- 1Institute of Marine Sciences, National Research Council, Naples, Italy
- 2Department of Environmental Civil Engineering Land, Building and Chemistry, Bari, Italy
- 3Water Research Institute, National Research Council, Taranto, Italy
The grain size, nutrients, and metals contents of sediments are important tracers for reconstructing the origin of the contaminants and the pathway of the sediments from the source to the sink. This is particularly important in areas with high geological variability and a high demographic pressure. The origin and distribution of contaminants and nutrients allow us to identify the source-to-sink pathway of the sediments. In particular, the Sarno River is one of the main contributing sources of anthropogenic contaminants due to the outflow from the large surrounding large industrial area. Geochemical and physical parameters of sediments were analyzed along a transect from the coastline to a water depth of 112 m, with the objective of exploring the spatial variability of sediment contamination in the continental shelf of Naples Bay. The latter is characterized by a complex geological area and is subject to river inflow from a highly industrialized area. The data have been analyzed using principal component analysis (PCA), hierarchical correlation analysis (CA), and analysis of variance (ANOVA). To distinguish between a natural and anthropogenic origin of contaminants and to construct the source-to-sink pathway, the relationship between geochemical and physical data and their distribution was examined, and the results were compared with published data collected onshore. Four distinct sectors with different associations of metals, grain size, and total organic carbon have been recognized: I) offshore Vesuvius volcano, the occurrence of coarse-grained sediments, associated with As, Fe, Mn, and low TOC values, suggests a natural origin of the contaminant due to the volcanic nature of the substrate; II) offshore the Sarno River, the presence of Cr, Cd, Cu, Pb and Zn, associated with the high TOC content, suggests an anthropogenic origin for the contaminants; III) offshore Sorrento Peninsula, the presence of As, Fe, Mn, and some gravel made up of pumice and bioclasts indicate a predominance of contaminants of natural origin; and IV) finally, the distal part is not subjected to a primary terrestrial input. Metals contamination of anthropogenic origin and the organic matter in sediments decreased rapidly with distance from the coast highlighting the main deposition area close to the river discharge.
1 Introduction
Anthropogenic processes in raw industrial wastewater, municipal wastewater, surface runoff, and so on, as well as the erosion of the rocks that make up the substrate, are responsible for the discharge of metals into coastal environments. The concentrations of metals in sediments are mainly influenced by the mineralogical and chemical composition of suspended materials, human activities, and various chemical processes (Meybeck and Helmer, 1989; Cobelo-Garcia and Prego, 2003; Prego and Cobelo-Garcia, 2003; Hu et al., 2015; Lu et al., 2017). Heavy metal elements (e.g., Pb, Cd, Ni, Cr, Cu, Zn, and As) are important contaminants in the environment because of their characteristics of multiple provenance supply, long residual time, difficult degradation, and easy accumulation. They have a high potential for toxicity to ecological systems because high concentrations might inhibit or modify the growth of organisms and be harmful to human health through transmission in the food chain (Chapman et al., 1998; Sundelin and Eriksson, 2001; Rainbow and Luoma, 2011; Roberts, 2012; Spada et al., 2012; Hill et al., 2013; Wang et al., 2013; Giandomenico et al., 2016; Lu et al., 2017; Mali et al., 2022). Despite inherent problems in inferring sediment provenance from the final product, the attempt at reconstructing mass transfer from source areas to the sedimentary basin has attracted increasing interest over the last decade. Recently, a study of the Holocene succession of the Po River Basin highlighted the spatial and temporal distribution of chromium and nickel concentrations as a powerful tool for reconstructing changes in sediment source and dispersal patterns through time (Amorosi, 2012).
On the other hand, organic matter and nitrogen content have been used widely as biomarkers for the reconstruction of the depositional environments and the environmental changes of the past (Dean, 1974; 1999; Avramidis et al., 2013). More than 90% of global marine organic carbon is indeed trapped in coastal sediments through complex physical, chemical, and biological processes (Gao et al., 2012), and the ratio between total organic carbon (TOC) and total nitrogen (TN) is often used to distinguish between a terrestrial, fluvial, or marine origin of organic matter (Lamb et al., 2006; Gao et al., 2012; Nazneen and Raju, 2017; Lu et al., 2020). Both factors, grain size and organic matter content, play an important role in the contamination pattern as the first governs metal mobility (Solomon and Forstner, 1984; Mali et al. 2015; Mali et al. 2016; Mali et al. 2017a; Mali et al. 2017b; Mali et al. 2018; Mali et al. 2019; Mali et al., 2022; Cotecchia et al., 2021).
Southeast Naples Bay represents a relatively wide continental shelf environment with terrestrial sediment supply from the Vesuvius volcano, the Sarno River, and the Sorrento Peninsula relief (Figure 1). The Sarno plain is the alluvial plain of the Sarno River and extends for approximately 440 km2. The river has a daily average flow of approximately 1 m3s−1 and a relatively short straight course (24 km) crossing heavily farmed land before discharging into the Bay of Naples (Arienzo et al., 2001). The area has a Mediterranean climate regime, with an average annual temperature of 17.2°C and average annual rainfall of 1,203 mm, mostly concentrated at the end of the summer (Ufficio Idrografico e Mareografico di Napoli 1960–1995). Much of the coastal zone of the basin and many inland areas are strongly urbanized and industrialized, with a total of 160 tanning plants operating in the upper valley of the basin (Arienzo et al., 2001). The rest of the plain, in particular the middle Sarno Valley, with a high population density (up to 2,000 inhabitants per km2; ISTAT 2011), is used for very intensive agriculture, consisting of field horticulture, orchards, vineyards, chestnuts, and greenhouse horticulture and floriculture. The inner part of the Sarno Valley is largely affected by the presence of numerous leather tanneries; almost 90% of the inhabitants are involved in this sector. In the middle Sarno Valley, crop production is the main economic source because of soil fertility and the Mediterranean climate. Finally, the lower Sarno Valley economy is characterized by several chemical-pharmaceutical, engineering, and manufacturing industries (De Pippo et al., 2006). The Somma-Vesuvius volcanic complex is formed by an older stratovolcano (Mount Somma) cut by a breached crater and by a stratocone (Vesuvius), which developed during historical times. It grew up to approximately 2000 m in height over a time span of ca. 20 ky (Cioni et al., 1999), mainly through the piling up of lava flows and spatter and loose scoria deposits. The main effusive activity of the Somma stratovolcano was interrupted at approximately 22 ky BP by the trachytic Pomici di Base Plinian eruption (Santacroce et al., 2008). This event was responsible for the formation of the breached crater and the deposition of a large debris avalanche in the submarine counterpart (Milia et al., 2003; 2012). The Vesuvius is quiescent since March 1944. The Sorrento Peninsula, bounding the southern margin of the Bay of Naples, is characterized by a thick Meso-Cenozoic succession of limestone and dolomite covered by clastic sediments. The latter are made up of clastic deposits of Miocenic succession in the western part of the Peninsula and by tephra deposits from Vesuvius and Campi Flegrei that cover the carbonatic relief.
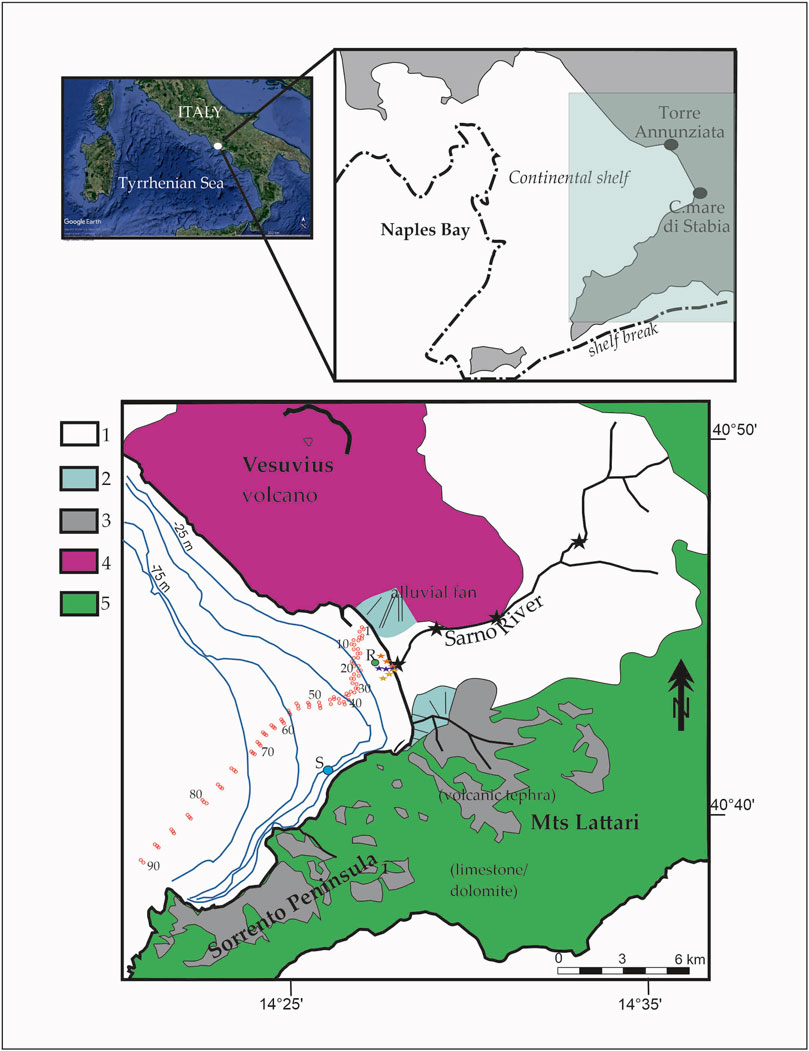
FIGURE 1. The study area extends on the continental shelf of Naples Bay (Eastern Tyrrhenian Sea) between the Vesuvius volcano and Sorrento Peninsula offshore the Sarno plain. The red circles represent the locations of the samples collected for this study. R, Rovigliano rock; S, Banco di Santa Croce reef. Black stars indicate the onshore data set; the red, blue, and yellow stars indicate the different directions of metal distributions. From Montuori et al. (2013).
