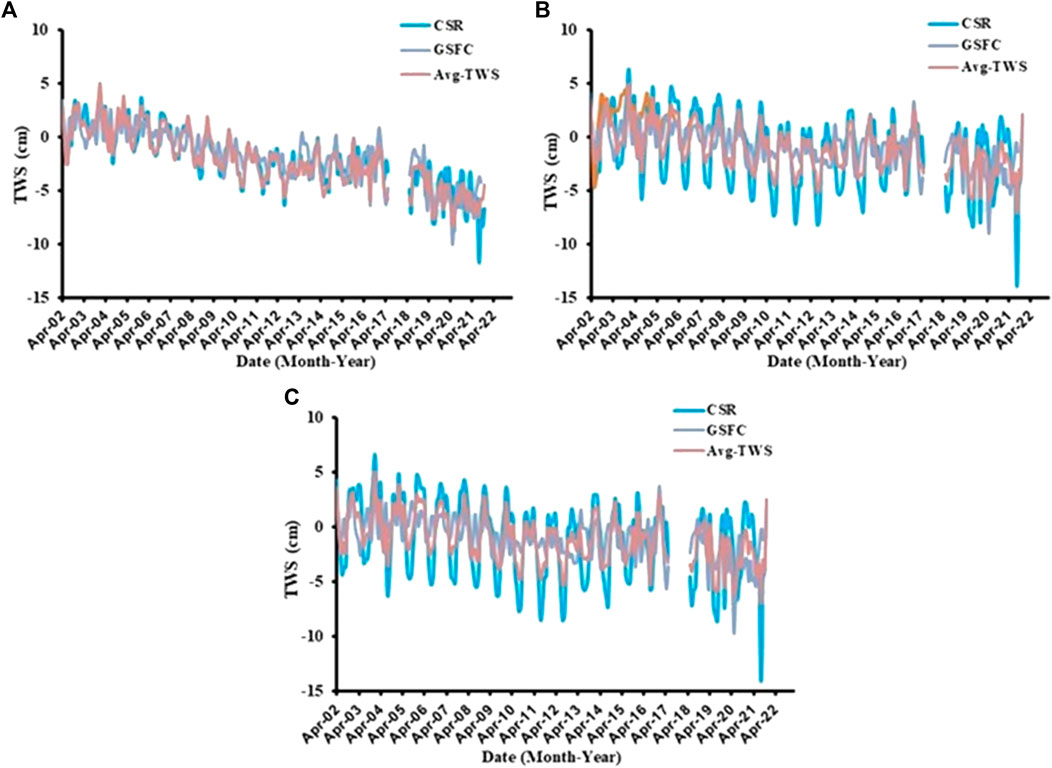
FIGURE 5. Temporal variations in GRACE-derived TWS for the Makah (A), surrounding zone of Wadi Fatima (B), and the Wadi Fatimah (C) from the different solutions and their mean.
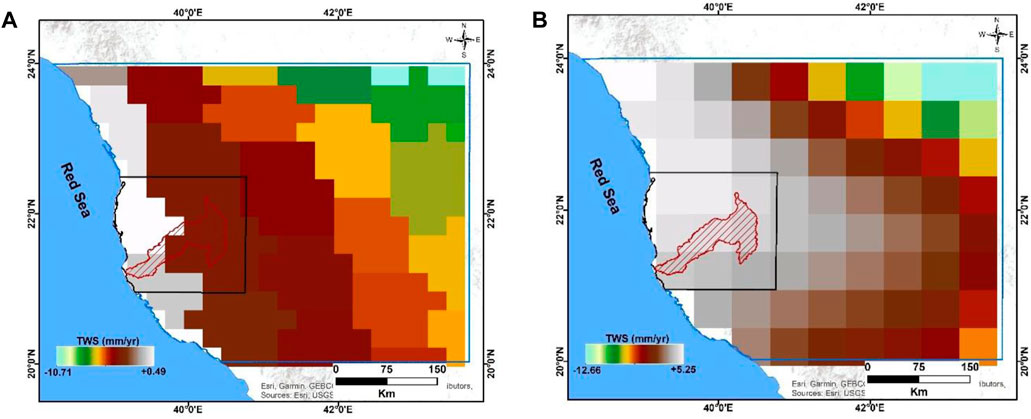
FIGURE 6. Spatiotemporal variations in GRACE-derived TWS for the study area using CSR (A) and GSFC (B) mascon solutions.
