- 1Curt-Engelhorn-Zentrum Archäometrie, Mannheim, Germany
- 2Department of Cultural Studies and Antiquities, Ludwig Maximilians University, Munich, Germany
- 3Eurasia Department, German Archaeological Institute, Berlin, Germany
- 4Institute of Prehistory, Early History and Medieval Archaeology, Schloss Hohentübingen, Eberhard Karls University, Tübingen, Germany
The Bronze Age in Central Asia was dominated by the Andronovo Culture and the Bactria-Margiana Archaeological Complex (BMAC). Both cultural entities produced bronze, however, the extent of bronze production and use varied considerably in space and time across their territories. The introduction and spread of bronze metallurgy in the region is commonly associated with the Andronovo Culture, but comparatively little is known about the copper and tin sources that were exploited to make the bronze. To shed light on this aspect, this paper examines 91 bronze artefacts from the Middle Bronze Age (MBA) and the Late Bronze Age (LBA) recovered from twenty sites of Andronovo and the BMAC through a combined evaluation of chemical and isotopic analyses. Trace element patterns and isotopic compositions of lead, tin, and copper are determined for the objects complemented by tin isotope analysis of Central Asian tin ores. The data shows a clear separation of two source areas in the MBA and LBA I: the BMAC obtained copper from polymetallic (tin-bearing) deposits in Iran (Deh Hosein, Nakhlak/Bagh Gorogh) and possibly Afghanistan, while the Andronovo Culture mainly used copper from the Tian Shan Mountains. With the transition to the LBA II, a change in the material basis can be recognised, in which the BMAC increasingly relied on metal deposits from the Andronovo territory. The most important result in this context is the analytical proof of the coextraction of copper and tin from the copper-tin mine at Mushiston, Tajikistan, and the first direct link of tin in bronze objects with a tin deposit. Mushiston apparently supplied both cultural macro regions with a “natural” bronze, which accounted for about one third of all objects analysed, but there is no indication yet that metal or ores from Mushiston were traded or used at a distance of more than 500 km. Moreover, the artefact data indicates a decline in the exploitation of the mine in the course of the developed LBA, while other copper and tin sources in the Tian Shan and probably the Hindukush were exploited. This testifies to the intensive use of the rich mineral resources of Central Asia and beyond, as well as the intensification of cultural and trade contacts between Andronovo and the BMAC.
1 Introduction
Central Asia is a geographic region with a rich metallurgical tradition since prehistory. During the Middle (MBA) and Late Bronze Age (LBA) (3rd–2nd millennia BCE), the territories of modern Turkmenistan, Afghanistan, Uzbekistan, and Tajikistan—known historically as Margiana and Bactria—were inhabited by the population of the Bactria-Margiana Archaeological Complex (BMAC) or Oxus civilisation (Figure 1). The communities of the BMAC founded settlements throughout the region and practiced agropastoral subsistence near rivers and in fertile oases. The largest site excavated so far is that of Gonur Depe, located in the Karakum desert, but there are other important settlements southwest of Gonur, such as Namazga Depe or Altyn Depe and further east in the Bactrian plains and adjacent hilly landscapes with Dashly Tepe, Sapalli Tepe, Dzharkutan or Molali (see Figure 1A). Through these sites and archaeological finds, we are well acquainted with the cultural repertoire and the lifestyle of the BMAC as well as its funeral rites (Lyonnet and Dubova, 2021). Nevertheless, many aspects such as the cultural interaction between local and supra-regional communities or the economic foundations of the BMAC are not yet fully understood.
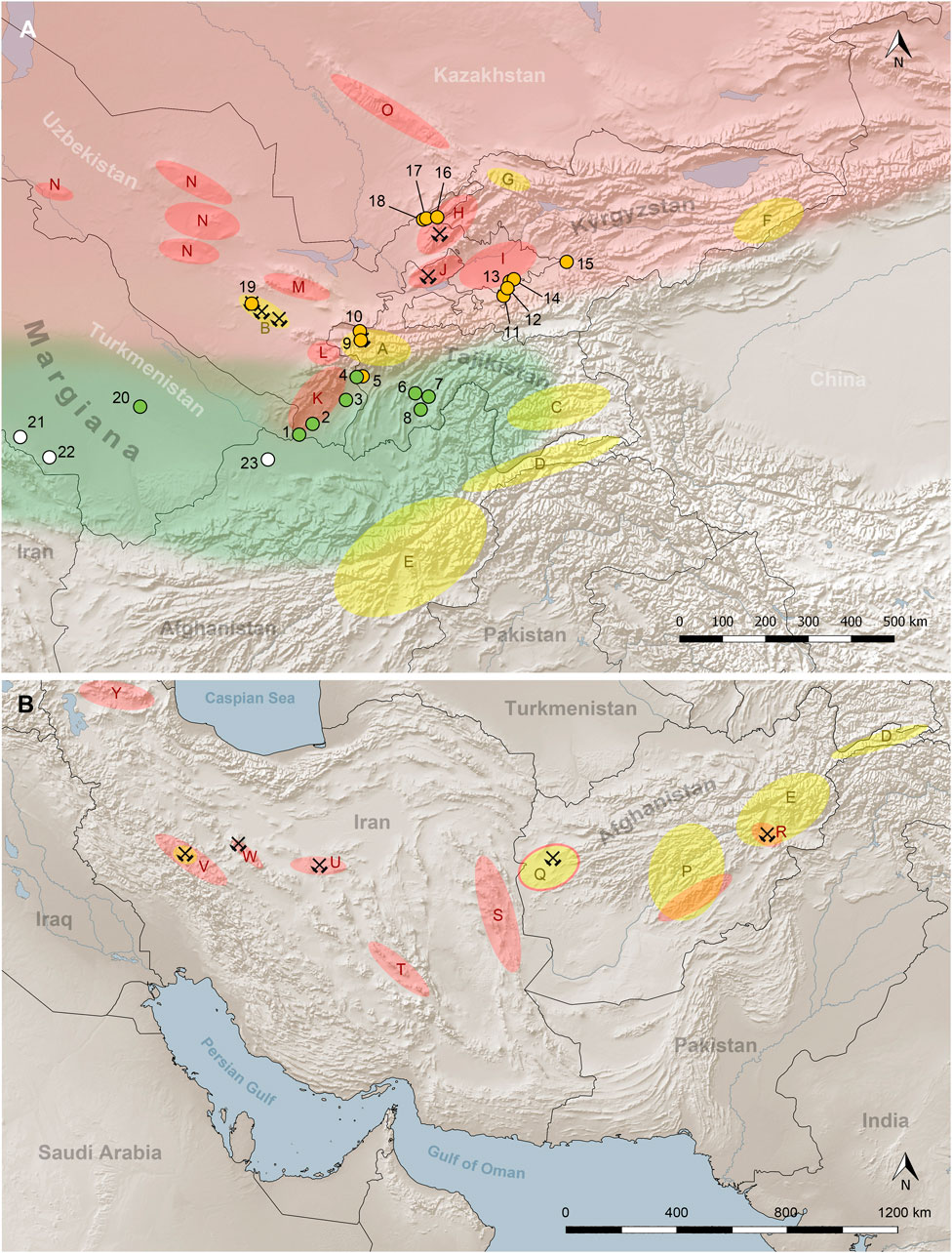
FIGURE 1. Topographic map of Central Asia (A) and parts of the Near East (B) with sites from which bronze objects were analysed in this study. Green symbols are sites belonging to the BMAC, while those in orange are attributed to the Andronovo cultural phenomenon. Other sites mentioned in the text are shown in white. The green and red shaded areas correspond to the distribution of the BMAC (green) and Andronovo (red). Sites: 1—Sapalli Tepe, 2—Dzharkutan, 3—Molali, 4—Tandyryul, 5—Kumsay, 6—Kangurttut, 7—Saridzhar, 8—Gelot, 9—Mushiston, 10—Dasht-i Kozi, 11—Vuadil’, 12—Yapagi, 13—Kashkarchi, 14—Arsif, 15—Uzgen, 16—Brichmulla, 17—Chimbaylyk, 18—Iskandar, 19—Karnab-Sichkonchi, 20—Gonur Depe, 21—Namazga Depe, 22—Altyn Depe, 23—Dashly Tepe; Ore deposits (red and yellow ovals): A—Hissar Mountains with Mushiston mine (Sn, Cu), B—Zirabulak Mountains with Karnab, Lapas and Changali mines (Sn), C—Southeast Pamirs (Sn, Ag), D—Afghan Badakhshan province (Sn), E—Hindukush (Sn), F—Kakschaaltoo (Sn), G—Talas ore district (Sn, Ag), H—Chatkal Mountains with Aktashkan copper mine (Cu), I—Ferghana (Cu), J—Karamazar, Kuraminsk and Kurama Mountains with Kani Mansur, Kaynar and Almalyk/Nakpeisai copper mines (Cu, Ag), K—Kugitangtau Mountains (Cu), L—Kashkadarya (Cu), M—Nuratau Mountains (Cu), N—Kyzylkum (Cu), O—Karatau Mountains (Cu), P—Central Afghanistan and southern Hindukush (Sn, Cu), Q—Afghan Herat province (Sn, Cu) with Misgaran and Shaida copper mines, R—Afghan Loghar province with Mes Aynak copper mine (Cu), S—Eastern Iran including Lut Block, T—Kerman province, U—Anarak area with Nahklak, Bagh Gorogh and Talmessi mines (Cu, Pb, Ag), V—Astaneh-Sarband area with Deh Hosein, Astaneh and Nezam Abad mines (Sn, Cu), W—Karkas area with Veshnaveh and Komjan mines (Cu, Pb, Ag), Y—North Iran [map: D. Berger, S. Kraus, C. Frank; ore deposits based on Berthoud et al. (1978), Nezafati (2006), Sverchkov (2010), Garner (2013), Thomalsky et al. (2013)].
One of the most important aspects, which is far from being solved satisfactorily, concerns the emergence and adoption of bronze metallurgy, which also includes the organisation of metallurgical activities, the distribution of metal and the origin of copper and tin. Shedding additional light on this aspect is of particular interest to cultural history, not least because Central Asia occupies an intermediate position between Southwest Asia, “the heartland in which bronze technology was innovated” (Pigott, 2020, 832), and China, to where the knowledge of bronze metallurgy was transmitted during the 3rd and 2nd millennia BCE (Pigott, 2020). Furthermore, Central Asia has extensive mineral resources such as copper and tin, but also gold and lapis lazuli (Parzinger et al., 2003; Peters et al., 2007; Garner, 2013; Thomalsky et al., 2013; Garner, 2015; Kraus et al., Forthcoming; Steiniger and Junker, 2020; Garner, 2021), and the region potentially supplied these materials to more distant regions.
Tin seems to be a key material in this context. As the major component of bronzes besides copper, tin deposits are inhomogeneously distributed across Eurasia and distinctly less common than the much more abundant copper. Especially in Mesopotamia, the presumed “heartland” of bronze metallurgy, from where most of the earliest bronze objects are known, the region lacks deposits that could have delivered tin for the production of bronze on a larger scale. The rich tin mineralisations of Afghanistan, Uzbekistan and Tajikistan (Figure 1) are therefore of particular importance, as they may have supplied not only sufficient tin for the local bronze industry, but also beyond. For this reason, some scholars consider Central Asia a potential source region of tin for early bronzes in the Near East (e.g. Parzinger et al., 2003; Thornton et al., 2005; Powell et al., 2021; Powell et al., 2022). Recently, it has even been claimed that Central Asian tin was traded to Anatolia, where it allegedly ended up in one third of the famous 14th-century BCE tin ingots from Uluburun, Turkey (Powell et al., 2022). However, as we show in another article in this special issue (Berger et al., 2023), this claim is unsustainable against the background of existing data, even though Afghanistan remains an option. In view of the ongoing debates, it is worthwhile from several perspectives to examine the metallurgical remains and supply chains of Central Asia in more detail by archaeometric methods in order to complete the overall picture of early bronze adoption and transmission.
The present article is not the first attempt in this regard. Since the mid-20th century, chemical analyses of Central Asian metal objects have been carried out to chart the development of ancient metallurgy. Initially, the analytical programs were dominated by Soviet scholars, who analysed hundreds of metal artefacts from the BMAC (e.g. Ruzanov, 1980; Ruzanov, 1982; Kouznetsova et al., 1988; Kuz’mina, 1991; Kuz’mina, 2004; Chernykh, 1992), but since the 1970s the number of interdisciplinary projects involving western scientists have been increasing (e.g. Jettmar, 1971; Berthoud et al., 1978; Isakov et al., 1987; Parzinger and Boroffka, 2003; Thomalsky et al., 2013; Kraus et al., Forthcoming). Over time, thousands of chemical datasets of metal objects from across the region have accumulated. They show that the use of unalloyed, arsenical, and leaded copper dominated in the Early Bronze Age (3rd millennium BCE), while the proportion of bronzes increased significantly with the turn of the MBA (3rd to 2nd millennium). The use of bronze in the early 2nd millennium BCE was apparently more widespread in Bactria, where it was used intensively by the LBA Sapalli Culture as the eastern branch of the BMAC (Chernykh, 1992; Ruzanov, 1999; Kaniuth, 2006; Kaniuth, 2007; Kraus, 2021). The reasons for this divergence are not yet entirely clear, but the lack of nearby tin sources exploited by the BMAC in the Bronze Age necessitated interconnections with the people of the Andronovo cultural phenomenon living to the north of Bactria who were controlling the exploitation of tin sources (Figure 1).
In contrast to Bactria (and Margiana), modern prospection by the Soviets and mining archaeological investigations in the former Andronovo territory have identified a number of prehistoric tin mines in the Zirabulak and Hissar Mountain ranges south of the valley of the Zeravshan River (Figure 1A) (Parzinger and Boroffka, 2001; Parzinger and Boroffka, 2003; Garner, 2013; Garner, 2015; Garner, 2021). For the mines of Karnab, Lapas, and Changali in the Zirabulak Mountains in Uzbekistan, open-pit mining of cassiterite ores can be traced back to the first half of the 2nd millennium BCE, while horse and camel bones as well as BMAC pottery and stone vessels in the Karnab-Sichkonchi settlement attest to relations between Andronovo and Bactria (Parzinger and Boroffka, 2003). Even earlier underground mining by the communities of Andronovo could be documented further east at Mushiston in Tajikistan at an altitude of about 3500 m above sea level in the Hissar Mountains (Figure 2). The mineralisation of Mushiston is of a special type in that copper and tin—the main bronze components—are associated here in the form of primary stannite (Cu2SnFeS4) and secondary tin and copper as well as mixed tin-copper minerals within a rich gossan (Alimov et al., 1998; Garner, 2013; Sun et al., 2017; Berger et al., 2022a; Konopelko et al., 2022). Radiocarbon dates prove exploitation as early as the late 3rd millennium BCE, and most recently a bronze slag from the early 2nd millennium has been correlated with Mushiston by means of isotopic and chemical data (Garner, 2013; Berger et al., 2022a). This testifies not only to tin ore mining in an adverse environment, but also to nearby ore smelting. With these findings, Mushiston is currently one of the most promising tin suppliers for the Central Asian Bronze Age, but the Uzbek deposits in the Zirabulak Mountains and those further east and north in Kyrgyzstan and Kazakhstan (Figure 1) are of equal interest for future investigation (Stöllner et al., 2011; Garner, 2013; Stöllner et al., 2013; Garner, 2015; Garner, 2021).