A geochemical prospect was conducted in the Sarno Plain by several authors (Adamo et al., 1999; 2003; Arienzo et al., 2001; Aiuppa et al., 2003; De Pippo et al., 2006; Albanese et al., 2007; 2013). In particular, Albanese et al. (2013) analyzed sediment samples collected in the Sarno alluvial plain and processed the data to generate the maps of the contamination factors and contamination degrees for As, Cd, Cr, Cu, Hg, Pb, and Zn. Furthermore, the authors performed a factor analysis to assess the nature and the extent of contamination sources for the river sediments. Moreover, Montuori et al. (2013) analyzed metal contamination (As, Hg, Cd, Cr, Cu, Ni, Pb, and Zn) along the river from the inner part to the distal part and in the proximal shore area (sample locations are indicated with stars in Figure 1). The authors performed the analyses in the water dissolved phase, with suspended particulate matter and sediment samples showing an increase of metal contamination from the inner part of the river toward the coast; offshore the metals are mainly redistributed toward the south.
The study area is the sector of the continental shelf offshore Sarno plain. Unlike many studies that focused on the distribution and concentration of metals in the offshore area (e.g., Zonta et al., 2018; Sprovieri et al., 2020; Wang et al., 2023), some studies have attempted to identify the origin and path of these between sources and depositional areas (Tamburrino et al., 2019; Oliveri et al., 2022). Our goal is to identify areas with different physical and geochemical characteristics to determine the origin, provenance, and path of the contaminants. In particular, we investigate the current grain size, nutrients (TOC, TN, and TP), and metals (Hg, Cd, As, Cr, Ni, Cu, Zn, Pb, Fe, and Mn) to distinguish the possible origin (natural vs. anthropogenic) of the latter using multivariate analysis. For this purpose, and to be able to reconstruct the path of the sediments, we have taken into consideration the results of previous studies in the Sarno plain.
2 Materials and methods
A total of 91 surface sediment samples, named GN1 to GN91 from the coast to the distal area, have been collected using a Van Veen grab along a transect (about 9 km) offshore the Sarno plain coast (Figure 1) at the end of 2014. The sediment samples were analyzed for grain size, metals (Hg, Cd, As, Cr, Ni, Cu, Zn, Pb, Fe, and Mn), total organic carbon (TOC), and nutrients, such as total nitrogen (TN) and total phosphorus (TP). For physical analyses, a first aliquot of sediment sample was stored in clean polyethylene bags and subsequently cooled at 4/6°C, whereas for the chemical analyses, a second aliquot was stored in decontaminated HDPE (high-density polyethylene) containers and frozen at −18°/−25°C. The granulometric features of the sediments were analyzed following the ICRAM Manual procedure: for grain size analysis, samples were treated with H2O2 solution to remove organic constituents and support deflocculation, washed and dried at 40°C, and then sieved using an ASTM series of meshes from −1 to 4 phi, with an interval of 0.5 phi (phi = −log2 of the mm value). The finest fraction (<63 μm) was analyzed using a laser diffraction granulometer (laser particle-size analyzer). The analytical procedures are summarized in Table 1. All measured data from the samples are shown in the Supplementary Material.
Multivariate statistical analyses (PCA and CA) were carried out using SIMCA 17.01 (17, MKS Umetrics AB, Sweden) coupled with STATISTICA 16.0 for ANOVA analysis to investigate significant differences in metal concentration following the organic matter and granulometric trend. The data set for the PCA analysis includes 91 sites and variables referring to the absolute values of concentration of As, Cd, Cr, Cu, Hg, Ni, Pb, Zn, Fe, and Mn, TOC, TN, TP, including the percentage of the Wentworth size classes for granulometry (gravels, sand, silt and clay), and C/N ratio for organic matter provenance (Lamb et al., 2006). Finally, regression analyses applying Pearson correlation were conducted to examine the correlation between metal content and TOC content.
3 Results
3.1 Sediment granulometry
The Wentworth size classes (clay, silt, sand, and gravel) have been identified in the Bay of Naples continental shelf up to a depth of 112 m (Figure 2). The predominant grain sizes are sand and silt, representing more than 70% of the sediment samples. The granulometric distribution and the variation in the percentage of the classes permit us to distinguish four sectors (A, B, C, and D) along the transect.
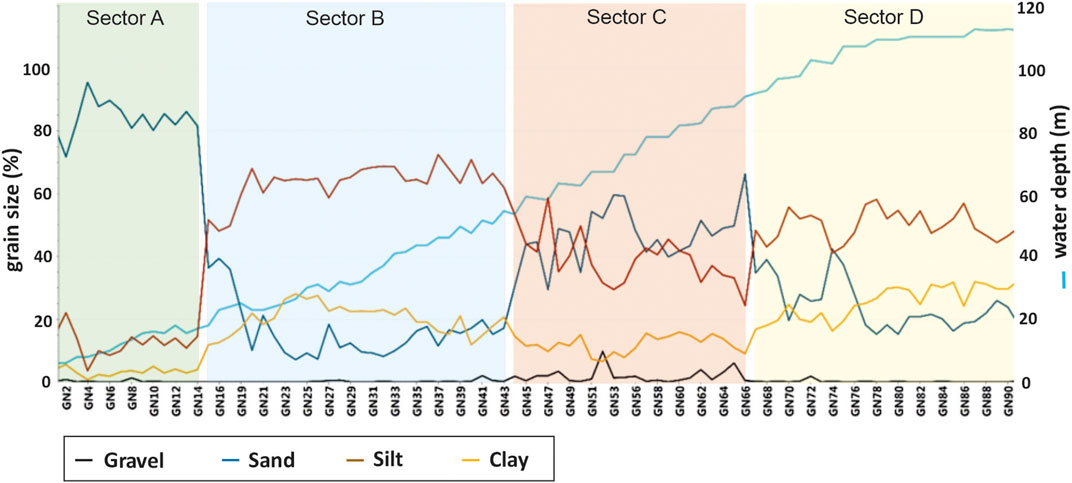
FIGURE 2. Depth and grain size of the sediment samples, expressed according to Wentworth size classes. The sectors (A, B, C, and (D) indicate areas with different granulometric characteristics.
Sector A lies close to the Torre Annunziata coast, between a water depth of 0 and 20 m (from GN1 to GN14), and it is characterized by the prevalence of sand, which accounts for more than 80% of the content until GN14 and decreases abruptly to less than 20% at GN20. In this sector, the silt is approximately 10%, whereas clay is between 0% and 5%. Sector B lies between the water depth of 20 m and 55 m (from GN15 to GN44) and is characterized by a relatively high silt component (between 60% and 70% of the total content), whereas sand accounts for approximately 20% of the content, as does clay. A change in the granulometric percentage occurs at the boundary of sectors B and C. In sector C (from GN45 to GN66), between a water depth of 55 m and 90 m, the sand accounts for 35% and 60% of the content, respectively, and silt is present at a similar proportion, whereas clay accounts for less than 20% of the content. Even at a low percentage (up to 10%), it is important to note the presence of gravel. The gravels are made up of pumice, slag, and bioclastic and organogenic material (bivalves, bryozoan, gastropods, posidoniae remains, and numerous coral fragments) (Figure 3). Moving toward a distal area, in sector D at a water depth of more than 90 m (from GN67 to GN91), the sand decreases in percentage from 40% to 20%. By contrast, silt increases up to 60% and clay increases from 20% to 30%.
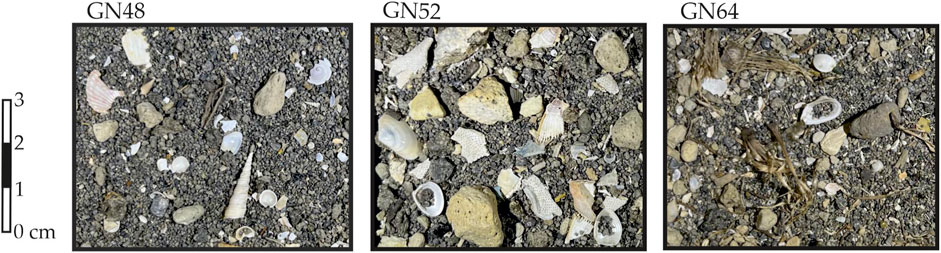
FIGURE 3. Gravel fraction of sector C made up of pumice and bioclasts (corals, bivalves, and gasteropoda).
Along the track, the distribution pattern of the sediments from the Vesuvius coast to the offshore of the Sorrento Peninsula displays two abrupt changes in the percentage of grain sizes at the boundaries of sectors A and B and B and C. The boundary between sectors A and B represents an abrupt change in the sand and silt content, with a rapid decrease in sand from 80% to 10%, whereas silt increases abruptly from 20% to more than 60% and is the prevalent grain size in sector B. In particular, the fine fraction <63 μm (silt and clay) increases from a maximum of 27% in sector A to more than 93% in sector B, with silt as the prevalent component (Figure 4). At the boundary of sectors B and C, it is possible to observe an abrupt change in the percentage of sand and silt that reaches similar values in sector C. By contrast, the fine fraction of <63 μm accounts for between 40% and 68% in sector C. Furthermore, this latter sector is characterized by the presence of gravel. The boundary between sectors C and D is more gradual: the percentage of sand decreases, whereas the fraction of <63 μm reaches 85%, with the clay fraction increasing up to 30% in the distal area.
3.2 Total organic carbon and nutrients (TN and TP)
The organic matter content in sediments is usually expressed in terms of total organic carbon (TOC) (Van Nugteren et al., 2009). The distribution of TOC within sediments of the study area ranges from 0.01 up to 5.88%w. The highest concentrations of TOC are present in sector B where TOC reaches 5.88 %w (Figure 5). A comparison with the adjacent area in Naples Bay reveals that this value is higher with respect to that detected in an adjacent area, offshore Naples, where the TOC ranges between 0.05% and 4% (Sprovieri et al., 2007). A positive relationship between TOC content and the silty fraction was detected (R = 0.72 at p=0.05) (Table 2).