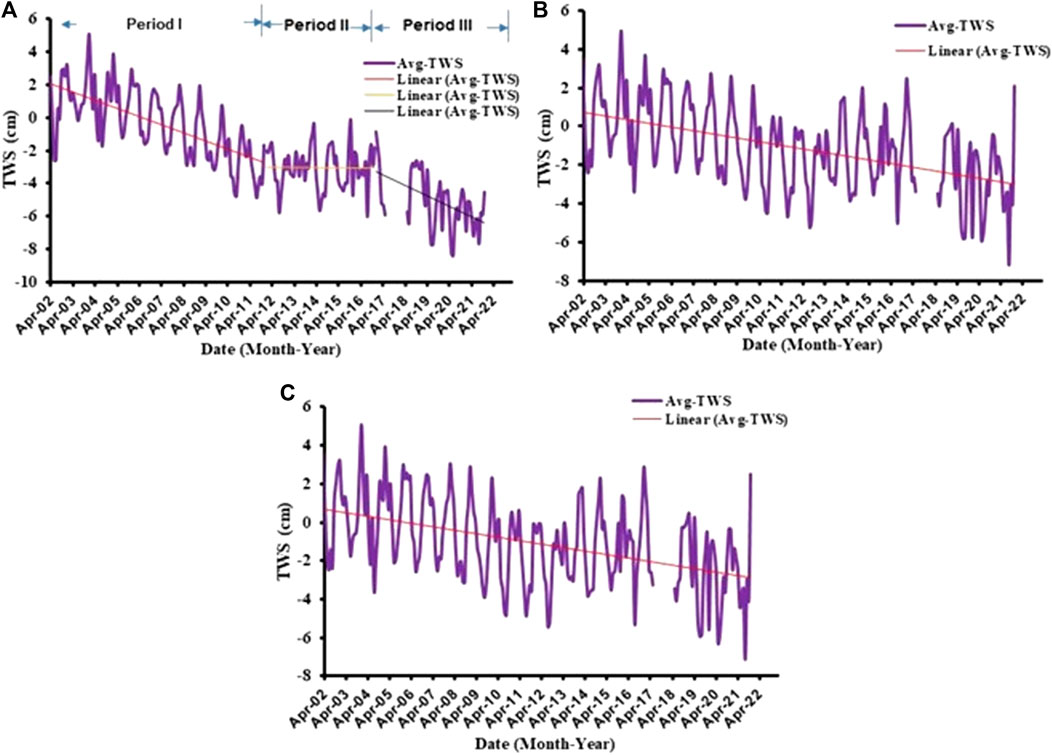
FIGURE 7. Time series of the averaging TWS and their trends for the Makah (A), surrounding zone of Wadi Fatimah (B), and the Wadi Fatimah (C) from 04/2002 to 12/2021.
Shifting the focus to Wadi Fatma, the temporal patterns in GRACE-derived TWS reveal slightly negative trends, estimated at −0.18 ± 0.039, −0.19 ± 0.019, and −0.18 ± 0.023 cm/yr, as determined by the mascon solutions from CSR, GSFC, and their averages (Table 2; Figure 7). The smaller area surrounding Wadi Fatma exhibits TWS patterns that align somewhat with those of Wadi Fatma (Figure 5). The TWS extracted through GRACE illustrates trend values estimated at −0.19 ± 0.036, −0.19 ± 0.017, and −0.19 ± 0.022 cm/yr when considering the GAREC mascon CSR, GSFC, and their averaging solutions (Table 2; Figure 7). As depicted in Figure 5, the seasonal fluctuations of TWS for Wadi Fatma exhibit relatively higher values from October to April and lower values from May to September. Importantly, these TWS peaks are noticeably delayed by 1–3 months compared to the peaks in rainfall. This temporal discrepancy may be attributed to the gradual response of TWS to changes in precipitation, potentially influenced by surface runoff within the study area. Additionally, the presence of surface basement rocks might hinder the infiltration of water, partly due to the moderately steep slope of the ground surface. Analyzing the long-term trends in TWS variations in Wadi Fatma reveals negative TWS values at the upstream part of the Wadi, gradually decreasing to slightly negative values as we move downstream (Figure 6).
4.3 Temporal variations in GLDAS-derived ΔSMS
Because GRACE lacks the vertical resolution to distinguish the various components of total water storage (TWS), such as surface water storage (SWS), groundwater storage (GWS), and soil moisture storage (SMS), it becomes challenging to attribute mass changes in a given geographical area to specific storage types. This limitation arises from the fact that the gravitational signal detected by GRACE results from the combined impact of mass changes in the atmosphere, oceans, and terrestrial water storage, as noted by Wahr et al. (2006). To address this challenge, we employed a range of methodologies, including the utilization of climate models, to identify and differentiate the contributions of each storage component. While it is worth noting that traditional soil moisture measurements have often been characterized by a lack of reliability and consistency, recent advances in both satellite and ground-based observational data products have significantly improved the accuracy of soil moisture (SM) data.
One valuable system in this regard is the Global Land Data Assimilation System (GLDAS), as developed by Rodell et al., in 2004 and 2009, as well as Moiwo et al. (2011). GLDAS integrates various sources of data to provide comprehensive information about land surface conditions, including soil moisture. These datasets play a crucial role in diverse applications, including climate prediction, hydrological studies, and water resource management.
To assess changes in SMS throughout the study period, we leveraged three GLDAS datasets, namely, VIC, CLM, and Noah. Despite differences in SMS trends, the variations in SMS ranged from +0.008 ± 0.002 mm/yr for the entire Makkah region to 0.046 ± 0.026 mm/yr for the smaller area surrounding the Wadi. This variation in SMS trends is influenced by factors such as local rainfall patterns. In the case of Wadi Fatma, characterized by slightly higher rainfall data and its position downstream from surrounding regions, the SMS exhibited a relatively higher trend, calculated at +0.41 ± 0.1 mm/yr over the entire study period.
4.4 Temporal variations in GLDAS-derived ΔGWS
The capabilities of GRACE to distinguish between different components of total water storage (TWS), including soil moisture, surface water, and groundwater, have limitations. To address this challenge, we employed GLDAS model results to estimate the non-groundwater components, as illustrated in Figure 8. Changes in surface water storage (SWS) were found to be insignificant due to the lower rates of surface runoff.