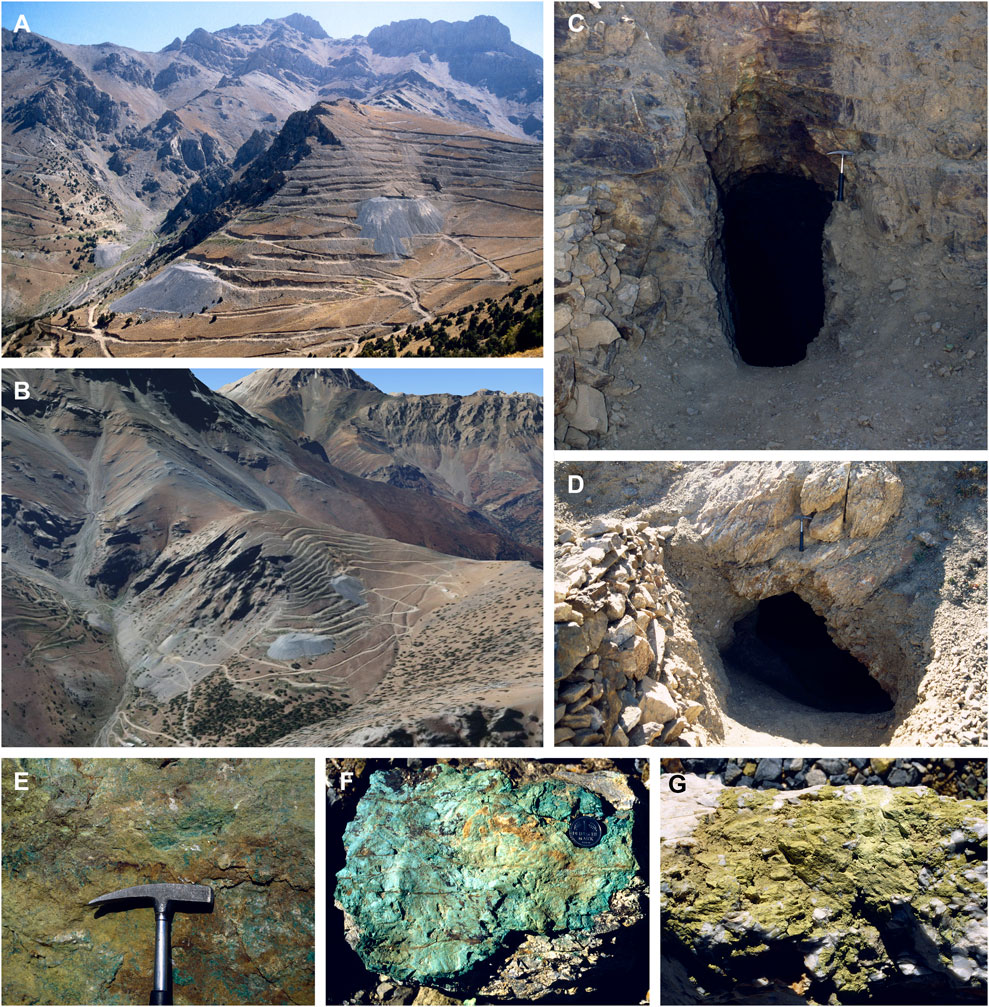
FIGURE 2. Views of the Mushiston deposit from the north and north-east (A, B), Bronze Age galleries (C, D), oxidised ores in one of the prehistoric adits (E), and copper (F) and tin ores (G) found in spoil heaps from modern prospecting (photos: J. Lutz).
Together with a number of prehistoric copper mines (cf. Figure 1), all tin mines identified so far were exploited by members of the Andronovo cultural phenomenon (Cierny, 1995; Parzinger and Boroffka, 2001; Cierny and Weisgerber, 2003; Parzinger and Boroffka, 2003; Garner, 2013; Garner, 2021). This association of steppe communities with its pronounced mobile lifestyle, inhabited a territory to the north of the BMAC that stretched from the Urals to China (Figure 1A) (Grigoriev, 2021). The members of these communities were accomplished miners and metalworkers and produced high-quality bronzes, the latter of which are steadily increasing in number thanks to on-going excavations and metal analyses. It is widely accepted that Andronovo populations played an important role not only in the exploitation of tin ores, but also in the production of bronze and its trans-regional distribution. Some scholars even suggested that Andronovo was responsible for the transmission of bronze to the east. Despite intensive research, however, it has not yet been possible to link any of the tin deposits to Andronovo bronzes, let alone to those of the BMAC or beyond. The chemical and the few lead isotope studies in the past (Kraus, 2016; Kraus, 2021; Kraus et al., Forthcoming) have contributed to a better understanding of the metallurgy and the metals and alloys used, but the origin of the tin for the bronzes remained enigmatic.
The following paper aims to fill this research gap. By analysing a series of bronzes from both the BMAC and the Andronovo circle, we attempt to trace the pathways and consumption of tin and copper from the MBA to the LBA. For this purpose, we use a multi-proxy approach of stable isotope (tin, copper), radiogenic isotope (lead) and chemical analysis, which already succeeded in linking the Mushiston slag (Figure 3F) with the nearby ores (Berger et al., 2022a). We additionally present tin isotope data from Central Asian tin mineralisations (Hindukush, Pamir Mountains, Zirabulak Mountains, Kazakhstan, Kyrgyzstan). Along with available data of copper ores, this should disclose the supply chains and trade routes of tin and copper, which could ultimately help to better understand the spread of bronze technology. Although it is not the primary aim of this paper to solve the long-standing “tin mystery” of the Bronze Age (von Baer, 1876; Muhly, 1985; Maddin, 1998), our work will give new impetus to the discussion of tin provenance in the Old World.
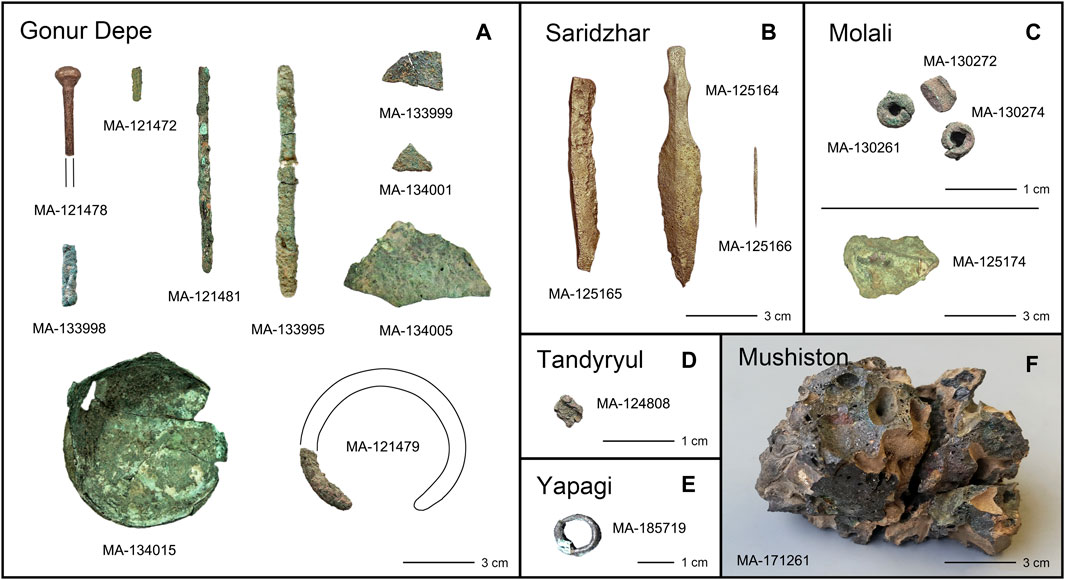
FIGURE 3. Part of the analysed bronze objects from the BMAC (A–E) and the Andronovo bronze slag (F) found only 4 km from the Mushiston deposit (photos: N. Boroffka, S. Kraus, M. Teufer, D. Berger).
2 Material and methods
2.1 Archaeological objects and cultural background
Ninety-one bronze artefacts were analysed in this study that cover a time span from the late 3rd millennium to around 1300 BCE, attributable to the MBA and the LBA. The majority of the objects is of LBA date and derive from both the BMAC and the Andronovo cultural phenomenon (Tables 1, 2; Supplementary Table S1). The bronzes of the BMAC (see Lyonnet and Dubova, 2021; Fontugne et al., 2021 for a catalogue of 14C dates) can be divided into three chronological groups: 1) Bronzes belonging to the MBA (2000–1950 BCE), 2) bronzes dating from the LBA I (1950–1750 BCE), and 3) bronzes coming from LBA II (1750–1450 BCE) contexts.
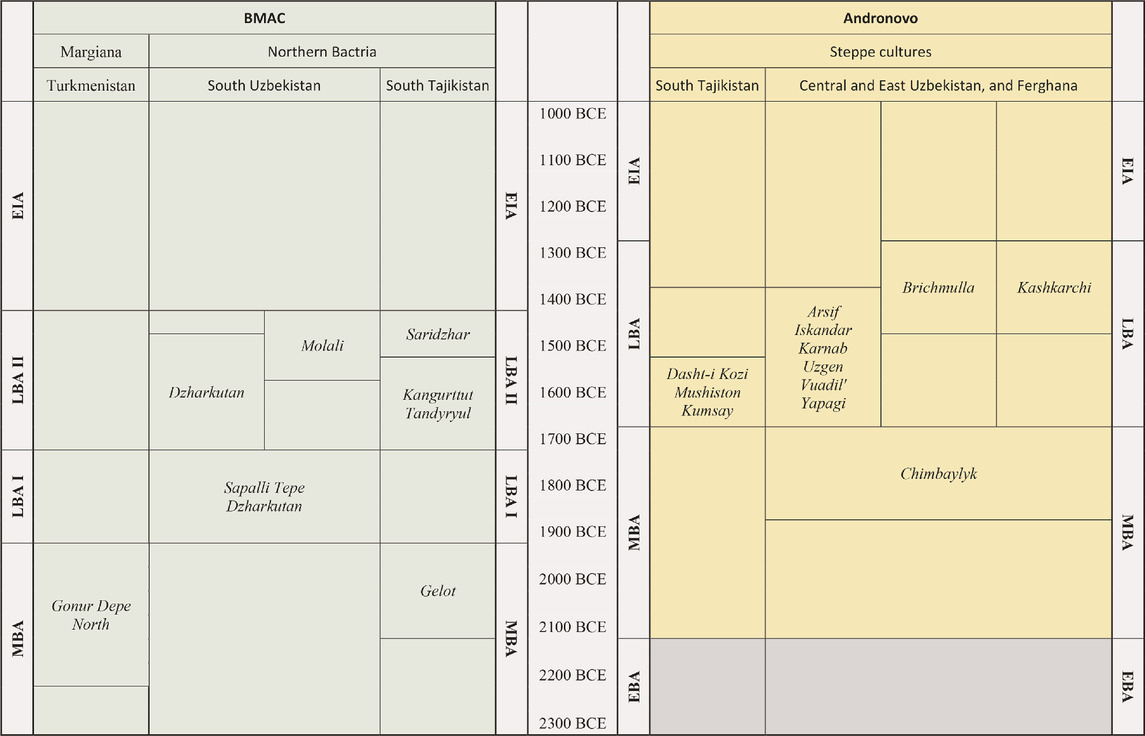
TABLE 1. Chronological overview of cultures and sites discussed in the text. Greenish background—BMAC-related, orange background—Andronovo-related.
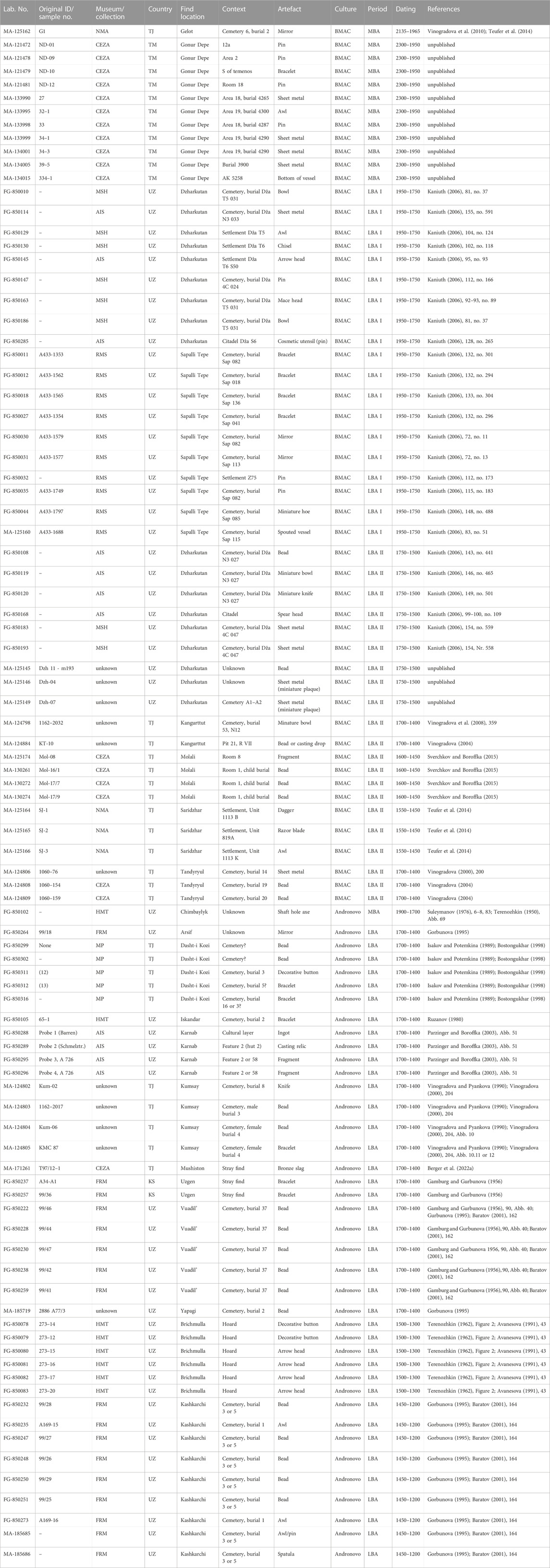
TABLE 2. Summary of analysed bronzes artefacts. Collections: AIS—Archaeological Institute Samarkand, CEZA—Curt-Engelhorn-Zentrum Archäometrie Mannheim, FRM—Ferghana Regional Museum, HMT—Historical Museum Tashkent, MP—Museum Pendzhikent, MSH—Museum Sherabad, now in Archaeological Museum Termez, NMA—National Museum of Antiquities Dushanbe, RMS—Registan Museum Samarkand, now in Samarkand State Museum for the History of Culture; Country: KS—Kyrgyzstan, TJ—Tajikistan, TM—Turkmenistan, UZ—Uzbekistan; Culture: BMAC—Bactria-Margiana Archaeological Complex; Period: MBA—Middle Bronze Age, LBA—Late Bronze Age.