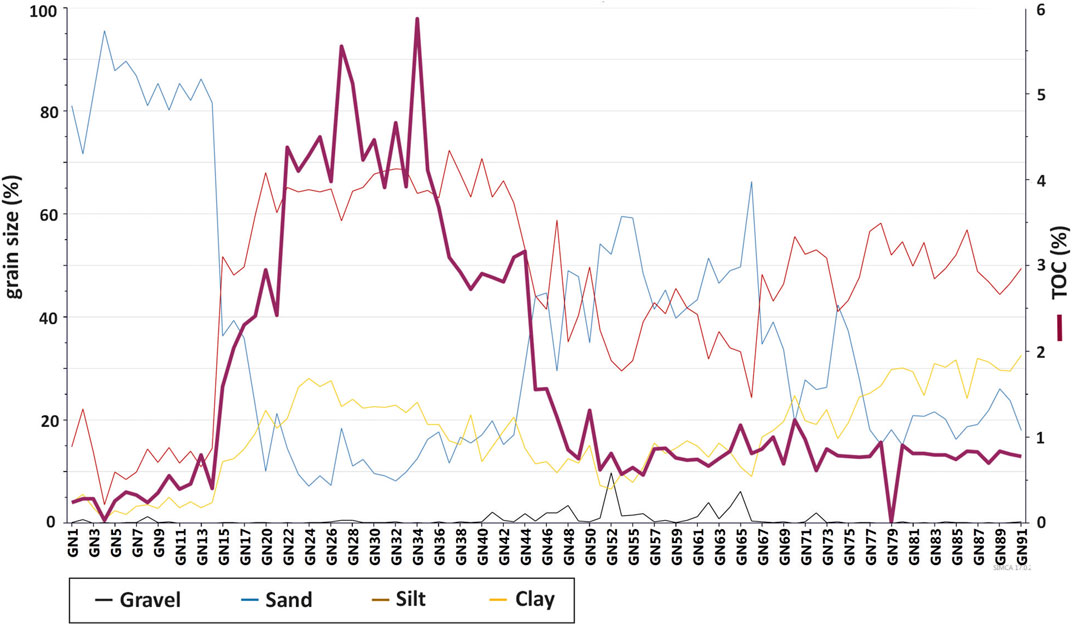
FIGURE 5. TOC distribution plotted on grain size. It is important to note the bell shape with the higher values of TOC in sector B.
The concentration values of TN and TP are reported in Figure 6 in the form of box plots. The distribution of TN follows the TOC trend, as shown by the high correlation between TOC vs. TN plots (R = 0.82 at p=0.05) (Table 2). TP was below the detection limit in almost all sectors (A, C, and D), except for sector B, where the TP values range between 500 and 1,534 mg/kg dry weight (d.w.). In general, a good positive relationship (R = 0.67 at p< 0.05) was observed between TN and TP (Table 2).
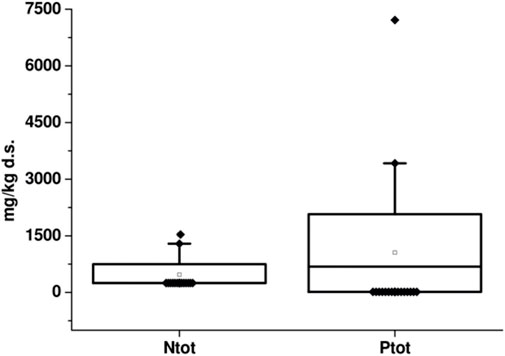
FIGURE 6. Box plot of TN and TP. The box represents the 25th and 75th percentiles, with the mean value within the box The outliers are represented by black diamond symbols.
It is worth noting that both TOC and nutrients registered a positive relationship with the finest grain size (silt and clay), as shown by the Pearson coefficient reported in the correlation matrix (Table 1). In turn, a negative relationship was logged between TOC, TN, and TP and sand content, with R values of −0.64, −0.63, and −0.52, respectively. Additionally, TN and TP content were under the detection limit in sector A, where sand had the highest values (ranging from 72% to 96%), and sector C (characterized by sandy silt).
In the investigated area, the C/N ratios (Figure 7) vary within wide ranges. Each of the sectors shows distinct C/N ratio trends, with particularly high values in sector A (from 19 to 528) and sector C (from 502 to 1,550) but low values in sector B (from 12 to 20) and sector D (from 3 to 11). According to Meyers (1994) and Tyson (1995), the organic matter content is characterized by low C/N ratios in the marine environment because it tends to be nitrogen rich.
3.3 Metals
Figure 8 summarizes the absolute, minimum, maximum, and average metal concentrations recorded in the sediment samples using box-plot graphs. The concentration ranges, represented by dry weight in mg/kg, are as follows: Hg (0.009–0.7), Cd (2.68–8.38), Pb (21.4–231.36), As (9.15–49.5), Zn (88.9–386), Cr (32.7–749.73), Cu (19.6–243.55), Ni (0.001–73.6), Fe (27,692–103,115), and Mn (255–1,546).
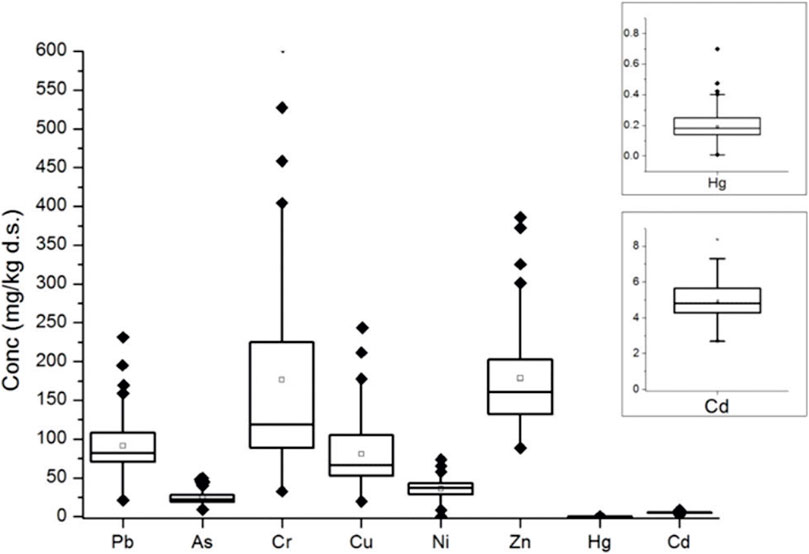
FIGURE 8. Box plots of Pb, As, Cr, Cu, Ni, and Zn. The boxes on the right represent the concentrations of Hg and Cd (with different magnitudes with respect to the other metals). The box represents the 25th and 75th percentiles, with the mean values within box. The outliers are represented by black diamond symbols.
Outlier concentrations were logged for Pb (which reached the highest value of 231.36 mg/kg at site GN36), Cr (which reached the highest value of 749.73 mg/kg at site GN34), Cu (highest concentration of 243.55 mg/kg at site GN34), and Zn (maximum value of 386 mg/kg at site GN36). Cd shows a more uniform distribution across the study area with a maximum value of 8.38 mg/kg at site GN82. Hg presents the highest value of 0.7 mg/kg at site GN76.
3.4 Multivariate analysis
PCA, CA, and ANOVA analyses were performed to investigate the correlation between sediment characteristics (grain size, organic matter, and nutrients) and metal/metalloid distribution. The data set, including the 91 sites and 21 variables (As, Cd, Cr, Cu, Hg, Ni, Pb, Zn, Fe, Mn, TOC, TN, TP, percentages of gravel, sand, silt, and clay, C/N ratio, w%, ps%, and depth), was enhanced with two qualitative variables. Indeed, to support a better understanding of multivariate analysis (PCA, CA, and ANOVA), two independent factors were included in the experimental design. The first one is represented by the granulometry, which was subdivided into the following five groups respectively named “silty”, “sandy”, “silty-sand”, “silty-clay,” and “sandy-gravel”: 1) “silty” includes samples in which the silty fraction is predominant (more than 55%); 2) “sandy” includes samples in which the sand fraction is predominant (more than 55%); 3) “silty-clay” and 4) “sandy-clay” include samples in which the two granulometric fractions are predominant; and 5) “sandy-gravel” includes samples in which the gravel fraction accounts for 2%–10% of the sample and the sandy fraction is predominant (more than 50%). The second independent factor included was “organic matter content” expressed in terms of TOC, which was grouped into three different range concentrations: “low TOC”, with concentrations of TOC from 0% to 1%; “medium TOC”, with concentrations ranging between 1% and 3%; and “high TOC”, with a TOC content more than 3%.
To validate the model performances, all data were centered on the mean, and the cross-validation method was applied before PCA and CA analysis. A model with four principal components (PCs) accounting for a cumulative variance of 72% was considered. An inspection of the biplot graphs (score plot and loading plots) and the relative hierarchical cluster dendrogram (Figures 9A, B), revealed four groups that seem to be governed by different pattern variables. It is important to note that the identified groups, more evident along the PC1, correspond to the previous individuated sectors (A, B, C, and D) (Figure 10). The distinction between the four groups is more evident along PC1, where they are displayed with different contaminant patterns. The biplot graphs (Figure 9A) show that in the PC1 vs. PC2 hyperspace, group 1 includes samples from sector A. Group 1, characterized by the negative value of PC1 and positive value PC2, is highly associated with “Sand,” Fe, and As, and to a lesser extent with Mn and Ni. Furthermore, group 1 is associated with “low TOC content” and shows an inverse trend with the nutrient concentrations. Group 2, characterized by PC1 and PC2 positive values, includes the samples from sector B. This group is characterized by the presence of Cr, Cu, Pb, Hg, Zn, Cd, “high TOC content”, and “silt”. Groups 3 and 4 are displayed in hyperspace with a negative value of PC2, and include the samples from sectors C and D, respectively. Both groups are characterized by the “medium value of TOC”. Group 3 is associated with the “sandy-gravel” fraction and has a high value of C/N, and group 4 is characterized by a silty-sand fraction and low values of C/N. Both groups do not have significant relationships with metals, albeit group 4 has a low positive relationship with Hg and Cd, and group 3 has a positive relationship with Mn and Ni.