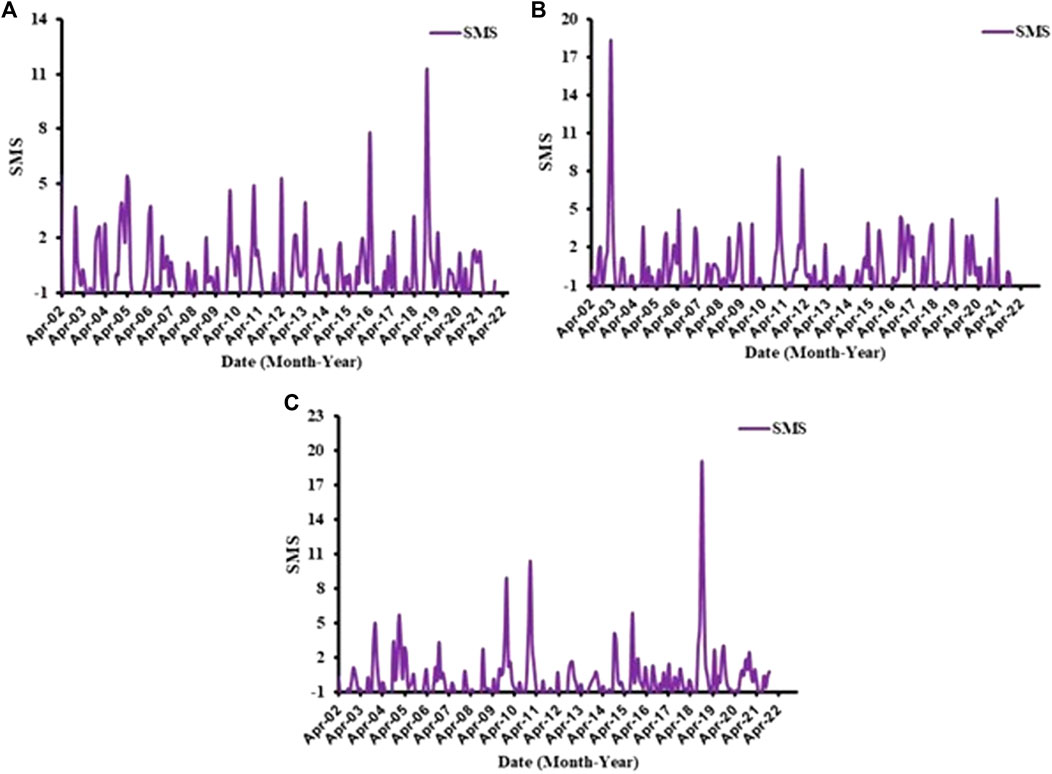
FIGURE 8. Temporal variations of the GLDAS-derived SMS for the Makah (A), surrounding zone of Wadi Fatimah (B), and the Wadi Fatimah (C) from 04/2002 to 12/2021.
We utilized Eq. 1 to compute groundwater storage (GWS) by subtracting the GLDAS-derived soil moisture storage (SMS) from the GRACE-derived total water storage (TWS). Figure 9 illustrates the variation in GWS, which, much like TWS, exhibits higher values between October and April and lower values from May to September.
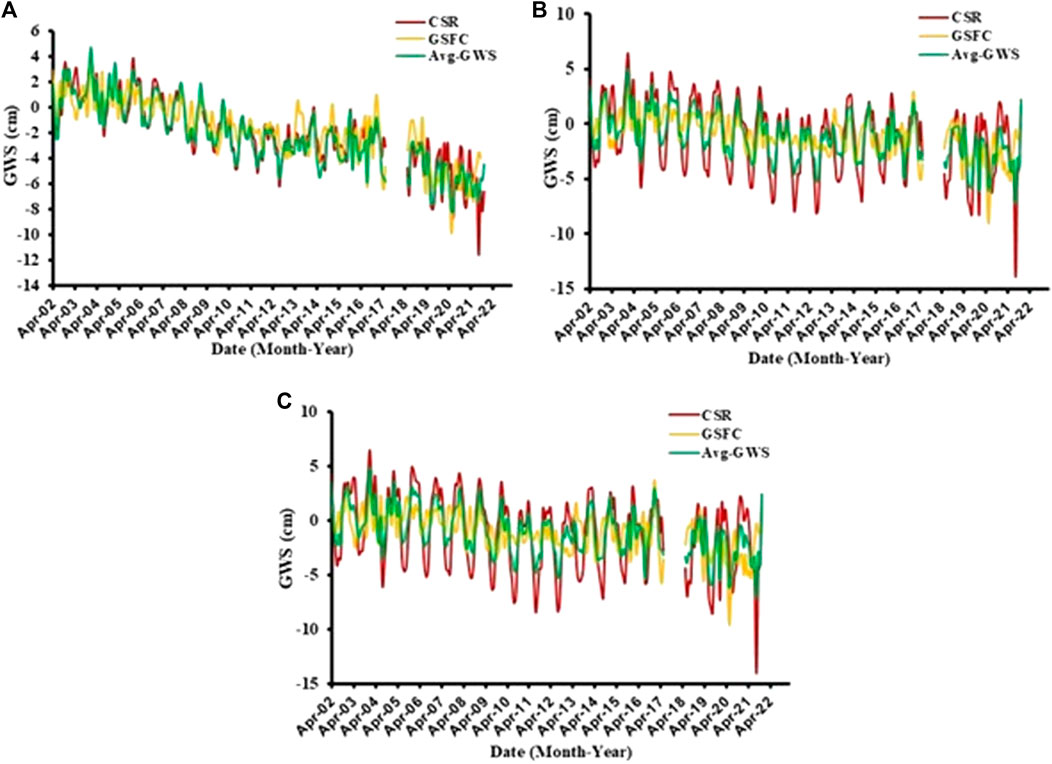
FIGURE 9. Temporal variations in GRACE-derived GWS for the Makah (A), surrounding zone of Wadi Fatimah (B), and the Wadi Fatimah (C) from the different solutions and their mean.