The earliest bronzes of our sample set were excavated at Gonur Depe, an urban complex in the inland delta of the Murghab River, Turkmenistan (Figure 1A) (Sarianidi, 1990; Sarianidi, 2001; Sarianidi, 2005; Sarianidi, 2007; Sarianidi and Dubova, 2010; Dubova, 2019). The complex consists of a MBA northern city (Gonur North) with monumental buildings, and several massive rectangular walls with towers, as well as the LBA I compound of Gonur South with further buildings and enclosures. Situated on the opposite bank of the former river Murghab, a large cemetery with several thousand graves was uncovered, whereas some very rich “royal” burials were found inside the outer wall of Gonur North. The eleven bronzes in question (Figure 3A) are a bracelet (MA-121479), five pins or awls (MA-121472, -78, -81, MA-133995, -98), the bottom of a vessel (MA-134015), and several pieces of bronze sheet metal (MA-133990, -99, MA-134001, -05). They were unearthed from different areas of Gonur North, either from the living areas or from graves; thus most of them are not directly related with each other except for MA-133999 and -134001 from burial 4290 (Table 2). The entire complex of the northern city has been radiocarbon-dated to between 2200 and 1950 BCE (Fontugne et al., 2021). A similar dating is suggested for a bronze mirror from a cemetery near the modern town of Gelot in southern Tajikistan (MA-125162) (Figures 1A, 4E; Tables 1, 2). It comes from a rich female burial and was found together with beads of gold and lapis lazuli, as well as many ceramic vessels. Radiocarbon dating on human teeth from the grave yielded consistent dates in the time bracket 2135–1965 BCE (Vinogradova et al., 2010; Teufer et al., 2014; Fontugne et al., 2021).
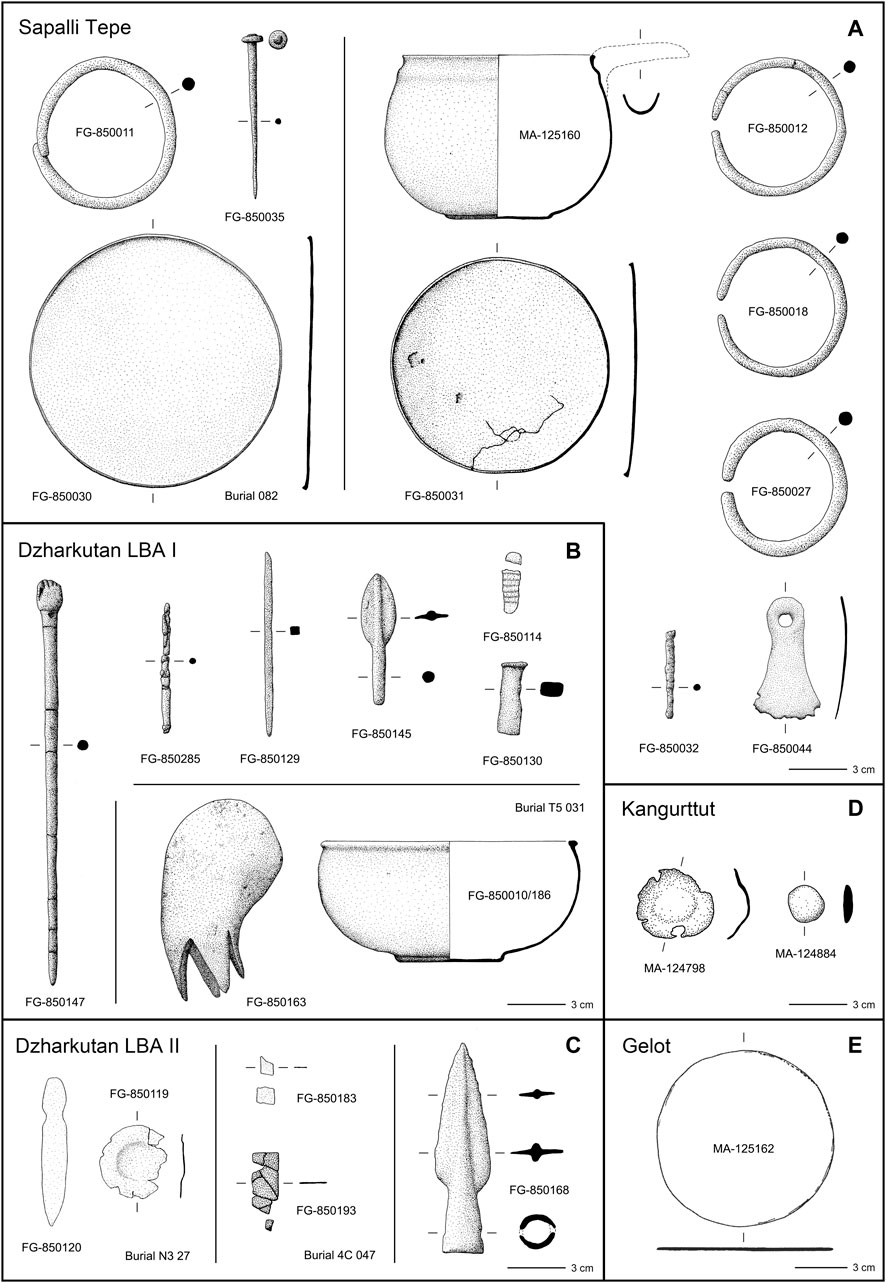
FIGURE 4. Analysed BMAC bronzes from Sapalli Tepe (A), Dzharkutan (B, C), Kangurttut (D) and Gelot (E) [drawings: U. Jansen; D. Berger and from Kaniuth (2006), Vinogradova et al. (2010)].
Eighteen objects included in this study (Figures 4A, B; Table 2) were discovered in burial and settlement contexts dating to the LBA I phase (1950–1750 BCE) of the southern Uzbek Sapalli Culture as the eastern tail of the BMAC. Shortly after the turn of the millennium, the Surkhandarya plain was settled by a BMAC population, possibly from neighbouring Turkmenistan. The two major settlements, Sapalli Tepe and Dzharkutan (Figure 1A), have been excavated since the 1970s by Soviet, Uzbek, German, and French missions (Askarov, 1973; Askarov, 1977; Askarov and Abdullaev, 1983; Askarov and Shirinov, 1993; Teufer, 2015; Bendezu-Sarmiento and Lhuillier, 2019). Both sites were centred on fortified complexes not unlike those discovered in Turkmenistan (Gonur Depe, Togolok) and Northern Afghanistan (Dashly Tepe). Burials yielded numerous metal artefacts consisting of a variety of alloys (arsenical copper, leaded copper, bronze), of which bronzes account for one quarter. The funerary customs of the time saw the burial of functional objects, which were withdrawn from circulation and may have been part of the deceased’s possessions (Kaniuth, 2006; Kaniuth, 2007). The majority of objects discovered were part of cosmetic sets from female burials, from which bracelets (FG-850011, -012, -018, -027), mirrors (FG-850030, -031) and pins (FG-850032, -035, -147) were included here (Figures 4A, B). Metal is comparatively rare in male burials, and may be overrepresented in the present sample set consisting of a miniature hoe (FG-850044), a mace-head (FG-850163), and a bowl (two samples: FG-850010/-186). Bronzes in child graves such as a vessel (MA-125160) are a rarity. Smaller, typologically indistinct tools from settlement contexts (FG-850114, -129, -130, -145, -285) make up the balance. It should be noted that two elite burials are represented multiple times in our sample set, the female burial 082 from Sapalli Tepe (FG-850011, -030, -035), and the potential male burial T5 031 from Dzharkutan (FG-850163, -010).
In the LBA II (1750–1450 BCE), there are clear changes in burial customs, metallurgical practices, and also the nature of archaeological evidence of the BMAC. Investigation of this period relies almost exclusively on burial finds, while objects from urban contexts are rare. The spectrum of finds is dominated by bronze beads and sheet metal objects made specifically for the use in funerary contexts. Compared to the preceding LBA I, the proportion of metal objects in male burials increased, and there is a change in the alloying practice. Half of the objects now consists of bronze, while the other half is unalloyed copper (Kaniuth, 2006; Kaniuth, 2007). Typologically, there are some indications of an Andronovo influence from the north, such as a socketed spearhead from Dzharkutan (FG-850168) (Figure 4C). However, the cultural attribution and dating of this particular object could be a case of debate as it does not have a clear context and typologically close spearheads are known from both the Andronovo circle (Avanesova, 1991) and northern Iran (Nokandeh et al., 2006). Thus, it is not possible to attribute the spearhead to either the LBA I or II. Other bronzes from Dzharkutan included in the study came from burial contexts and clearly belong to the LBA II: A bronze bead (FG-850108), a miniature bowl (FG-850119), and a miniature knife (FG-850120) were found associated in burial N3 027, while from burial 4C 047 two metal fragments (FG-850183, -193) are available (Figure 4C). Another three objects, a bead (MA-125145) and two miniature plates (MA-125146, -149) were found in other burials at Dzharkutan.
The almost contemporary sites of Tandyryul and Kangurttut provided further objects for the study. Tandyryul, located near the village of Negmat-Baca in the Hissar valley in southern Tajikistan (Figure 1A), displays a clear Sapalli Culture material assemblage, but with a higher proportion of handmade ceramic vessels. From 1974 to 1977, a total of 34 graves were excavated in the necropolis (Vinogradova, 2004). As in LBA II Dzharkutan, bronze burial gifts are either sheet metal or beads, three of which from different graves were analysed by us (MA-124806, -808, -809) (Figure 3D; Table 2). The settlement and the cemetery of Kangurttut are also located in southern Tajikistan, between the Nursek Lake and the Tairsu River near the eponymous village (Figure 1A). Traces of a Neolithic occupation and sherds of both the Yaz Culture (Early Iron Age) and Andronovo were found in the area of the Bronze Age settlement, indicating that the entire place was used over an extended period of time. On the other hand, the 91 graves from the cemetery contained only material of the Sapalli Culture, from which again a bronze bead (MA-124884) and a miniature bowl (MA-124798) were analysed (Vinogradova, 2004; Vinogradova et al., 2008) (Figure 4D; Table 2).
A further seven bronzes objects came from the urban structures and burials in the settlement of Molali, southern Uzbekistan, and the settlement of Saridzhar in the Yakhsu valley of southern Tajikistan (Figure 1A). Both sites belong to the later phase of the LBA II (Table 1). Molali was originally discovered in 1970 (Belyaeva and Khakimov, 1973), but larger excavations were carried out during an Uzbek-German expedition in 2012 and 2013, uncovering parts of the large settlement. It had been enclosed by a massive circular wall with radially arranged inner architecture. Three main building levels were radiocarbon-dated to ca. 1600–1400 BCE (centred mainly around 1500 BCE) (Sverchkov and Boroffka, 2015). One fragment of an undetermined bronze artefact (MA-125174) was found inside one of the buildings, while three beads from a wristband (MA-130261, -272, -274) originate from a child burial underneath the floor of another building (Figure 3C; Table 2) (Sverchkov and Boroffka, 2015). The three artefacts from Saridzhar analysed in the study are a dagger (MA-125164), a razor blade (MA-125165), and an awl (MA-125166) (Figure 3B; Table 2). All three objects were found during excavations in 2012–2013 in the contexts of the settlement. Radiocarbon dates from the site fall between 1735 and 1388 BCE (Teufer et al., 2014), ceramics parallel the site with Molali, i.e. the late 16th and 15th centuries BCE. Interestingly, the bronze dagger is a typical Andronovo form of the Fedorovo phase, so again, we face a cultural influence from territories in the north. In addition, the pottery is mostly handmade, reflecting the transitional nature of the settlement between the Bronze and Iron Ages, as well as its intermontane cultural and economic background.
Between 2100 and 1400 BCE, populations appeared in southern Central Asia displaying a material culture with clear Eurasian steppe origins (Figure 1A). We follow here their labelling as “Andronovo”, all the while acknowledging that this term is no more than a proxy for steppe-related artefacts and burial customs, and—that strictly speaking—they would be better referred to as either Kayrak-Kum (Ferghana)-, Tazabagyab-, Federovo- or Zeravshan-group sites and objects. Still, with discussions over the correct taphonomy of 2nd millennium BCE cultures in the southern Urals and Kazakhstan ongoing unabated (Avanesova, 1991; Koryakova and Epimakhov, 2007; Molodin and Polos’mak, 2014; Grigoriev, 2021), we use the established label of “Andronovo” when referring to a material culture with Eurasian steppe background. Between 1700 and 1500 BCE, i.e. during the LBA (Table 1), BMAC and Andronovo communities maintained cultural relations and coexisted on the territory of southern Tajikistan, with few indications for acculturation or hybridisation (Vinogradova, 2000; Luneau, 2017). The site of Kumsay (Figure 1A) is the best example of this, as the material reflects different traditions. The neighbouring site of Tandyryul also shows mixed cultural traditions, but Andronovo material did not end up in the graves unlike at Kumsay.
The earliest Andronovo find within our sample set is a shaft-hole axe head from Chimbaylyk. It was discovered in 1898 along with two other artefacts during excavation by N. Nikolai between the villages of Saylyk and Chimbaylyk on the middle Chirchik River in Eastern Uzbekistan (Terenozhkin, 1950; Litvinskij et al., 1962; Suleymanov, 1976). No context is known for the finds, but the axe is an early Andronovo type (Figure 5A; Table 2), closer to the Petrov and Novij Kumak material than to that of Fedorovo (Avanesova, 1991, 13). A date of 1900–1700 BCE is therefore likely for this piece, meaning an attribution to the MBA of the steppe cultures and contemporaneity with LBA I Sapalli Tepe and Dzharkutan.