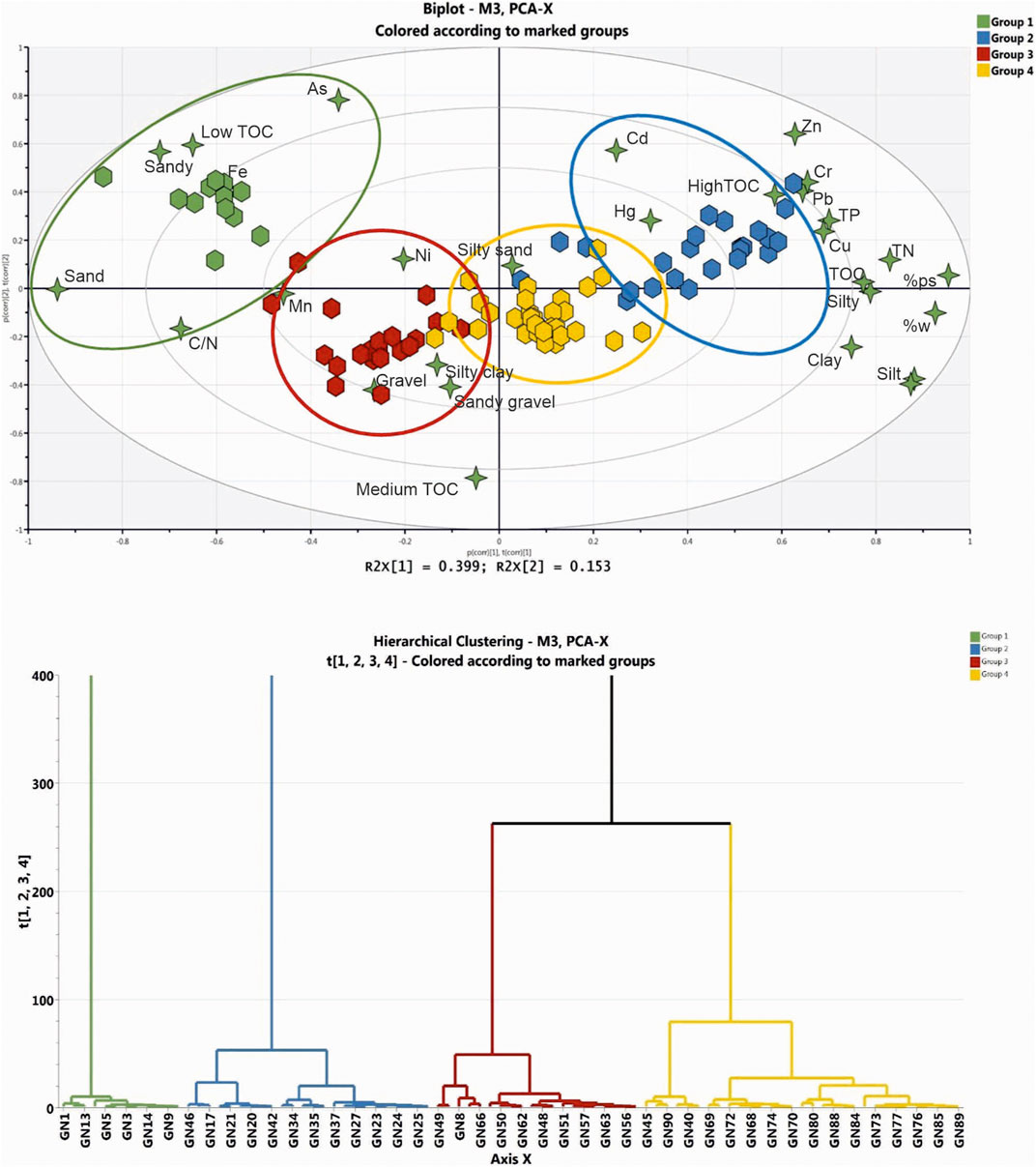
FIGURE 9. In the upper panel, biplots (score and loadings) of PC1 vs. PC2 from PCA analysis in the study area. In the lower panel, dendrograms of the hierarchical analysis.
3.5. ANOVA analysis
The ANOVA analysis, using TOC as an independent variable (Figure 11), distinguished three groups of metals:
i) Fe, As, Mn, and Ni decrease their concentration in correspondence to increasing TOC content;
ii) Cr, Cd, Cu, Pb, and Zn concentrations increase with increasing TOC content;
iii) An irregular trend is shown by Hg, which is present, more or less, along the whole track.
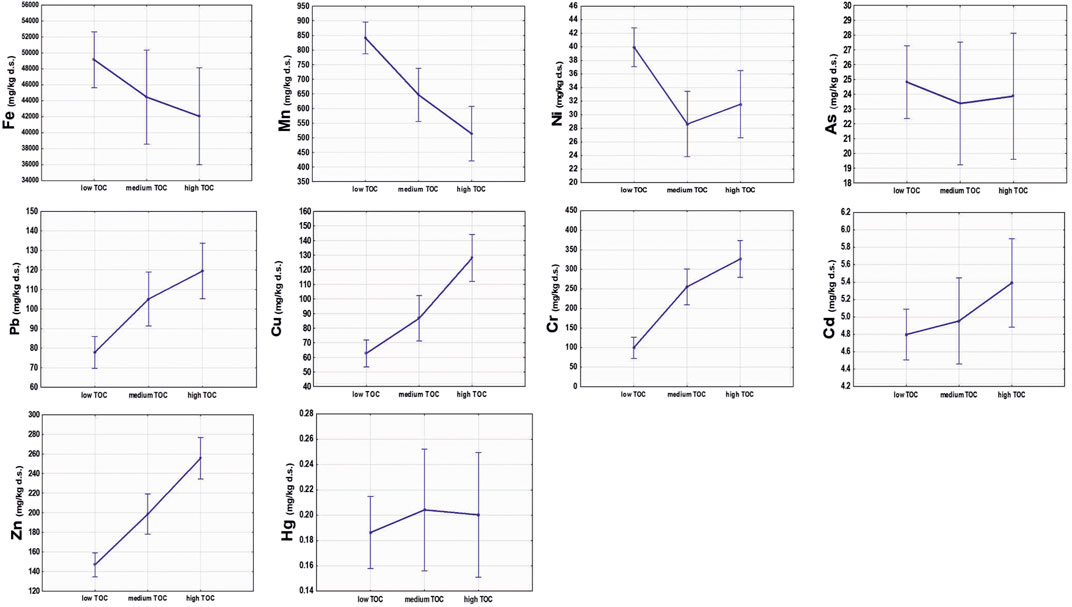
FIGURE 11. One-way ANOVA representing the variability of the concentrations of Pb, Cr, Cu, Zn, Cd, Fe, Mn, Ni, As, and Hg within the different defined TOC content classes. The vertical bars denote 0.95 confidence intervals. Wilks lambda=.26442, F (20, 158) =7.4632, p=.00000.
4 Discussion
4.1 Natural vs. anthropogenic source
The area is characterized by a heterogeneous lithological substrate, which includes sediments derived from volcanic and sedimentary deposits, and by the high presence of industries in the Sarno plain. Taking this into account, we can try to evaluate whether it is possible to discriminate between areas mainly influenced by natural or anthropogenic sources. As the Sarno River can transport contaminants in its catchment area to the sea, the results of this study were compared with the published data collected on land, to investigate the nature of the contaminants.
Among others, the study by Albanese et al. (2013), conducted onshore in the Sarno Plain, identified metal associations that have been linked to natural or anthropogenic origins. Of the latter, tannery or cannery industries, agriculture, or traffic and urban mobility have been considered. The authors have recognized four metal associations: F1 (As, La, Al, Mn, U, and Fe) associated with pyroclastic volcanic soils; F2 (Sr, K, P, V, Cu, Co, and U), typical of agricultural areas; F3 (Zn, Pb, Cd, Sb, and Hg), due to human activities; and F4 (Ni, Cr, and Fe), associated with the tannery district (Albanese et al., 2013). According to the authors, the metals related to the presence of pyroclastics and volcanic soils mostly covering hilly and mountainous areas surrounding the Sarno River plain, included As, Fe, and Mn. Relatively high values of As characterized sediments collected onshore at the slopes of Mount Somma-Vesuvio. Generally, the distribution of As in Campania stream sediments has been mostly related to their volcanoclastic nature (Aiuppa et al., 2003; Albanese et al., 2007). In addition, it cannot be excluded that the application in agriculture of inorganic arsenical pesticides and herbicides might contribute to increase the As level in soils and sediments (Carbonell-Barrachina et al., 2009; Fitzmaurice et al., 2009).
Sector A, offshore Torre Annunziata, where the substrate corresponds to the submarine counterpart of the Vesuvius volcano (Milia et al., 2012), is characterized by As, Fe, and Mn, and mainly by sand, as confirmed by PCA analysis. Additionally, these contaminants have been detected in samples from sector C, where in adjacent outcrops volcanic tephra covers the carbonatic relief. The geologic frame leads us to suppose that the concentration of these metals might be associated mainly with the geogenic sources as documented by the pumice clasts found in the gravels sampled in sector C. This evidence documents that the concentration of As, Fe, and Mn can be associated with geogenic sources, although a slight influence of anthropogenic sources cannot be ruled out.