The time series of ΔGWS, derived using Eq 1, is presented in Figure 10. The analysis of this figure reveals that the average GWS across the entire Makkah region is declining at a rate of −0.38 ± 0.017 cm/year throughout the study period. Period I displays a negative GWS trend, estimated at −0.49 ± 0.049 cm/yr. In Period II, the GWS trend remains relatively stable, computed at −0.0172 ± 0.11 cm/yr, while Period III indicates a significant decrease in GWS, with a trend of −0.607 ± 0.15 cm/yr (Table 1; Figure 10).
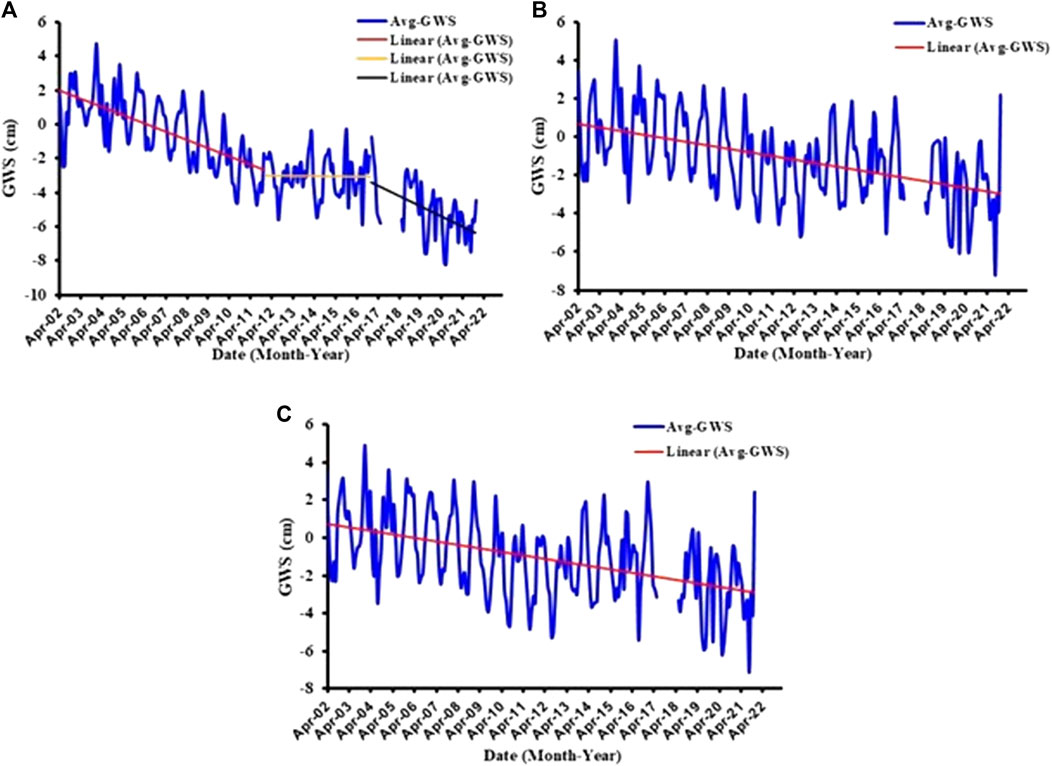
FIGURE 10. Time series of the averaging GWS and their trends for the Makah (A), surrounding zone of Wadi Fatimah (B), and the Wadi Fatimah (C) from 04/2002 to 12/2021.
For Wadi Fatma, employing the adjacent mascon solutions from GARCE CSR and GSFC, we determined a slightly negative trend in GRACE-derived GWS at −0.18 ± 0.022 cm/yr. The GWS patterns in the surrounding area closely resemble those of Wadi Fatma. Using this averaged mascon solution yielded a trend similar to that of Wadi Fatma, calculated at −0.185 ± 0.022 cm/yr (Table 2; Figure 10). Notably, Wadi Fatma experiences a slower rate of groundwater depletion compared to the entire Makkah region, attributed to its proximity to the coast and slightly higher precipitation rates. Furthermore, the structural influence of the southwest-trending fault zone plays a role in shaping the behavior of groundwater storage in Wadi Fatma, distinguishing it from the broader Makkah region.
4.5 Sediment thickness
We obtained data on sediment thickness from the website of the National Oceanic and Atmospheric Administration’s National Geophysical Data Center (Divins, 2003). The sedimentary layers exhibit variations in thickness within the Wadi area. The eastern part of the Wadi has sediments, approximately 100 m thick, whereas the central part has sediment thickness exceeding 700 m. As we move towards the western part of the Wadi, the sediment thickness reduces to around 600 m. In a small section in the southwestern part, notably, there are elevated values of sediment thickness, reaching up to 1800 m (as shown in Figure 11).
The significant sediment thickness observed in the central and southwestern portions of the Wadi, along with the slightly negative total water storage (TWS) signals in this area, suggests the presence of a substantial groundwater reservoir. The eastern part of the Wadi receives substantial precipitation, which is likely to drain into the central and downstream regions, contributing to the recharge of groundwater aquifers. However, it is worth noting that groundwater levels are declining at a rate of −0.18 ± 0.022 cm per year over the entire study period, primarily due to the heavy exploitation of groundwater resulting from the growing population and increased agricultural activities.
4.6 Watershed analysis
The topographical information provided by Figure 12, which depicts the elevation variations in the Wadi Fatimah basin, can be crucial in understanding and integrating water storage studies using GRACE data.The elevation differences observed in the upstream section, ranging from 1000 to 2000 m above sea level, are indicative of the landscape’s topographic complexity. In areas with significant elevation changes, water storage dynamics can exhibit unique patterns and responses to various hydrological factors.