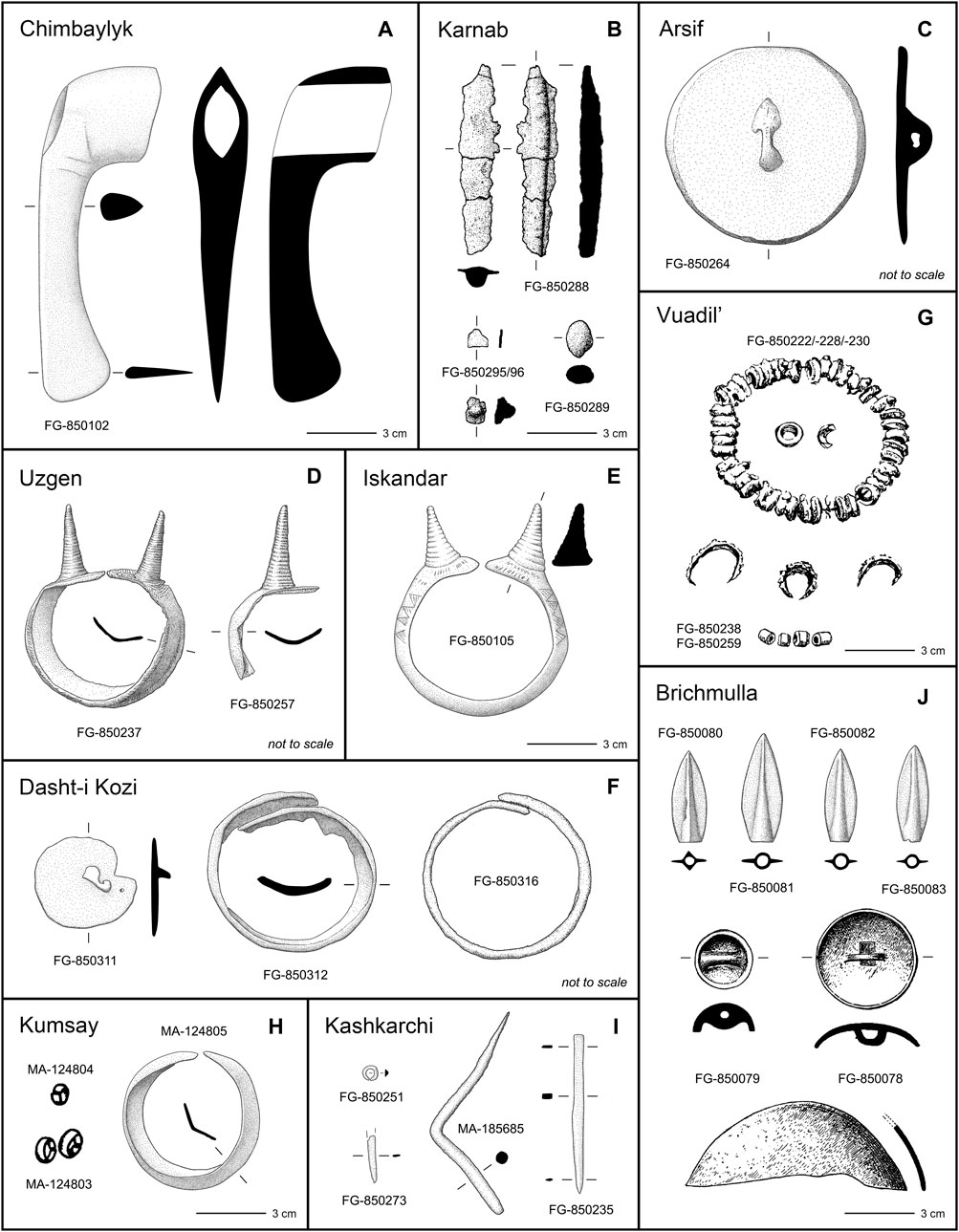
FIGURE 5. Central Asian bronze objects of the Andronovo circle analysed in the study and found at Chimbaylyk (A), Karnab (B), Arsif (C), Uzgen (D), Iskandar (E), Dasht-i Kozi (F), Vuadil’ (G), Kumsay (H), Kashkarchi (I) and Brichmulla (J) [drawings: M. Lerchl; D. Berger and from Gamburg and Gorbunova (1957), Parzinger and Boroffka (2003)].
A majority of 26 Andronovo objects in this study are contemporary with and slightly later than the LBA II of the BMAC. Two bracelets with curved section and spiral ends (FG-850237, -257) were discovered in the 1920s near Uzgen in the eastern Ferghana valley of Kyrgyzstan (Figures 1A, 5D; Table 2) (Gamburg and Gorbunova, 1956). They do not have an archaeological context either, but belong typologically to the bracelets of Fedorovo type now securely dated to the time period 1700–1400 BCE (Avanesova, 1991). The same chronology applies to another bracelet from Iskandar on the Chatkal River, not far away from Chimbaylyk (Figures 1A, 5E; Table 2). The site itself belongs to the LBA/Early Iron Age Chust Culture, but the studied bracelet (FG-850105) as well as two further ones from mounded burials are of clear Fedorovo type and thus dateable to the steppe LBA (Voronets, 1948; Kuz’mina and Vinogradova, 1983). Comparable bracelets were also found in the cemetery of Dasht-i Kozi, for which much better documentation exits. The site is situated in the Zeravshan valley near the Mushiston deposit (Figure 1A), and 27 graves of the necropolis were investigated from 1983 until 1986 (Isakov and Potemkina, 1989; Bostongukhar, 1998). Two bracelets with curved section (FG-850312, -316) analysed by us, were probably found in burials 6 and 15, whereas burial 3 yielded a bronze button (FG-850311). Two sampled beads also originate from grave contexts (FG-850299, -302) of the site, but it is difficult to attribute them to specific burials with the help of the available records (Figure 5F; Table 2). Recent radiocarbon dating of two human bones gave dates of 1620–1440 BCE (Narasimhan et al., 2019, Supplementary Material S2.2.3.19). Typological parallels with Tazabagyab/Kayrak-Kum sites (Gudzhaili, Muminabad) support this chronological attribution.
The cemetery of Kumsay in southern Tajikistan (Figure 1A) is also well-documented. Overall, 21 burials were studied in the years 1987–1988 (Vinogradova and Pyankova, 1990). Even though a more recent study of the site suggests a date in the third quarter of the 2nd millennium BCE (Kutimov, 2013), the authors correctly draw comparisons with Dasht-i Kozi (see above), and the wider cultural groups of Tazabagyab and Fedorovo are in line with the chronological horizon 1700–1400 BCE, advocated here for contemporary material. From the discovered grave inventories, four items were chosen for analysis: A bracelet—again with curved section (MA-124805)—came out of the female burial 4 and was accompanied by Andronovo pottery and bronze beads (MA-124804) (Figure 5H; Table 2). The male burial 3 contained a fragmentary wheel-made vessel and also several bronze beads of different styles, one of which was analysed (MA-124803), but could not be conclusively matched to the documentation. Also, no information is available for a knife (MA-124802) from burial 8.
Further bronzes of our sample set originate from the cemeteries of Vuadil’ and the nearby Yapagi and Arsif, all situated in the Ferghana valley in eastern Uzbekistan (Figure 1A). Thirty-seven graves of Vuadil’ and twelve burials of Yapagi were excavated in 1954–1956 and found to contain a pure Andronovo-Tazabagyab inventory; thus a date between 1700 and 1400 BCE is warranted. The five beads from Vuadil’ in our sample set apparently derive from a single grave, no. 37 (Gamburg and Gorbunova, 1957 Fig. 40,1). Three of them were part of a wristband (FG-850222, -228, -230), whereas the other two (FG-850238, -259) are of different style (Figure 5G; Table 2). The single bead from Yapagi (MA-185719) was found in burial two of the necropolis (Gorbunova, 1995) and is typologically similar with the three beads from the Vuadil’ wristband (Figure 3E). Not much information is available for the sampled mirror (FG-850264) from Arsif (Figure 5C; Table 2) or its context (kurgan burial no. 9), but the site is considered Andronovo-related (Gorbunova, 1995).
Four objects were unearthed from settlement contexts at Karnab-Sichkonchi. This site is of special interest for mining and metallurgy as it is a settlement associated with the tin mining district in the Zirabulak and Ziyaddin Mountains in central Uzbekistan (Figure 1A). It was discovered by B. Litvinskij in 1946 and intensively surveyed and excavated from 1997 to 1999 by a joint Uzbek-German expedition (Parzinger and Boroffka, 2003; Garner, 2013). As mentioned above, mining targeted the tin mineral cassiterite and processed in open-pit manner from 1700 BCE to the Early Iron Age (1300–800 BCE). The settlement has been radiocarbon-dated to the 17th–14th centuries BCE and consisted of at least eight—presumably seasonal—phases of inhabitation. Beside simple fireplaces indicating light living structures (tents or yurts), more permanent pyrotechnical structures with large integrated stones were used in several phases. The soil from one of the fireplaces showed significantly higher tin contents than the surrounding areas. Together with ore pieces (cassiterite in quartz), large numbers of mining and grinding tools, the pyrotechnical installations of the settlement are therefore most likely connected to ore processing and tin and/or bronze metallurgy. The bar-ingot (FG-850288) and the casting drop (FG-850289) analysed in the study are very likely related to the metallurgical operations at the site, while this is not certain for two bronze fragments (FG-850295/-96) (Figure 5B; Table 2).
The youngest bronze artefacts of this study from the end of the LBA came from the hoard of Brichmulla and the burial ground of Kashkarchi. The hoard was found in 1954 or 1955 in the village of Brichmulla at the shores of the Charvak reservoir in Uzbekistan (Figure 1A), but no information concerning the circumstances of its discovery or its integrity are known. At present, the hoard comprises a large shafted weapon (of unclear function, and omitted from our sample set), four arrowheads (FG-850080–83), and two decorative buttons (FG-850078–79) (Figure 5J; Table 2). Terenozhkin (1962) attributed the hoard to the Tashkent-Ferghana variant of the Andronovo Culture, considering the possibility that the buttons could have been part of a horse gear. Avanesova (1991, 43) discussed mainly the arrowheads and dated them typologically to the 12th–9th centuries BCE. Today they are rather placed in an earlier time period, most probably around 1500–1300 BCE. The cemetery of Kashkarchi, situated 6 km outside Ferghana, was also discovered in the 1950s (Figure 1A). Excavations of six aligned subterranean chambers with at least nine interred individuals were carried out in 1988 by G. Ivanov (1988). No radiocarbon dates are available, so the dating relies exclusively on ceramic and bronze typology. According to the excavator, the pottery is very similar to that of Vuadil’. One vessel with so-called “Valikovaya” (relief) decoration could date slightly later than the other Andronovo sites discussed above. The arrangement of individual burials in a row also finds comparisons in the subsequent Early Iron Age (Gorbunova, 1995; Baratov, 2001). However, similar “Valikovaya” pottery has more recently also been found at Molali (see above), and among the metal objects, a specific lock-ring type is clearly of Late Andronovo type, so that the burials should rather be dated sometime from the mid-2nd millennium BCE onwards. Nine artefacts from this closely grouped assemblage were analysed, two awls from burial 1 (FG-850273, -235) and five beads (FG-850232, -247, -248, -250, -251) and two additional awls either from burial 3 or 5 (Figure 5I).
2.2 Geological material
Besides the artefacts, 60 cassiterite samples from primary and secondary tin mineralisations in Central Asia were analysed in this study. As part of the CEZA sample collection, they either derive from previous research projects, from legacy collections and collaborators, or were bought from mineral collectors for the present study (Supplementary Table S2). The latter was particularly helpful in case of cassiterite from Afghanistan. Due to the ongoing unstable political situation in the country and the related security issues, it was impossible to take samples in the field or to acquire material from collaborators. The use of commercially available samples was thus justifiable, even if limitations regarding exact sampling locations had to be accepted. In total, forty samples from localities in the Hindukush in eastern Afghanistan, mainly large single crystals of pegmatitic cassiterite, were analysed (Peters et al., 2007; Garner, 2013; Thomalsky et al., 2013; Lyckberg, 2017). Nine additional cassiterites came from hydrothermal mineralisation of the Bazardara, Mardjanai and Buguchijilga ore clusters at Trezubets, Ghilnoye and Elisu in the South Pamir Mountains (Pavlova and Borisenko, 2009; Pavlova et al., 2015). Two cassiterite samples were recovered from mine dumps at the Takfon skarn deposit, located in the Hissar Mountains about 50 km south-west of the Mushiston copper-tin mine (Garner, 2013). Of the well-known and prehistorically exploited hydrothermal tin mineralisation in the Zirabulak Mountains south of the Zeravshan Valley, only three ore samples from Lapas could be analysed so far, although the CEZA collection comprises almost three dozen hand samples collected during the ZGMA project (Alimov et al., 1998; Parzinger and Boroffka, 2003; Garner, 2013, 2015). However, the tin contents of most of them proved to be very low, so that neither pure nor large enough cassiterite samples could be obtained for preparation and analysis. The situation was better for cassiterite collected from five sites in Kazakhstan and Kyrgyzstan. All of them consisted of rather pure cassiterite, and the samples from the Karasa Mine and Sari-Djaz in Kyrgyzstan represented the only alluvial tin of the entire sample set. The specimen of primary cassiterite from Askaraly in the Altai Mountains in eastern Kazakhstan for its part came from an archaeological context (Stöllner et al., 2013).
2.3 Sample preparation and analytical methods
2.3.1 Sampling and sample treatment
The artefact samples were obtained from former research projects (ZGMA, ROXIANA, PMU; abbreviations explained below) either by drilling objects with steel drill bits or by removing fragments with pliers. The state of metal preservation varied greatly between sites and even between artefacts from the same site. There were objects with uncorroded metal cores, as well as artefacts that were completely corroded. For the analysis, the artefacts that still had preserved metal cores were preferred. Drill samples were cleaned for this purpose by sieving and hand picking from weathered material, while fragments of artefacts were freed from their corrosion layers using conventional steel scalpels. Nevertheless, a separation of metal and corroded products was not always possible, thus we decided to use such adulterated samples when only single artefacts were available from a site. For the same reason, a number of completely corroded bronzes were subjected to analysis, when proved relevant to the scope of this work. However, in order to perform tin isotope analysis, the corroded samples required additional preparation, which is described in detail in the following paragraph. To enable readers to assess the quality of the data, the state of preservation of each sample is noted in Supplementary Table S1.