Sector B mostly contains samples with high concentrations of toxic metals, including Cu, Zn, Pb, Cd, Hg, and Cr, associated with an increase in the silty and clay fraction, and a high TOC content. The combination of these features led us to suppose that contaminants of this cluster are controlled by organic matter, probably due to exchange absorption, complexation, or chelation phenomena that increase, hence their hazard (Meyers, 1994; Baran et al., 2019). In addition, a coupling phenomenon of grain size and TOC might influence the enrichment of metals in this area. Because of the high specific surface area and humic substance content, metals are prone to being bound in the fine-grained fractions (<63 μm) of the sediments (Horowitz and Elrick, 1987; Moore et al., 1989; Mali et al., 2015). Analysis of the trend of these metals in the onshore area and of their origin was performed by different authors. According to Giuffré de Lopez Camelo et al. (1997), phosphate fertilizers can be considered a potential source of Cu produced from rock phosphates. Albanese et al. (2013) attributes the correlation of Cu with TOC to agricultural activities, likely in terms of NPK (nitrogen, phosphorous, and potassium) fertilizer use. Additionally, this is consistent with observations made by Adamo et al. (2006) and D’Ascoli et al. (2006) in soils from the inner part of the Sarno plain, where Cu contamination seems to depend on past widespread agricultural practices involving Cu-rich material utilization. As for Zn, Pb, Cd, and Hg in the onshore area, these metals correlated with different sources. Sanchez-Martin et al. (2000) suggested that traffic and urban mobility probably play the main role in the Zn and Cd accumulation process. Onshore, the highest values of Zn content in sediments has been measured in the highly urbanized and crowded parts of the Sarno plain between Torre Annunziata and Castellammare di Stabia (along the coastline), as well as close to the Lattari Mountains. Additionally, relatively high concentrations of Cd in sediments have been found in the coastal area, close to Castellammare di Stabia, and in correspondence to the hilly areas overlooking the urban settlements.
Furthermore, high Hg values, which could stem from the atmospheric fallout of exhaust gases of petrol, diesel, and LPG vehicles, have been revealed in the Sarno River sediments and in correspondence to the highly urbanized areas of the Sarno plain Albanese et al. (2013) found that the concentration levels of Hg in the Sarno sediment were much higher than those found in the Gediz River (Eastern Aegean; Kucuksezgin et al., 2008), Homa Lagoon (Turkey; Uluturhan et al., 2011), and offshore Bagnoli in the Gulf of Naples (Italy; Romano et al., 2004), but lower than those reported in Naples harbor (Sprovieri et al., 2007) and the Venetian Lagoon (Zonta et al., 2007). Therefore, we suppose that, similarly with the literature, an Hg contribution should be sought in the marine sources, probably due to marine fuel combustion and losses.
Following the authors analysis, Pb was present in the stream sediments from the Sarno plain, registering the highest concentrations in the sediments that lie between urban areas and in the coastal belt between Castellammare di Stabia and Torre Annunziata. In the urban environment, motor vehicle emissions were major source of Pb in sediments. By contrast, in the remaining areas, including hilly and mountainous areas north of Sarno covered by Vesuvius tephra, which are characterized by Pb concentrations in a similar range to those of the Campanian background for volcanoclastic deposits, there were low levels of contamination. For this reason, Albanese et al. (2013) attribute the element accumulation in the environment to the traffic load and human mobility, with special emphasis on the tire consumption process of motor vehicles.
With the reference to Cr, considering the findings of Albanese et al. (2013), the concentrations of this element can be related to the activity of numerous tannery plants, which caused, in the past, a Cr enrichment of the river waters (Basile et al., 1985). Nevertheless, strong enrichment of Cr in some onshore sediments collected at the base of the slopes of the Sarno and Lattari Mountains has been found to be related to the presence of some limestone quarries in the area (Albanese et al., 2013). The local rise of pH might have promoted Cr immobilization as this metal, already characterized by general low mobility, is even more immobile in an alkaline environment.
Although the Sarno plain can be distinguished into areas characterized by different types of metal contamination originating from agricultural practices, traffic load and human mobility, or tannery industries, the marine area contains all these contaminants together in the same cluster of sector B. Zn, Pb, Cd, and Cu were highly correlated with “high TOC content” and “silt”. Consequently, the distribution of these contaminants was influenced by particle-size and organic matter, probably due to the organic matter degradation process affecting pH and Eh values (Solomon and Forstner, 1984; Mali et al., 2015; Mali et al. 2016; Mali et al. 2017a; Mali et al. 2017b; Mali et al. 2018; Mali et al. 2019). Indeed, as can be observed with the correlation matrix (Table 1), the relationship of TOC values with the main contaminants shows significantly positive correlation coefficients (p < 0.05): TOC vs. Pb and TOC vs. Cu, both R2 > 0.50, and to a lesser extent for TOC vs. Cr. We suppose that the good correlation determined between Cr vs. Ni and Cr vs. Fe can be strictly correlated with the tannery district located in the Sarno River plain (as suggested by Albanese et al., 2013). Definitively, it can be asserted that the contamination sources of sector B are of an anthropogenic nature.
4.2 Source to sink
A reconstruction of the sediment path from the coast to the depositional area was made taking into account the following considerations: i) the peculiarity of the clusters recognized in the marine area highlighting the relationships between metals, organic matter content, and grain size; ii) the geology of the area; iii) the origin of the contaminants through comparison with those recognized in the onshore area; and iv) the origin of the organic matter (OM) (Table 3).
The marine area may receive considerable amounts of contaminants from land sources through coastal or river discharge. In addition, metals, owing to high persistence, can accumulate and remain in sediments for relatively long periods of time. Consequently, the marine areas are the natural sinks and environmental reservoirs for metals, and the assessment of metals in the submarine environment provides a great opportunity for reconstructing the pathway of contaminants.
On the other hand, the organic material in coastal sediments might originate from marine phytoplankton growing on the sediment surface, as well as from organic material of terrestrial origin transported to the sea from elsewhere, such as with the tide or by a river. Owing to the interplay between the different sources of organic matter in sediments, the total organic carbon to total nitrogen ratio (TOC/TN) is often used to determine the different signatures of organic matter sources (Lamb et al., 2006; Gao et al., 2012). Generally, the C/N ratio for organic matter of the sea is approximately 7, ranges from 8 to 20 for soil organic matter, and is in excess of 25 for organic matter from terrigenous sediments (Emerson and Hedges, 1988; Meyers, 1994; Lamb et al., 2006).
In the investigated area, the C/N ratio varies within large ranges. Additionally, a distinct trend was revealed within the four sectors, with high C/N values in sectors A and C: from 19 to 528 in sector A and from 502 to 1,550 in sector C. These values suggest that the organic matter comes from terrigenous sediments characterized predominantly by terrestrial plants. In sector B, the C/N ratio is low, ranging from 12 to 20, suggesting a mixed origin (terrestrial and marine) of the organic content. Finally, in sector D, the area approaching the deepest water depth, the C/N values decrease to low values ranging between 3 and 11, suggesting a marine origin. These latter values are probably generated by phytoplankton, which according to Meyers (1994) and Tyson (1995) tends to be nitrogen-rich and consequently has low C/N ratios.
It must be taken into account that the mixing of organic matter from different sources may result in C/N values that fall out of the fields established for terrestrial land plants and phytoplankton, and this is a situation particularly expected in coastal settings (Kendall et al., 2001; Lamb et al., 2006), casting doubt on the utility of the C/N value as bioindicator of organic matter provenance. Two factors might influence this ratio: i) the anthropogenic input of organic matter and nutrients, originating from agricultural runoff, fertilization, and industrial domestic waste discharge, which can disturb the natural balance between the production and decomposition of organic matter and then alter the natural C/N ratio, and ii), sediment grain size, which is another key factor influencing organic matter and nutrient accumulation in surface sediments.
The analysis of sediment samples reveals a positive correlation between TN and TOC that could be attributed to natural or anthropogenic factors. According to Avramidis et al. (2015), the relationship between TOC and TN could be the result of the high concentration of both nutrients in the make-up of animals and plants that, coming from the surrounding terrigenous sources, decompose in the waterbody. Moreover, the anthropogenic organic pollutants coming from the runoff waters and through the Sarno River in the sea basin could also affect this correlation.
In our case, the sectors A and C, where the highest C/N ratios have been registered, are characterized by low TN and TP concentrations in almost all samples. In some cases, the measured values were under the detection limit of these two variables. In this track, TOC values were also very low, even if not to the same extent as TN and TP. The finest granulometry (silt and clay), with a high active surface area, enhance the absorption of nutrients in marine sediment; by contrast, coarser grains (gravel and sand) experience nutrient dispersion and organic matter and nutrient depletion because of their size (El-Moselhy and Abd El-Azim, 2005; Rodrìguez-Barroso et al., 2010). With regard to sector A, it is possible to suppose that the predominance of the sand fraction exerts major control on the nutrients; indeed, it is predominant with respect to the TOC content, thus impacting the C/N values. The prevalence of the sand grain size is in accordance with the geologic features characterized by the slope of the Vesuvius volcano with an alluvial fan, made up of volcanoclastic deposits, that reaches the coast and supplies coarse grained sediments to the submarine area. The prevalence of the geogenic origin of the contaminants, the terrestrial origin of the organic matter, and the geologic features witness that sector A represents the sink area of the sediment arriving from the volcano (Figure 12). By contrast, sectors B, C, and D are characterized by a high percentage of sediments of <63 μm with clay that increases approaching the distal area, albeit with a different content of organic matter and contaminants that is only of high concern in sector B. The circumstance suggests that sediment grain size is not the only parameter that controls the presence of contaminants and organic matter. In particular, only sector B contains almost all toxic metals, including Cu, Zn, Pb, Cd, Hg, and Cr. This can be explained by considering the provenance of the contaminants. In fact, these contaminants appear to be of anthropogenic origin and in particular from the Sarno plain, as described in the previous section. The hypothesis is in compliance with the findings of Montuori et al. (2013) who analyzed the contamination by eight metals (As, Hg, Cd, Cr, Cu, Ni, Pb, and Zn) in the water dissolved phase, suspended particulate matter, and sediment sample collected close to the river source, in the middle part and close to mouth of the Sarno River, and in the proximal shore 50 m and 150 m from the coast. The authors reconstructed the pathway of these metals along the river until the river delta front. As documented by Montuori et al. (2013), the grain sizes of sediments discharged from the Sarno River are very fine, suggesting that the very high percentage of silt sediment in sector B, associated with the highest TOC content for the study area, is strictly related to the sediment coming straight from the river and representing the depositional environment of the Sarno River prodelta. According to Horowitz and Elrick (1987) and Moore et al. (1989), because of the high specific surface area and humic substance content, metals are prone to being bound in the fine-grained fractions (<63 μm) of the sediments. In view of this, the presence of all the anthropogenically derived metals in sector B, together with the peculiarly high percentage of silt, strengthens the interpretation that this sector represents the sink of the sediments discharged in the sea from the Sarno River (Figure 11).