Furthermore, the presence of rugged igneous and metamorphic rock formations, as well as the network of narrow faulted wadis or channels, can influence groundwater flow and storage in the region. These geological features may affect the permeability of subsurface layers, impacting the movement and retention of water. The small wetland areas, responsible for channeling surface water towards the central and southwestern parts of the region, are essential components of the local hydrological system. These areas can act as natural conveyors of water, potentially contributing to groundwater recharge in the adjacent aquifers.
The periodic runoff events and the saturation of alluvial deposits above the weathered basement following rainfall occurrences are critical aspects of the water cycle in the region. These processes can significantly influence water storage variations, particularly in the middle and downstream segments of the Wadi.
5 Conclusion
Saudi Arabia has limited surface water, so groundwater is the main source of water for drinking, agriculture, and sustainable development. This study used GRACE gravity data and GLDAS model outputs to estimate changes in groundwater storage in Wadi Fatimah and the Makkah region from 2002 to 2021. The study found that average annual precipitation (AAP) is low in the Makkah region (99.3 mm/year), but slightly higher in the southern portion, including Wadi Fatimah (160–270 mm/year). The AAP in Wadi Fatimah was estimated to be 131 mm during the study period. The average annual groundwater depletion rate in the Makkah region is −0.38 ± 0.017 mm/year, but it is lower in Wadi Fatimah (−0.18 ± 0.022 mm/year). This is because heavy rainfall in the upstream portion of Wadi Fatimah produces surface runoff that flows into the middle and lower portions of the Wadi, recharging the shallow groundwater aquifers and draining into the sea during rainstorms. This small recharge rate partially compensates for some of the groundwater withdrawal. The thick sediment layer (more than 600 m) above the basement rocks in the central and downstream portions of Wadi Fatimah is also essential for maintaining groundwater for future generations. This sediment layer partially feeds the groundwater with surface runoff that flows along faults and upper permeable Quaternary deposits.
The integration of GRACE and GLDAS data allows for a comprehensive assessment of water storage and its temporal variations. The findings emphasize the significance of precipitation patterns, sediment thickness, and geological features in influencing groundwater dynamics. The study relied on a relatively short data record (2002–2021) and satellite-based data sources, which may have limited its ability to capture long-term groundwater trends and localized changes. Future research should incorporate longer observation periods, higher spatial resolution data, and ground-based measurements to improve the accuracy and comprehensiveness of the findings. In addition, engaging local communities and stakeholders will be helpful developing effective and sustainable water resource management strategies.
Data availability statement
The datasets presented in this article are not readily available but are available on request. Requests to access the datasets should be directed to ZmFsc2hlaHJpYUBrc3UuZWR1LnNh.
Author contributions
All authors listed have made a substantial, direct, and intellectual contribution to the work and approved it for publication.
Funding
This research was funded by the Deputyship for Research and Innovation, Ministry of Education in Saudi Arabia, project no. (IFKSURC-1-7303).
Acknowledgments
The authors extend their appreciation to the Deputyship for Research and Innovation, Ministry of Education in Saudi Arabia for funding this research work through project no. (IFKSURC-1-7303).
Conflict of interest
The authors declare that the research was conducted in the absence of any commercial or financial relationships that could be construed as a potential conflict of interest.
Publisher’s note
All claims expressed in this article are solely those of the authors and do not necessarily represent those of their affiliated organizations, or those of the publisher, the editors and the reviewers. Any product that may be evaluated in this article, or claim that may be made by its manufacturer, is not guaranteed or endorsed by the publisher.
References
Ahmed, M., Sultan, M., Yan, E., and Wahr, J. (2016). Assessing and improving land surface model outputs over africa using GRACE, field, and remote sensing data. field, remote Sens. data Surv. Geophys. 37 (3), 529–556. doi:10.1007/s10712-016-9360-8
Al-Garni, M. A. (2009). Geophysical investigations for groundwater in a complex subsurface. Terrain, wadi Fatima, KSA: a case history. Jordan J. Civ. Eng. 3, 118–136.
Alshehri, F., and Abdelrahman, K. (2023a). Integrated approach for the investigation of groundwater quality using hydrochemical and geostatistical analyses in Wadi Fatimah, western Saudi Arabia. Front. Earth Sci. 11, 1166153. doi:10.3389/feart.2023.1166153
Alshehri, F., and Abdelrahman, K. (2023b). Groundwater potentiality of wadi Fatimah, western Saudi Arabia: geophysical and remote sensing integrated approach. Water 15 (10), 1828. doi:10.3390/w15101828
Alshehri, F., Abuamarah, B. A., and Abd El-Hamid, H. T. (2023a). Impact of land use dynamics on land surface temperature using optical remote sensing data integrated with statistical analysis in Riyadh, Saudi Arabia. Adv. Space Res. 72, 1739–1750. doi:10.1016/j.asr.2023.04.051
Alshehri, F., El-Sorogy, A. S., Almadani, S., and Aldossari, M. (2023b). Groundwater quality assessment in western Saudi Arabia using GIS and multivariate analysis. J. King Saud University-Science 35 (4), 102586. doi:10.1016/j.jksus.2023.102586
Alshehri, F., and Mohamed, A. (2023). Analysis of groundwater storage fluctuations using GRACE and remote sensing data in wadi as-sirhan, northern Saudi Arabia. Water 15 (2), 282. doi:10.3390/w15020282
Alyamani, M. S., and Hussein, M. T. (1995). Hydrochemical study of groundwater in recharge area, Wadi Fatimah basin, Saudi Arabia. GeoJournal 37 (1), 81–89. doi:10.1007/BF00814887
Al-Zyoud, S., Rühaak, W., Forootan, E., and Sass, I. (2015). Over exploitation of groundwater in the centre of amman zarqa basin—Jordan: evaluation of well data and GRACE satellite observations. Resources 4 (4), 819–830. doi:10.3390/resources4040819
Bierkens, M. F. P., and Wada, Y. (2019). Non-renewable groundwater use and groundwater depletion: a review. Environ. Res. Lett. 14 (6), 063002. doi:10.1088/1748-9326/ab1a5f
Chinnasamy, P., Maheshwari, B., and Prathapar, S. (2015). Understanding groundwater storage changes and rechargein Rajasthan, India through remote sensing. WaterSwitzerl. 7, 5547–5565. doi:10.3390/w7105547
Chinnasamy, P., and Sunde, M. G. (2016). Improving spatiotemporal groundwater estimates after natural disasters using remotely sensed data-a case study of the Indian Ocean Tsunami. Earth Sci. India 9, 101–111. doi:10.1007/s12145-015-0238-y
Crowley, J. W., Mitrovica, J. X., Bailey, R. C., Tamisiea, M. E., and Davis, J. L. (2008). Annual variations in water storage and precipitation in the Amazon Basin: bounding sink terms in the terrestrial hydrological balance using GRACE satellite gravity data. J. Geodesy 82, 9–13. doi:10.1007/s00190-007-0153-1
de Vries, J. J., and Simmers, I. (2002). Groundwater recharge: an overview of processes and challenges. Hydrogeology J. 10, 5–17. doi:10.1007/s10040-001-0171-7
Divins, D. (2003). Total sediment thickness of the world’s oceans and marginal seas. Boulder, CO, USA: NOAA National Geophysical Data Center.