Hand samples or single crystals of cassiterite were crushed and fifty to 100 individual cassiterite grains or crystal fragments were picked, avoiding large amounts of gangue. The resulting concentrates were pulverised. Afterwards, cassiterite was subjected to thermal reduction with potassium cyanide, which is a standard method for the conversion of cassiterite into tin metal for tin isotope analysis (Haustein et al., 2010; Haustein, 2013; Mathur et al., 2017; Berger et al., 2019a). This preparation step was necessary, as cassiterite is almost insoluble in acids (Hall, 1980; Tapster and Bright, 2020). For reduction, ca. 10 mg of sample powder were loaded with a tenfold mass of potassium cyanide (99.8%) in graphite plates. The loaded plates were embedded in active carbon within a lidded corundum crucible and heated to 1100°C for 60 min with a muffle kiln. The same procedure was followed with the corroded artefact samples since corrosion layers on bronzes might contain appreciable amounts of tin dioxide (SnO2) or hydrated tin compounds (Robbiola et al., 1998). As with natural cassiterite (SnO2), these mineral species are typically highly resistant to dissolution in acids, which eventually leads to insufficient concentrations of tin in the sample solutions and to undesired fractionation of tin. Thus, thermal reduction helps to ensure obtaining correct isotope data. However, we have refrained here from the conventional thermal reduction procedure with potassium cyanide. In previous experiments and also in tests with the present corrosion samples, extensive evaporation of tin oxide and copper compounds during the reduction were observed, which should be avoided because of potential fractionation (Berger et al., 2019a). For this reason, 5–20 mg of sample powder were covered with five times the mass of copper powder (99.9% Cu) and additional potassium cyanide and reduced at 1100°C for 60 min in graphite plates. Large prills of easily dissolvable bronze developed that absorbed the entire tin and most trace elements of the former patina. No evaporation was observed with this method, thus, it is best suited for the reduction of bronze patinas. It is also important to note that no fractionation of tin isotopes between metal phase and corrosion layers was observed in experiments so far, so tin isotope data from corrosion layers of artefacts from terrestrial contexts reliably reflects that of the original metal (Berger et al., 2018a; Berger et al., Forthcoming).
2.3.2 Chemical analysis
Chemical analysis of solid metal samples was carried out with energy dispersive X-ray fluorescence spectroscopy (EDXRF) at the Curt-Engelhorn-Zentrum Archäometrie Mannheim (CEZA), Germany, using an ARL Quant’X spectrometer (Fa. Thermo Fisher Scientific Bremen, Germany). This device was equipped with a rhodium X-ray tube, a Peltier-cooled SD detector and different filters to adjust the X-ray beam to the required excitation conditions. Two excitations at 28 kV (900 s and palladium filter) and at 50 kV (600 s and copper filter) were run. Calibration was achieved with a set of certified reference copper alloys (BAM-367, BAM-368, ERM-EB374, ERM-EB375, BAM-376) and self-prepared copper alloy in-house references. Quantification occurred after a slightly modified empirical method after Lutz and Pernicka (1996). The data was normalised to 100%, and element concentrations are reported in mass% throughout the paper. A number of artefacts were analysed previously by neutron activation analysis (during the ROXIANA project), but since weathering products were not removed prior to analysis, the samples were re-analysed for this paper with EDXRF after thorough removal of corrosion. For discussion, we will only use the EDXRF data.
2.3.3 Isotope analysis
Combined lead, tin and copper isotope compositions on the artefact samples were determined, whereas reduced ore samples were only analysed for their tin isotope composition. In case of metallic material (artefacts and reduced tin and bronze), the samples were dissolved with 6N HCl and H2O2. Pulverised and homogenised corrosion layers were dissolved in aqua regia. Aliquots of the sample solutions were processed according to the respective isotope system and analysed with a Neptune Plus (Thermo Fisher Scientific, Bremen, Germany) high-resolution multi-collector inductively coupled plasma mass spectrometer (HR-MC-ICP-MS) at CEZA. The analytical procedure is fully documented in Brügmann et al. (2017b) and Berger et al. (2022b).
The copper isotope composition is expressed as difference to the international reference material NIST SRM 976 in permill (‰) as δ65Cu (= δ65Cu/63Cu). Analytical precision for each measurement was typically ±0.02‰ and all analytical errors are given as two standard deviations (2SD). Tin isotope values are referred relative to NIST SRM 3161a tin solution. Nine isotope ratios were determined (116Sn/120Sn, 117Sn/120Sn, 118Sn/120Sn, 119Sn/120Sn, 122Sn/120Sn, 124Sn/120Sn, 124Sn/116Sn, 122Sn/116Sn, 117Sn/119Sn). However, for discussion, we exclusively use δSn in permill per atomic mass unit (‰ u−1), which is defined by the slope calculated from a linear regression analysis of all measured isotope values as a function of the corresponding mass difference. The error of the slope represents the analytical uncertainty of the δSn value. δSn is used here because there is still no consistent application of tin isotope values and not even an internationally certified isotope reference material is available, although tin isotope analysis is increasingly applied in archaeological and geological research (Haustein et al., 2010; Balliana et al., 2012; Yamazaki et al., 2014; Brügmann et al., 2017a, 2017b; Creech et al., 2017; Schulze, 2018; Wang et al., 2018, 2019; Berger et al., 2019b, 2022b; Bower et al., 2019; Friebel et al., 2020; Mason et al., 2020; Liu et al., 2021; Zhou et al., 2022; She et al., 2023). This comes at the expense of comparability of data sets, especially for the unexperienced reader. We think that the use of δSn based on NIST SRM 3161a facilitates comparison of tin isotope data in the future, as it is independent from a single isotope ratio. Analytical precision for tin isotope analysis was typically ±0.02‰ (for δ124Sn/120Sn). All measured isotope values are reported in the electronic supplements (Supplementary Tables S1, 2) relative to NIST and additionally relative to Puratronic (ultrapure tin metal) used as in-house reference material in several studies in the past.
3 Results and discussion
3.1 Bronzes of the BMAC
3.1.1 Middle Bronze Age bronzes
Of the eleven samples from the earliest BMAC horizon considered here, the majority originated from Gonur Depe in the Margiana, while from Bactria only one mirror from the Gelot cemetery could be included. Unfortunately, the state of preservation of the metal was very poor throughout. Uncorroded material could only be analysed from two artefacts from Gonur Depe (MA-121478 and -481) and yielded tin contents of 2 and 1.1% respectively (Supplementary Table S1). These contents are too low to refer to the metal as proper or intentionally produced bronze, not least because the arsenic and lead contents are high (4.2 and 1.4% As; 1.7 and 0.14% Pb; Supplementary Table S1). In contrast, the tin concentrations of the corroded artefacts are higher, ranging between 4.3 and 14% for Gonur, and amounting to about 12% in the mirror from Gelot. Since tin (and other elements) tend to be enriched in corrosion layers of copper-tin alloys relative to copper (Robbiola et al., 1998; Piccardo et al., 2007), the tin contents of the artefacts might once have been lower. On the other hand, corrosion took place in an arid environment, where copper and other metals with easily soluble compounds are probably not as mobile as under humid conditions. Therefore, enrichment/depletion effects may have been less pronounced and the determined concentrations could be more or less the same as the original composition. Due to these uncertainties, we can ultimately only speculate about the quantitative composition of the objects. If the tin concentrations in the metal of the corroded objects were indeed in the same range as in the uncorroded metals (i.e. very low), then it is conceivable that these few percent of tin were accidentally introduced through the smelting of tin-bearing polymetallic ores rather than through intentional alloying. The trace element patterns support this view. All bronzes contain significant amounts of arsenic and lead (both up to 4%), as well as nickel concentrations of up to 0.5% and selenium, silver, antimony and bismuth contents up to 0.2% (Supplementary Table S1). Based on these values, Kraus (2021) argued for an arsenical copper ore as the most likely base for the bronzes.
These conclusions make Mushiston, the largest and most prominent tin-bearing polymetallic ore deposit in the region, a less likely source for the analysed bronzes. The stannite and secondary copper-tin minerals of Mushiston are characterised by high concentrations of arsenic, selenium, silver, cadmium, antimony, lead and bismuth, most of which normally pass into the metal phase during smelting (Pernicka, 1987; Pernicka, 1990; Pernicka, 2014; Berger et al., 2022a). Zinc is present as well, but normally evaporates during ore smelting and is thus not expected to occur in Mushiston metal in high amounts. At the same time, nickel is almost absent in primary and secondary ores, while its content is elevated in the analysed bronzes. Since antimony and silver contents of the metal artefacts are both low, their chemical composition does not match that of the Mushiston ores (Figures 6A–C). This is further emphasised by the lead and tin isotope composition. The lead isotope ratios of the bronzes are more radiogenic than those of the ores (Figures 7A, B), while the tin isotope values in the metal are isotopically much heavier at about 0.04–0.18‰ u−1 δSn. The latter values remain still outside the isotopic range of Mushiston even when a shift in the tin isotope composition to higher values during smelting is taken into account (leading to 0.01–0.15‰ u−1 δSncorr; Figure 8A) (Berger et al., 2018b; Berger et al., 2022a). Thus, the use of the copper-tin ores from Mushiston can be definitely ruled out. Instead, in case of the Gelot bronze (MA-125162) and a sheet metal from Gonur (MA-133990), the agreement of the lead isotope ratios with those of the well-known deposit of Deh Hosein, western Iran (Figure 1B; Figures 7A,B), is good. This polymetallic mineralisation also hosts tin minerals such as cassiterite and stannite, which occur together with arsenopyrite, chalcopyrite, galena, and other primary and secondary ore minerals (Nezafati, 2006). Exploitation of the ores is documented for prehistoric times, and scientific analyses indicate their use for the production of ancient bronzes (Nezafati, 2006; Nezafati et al., 2009; Nezafati et al., 2011; Oudbashi et al., 2021a; Oudbashi et al., 2021b). The chemical properties of the Deh Hosein ores with predominant arsenic and lead largely conform to the chemical composition of the Gelot mirror; only the elevated nickel content (0.4%) and low silver concentration (0.015%) are to some extent debatable (Figures 6A–C). On the other hand, the determined gold content of 0.08% (Supplementary Table S1) could be another clue for Deh Hosein or comparable mineralisation in the Astaneh-Sarband area, a gold-bearing region (Nezafati, 2006). For the same reason, a bronze pin from Gonur with 0.53% gold (MA-121472) could originate from a similar ore assemblage, but its lead isotope ratios along with those of a bracelet (MA-121479) match with ores in the Anarak district slightly better than with Deh Hosein (Figures 7A, B). In the Anarak region, the well-known lead-silver mine of Nakhlak is located, for which there is also evidence of ancient mining (Pernicka et al., 2011; Nezafati et al., 2021). However, the mine is neither tin- nor copper-bearing, so that the copper of MA-121472 could rather have been extracted from the nearby Bagh Ghorogh copper deposit with similar lead isotope signatures as Nakhlak (Pernicka et al., 2011; Nezafati et al., 2021). The origin of the tin would remain unclear in this case, as tin is not found at Bagh Ghorogh either (Pernicka et al., 2011). Due to its high lead content of 4.4%, Nakhlak would be eligible for MA-121479, but only if lead was added intentionally.
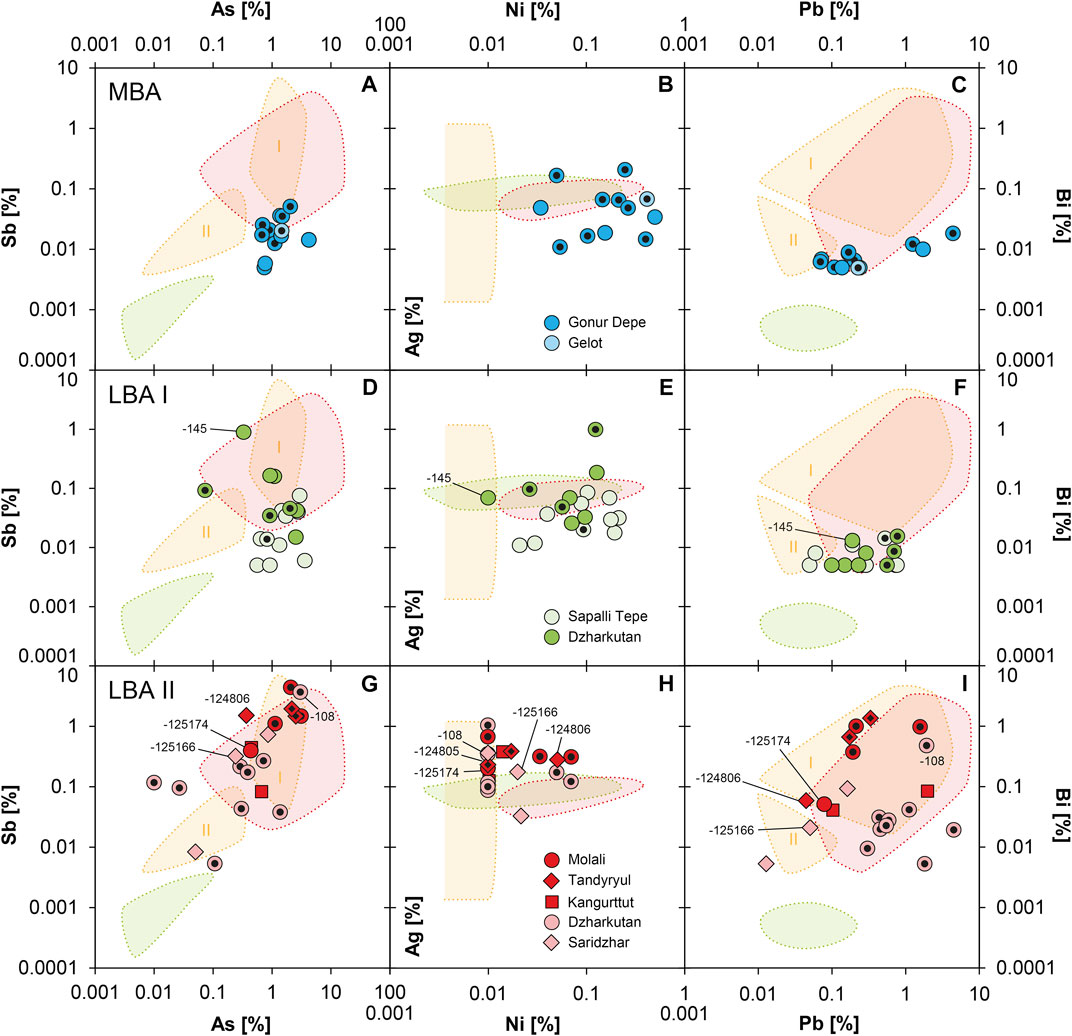
FIGURE 6. Chemical composition of the BMAC bronzes compared to the chemical composition of potential copper ore districts in Central Asia and Iran. MBA (A–C), LBA I (D–F), LBA II (G–I). Chemical data of ores have been normalised to their copper and tin contents for being comparable with artefacts and are illustrated as polygons for better legibility of the charts. The orange polygons belong to Mushiston (I—Sn-rich ores; II—Cu-rich ores), the red to Deh Hosein mine, Astaneh-Sarband area, Iran, and the green to Veshnaveh mine, Karkas area, Iran. Symbols with black dots are corroded objects; specified numbers correspond to the last three or six figures of the laboratory no. in Table 2 (diagrams and artefact data: J. Lutz, D. Berger; ore data: from Nezafati, 2006; Nezafati and Stöllner, 2017; Berger et al., 2022a).