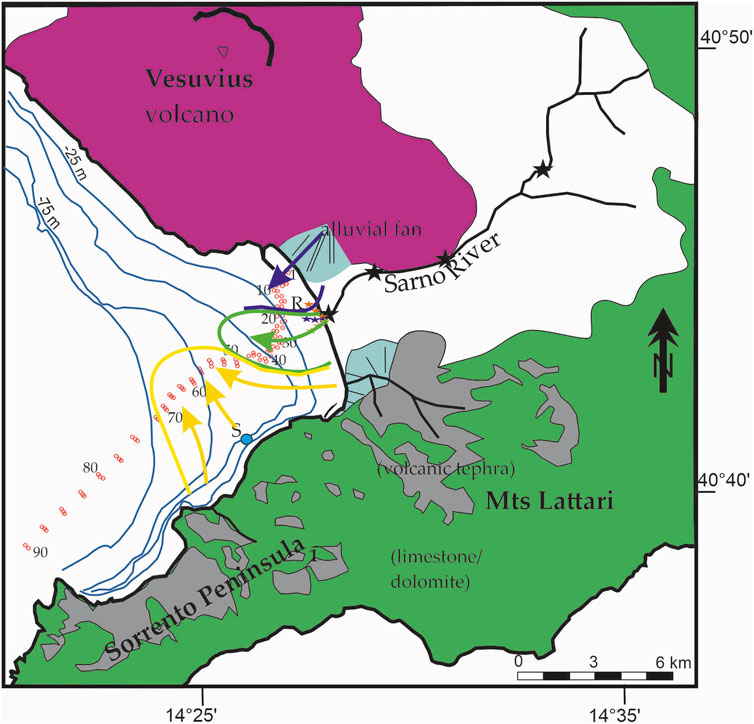
FIGURE 12. Individuation of distinct marine sectors with different geochemical associations and reconstruction of the source-to-sink pathway of contaminants on the continental shelf.
Sector C is positively associated with As, Mn, and Fe. These metals of mainly volcanic origin can be associated with the erosion of the volcanic tephra that covers Mounts Lattari (Figure 12) and were transported toward the sea by the minor drainage system that incise the carbonatic relief, as witnessed by the pumice elements in the gravel fraction. Indeed, the tephra layers are highly erodible loose material and more prone to be transported with respect to the carbonatic rock. This agrees with the terrestrial origin of the organic matter in this sector. The presence of bioclasts, such as coral fragments, suggests a source area from reefs that is present offshore the Sorrento Peninsula (e.g., Banco di Santa Croce). These elements allow us to interpret that sector C benefits from sediments coming from the emerged and submerged area of the Sorrento Peninsula (Figure 12). Finally, the slight metal contamination in sector D might be attributed to marine traffic affecting the investigated track.
This reconstruction suggests that the contamination originating from the Sarno Plain is dispersed toward the west in the Bay of Naples and decreases rapidly, moving toward the distal area. Additionally, the analysis allowed the limitation of the area of influence of the river supply in the prodelta marine environment. A similar finding has been observed offshore the Yangtze River, where the authors considered the dominant contributor to the inner-shelf mud area deriving from finer suspended sediments and transported southward by the Zhejiang-Fujian Coastal Current (Guo et al., 2004). The grain sizes of the sediments discharged from the Sarno River are very fine and influence metal binding to the fine-grained fractions (<63 μm) of the sediments. We can thus conclude that coupling phenomena, natural (TOC, grain size, and hydrodynamic river) and anthropogenic (contaminants from tannery industry and massive agriculture practices), are responsible for the contamination pattern in sector B.
According to Yao et al. (2015), statistical analysis of the correlation analyses between metals and Fe, as well as Mn, in different fractions of the samples in the Yellow River further support the potential sources and behavior of the metals. The authors suggest that the strong association between Fe, Mn, and metals can be explained by the particle size and Fe/Mn oxides in controlling metal concentrations. The loadings of metals exhibited a substantial accumulation in particle size fractions of <63 μm. By contrast, in the study area, Fe and Mn are associated with group 1 (sector A), where sand is predominant, while the metals Cu, Pb, Zn, Cr, and Cd characterize sector B, where fractions of <63 μm are prevalent, reinforcing the hypothesis that the sediments of the two groups come from different source areas.
Sectors C and D are not influenced by anthropogenic contamination; therefore, natural factors, such as the influence of sediment discharges from the Lattari Mountains-Sorrento Peninsula, should be considered. The slight metal contamination in the last two sectors might be attributed to marine traffic affecting the investigated track (Daniela Malcangio et al., 2017).
In conclusion, the investigation of the geochemical and physical parameters, performed with the support of PCA, HCA, and ANOVA, allowed us distinguish four distinct sectors with different contamination patterns. The comparison of the metal associations recognized offshore through published onshore data also allow us to individuate the origin of the contaminates in each area, which are mainly geogenic in the continental shelf except for sector B, where almost all the toxic metals are present and associated with anthropogenic contamination sources (agricultural, human activities, and tannery industries). The distribution of the contaminants is strictly controlled by grain size, TOC content, and the geologic environment of the area that highlights the pathway of the sediments from source to sink. Sector A is influenced by the sand eroded from the Vesuvius slope and deposited close to the Torre Annunziata coast, whereas sector B reflects the contaminant arriving from the Sarno plain via Sarno river flow. The latter are associated with the peculiar feature of high TOC content, suggesting that the discharges of sediments from the Sarno River are the main driving force of the accumulation of organic matter in the marine basin. The samples from sector C were slightly similar to sector A but in a different geologic context; sector C collects extrabasinal sediments from the tephra layers covering the Sorrento Peninsula, which have been eroded and transported by a minor river to the sea and intrabasinal marine bioclasts. Finally, sector D, which lies below a water depth of 110 m, is characterized by lower contamination, suggesting that the metals from the Sarno plain are mainly deposited close to the source area in the Sarno river prodelta and do not affect the open marine environment.
Data availability statement
Publicly available datasets were analyzed in this study. This data can be found here: https://doi.org/10.1007/s11356-022-19989-z.
Author contributions
AM, substantial contributions to the conception, drafting the work; acquisition, interpretation of data for the work. FB, contributions to the conception or design of the work, provide approval for publication of the content; the acquisition, analysis, interpretation of data for the work. MM, statistical analysis of the data, contribution to the draft of the work; SG, geochemical analysis and interpretation, contribution to the draft of the work; AL, geochemical analysis; LS, geochemical analysis, LF, data acquisition, revision; LG, data acquisition. All authors contributed to the article and approved the submitted version.
Conflict of interest
The authors declare that the research was conducted in the absence of any commercial or financial relationships that could be construed as a potential conflict of interest.
Publisher’s note
All claims expressed in this article are solely those of the authors and do not necessarily represent those of their affiliated organizations, or those of the publisher, the editors and the reviewers. Any product that may be evaluated in this article, or claim that may be made by its manufacturer, is not guaranteed or endorsed by the publisher.
Supplementary material
The Supplementary Material for this article can be found online at: https://www.frontiersin.org/articles/10.3389/feart.2023.1225658/full#supplementary-material
References
Adamo, P., Colombo, C., Terribile, F., and De Santo, A. “Degradation processes in volcanic soils joint meeting WG3/4 cost action 622,” in Proceedings of the Degradation Processes in Volcanic Soils, Napoli, May 1999, 24–47.
Adamo, P., Denaix, L., Terribile, F., and Zampella, M. (2003). Characterization of heavy metals in contaminated volcanic soils of the Solofrana river valley (southern Italy). Geoderma 117, 347–366. doi:10.1016/s0016-7061(03)00133-2
Adamo, P., Zampella, M., Gianfreda, L., Renella, G., Rutigliano, F. A., and Terribile, F. (2006). Impact of river overflowing on trace element contamination of volcanic soils in south Italy: part i. trace element speciation in relation to soil properties. Environ. Pollut. 144, 308–316. doi:10.1016/j.envpol.2006.03.006
Aiuppa, A., D’Alessandro, W., Federico, C., Palumbo, B., and Valenza, M. (2003). The aquatic geochemistry of arsenic in volcanic groundwaters from southern Italy. Appl. Geochem. 18, 1283–1296. doi:10.1016/s0883-2927(03)00051-9
Albanese, S., Iavazzo, P., Adamo, P., Lima, A., and De Vivo, B. (2013). Assessment of the environmental conditions of the Sarno River basin (south Italy): A stream sediment approach. Environ. Geochem. Health 35, 283–297. doi:10.1007/s10653-012-9483-x
Albanese, S., De Vivo, B., Lima, A., and Cicchella, D. (2007). Geochemical background and baseline values of toxic elements in stream sediments of Campania region (Italy). J. Geochem. Explor. 93, 21–34. doi:10.1016/j.gexplo.2006.07.006
Amorosi, A. (2012). Chromium and nickel as indicators of source-to-sink sediment transfer in a Holocene alluvial and coastal system (Po Plain, Italy). Sed. Geol. 280, 260–269. doi:10.1016/j.sedgeo.2012.04.011
Arienzo, M., Adamo, P., Bianco, M. R., and Violante, P. (2001). Impact of land use and urban runoff on the contamination of the Sarno river basin in southwestern Italy. Water Air Soil. Pollut. 131, 349–366. doi:10.1023/a:1011908019933
Avramidis, P., Geraga, M., Lazarova, M., and Kontopoulos, N. (2013). Holocene record of environmental changes and palaeoclimatic implications in alykes lagoon, zakynthos island, western Greece, mediterranean sea. Quatern. Int. 293, 184–195. doi:10.1016/j.quaint.2012.04.026
Avramidis, P., Nikolaoua, K., and Bekiari, V. (2015). Total organic carbon and total nitrogen in sediments and soils: A comparison of the wet oxidation – titration method with the combustion-infrared method. Agric. Agric. Sci. Procedia 4, 425–430. doi:10.1016/j.aaspro.2015.03.048
Baran, A., Mierzwa-Hersztek, M., Gondek, K., Tarnawski, M., Szara, M., Gorczyca, O., et al. (2019). The influence of the quantity and quality of sediment organic matter on the potential mobility and toxicity of trace elements in bottom sediment. Environ. Geochem. Health 41, 2893–2910., doi:10.1007/s10653-019-00359-7
Basile, G., Palmieri, F., and Violante, P. (1985). Il fiume Sarno: valutazione delle variazioni dell’inquinamento. Atti del Convegno Naz. Inquin. idrico Conserv. dell’Ecosistema, 258–293.