Fallatah, O. A., Ahmed, M., Cardace, D., Boving, T., and Akanda, A. S. (2019). Assessment of modern recharge to arid region aquifers using an integrated geophysical, geochemical, and remote sensing approach. J. Hydrol. 569, 600–611. doi:10.1016/j.jhydrol.2018.09.061
Fallatah, O. A., Ahmed, M., Save, H., and Akanda, A. S. (2017). Quantifying temporal variations in water resources of a vulnerable middle eastern transboundary aquifer system. Hydrol. Process 31, 4081–4091. doi:10.1002/hyp.11285
Famiglietti, J. S., Lo, M., Ho, S. L., Bethune, J., Anderson, K. J., Syed, T. H., et al. (2011). Satellites measure recent rates of groundwater depletion in California's Central Valley. Res. Lett. 38 (3). doi:10.1029/2010gl046442
FAO (Food and Agriculture Organization of the United Nations) (2020). The state of food and agriculture 2020: overcoming 500 water challenges in agriculture. Rome: FAO. doi:10.4060/cb1447en
Ferreira, V. G., Gong, Z., and Andam-Akorful, S. A. (2012). Monitoring mass changes in the Volta River basin using GRACE satellite gravity and TRMM precipitation. Bol. Ciências Geodésicas 18, 549–563. doi:10.1590/S1982-21702012000400003
Frappart, F., and Ramillien, G. (2018). Monitoring groundwater storage changes using the gravity Recovery and climate experiment (GRACE) satellite mission: a review. Remote Sens. 10, 829–515. doi:10.3390/rs10060829
Gonçalvès, J., Petersen, J., Deschamps, P., Hamelin, B., and Baba-Sy, O. (2013). Quantifying the modern recharge of the “fossil” Sahara aquifers. Geophys. Res. Lett. 40, 2673–2678. doi:10.1002/grl.50478
Grainger, D. J. (1992). A geological excursion in the Wadi Fatima area, near Jiddah, Saudi Arabia. Geol. Today 8 (6), 215–219. doi:10.1111/j.1365-2451.1992.tb00458.x
Houborg, R., Rodell, M., Li, B., Reichle, R., and Zaitchik, B. F. (2012). Drought indicators based on model-assimilated gravity recovery and climate experiment (GRACE) terrestrial water storage observations. Water Resour. Res. 48 (7), 2011WR011291. doi:10.1029/2011WR011291
Huo, A.-D., Dang, J., Song, J.-X., Chen, X. H., and Mao, H.-R. (2016). Simulation modeling for water governance in basins based on surface water and groundwater. Agric. Water Manag. 174, 22–29. doi:10.1016/j.agwat.2016.02.027
Joodaki, G., and WahrSwenson, J. S. (2014). Estimating the human contribution to groundwater depletion in the Middle East, from GRACE data, land surface models, and well observations. Water Resour. Res. 50, 2679–2692. doi:10.1002/2013WR014633
Kummerow, C., Barnes, W., Kozu, T., Shiue, J., and Simpson, J. (1998). The tropical rainfall measuring mission (TRMM) sensor package. J. Atmos. Ocean. Technol. 15 (3), 809–817. doi:10.1175/1520-0426(1998)015<0809:ttrmmt>2.0.co;2
Lakshmi, V. (2016). Beyond GRACE: using satellite data for groundwater investigations. Groundwater 54, 615–618. doi:10.1111/gwat.12444
Laurent Longuevergne, L., Scanlon, B. R., and Wilson, C. R. (2010). GRACE hydrological estimates for small basins: evaluatingprocessing approaches on the high plains aquifer, USA. Water Resour. Res. 46, W11517. doi:10.1029/2009WR008564
Leblanc, M. J., Tregoning, P., Ramillien, G., Tweed, S. O., and Fakes, A. (2009). Basin-scale, integrated observations of the early 21st century multiyear drought in southeast Australia. Water Resour. Res. 45, W04408. doi:10.1029/2008WR007333
Long, D., Chen, X., Scanlon, B. R., Wada, Y., Hong, Y., Singh, V. P., et al. (2016). Have GRACE satellites overestimated groundwater depletion in the Northwest India Aquifer? Sci. Rep. 6, 24398. doi:10.1038/srep2.4398
Luthcke, S. B., Sabaka, T. J., Loomis, B. D., Arendt, A. A., McCarthy, J. J., Camp, J., et al. (2013). Antarctica, Greenland and gulf of Alaska land-ice evolution from an iterated GRACE global mascon solution. J. Glaciol. 59 (216), 613–631. doi:10.3189/2013jog12j147
Margat, J., and Van der Gun, J. (2013). Groundwater around the world: a geographical synopsis. Boca Raton, Fla: CRC Press, 497.