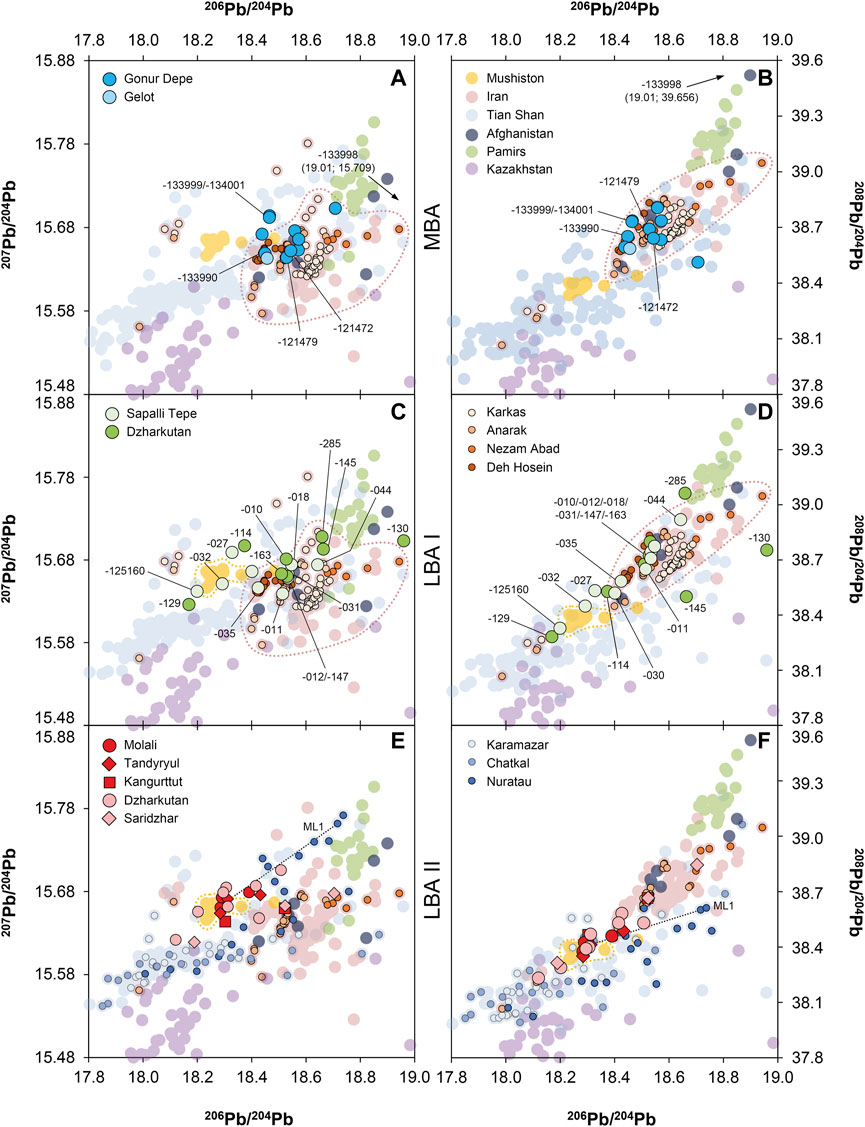
FIGURE 7. Lead isotope composition of BMAC bronzes compared to copper ores in Central Asia, Iran and Afghanistan. MBA (A–B), LBA I (C–D), LBA II (E–F). Also shown is a potential mixing line of Mushiston ores with those from the Nuratau Mountains (ML1). Specified numbers correspond to the last three or six figures of the laboratory no. in Table 2 (diagrams: D. Berger, artefact data: B. Höppner; ore data: from Brill et al., 1997; Chiaradia et al., 2006; Nezafati, 2006; Begemann and Schmitt-Strecker, 2009; Nezafati et al., 2009; Pavlova and Borisenko, 2009; Shafiei, 2010; Pernicka et al., 2011; Thomalsky et al., 2013; Merkel, 2016; Chernyshev et al., 2017; Kraus et al., Forthcoming; Nezafati and Stöllner, 2017; Wong et al., 2017; Cao et al., 2018; Berger et al., 2022a).
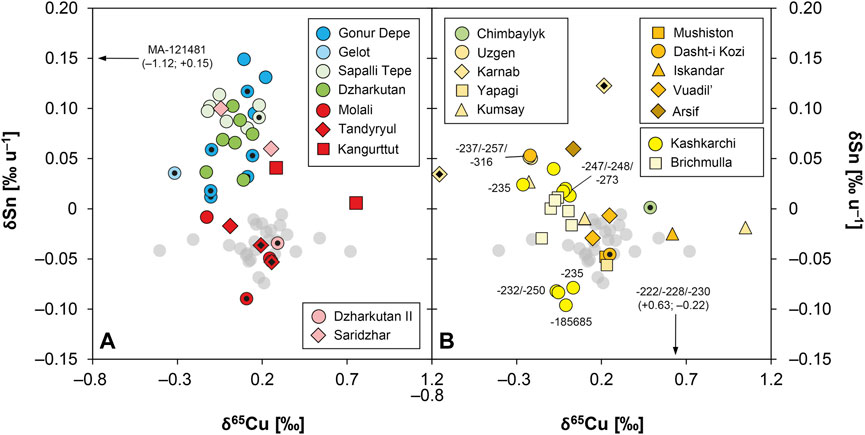
FIGURE 8. Stable isotope systematics of BMAC (A) and Andronovo (B) bronzes compared with the data of primary and the secondary copper-tin ores from Mushiston (grey circles). Symbols with black dots are corroded objects; specified numbers correspond to the last three or six figures of the laboratory no. in Table 2 (diagrams: D. Berger; artefact data: G. Brügmann and A. Wittke; ore data: from Berger et al., 2022a).
Including the few isotope data from copper mineralisation in Afghanistan opens the alternative that also copper ores from this region could have been used (Figures 7A, B). A good match for sample MA-121472 and three other specimens from Gonur Depe (MA-121478, -481, MA-134015) is obtained with ores from Shaida in the Herat province, western Afghanistan, while a bronze pin (MA-133998) has a radiogenic signature as observed for mineralisations in the Hindukush (Supplementary Table S1) (Brill et al., 1997; Begemann and Schmitt-Strecker, 2009; Thomalsky et al., 2013). Interestingly, Cleuziou and Berthoud (1982) report on polymetallic copper deposits in Herat (e.g. Misgaran) and central Afghanistan containing tin (cf. also Stech and Pigott, 1986; Thomalsky et al., 2013). The general overlap with Iranian mineralisations, as already underlined by Begemann and Schmitt-Strecker (2009), prevents a more accurate assignment, not least because lead isotope data of ores across the region is still rudimentary. However, from Figures 7A, B, it becomes clear that for the Gonur bronzes we are looking for copper sources to the south or south-west of Margiana and Bactria, maybe in Iran and Afghanistan, rather than for copper deposits in the territory occupied later by Andronovo communities. A match with ores in the Karakum and Kyzyl-Kum desert or the Tian Shan and beyond is unconvincing or even non-existent (Figures 7A, B). Smaller tin-bearing polymetallic deposits, which are widespread in the Tian Shan (Sverchkov, 2010; Garner, 2021), are therefore no likely sources either for copper or tin.
Unfortunately, the above considerations can neither be corroborated nor refuted by the tin and copper isotopes. No data is yet available for Deh Hosein and other Iranian deposits, or for tin and copper mineralisations in western and central Afghanistan. Yet, the small variation in the copper isotope values between –0.10 and +0.20‰ (Supplementary Table S1) suggests the use of similar ore bases, most likely primary sulphides such as chalcopyrite, and a secondary copper sulphide such as chalcocite according to the very negative copper isotope value of –1.12‰ in one case (MA-121481) (Klein et al., 2009; Jansen et al., 2018). These mineral species would be consistent with the deposits reconstructed above. On the other hand, the tin isotope values of pegmatitic cassiterites from the provinces in the Hindukush in eastern Afghanistan are consistently lower than those of five bronzes of Gonur Depe with δSncorr >0.09‰ u−1 (Figure 9). Comparably high δSn values were so far only determined in cassiterites from the Pamir Mountains (Buguchijilga and Bazardara ore districts) and the Zirabulak Mountains south of the Zeravshan valley (Lapas) (Supplementary Table S2; Figure 9). Signatures as those determined in the Gelot mirror and five bronzes from Gonur Depe (δSncorr <0.06‰ u−1) are, however, common in eastern Afghanistan, so that the use of such sources cannot be excluded. Since we currently cannot be sure about a deliberate alloying of copper and tin in the MBA, and no exploitation of tin ores in the region is attested, the contribution of Afghan tin to the production of the bronzes must remain as uncertain as that of tin from polymetallic copper deposits.
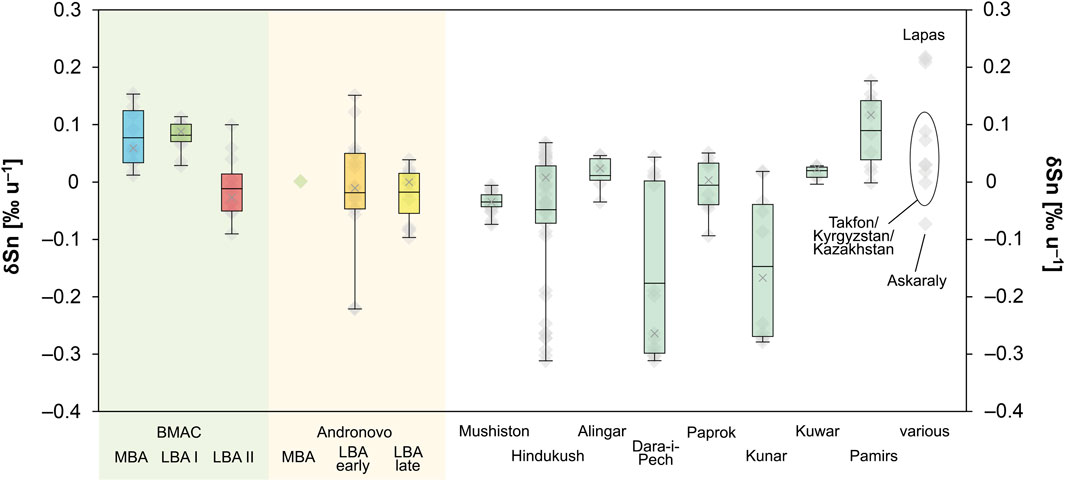
FIGURE 9. Individual tin isotope values and box-whisker-plots with averages (grey crosses) of the analysed tin ore deposits and archaeological artefacts in the study. “Hindukush” subsumes all data of the locations Alingar, Dara-i-Pech, Paprok, Kunar and Kuwar in Afghanistan, which are also shown as discrete locations. Full tin isotope composition can be found in Supplementary Tables S1, S2 (diagrams: D. Berger; data: G. Brügmann and A. Wittke).
A potentially revealing observation concerning the question of tin-bearing polymetallic ores or deliberate alloying comes from two bronze fragments found within the same grave in Gonur Depe (MA-133999 and -134001) (Figure 3A). They are identical in terms of lead and copper isotope systematics, and also have very similar chemical composition, but their tin isotope values differ considerably (0.032 ± 0.005 and 0.053 ± 0.004‰ u−1 δSncorr) (Supplementary Table S1). Even though copper from the same batch was apparently used, the tin (and tin content) was different. Accordingly, the objects were not produced from a common bronze melt, but are the products of several production processes, in which copper was alloyed with tin. A polymetallic ore base with tin is thus implausible, even if the observation does not allow a general statement.
3.1.2 Bronzes of the Late Bronze Age I
The sample set of the second chronological horizon—the LBA I—comprises ten bronze artefacts from Sapalli Tepe and nine from Dzharkutan. Some of the samples were heavily corroded so that statements on their chemistry are again limited. The uncorroded bronzes from Sapalli Tepe have tin contents between 3 and 9%, while a single corroded object has a similar concentration at the upper end of 8% tin. Further corroded items from Sapalli Tepe show lower values of tin of around 1% (Supplementary Table S1). Among the uncorroded artefacts from Dzharkutan, also pieces with only 1 or 2% tin exist; others reach tin contents of up to 9%. The same range is observed for the corroded items from the site, but due to weathering, a bowl (FG-850010/-186) is enriched in tin by up to 16% (Supplementary Table S1).
The main impurity elements of the bronzes from both sites are arsenic and lead reaching concentrations of up to 3 and 2%, whereby arsenic contents of most of the artefacts range between 1 and 3%; lead contents are mainly below 1% (Figures 6D–F; Supplementary Table S1). In addition, the bronzes contain nickel around 0.1–0.2%, silver and antimony below 0.1%, and selenium and bismuth concentrations mainly below or slightly above the detection limit of 0.005% of the EDXRF. An exception is an arrow head from Dzharkutan (FG-850145), which stands out for having much lower nickel (<0.01%) and arsenic (0.3%), and higher antimony concentrations (0.9%) (Figures 6D–F; Supplementary Table S1). Nevertheless, with these results, the analysed objects from the LBA I are chemically comparable to the MBA copper-tin alloys from Gonur Depe and Gelot (Group 1), which points to the use of the same polymetallic ore sources.