Carbonell-Barrachina, A. A., Signes-Pastor, A. J., Vázquez-Araújo, L., Burló, F., and Sengupta, B. (2009). Presence of arsenic in agricultural products from arsenic-endemic areas and strategies to reduce arsenic intake in rural villages. Mol. Nut. Food Res. 53, 531–541. doi:10.1002/mnfr.200900038
Chapman, P. M., Wang, F., Janssen, C., Persoone, G., and Allen, H. E. (1998). Ecotoxicology of metals in aquatic sediments: binding and release, bioavailability, risk assessment, and remediation. Can. J. Fish. Aquat. Sci. 55, 2221–2243. doi:10.1139/f98-145
Cioni, R., Santacroce, R., and Sbrana, A. (1999). Pyroclastic deposits as a guide for reconstructing the multi-stage evolution of the Somma-Vesuvius Caldera. Bull. Volcanol. 61, 207–222. doi:10.1007/s004450050272
Cobelo-García, A., and Prego, R. (2003). Heavy metal sedimentary record in a Galician ria (NW Spain): background values and recent contamination. Mar. Pollut. Bull. 46, 1253–1262. doi:10.1016/s0025-326x(03)00168-1
Cotecchia, F., Vitone, C., Sollecito, F., Mali, M., Miccoli, D., Petti, R., et al. (2021). A geo-chemomechanical study of a highly polluted marine system (Taranto, Italy) for the enhancement of the conceptual site model. Sci. Rep. 11, 4017. doi:10.1038/s41598-021-82879-w
Daniela Malcangio, D., Melena, A., Damiani, L., Mali, M., and Saponieri, A. (2017). Numerical study of water quality improvement in a port through a forced mixing system. WIT Trans. Ecol. Environ. 220, 69–80.
D’Ascoli, R., Rao, M. A., Adamo, P., Renella, G., Landi, L., Rutigliano, F. A., et al. (2006). Impact of river overflowing on trace element contamination of volcanic soils in south Italy: part ii. soil biological and biochemical properties in relation to trace element speciation. Environ. Pollut. 144, 317–326. doi:10.1016/j.envpol.2005.11.017
De Pippo, T., Donadio, C., Guida, M., and Petrosino, C. (2006). The case of Sarno River (southern Italy). Effects of geomorphology on the environmental impacts (8 pp). Environ. Sci. Pollut. Res. 13 (3), 184–191. doi:10.1065/espr2005.08.287
Dean, W. E. (1974). Determination of carbonate and organic matter in calcareous sediments and sedimentary rocks by loss on ignition: comparison with other methods. J. Sediment. Petrol. 44, 242–248.
Dean, W. E. (1999). The carbon cycle and biogeochemical dynamics in lake sediments and sedimentary rocks by loss on ignition: comparison with other methods. J. Paleolimn. 21, 375–393. doi:10.1023/a:1008066118210
El-Moselhy, Kh. M., and Abd El-Azim, H. (2005). Heavy metals content and grain size of sediments from Suez Bay, Red Sea, Egypt. Egypt. J. Aquatic Res. 31 (2), 224–238.
Emerson, S., and Hedges, J. I. (1988). Processes controlling the organic carbon content of open ocean sediments. Paleoceanography 3, 621–634. doi:10.1029/PA003i005p00621
Fitzmaurice, A. G., Bilgin, A. A., O’Day, P. A., Illera, V., Burris, D. R., Reisinger, H. J., et al. (2009). Geochemical and hydrologic controls on the mobilization of arsenic derived from herbicide application. App. Geochem. 24, 2152–2162. doi:10.1016/j.apgeochem.2009.09.019
Gao, L., Li, D., and Zhang, Y. (2012). Nutrients and particulate organic matter discharged by the Changjiang (Yangtze River): seasonal variations and temporal trends. J. Geophys. Res. 117, G04001. doi:10.1029/2012JG001952
Giandomenico, S., Cardellicchio, N., Spada, L., Annicchiarico, C., and Di Leo, A. (2016). Metals and PCB levels in some edible marine organisms from the ionian sea: dietary intake evaluation and risk for consumers. Environ. Sci. Pollut. Res. 23 (13), 12596–12612. doi:10.1007/s11356-015-5280-2
Giuffré de López Camelo, L., Rato de Miguez, S., and Marbán, L. (1997). Heavy metals input with phosphate fertilizers used in Argentina. Sci. Tot. Environ. 204, 245–250. doi:10.1016/s0048-9697(97)00187-3
Guo, L., Zhang, J.-Z., and Guéguen, C. (2004). Speciation and fluxes of nutrients (N, P, Si) from the upper Yukon River. Glob. Biogeochem. Cycles 18, GB1038. doi:10.1029/2003GB002152
Hill, N. A., Simpson, S. L., and Johnston, E. L. (2013). Beyond the bed: effects of metal contamination on recruitment to bedded sediments and overlying substrata. Environ. Pollut. 173, 182–191. doi:10.1016/j.envpol.2012.09.029
Horowitz, A. J., and Elrick, K. A. (1987). The relation of stream sediment surface area, grain size and composition to trace element chemistry. Appl. Geochem. 2, 437–451. doi:10.1016/0883-2927(87)90027-8
Hu, G., Bi, S. P., Xu, G., Zhang, Y., Mei, X., and Li, A. C. (2015). Distribution and assessment of heavy metals off the Changjiang River mouth and adjacent area during the past century and the relationship of the heavy metals with anthropogenic activity. Mar. Pollut. Bull. 96, 434–440. doi:10.1016/j.marpolbul.2015.05.009
Kendall, C., Silva, S. R., and Kelly, V. J. (2001). Carbon and nitrogen isotopic compositions of particulate organic matter in four large river systems across the United States. Hydrol. Process. 15, 1301–1346. doi:10.1002/hyp.216
Kucuksezgin, F., Uluturhan, E., and Batki, H. (2008). Distribution of heavy metals in water, particulate matterand sediments of Gediz River (Eastern Aegean). Environ. Monit. Assess. 141, 213–225. doi:10.1007/s10661-007-9889-6
Lamb, A. L., Wilson, G. P., and Leng, M. J. (2006). A review of coastal palaeoclimate and relative sea-level reconstructions using δ13C and C/N ratios in organic material. Earth Sci. Rev. 75, 29–57. doi:10.1016/j.earscirev.2005.10.003
Lu, J., Li, A., and Huang, P. (2017). Distribution, sources and contamination assessment of heavy metals in surface sediments of the South Yellow Sea and northern part of the East China Sea. Mar. Pollut. Bull. 24 (1), 470–479. doi:10.1016/j.marpolbul.2017.07.007
Lu, X., Zhou, F., Chen, F., Lao, Q., Zhu, Q., Meng, Y., et al. (2020). Spatial and seasonal variations of sedimentary organic matter in a subtropical Bay: implication for human interventions. Int. J. Environ. Res. Public Health 17, 1362. doi:10.3390/ijerph17041362
Mali, M., Dell’Anna, M. M., Damiani, L., Mastrorilli, P., Cardellicchio, A., Ungaro, N., et al. (2019). Long-term monitoring programs to assess environmental pressures on coastal area: weighted indexes and statistical elaboration as handy tools for decision-makers. Ecol. Indic. 101, 838–850. doi:10.1016/j.ecolind.2019.01.085
Mali, M., Dell’Anna, M. M., De Serio, F., Mastrorilli, P., Damiani, L., and Mossa, M. (2017b). Enhancing the performance of hazard indexes in assessing hot spots of harbour areas by considering hydrodynamic parameters. Ecol. Indic. 73, 38–45. doi:10.1016/j.ecolind.2016.09.028
Mali, M., Dell’Anna, M. M., Mastrorilli, P., Damiani, L., Ungaro, N., Belviso, C., et al. (2015). Are conventional statistical techniques exhaustive for defining metal background concentrations in harbour sediments? A case study: the coastal area of bari (southeast Italy). Chemosphere 138, 708–717. doi:10.1016/j.chemosphere.2015.07.046
Mali, M., Dell’Anna, M. M., Notarnicola, M., Damiani, L., and Mastrorilli, P. (2017a). Combining chemometric tools for assessing hazard sources and factors acting simultaneously in contaminated areas. Case study: “Mar piccolo” taranto (south Italy). Chemosphere 184, 784–794. doi:10.1016/j.chemosphere.2017.06.028
Mali, M., Dell’Anna, M. M., Ungaro, N., Gredilla, A., Fortez, O., De Vallejuelo, S., et al. (2016). Identification of hot spots within harbour sediments through a new cumulative hazard index. Case study: port of bari, italy. Italy. Ecol. Indic. 60, 548–556. doi:10.1016/j.ecolind.2015.07.024
Mali, M., Di Leo, A., Giandomenico, S., Spada, L., Cardellicchio, N., Calò, M., et al. (2022). Multivariate tools to investigate the spatial contaminant distribution in a highly anthropized area (Gulf of Naples, Italy). Environ. Sci. Poll. Res. 29, 62281–62298. doi:10.1007/s11356-022-19989-z
Mali, M., Malcangio, D., Dell’Anna, M. M., Damiani, L., and Mastrorilli, P. (2018). Influence of hydrodynamic features in the transport and fate of hazard contaminants within touristic ports Case study: torre a mare (italy). Heliyon 4 (1), e00494. doi:10.1016/j.heliyon.2017.e00494
Meybeck, M., and Helmer, R. (1989). The quality of rivers: from pristine stage to global pollution. Glob. Planet. Chang. 1, 283–309. doi:10.1016/0031-0182(89)90191-0