Milewski, A., Sultan, M., Yan, E., Becker, R., Abdeldayem, A., Soliman, F., et al. (2009). A remote sensing solution for estimating runoff and recharge in arid environments. J. H. Ydrol. 373, 1–14. doi:10.1016/j.jhydrol.2009.04.002
Mohamed, A. (2019). Hydro-geophysical study of the groundwater storage variations over the Libyan area and its connection to the Dakhla basin in Egypt. J. Afr. Earth Sci. 157, 103508. doi:10.1016/j.jafrearsci.2019.05.016
Mohamed, A. (2020a). Gravity applications in estimating the mass variations in the Middle East: a case study from Iran. Arab. J. Geosci. 13, 364. doi:10.1007/s12517-020-05317-7
Mohamed, A. (2020b). Gravity applications to groundwater storage variations of the Nile Delta Aquifer. J. Appl. Geophys. 182, 104177. doi:10.1016/j.jappgeo.2020.104177
Mohamed, A. (2020c). Gravity based estimates of modern recharge of the Sudanese area. J. Afr. Earth Sci. 163, 103740. doi:10.1016/j.jafrearsci.2019.103740
Mohamed, A., Abdelrahman, K., and Abdelrady, A. (2022a). Application of time- variable gravity to groundwater storage fluctuations in Saudi Arabia. Front. Earth Sci. 10, 873352. doi:10.3389/feart.2022.873352
Mohamed, A., Ahmed, E., Alshehri, F., and Abdelrady, A. (2022b). The groundwater flow behavior and the recharge in the Nubian Sandstone Aquifer System during the wet and arid periods. Sustainability 14, 6823. doi:10.3390/su14116823
Mohamed, A., Al Deep, M., Othman, A., Taha, A. I., Alshehri, F., and Abdelrady, A. (2022c). Integrated geophysical assessment of groundwater potential in southwestern Saudi Arabia. Front. Earth Sci. 10, 937402. doi:10.3389/feart.2022.937402
Mohamed, A., Faye, C., Othman, A., and Abdelrady, A. (2022d). Hydro-geophysical evaluation of the regional variability of Senegal’s terrestrial water storage using time-variable gravity data. Remote Sens. 14, 4059. doi:10.3390/rs14164059
Mohamed, A., and Gonçalvès, J. (2021). Hydro-geophysical monitoring of the North Western Sahara Aquifer System’s groundwater resources using gravity data. J. Afr. Earth Sci. 178, 104188. doi:10.1016/j.jafrearsci.2021.104188
Mohamed, A., Sultan, M., Ahmed, M., Yan, E., and Ahmed, E. (2017). Aquifer recharge, depletion, and connectivity: inferences from GRACE, land surface models, and geochemical and geophysical data. Bull. Geol. Soc. Am. 129, 534–546. doi:10.1130/B31460.1
Moiwo, J. P., Yang, Y., Tao, F., Lu, W., and Han, S. (2011). Water storage change in the himalayas from the gravity recovery and climate experiment (GRACE) and an empirical climate model. Water Resour. Res. 47 (7), 2010WR010157. doi:10.1029/2010WR010157
Mushin, I. (2022). Editorial: turn design and epistemic management in small communities. J. Pragmat. 193, 21–26. doi:10.1016/j.pragma.2022.03.007
Rodell, M., Chen, J., Kato, H., Famiglietti, J. S., Nigro, J., and Wilson, C. R. (2009). Estimating groundwater storage changes in the Mississippi River basin (USA) using GRACE. Hydrogeol. J. 15, 159–166. doi:10.1007/s10040-006-0103-7
Rodell, M., Famiglietti, J. S., Wiese, D. N., Reager, J. T., Beaudoing, H. K., Landerer, F. W., et al. (2018). Emerging trends in global freshwater availability. Nature 557 (7707), 651–659. doi:10.1038/s41586-018-0123-1
Rodell, M., Houser, P. R., Jambor, U., Gottschalck, J., Mitchell, K., Meng, C.-J., et al. (2004). The global land data assimilation system. Bull. Am. Meteorol. Soc. 85 (3), 381–394. doi:10.1175/bams-85-3-381
Save, H., Bettadpur, S., and Tapley, B. D. (2016). High-resolution CSR GRACE RL05 mascons. J. Geophys. Res. Solid Earth 121 (10), 7547–7569. doi:10.1002/2016JB013007
Scanlon, B. R., Healy, R. W., and Cook, P. G. (2002). Choosing appropriate techniques for quantifying groundwater recharge. Hydrogeology J. 10, 18–39. doi:10.1007/s10040-001-0176-2
Sharaf, M. A. (2013). Major elements hydrochemistry and groundwater quality of wadi Fatimah, west central arabian shield, Saudi Arabia. Arab. J. Geosci. 6 (7), 2633–2653. doi:10.1007/s12517-012-0544-3
Sharaf, M. A., Alyamani, M. S., and Subyani, A. M. (2004). Regional study of the rare and trace elements in the groundwater of the major wadi basins (An Numan, Usfan, and Fatimah) in western Saudi Arabia and their suitability for various purposes. Final report, Project, 204–423.