This view is confirmed by the isotope data. As with the matching trace elements, the majority of the LBA I bronzes show large similarities with the bronzes from the MBA in their lead isotope ratios (Figures 7C, D). Nine artefacts from Sapalli Tepe and Dzharkutan cluster in a narrow range between 18.40 and 18.55 206Pb/204Pb, 15.64 and 15.68 207Pb/204Pb and 38.52 and 38.80 208Pb/204Pb, which have been attributed to polymetallic deposits in Iran and/or Afghanistan in the case of Gonur Depe and Gelot. This conclusion might also apply to the nine objects in question, considering the similar trace elemental characteristics.
A bronze pin from Sapalli Tepe (FG-850035) shares the lead isotope ratios and trace element pattern with the polymetallic ores from Deh Hosein (Figures 6D–F; Figures 7C, D), so that the metal most likely derives from ores of this mine. Several bronzes from Dzharkutan (FG-850010/-186, -147, -163) are close to the isotope ratios of Deh Hosein (Supplementary Table S1). However, the match of these bronzes is not as good as for the pin, so that an alternative origin of the copper from ores in the Anarak district in Iran might be considered (Figure 1B; Figures 7C, D). The ore deposits from there are also in good isotopic correspondence with a mirror and three bracelets from Sapalli Tepe (FG-850011, -12, -18, -31), and the ores from the Nakhlak mine currently agree at best. However, as mentioned above, Nakhlak is an ancient lead-silver mine, and the objects are low in lead (Supplementary Table S1). So, again, the complex copper ores of the nearby Bagh Ghorogh deposit, or mineralisation in Afghanistan are the more likely suppliers of the copper for these objects (Pernicka et al., 2011; Nezafati et al., 2021). If this is true, one has to consider alloying with tin, since Bagh Ghorogh is not tin-bearing. These are important findings as they attest to the continued procurement of ores from Deh Hosein and Anarak in the early 2nd millennium BCE and a close relationship of the BMAC to the cultures in Iran.
In contrast, it is currently not possible to estimate the origin of the copper for four bronzes from Dzharkutan (FG-850130, -114, -145, -285) and further three from Sapalli Tepe (FG-850027, -30, -44), which lie outside the specified isotopic range. There is no convincing overlap with available ore data of the region. This conclusion applies to another three bronzes with less radiogenic lead isotope compositions (Figures 7C, D). Two of them—a pin and a vessel from Sapalli Tepe (FG-850032, MA-125160)—cluster close to the isotopic field of Mushiston, but given their very positive tin isotope composition (0.087 and 0.091‰ u−1 δSncorr) and deviating trace element concentrations (Figures 6D–F; Figure 8A), the metal cannot come from there.
A bracelet, a mirror and a pin from Sapalli Tepe (FG-850011, -30, -35) merit special consideration, as their association in the same burial (grave 82) exemplifies the diversity of raw materials used at Sapalli Tepe. All three items were made from copper of various sources (one likely from Deh Hosein, one from Anarak, one unspecified) indicated by differing lead and copper isotope compositions (Figures 7C, D; 8A; Supplementary Table S1). On the other hand, tin from the same deposit was likely used for two of them (FG-850011, -35) according to very similar δSncorr values (0.097 and 0.103‰ u−1). However, the lower δSncorr of the third object (0.080‰ u−1) could indicate the same source as all values are well within the range of a single deposit or mineralisation, which also applies to other objects from Sapalli Tepe and few from Dzharkutan. While resorting to a variety of polymetallic copper sources dominated by primary copper sulphides like chalcopyrite (this is inferred from δ65Cu near 0), the people of the LBA I BMAC obviously had access to a limited number of tin deposits as shown by the low variation of the tin isotope composition (compared to that of the MBA) and the predominance of values between 0.075 and 0.115‰ u−1 δSncorr (Figure 9). Defining the region that supplied the tin of the LBA I bronzes is hardly possible. From the tin deposits analysed in the present and a previous study (Berger et al., 2022a), only those from the Tajik Pamir Mountains exhibit a similar range (Figure 9; Supplementary Table S2). Cassiterite from eastern Afghanistan appears to be systematically enriched in light tin isotopes having lower isotopic values, while the few samples from the Uzbek deposit at Lapas are isotopically very heavy. The remaining cassiterite samples from Kazakhstan and Kyrgyzstan are rather diverse and statistically not useful at present, even though there are a few that exhibit comparable isotopic signatures as the metal objects (Figure 9; Supplementary Table S2). Together with the origin of the tin, the lapis lazuli deposits in the eastern Pamir Mountains may be of some significance (Thomalsky et al., 2013; Steiniger, 2019; Steiniger and Junker, 2020), as they can be considered a likely source of the numerous artefacts from Sapalli Tepe and Dzharkutan made of lapis lazuli (Chorievna, 2021). If the highly coveted material of the BMAC originated from there, an origin of the tin from the same region might be similarly likely.
3.1.3 Bronzes of the Late Bronze Age II
The third chronological horizon of the BMAC is represented by twenty-one Bactrian bronze artefacts from Dzharkutan (9), Molali (4), Tandyryul (3), Saridzhar (3), and Kangurttut (2), half of which were again completely corroded (Supplementary Table S1). The tin content of the well-preserved bronzes from the different sites as well as most of the corroded ones from Dzharkutan vary between 3 and 8%, while the content rises to 44% in the weathered metal beads from Tandyryul, Molali and Dzharkutan (Supplementary Table S1). One can of course only speculate about the original composition of the corroded objects from the latter three places, but compared with weathered bronzes from the MBA and the LBA I, the higher tin content in the corrosion is conspicuous and could indicate a once higher tin content of the fresh bronze. If true, this observation could relate to a change in the availability of the metal bases and probably in the mode of bronze production.
The general differences in the trace element pattern with that of objects of the earlier periods support this impression. The bronzes from Molali, Tandyryul and Kangurttut still contain appreciable amounts of arsenic (up to 3%) and lead (up to 4%), as did bronzes of the preceding chronological horizons (Figures 6G–I; Supplementary Table S1), but show an increase in the concentrations of antimony, silver, and bismuth, and a drop in nickel. Antimony is now often present in concentrations in the percentage range; silver and bismuth have concentrations one to two orders of magnitude higher than earlier bronzes (Figures 6G–I). Nickel for its part is often below the limit of detection of the EDXRF (<0.01%). The bronze bead from Dzharkutan with its high tin content (FG-850108) follows this pattern, whereas the effect on the trace elements in the remaining bronzes from Dzharkutan appears to be less pronounced. Direct comparison with the older artefacts from the site is limited due to corrosion, but shows the concentrations of the named elements to be only slightly enriched (Ag, Sb, Bi) or depleted (Ni). The same is observed for the razor blade and the awl from Saridzhar, while the dagger is in line with the elemental pattern of Molali, Tandyryul, and Kangurttut. Overall, the chemical composition of the LBA II bronzes thus seems to reflect a fundamental change in the material bases for bronze production. However, polymetallic ore deposits still supplied the bulk of the metal. Given the good match in the trace elements (Figures 6G–I), one of these bases could have been the copper-tin ores from Mushiston, which not only should give a “natural bronze” when smelted, but introduce all trace elements actually present in half of the bronzes of the sample set (Cierny, 1995; Alimov et al., 1998; Cierny and Weisgerber, 2003; Berger et al., 2022a).
The isotopic composition of the artefacts is in line with this conclusion. Several of the LBA II bronzes have lead isotope ratios consistent with those of Mushiston or close to it (Figures 7E, F), clustering in a range of 18.20 and 18.32 206Pb/204Pb, 15.64 and 15.69 207Pb/204Pb and 38.29 and 38.47 208Pb/204Pb. Agreement is also observed for the tin and copper isotope systematics, and the consistently negative δSn of the Mushiston ores serves as a useful discriminating fingerprint (Figure 8A). Tin ores and cassiterite of other tin mineralisation often have higher and more positive δSn values (Supplementary Table S2) (Berger et al., 2019b; Mason et al., 2020). The only exception is the cassiterite from Askaraly in the Altai Mountains in eastern Kazakhstan as well as the pegmatitic cassiterites of the Afghan Hindukush, which stand out due to similar or even extremely negative tin isotope compositions (Figure 9). There is to date no indication that the Afghan tin sources were exploited in the Bronze Age, but at Askaraly, prehistoric mining was documented (Weisgerber, 2004; Stöllner et al., 2011; Garner, 2013; Stöllner et al., 2013; Thomalsky et al., 2013; Garner, 2015). However, because of the match of all four analytical proxies (Pb, Sn, Cu isotopes, chemistry) between artefacts and the Mushiston ores, there is currently no reason to prefer a Kazakh origin of the tin over Mushiston based on one ore sample alone. Thus, there remains little doubt that part of the LBA II copper and tin of the BMAC originate from the polymetallic ores of the Mushiston mine in the form of bronze. This finding is of tremendous significance because such clear determination of the origin of metal was rarely possible before, and certainly not with tin.
Nevertheless, sources other than Mushiston were still in use or became available between 1750 and 1450 BCE. This is most clearly indicated by two bronzes from Saridzhar, and one each from Kangurttut and Dzharkutan (Figures 7E, F). The lead isotope ratios and trace elements of the Saridzhar awl (MA-125166) suggest polymetallic copper ores from the Anarak province in Iran, of which those from the Bagh Ghorogh mine show the best match. Strikingly, this composition is almost identical with the bronze bead from Kangurttut (MA-124884) and goes along with very similar copper isotope values (δ65Cu = 0.25 and 0.28‰) and chemical compositions (Figures 6, 7). From this, one can infer a common copper origin for both objects, not least because the sites are located close to each other (Figure 1A). For its part, the data of the razor blade from Saridzhar (MA-125165) probably leads to chalcopyrite ores of the Nezam Abad deposit in the Iranian Astaneh-Sarband area. This is not only indicated by the lead isotope ratios, but also by the absence of practically all trace elements, typical for this compound, and the δ65Cu near zero (Figures 6G–I; Supplementary Table S1). The lead isotope composition of the spearhead (FG-850168) in turn is consistent with the Deh Hosein mine. Thus, all these artefacts still follow the metallurgical traditions of the preceding LBA I. The reasons for this observation can be twofold. One possibility is that raw material from these source regions continued to reach the Central Asian markets during the LBA II. This, however, at a time when contacts are no longer documented archaeologically. Alternatively, it might reflect a widespread practice of recycling old metal, either by re-melting it or by systematically plundering older LBA I burials with their rich furnishings (Kaniuth, 2006; Teufer, 2015).
On the other hand, a few bronzes from Molali (MA-125174), Tandyryul (MA-124806) and Dzharkutan (FG-850108) hint at additional sources. Their lead isotope composition shows a tendency towards ratios of mineral deposits in the southern Tian Shan, probably the Nuratau Mountains (Figure 1A), even though their chemical and stable isotope composition is in line with Mushiston, just like the other artefacts from these sites (Figures 6G–I, 8A). Since the objects seem to form some kind of mixing line between Mushiston and locations of the Nuratau region, mixing of metal batches from several sources could explain the variations in the lead isotope ratios. Similarly, the dagger from Saridzhar (MA-125164) with Mushiston-compatible copper isotope and chemical composition (no tin isotopes are available), was probably produced by mixing Mushiston metal with metal that may have come from ores in the central part of the Tien Shan Mountain range. Its lead isotope composition is clearly shifted to less radiogenic ratios (Figures 7E, F). If all these conclusions were true, there seems to exist evidence to suggest that, besides Mushiston, other ore deposits from the Andronovo territory were supplying the BMAC metal industry in the LBA II.
Three bronze beads made with Mushiston ores are additionally revealing. Two of them were part of a wristband and lay in a child burial at Molali (MA-130261, -272), while the third (MA-124809) originates from a grave context at Tandyryul (Figures 3C, D). Although the objects were found at different locations, they exhibit identical lead and stable isotope compositions (δSncorr = –0.049, –0.052 and –0.053‰ u−1, δ65Cu = 0.25 and 0.26‰). In addition, their chemical compositions are very similar, too, given the high degree of degradation by weathering (Figures 6–8). Such a strong match in the data cannot be a coincidence. It can only be explained by the use of the same sources or batches of metal and the mass production in a single workshop, which supplied goods to different consumers. In turn, a third bead from the Molali grave (MA-130274) and another one from a burial in the Tandyryul cemetery (MA-124808) are slightly or significantly different in the isotopic and the chemical composition, which indicates their production either from other ore/bronze batches or at different times by another craftsman, or even in a different workshop. The same applies to the bead from Dzharkutan (FG-850108), whose lead isotope composition deviates clearly. Nevertheless, a certain preference of Mushiston metal for making bronze beads is evident.
Interestingly, four bronzes from Dzharkutan (FG-850119, -183, -193, MA-125149) also exhibit lead isotope compositions very close to Mushiston (Figures 7E, F). However, their chemical pattern is not fully consistent with the ores from the mine, even though different from that of the LBA I bronzes from the site (Figure 6). Because no stable isotope data is available for these artefacts, a relationship with Mushiston can currently neither be established nor ruled out. At least, a contribution of its ores to these bronzes to a certain degree cannot principally be refuted. If no further data can be produced it will ultimately be impossible to identify other components when mixing is a likely scenario, just as it was considered for other LBA II bronzes (see above).
Limitations also exist for the origin of the tin of several bronzes that cannot be related to the Mushiston ores. The tin isotope values of the bronzes from Saridzhar (MA-125166) and Kangurttut (MA-124798, - 884) are lower than those of the LBA I bronzes (Figure 8A), but as comparable values are observed for different regions with tin deposits, the tin isotope data is not diagnostic enough to conclude on specific source regions (Figure 9). This situation might become even more complicated if reuse or recycling scenarios are considered. On the other hand, the δSncorr of 0.100‰ u−1 of the razor blade from Saridzhar is very similar to several LBA I bronzes from Sapalli Tepe and Dzharkutan and thus seems to reflect the tradition of the early phase of the LBA as was already concluded from the matching lead isotope ratios with Iranian deposits (Figure 8A). Nevertheless, the general shift of the tin isotope values in the majority of the bronzes in the LBA II towards lighter compositions is striking and clearly testifies to a change in tin procurement.