Meyers, P. A. (1994). Preservation of elemental and isotopic source identification of sedimentary organic matter. Chem. Geol. 114 (3-4), 289–302. doi:10.1016/0009-2541(94)90059-0
Milia, A., Torrente, M. M., and Bellucci, F. (2012). A possible link between faulting, cryptodomes and lateral collapses at Vesuvius Volcano (Italy). Glob. Planet. Change 90-91, 121–134. doi:10.1016/j.gloplacha.2011.09.011
Milia, A., Torrente, M. M., and Zuppetta, A. (2003). Offshore debris avalanches at somma-vesuvius volcano (Italy): implications for hazard evaluation. J. Geol. Soc. 160, 309–317. doi:10.1144/0016-764902-045
Montuori, P., Lama, P., Aurino, S., Naviglio, D., and Triassi, M. (2013). Metals loads into the mediterranean sea: estimate of sarno river inputs and ecological risk. Ecotoxicology 22, 295–307. doi:10.1007/s10646-012-1026-9
Moore, J. N., Brook, E. J., and Johns, C. (1989). Grain size partitioning of metals in contaminated, coarse-grained river floodplain sediment: clark fork river, montana, U.S.A. U.S.A. Environ. Geol. Water Sci. 14, 107–115. doi:10.1007/bf01728502
Nazneen, S., and Raju, N. J. (2017). Distribution and sources of carbon, nitrogen, phosphorus and biogenic silica in the sediments of Chilika lagoon. J. Earth Syst. Sci. 126, 13. doi:10.1007/s12040-016-0785-8
Oliveri, E., Ausili, A., Barsanti, M., Conte, F., Delbono, I., Del Core, M., et al. (2022). Interferences between natural and anthropic hazards in marine-coastal environments: assessing transport from land to the offshore systems in the crotone basin (ionian sea). Estuar. Coast. Shelf Sci. 271, 107854. doi:10.1016/j.ecss.2022.107854
Prego, R., and Cobelo-García, A. (2003). Twentieth century overview of heavy metals in the Galician Rias (NW Iberian Peninsula). Environ. Pollut. 121, 425–452. doi:10.1016/s0269-7491(02)00231-2
Rainbow, P. S., and Luoma, S. N. (2011). Metal toxicity, uptake and bioaccumulation in aquatic invertebrates-modelling zinc in crustaceans. Aquat. Toxicol. 105, 455–465. doi:10.1016/j.aquatox.2011.08.001
Roberts, D. A. (2012). Causes and ecological effects of resuspended contaminated sediments (RCS) in marine environments. Environ. Int. 40, 230–243. doi:10.1016/j.envint.2011.11.013
Rodríguez-Barroso, M. R., Benhamou, Y., El Moumni, B., Coello, D., and García-Morales, J. L. (2010). Concentration, enrichment and distribution of heavy metals in surface sediments of the Tangier Bay, Morocco. Sci. Mar. 74, 107–114. doi:10.3989/scimar.2010.74s1107
Romano, E., Ausili, A., Zharova, N., Magno, M. C., Pavoni, B., and Gabellini, M. (2004). Marine sediment contamination of an industrial site at Port of Bagnoli, Gulf of Naples, Southern Italy. Mar. Pollut. Bull. 49 (5-6), 487–495. doi:10.1016/j.marpolbul.2004.03.014
Sánchez-Martín, M. J., Sánchez-Camazano, M., and Lorenzo, L. F. (2000). Cadmium and Lead Contents in Suburban and Urban Soils from Two Medium-Sized Cities of Spain: influence of traffic intensity. B. Environ. Contam. Tox. 64 (2), 250–257. doi:10.1007/s001289910037
Santacroce, R., Cioni, R., Marianelli, P., Sbrana, A., Sulpizio, R., Zanchetta, G., et al. (2008). Age and whole rock–glass compositions of proximal pyroclastics from the major explosive eruptions of Somma-Vesuvius: A review as a tool for distal tephrostratigraphy. J. Volcanol. Geoth. Res. 177 (1), 1–18. doi:10.1016/j.jvolgeores.2008.06.009
Solomons, W., and Förstner, U. (1984). Metals in the hydrocycle. Berlin, Germany: Springer Verlag, 349. doi:10.1007/978-3-642-69325-0
Spada, L., Annicchiarico, C., Cardellicchio, N., Giandomenico, S., and Di Leo, A. (2012). Mercury and methylmercury concentrations in Mediterranean seafood and surface sediments, intake evaluation and risk for consumers. Inter. J. Hyg. Environ. Health 215, 418–426. doi:10.1016/j.ijheh.2011.09.003
Sprovieri, M., Passaro, S., Ausili, A., Bergamin, L., Finoia, M. G., Gherardi, S., et al. (2020). Integrated approach of multiple environmental datasets for the assessment of sediment contamination in marine areas affected by long-lasting industrial activity: the case study of Bagnoli (southern Italy). J. Soils Sediments 20, 1692–1705. doi:10.1007/s11368-019-02530-0
Sprovieri, M., Feo, M. L., Prevedello, L., Manta, D. S., Sammartino, S., Tamburrino, S., et al. (2007). Heavy metals, polycyclic aromatic hydrocarbons and polychlorinated biphenyls in surface sediments of the Naples harbour (southern Italy). Chemosphere 67 (463), 998–1009. doi:10.1016/j.chemosphere.2006.10.055
Sundelin, B., and Eriksson, A. K. (2001). Mobility and bioavailability of trace metals in sulfidic coastal sediments. Chemosphere 20, 748–756. doi:10.1002/etc.5620200408
Tamburrino, S., Passaro, S., Barsanti, M., Schirone, A., Delbono, I., Conte, F., et al. (2019). Pathways of inorganic and organic contaminants from land to deep sea: the case study of the gulf of cagliari (w tyrrhenian sea). Sc. Tot. Environ. 647, 334–341. doi:10.1016/j.scitotenv.2018.07.467
Tyson, R. V. (1995). Sedimentary organic matter: Organic facies and palynofacies. London, England: Chapman & Hall, 615. doi:10.1007/978-94-011-0739-6
Uluturhan, E., Kontas, A., and Can, E. (2011). Sediment concentrations of heavy metals in the Homa Lagoon (Eastern Aegean Sea): assessment of contamination and ecological risks. Mar. Pollut. Bull. 62 (9), 1989–1997. doi:10.1016/j.marpolbul.2011.06.019
United States Environmental Protection Agency, (2014). “Inductively Coupled Plasma-Mass Spectrometry,”(Washington, D.C., United States: United States Environmental Protection Agency).
United States Environmental Protection Agency, (2004). “Microwave Assisted Acid Digestion of Siliceous and Organically Based Matrices,”(Washington, D.C., United States: United States Environmental Protection Agency).
van Nugteren, P., Herman, P. M. J., Moodley, L., Middelburg, J. J., Vos, M., and Heip, C. H. R. (2009). Spatial distribution of detrital resources determines the outcome of competition between bacteria and a facultative detritivorous worm. Limnol. Oceanogr. 54 (5), 1413–1419. doi:10.4319/lo.2009.54.5.1413
Wang, S. L., Xu, X. R., Sun, Y. X., Liu, J. L., and Li, H. B. (2013). Heavy metal pollution in coastal areas of South China: a review. Mar. Pollut. Bull. 76, 7–15. doi:10.1016/j.marpolbul.2013.08.025
Wang, Y., · Wang, Y., · Chen, X., and · Zhang, L. (2023). Source-to-sink process and risk assessment of heavy metals for the surface sediment in the northern South China Sea. Environ. Sc. Poll. Res. 30, 2721–2739. doi:10.1007/s11356-022-22281-9
Yao, Q., Wang, X., Jian, H., Chen, H., and Yu, Z. (2015). Characterization of the Particle Size Fraction associated with Heavy Metals in Suspended Sediments of the Yellow River. Int. J. Environ. Res. Public Health 12 (6), 6725–6744. doi:10.3390/ijerph120606725
Zonta, R., Botter, M., Cassin, D., Bellucci, L. G., Pini, R., and Dominik, J. (2018). Sediment texture and metal contamination in the Venice Lagoon (Italy): A snapshot before the installation of the MOSE system. Coast. Shelf Sci. 205, 131–151. doi:10.1016/j.ecss.2018.03.007
Keywords: metals, statistical analysis, natural vs. anthropogenic, source to sink, Naples Bay, eastern Tyrrhenian Sea
Citation: Milia A, Buonocunto FP, Mali M, Giandomenico S, Leo AD, Spada L, Ferraro L and Giordano L (2023) Natural vs. anthropogenic metals for reconstructing the source-to-sink pathway (Naples Bay, eastern Tyrrhenian Sea). Front. Earth Sci. 11:1225658. doi: 10.3389/feart.2023.1225658
Received: 19 May 2023; Accepted: 07 August 2023;
Published: 30 August 2023.
Edited by:
Dario Omanovic, Rudjer Boskovic Institute, CroatiaReviewed by:
Nicolas Layglon, University of Geneva, SwitzerlandNeven Cukrov, Ruđer Bošković Institute, Croatia
Copyright © 2023 Milia, Buonocunto, Mali, Giandomenico, Leo, Spada, Ferraro and Giordano. This is an open-access article distributed under the terms of the Creative Commons Attribution License (CC BY). The use, distribution or reproduction in other forums is permitted, provided the original author(s) and the copyright owner(s) are credited and that the original publication in this journal is cited, in accordance with accepted academic practice. No use, distribution or reproduction is permitted which does not comply with these terms.
*Correspondence: Alfonsa Milia, alfonsa.milia@cnr.it