Shiklomanov, I. A., and Rodda, J. C. (2003). World water Resources at the beginning of the twenty-first century. Cambridge: Cambridge University Press. Google Search (Accessed November 12, 2023).
Sultan, M., and Save, H. (2020). GRACE-derived seasonal variations, a key to understanding aquifer sources, recharge and groundwater flow patterns. Copernicus Meetings.
Swenson, S., Chambers, D., and Wahr, J. (2008). Estimating geocenter variations from a combination of GRACE and ocean model output. J. Geophys. Res. Solid Earth 113 (B8), 2007JB005338. doi:10.1029/2007JB005338
Syed, T. H., Famiglietti, J. S., Rodell, M., Chen, J., and Wilson, C. R. (2008). Analysis of terrestrial water storage changes from GRACE and GLDAS. Water Resour. Res. 44. doi:10.1029/2006WR005779
Taha, A. I., Al Deep, M., and Mohamed, A. (2021). Investigation of groundwater occurrence using gravity and electrical resistivity methods: a case study from Wadi Sar, Hijaz Mountains, Saudi Arabia. Arab. J. Geosci. 14, 334. doi:10.1007/s12517-021-06628-z
Tapley, B. D., Bettadpur, S., Ries, J. C., Thompson, P. F., and Watkins, M. M. (2004). GRACE measurements of mass variability in the Earth system. Science 305, 503–505. doi:10.1126/science.1099192
Tiwari, V. M., Wahr, J., and Swenson, S. (2009). Dwindling groundwater resources in northern India, from satellite gravity observations. Geophys. Res. Lett. 36, L18401. doi:10.1029/2009gl039401
Trigo, R. M., Gouveia, C. M., and Barriopedro, D. (2010). The intense 2007–2009 drought in the fertile crescent: impacts and associated atmospheric circulation. Agric. For. Meteorology 150 (9), 1245–1257. doi:10.1016/j.agrformet.2010.05.006
United Nations (2022). The united Nations world water development report 2022: groundwater: making the invisible visible. Paris: UNESCO.
Voss, K. A., Famiglietti, J. S., Lo, M., De Linage, C., Rodell, M., and Swenson, S. (2013). Groundwater depletion in the Middle East from GRACE with implications for transboundary water management in the Tigris–Euphrates–western Iran region. Water Resour. Res. 49, 904–914. doi:10.1002/wrcr.20078
Wada, Y., Van Beek, L. P., Van Kempen, C. M., Reckman, J. W., Vasak, S., and Bierkens, M. F. (2010). Global depletion of groundwater resources. Geophys. Res. Lett. 37 (20). doi:10.1029/2010gl044571
Wahr, J., Molenaar, M., and Bryan, F. (1998). Time variability of the Earth’s gravity field: hydrological and oceanic effects and their possible detection using GRACE. J. Geophys. Res. 103, 30205–30229. doi:10.1029/98JB02844
Wahr, J., Swenson, S., and Velicogna, I. (2006). Accuracy of GRACE mass estimates. Gephys Res. Lett. 33. doi:10.1029/2005GL025305
Wahr, J., Swenson, S., Zlotnicki, V., and Velicogna, I. (2004). Time-variable gravity from GRACE: first results. Geophys Res. Lett. 31. doi:10.1029/2004GL019779
Watkins, M. M., Wiese, D. N., Yuan, D. N., Boening, C., and Landerer, F. W. (2015). Improved methods for observing earth’s time variable mass distribution with GRACE using spherical cap mascons. J. Geophys. Res. Solid Earth 120 (4), 2648–2671. doi:10.1002/2014JB011547
Wiese, D. N., Landerer, F. W., and Watkins, M. M. (2016). Quantifying and reducing leakage errors in the JPL RL05M GRACE mascon solution. Water Resour. Res. 52 (9), 7490–7502. doi:10.1002/2016WR019344
Wouters, B., Bonin, J. A., Chambers, D. P., Riva, R. E. M., Sasgen, I., and Wahr, J. (2014). GRACE, time-varying gravity, Earth system dynamics and climate change. Rep 77, 116801. doi:10.1088/0034-4885/77/11/116801
Xavier, L., Becker, M., Cazenave, A., Longuevergne, L., Llovel, W., Rotunno Filho, O. C., et al. (2010). Interannual variability in water storage over 2003–2008 in the amazon basin from GRACE space gravimetry, in situ river level and precipitation data. Remote Sens. Environ. 114 (8), 1629–1637. doi:10.1016/j.rse.2010.02.005
Keywords: gravity data, groundwater storage variations, depletion, Wadi Fatimah, Makkah province, Saudi Arabia
Citation: Alshehri F and Mohamed A (2023) Investigation of groundwater potential using gravity data in Wadi Fatimah and its surroundings, Western Saudi Arabia. Front. Earth Sci. 11:1225992. doi: 10.3389/feart.2023.1225992
Received: 20 May 2023; Accepted: 08 November 2023;
Published: 23 November 2023.
Edited by:
Juergen Pilz, University of Klagenfurt, AustriaReviewed by:
Prabuddh Kumar Mishra, University of Delhi, IndiaZhiming Han, Northwest A and F University, China
Copyright © 2023 Alshehri and Mohamed. This is an open-access article distributed under the terms of the Creative Commons Attribution License (CC BY). The use, distribution or reproduction in other forums is permitted, provided the original author(s) and the copyright owner(s) are credited and that the original publication in this journal is cited, in accordance with accepted academic practice. No use, distribution or reproduction is permitted which does not comply with these terms.
*Correspondence: Fahad Alshehri, ZmFsc2hlaHJpYUBrc3UuZWR1LnNh