3.2 Andronovo bronzes
3.2.1 Bronze of early Andronovo
The only artefact of the early horizon from the Andronovo territory in our sample set is a ceremonial axe from Chimbaylyk (FG-850102), located at the Chirchik River and the foothills of the Chatkal Mountains in Uzbekistan (Figure 1A). It contains 9% tin, but as the axe is completely corroded, this value will not reflect the original tin concentration. Nevertheless, the EDXRF results reveal very pure copper with cobalt (0.01%), silver (0.007%), and lead (0.05%) as the only trace elements in the detectable range (Figures 10A–C; Supplementary Table S1). This finding points to a production of the object from a pure malachite ore, for which the high copper isotope value of 0.50‰ (Figure 8B) is an additional clue (Klein et al., 2009; Jansen et al., 2018). Its lead isotope composition is fully consistent with mineral deposits in the southern Tian Shan and clearly different from the available ore data from Iran and Afghanistan (Figures 11A, B). Narrowing down the origin of the copper further is, however, difficult. Most copper mineralisation in the Tien Shan overlap significantly, and there is too little data of specific copper deposits so far (Kraus et al., Forthcoming). Yet, from the isotopic composition, a provenance of the copper from the proximate Karamazar or Kurama Mountains is not unlikely (Figure 1A). The tin isotope composition (δSncorr = –0.001‰ u−1) does not allow any conclusion to be drawn about a specific tin deposit either, but the use of ores from Mushiston and other polymetallic deposits can be excluded with regard to the very pure copper (Figures 10A–C).
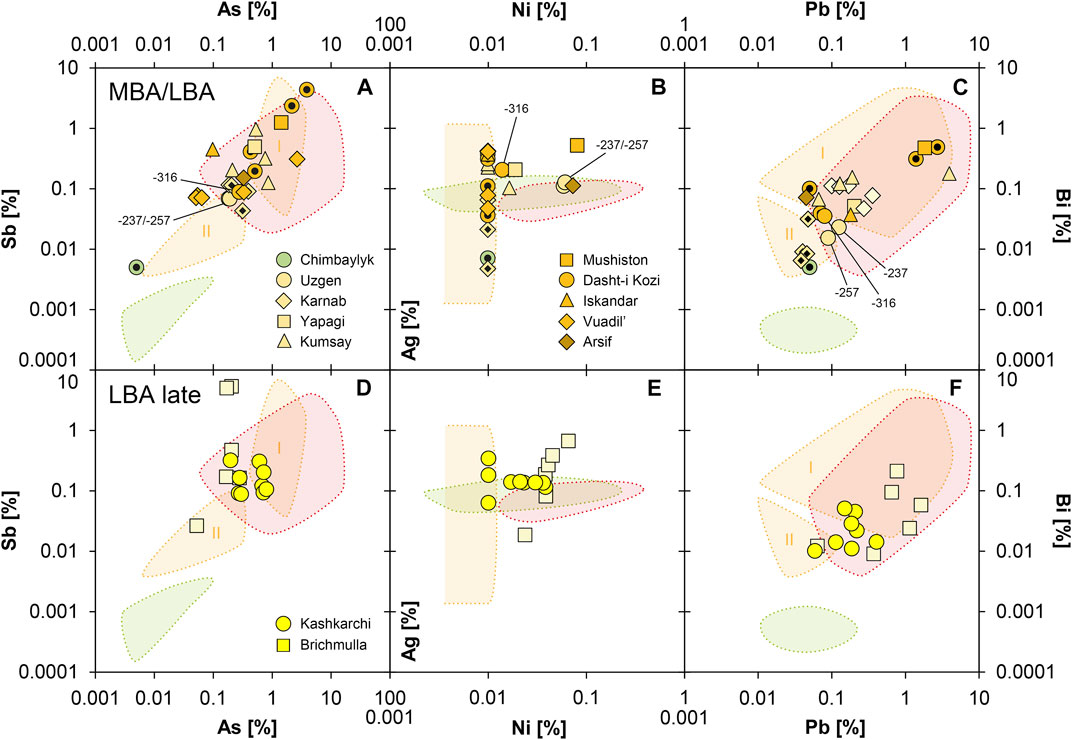
FIGURE 10. Chemical composition of Andronovo bronzes compared to potential copper ore districts in Central Asia and Iran. MBA/LBA early (A–C), LBA late (D–F) Chemical data of ores have been normalised to their copper and tin contents for being comparable with artefacts and are illustrated as polygons for better legibility of the charts. The orange polygons belong to Mushiston (I—Sn-rich ores; II—Cu-rich ores), the red to Deh Hosein mine and the green to Veshnaveh mine. Symbols with black dots are corroded objects; specified numbers correspond to the last three figures of the laboratory no. in Table 2 (diagrams and artefact data: J. Lutz, D. Berger; ore data: from Nezafati, 2006; Nezafati and Stöllner, 2017; Berger et al., 2022a).
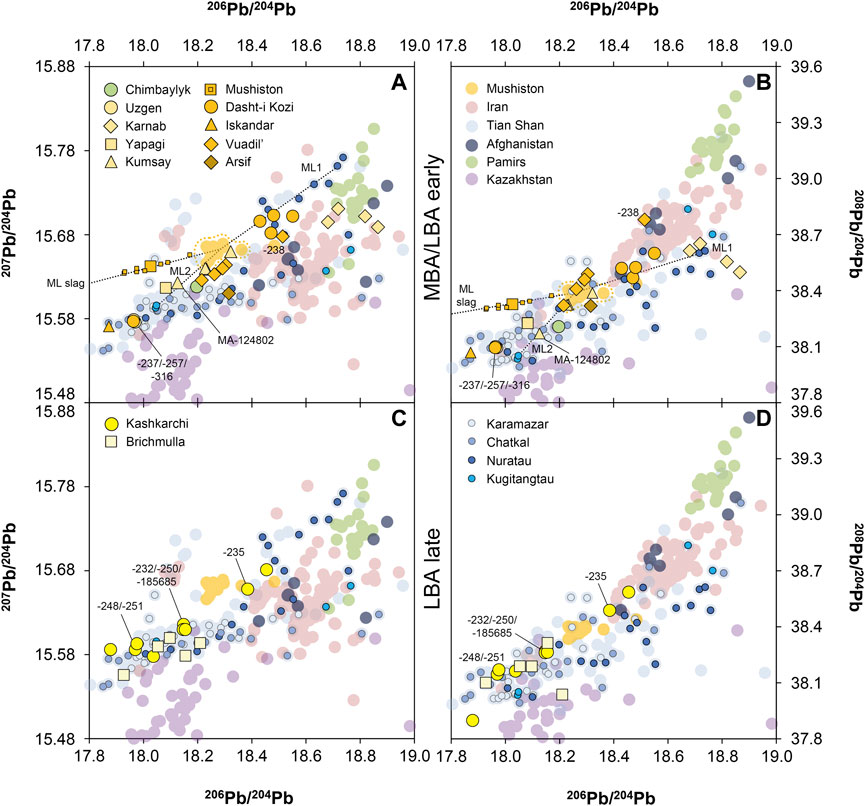
FIGURE 11. Lead isotope ratios of Andronovo bronzes compared to copper ores in Central Asia, Iran and Afghanistan. MBA/LBA early (A–B), LBA late (C–D). Also shown are potential mixing lines of Mushiston ores with those from other ore deposits: ML1—ores from Nuratau Mountains, ML2—ores from Kugitangtau Mountains, ML slag—ores from unknown deposit based on single bronze prills (small orange squares) from the Mushiston slag (MA-171261). Specified numbers correspond to the last three or six figures of the laboratory no. in Table 2 (diagrams: D. Berger, artefact data: B. Höppner; ore data: from Brill et al., 1997; Chiaradia et al., 2006; Nezafati, 2006; Begemann and Schmitt-Strecker, 2009; Nezafati et al., 2009; Pavlova and Borisenko, 2009; Shafiei, 2010; Pernicka et al., 2011; Thomalsky et al., 2013; Merkel, 2016; Chernyshev et al., 2017; Kraus et al., Forthcoming; Nezafati and Stöllner, 2017; Wong et al., 2017; Cao et al., 2018; Berger et al., 2022a).
3.2.2 Bronzes from early Late Bronze Age Andronovo
3.2.2.1 Karnab
Three chemical groups are discernible among the 26 bronzes of this set: One group with a low amounts of impurities (less than 0.5% in total, not counting iron and tin), a second group with medium amounts of traces (0.5–1.5%) and a high impurity group (6–12%) (Figures 10A–C; Supplementary Table S1). Eight samples were corroded. This applies for instance to four artefacts from Karnab-Sichkonchi (Figure 5B, Supplementary Table S1). The heavily weathered bronzes with 3–10% tin and rather pure copper are especially important, since Karnab is a miner’s settlement located just 1–2 km from the prehistoric tin mines belonging to the Zirabulak Mountains group (Figure 1A). An origin of the tin from the immediate surroundings thus stands to reason, not least because one of them seems to be production waste in the form of a casting drop (FG-850289). Unfortunately, no tin isotope data could be obtained for this object due to preparative and analytical reasons, but for an ingot (FG-850288), a high δSncorr value of 0.152‰ u−1 was determined (Figure 8B). This value is actually among the most positive tin isotope compositions of all analysed bronzes in the study. Almost identical isotopic compositions were only observed in the MBA bronzes from Gonur Depe, for which an origin from the deposits in the Zirabulak Mountains was already considered. The data of the Karnab ingot further substantiates this assumption as cassiterite ores from the nearby tin mine of Lapas have comparably high δSn values, which are otherwise rare among tin mineralisation in Central Asia (Figure 9). However, there is no data yet for the Karnab tin mine and only a few analyses overall from the Zirabulak region. As similar high δSn values exist in the ores of the Pamirs, a nearby origin of the tin is not certain. In addition, two bronze fragments from Karnab have other tin isotope compositions, one of which (δSncorr value of 0.123‰ u−1) was found to prevail in LBA I finds of the BMAC from Sapalli Tepe and Dzharkutan (Figure 8). This finding could be indicative of the use of the same tin deposits by the Andronovo Culture and the BMAC.
Statements about copper provenance are similarly uncertain. The Karnab finds are chemically very similar and exhibit comparable lead isotope signatures (Figures 10, 11), so that the use of the same ores can be inferred. However, both points of evidence do not permit definite conclusions concerning the origin of copper, but at present, sources in the Tian Shan (e.g. Nuratau Mountains) appear more likely than mineralisation in Iran and Afghanistan.
3.2.2.2 Kumsay, Dasht-i Kozi and Yapagi
Four artefacts from the Kumsay cemetery with 4–8% tin (Figure 1A) are also of particular interest, as the chemical and isotopic composition of three of them (MA-124803–05) are largely consistent with the Mushiston deposit (Figures 8, 10). In addition, the material is of clear Andronovo style, but the burial traditions of the site and the settlement are BMAC-related. Therefore, Kumsay is an important place documenting the cultural entanglements between both traditions, which is reflected best by two Kumsay beads and their BMAC counterparts from Molali, Tandyryul and Dzharkutan. All these jewellery pieces share the same metal base, namely the Mushiston ores. This is also true for several artefacts from Dasht-i Kozi, which is important due to its proximity to the Mushiston mine (10 km distance). All objects are corroded; nevertheless, two bronze beads (FG-850299, -302) with high tin content (up to 48% Sn) are again clearly made from the characteristic high-impurity metal of Mushiston (Figures 10A–C). They not only show the typical chemical signature with high arsenic, antimony, silver, lead and bismuth concentrations but also the deposit’s typical stable isotope composition. In addition, they are very similar to the beads mentioned above (Figures 6–8). However, what both objects from Dasht-i Kozi also share with some of the BMAC bronzes is their shift towards more radiogenic lead isotope ratios. Neither the two beads nor the other artefacts from Dasht-i Kozi are consistent with Mushiston (Figures 11A, B). This result is again surprising. All parameters but the lead isotope ratios point to the Mushiston mine, at least in case of the beads. One possible explanation could be mixing with lead-rich copper from another source. This option was considered for the BMAC bronzes, and the objects from Dasht-i Kozi strikingly align with the finds from Molali, Tandyryul and Dzharkutan on the presumed mixing line between Mushiston and the Nuratau Mountains (Figures 11A, B, ML1). Thus, mixing with Nuratau ores (or others from the Tian Shan) could be a common explanation for both the Andronovo and the BMAC bronzes. The knife from Kumsay (MA-124802), for its part, could have been made by mixing Mushiston ores with malachite from the Kugitangtau Mountains near the site (Figure 1A). The sample lies on a hypothetical mixing line between the oxidised and chemically rather pure ores of the latter region (with elevated lead contents) and the chemically complex ones from Mushiston (Figures 11A, B, ML2). The very positive δ65Cu of 1.05 ± 0.01‰ of the bronze may indeed indicate smelting of an oxidised copper ore (e. g. malachite), but to alter the lead isotopic signature of Mushiston on mixing, this must have contained much lead. Analyses of the Mushiston ores revealed high lead concentrations (see Figure 10), especially in the tin-rich secondary assemblages (Berger et al., 2022a). Hence, if the bulk of the Kumsay knife should in fact derive from Mushiston, as the negative δSncorr value (–0.019‰ u−1) and the trace element pattern suggest, then the potential second component must have been lead-rich. This would be the case for malachite from the Kugitangtau (Kraus et al., Forthcoming), and the high lead content of the bronze of 4% would support this hypothesis.
However, recent results from the investigation of a unique bronze slag offer an alternative explanation. This slag (Figure 3F) was found very close to the old workings of Mushiston, and although compatible with the deposit in all parameters, the lead isotope composition of the extracted bronze prills does also not agree with the nearby ores. They rather establish a mixing line between Mushiston and an unknown source as the second endmember of mixing (Figures 8B, 10, 11, ML slag) (Berger et al., 2022a). Based on the slag’s high iron content and its mineralogical inhomogeneities, fluxing with lead-rich iron ores during the smelting process appears the most likely reason for the different lead isotope composition. Given such a smelting scenario, a similar explanation is conceivable for the Dasht-i Kozi finds and comparable bronzes such as those from Molali, Tandyryul, Dzharkutan and an Andronovo bead from Yapagi (MA-185719). The latter contains 5% tin (just as the slag) and its impurity pattern as well as its stable isotope composition can be paralleled with the slag and Mushiston (Figures 8B, 10A–C). Conspicuously, its lead isotope composition mismatches Mushiston again (Figures 11A, B). If fluxing were indeed an option, in this case just like in case of the Dasht-i Kozi finds, one would have to assume other origins for the flux than for the smelting process related to the Mushiston slag because the lead isotope ratios of the bronzes are different. Admittedly, there is only weak evidence for this theory at present, and one has to bear in mind that other polymetallic deposits with comparable characteristics could exist (Sverchkov, 2010; Garner, 2021). However, if applicable, the observations would provide important insights into the metallurgical techniques of the Andronovo Culture and its relationship with bronzes of the BMAC.
3.2.2.3 Vuadil’
A somewhat different picture emerges for five bronze beads from a burial at Vuadil’. Three of them were part of a wristband (FG-850222, -228, -230) and were certainly the products of a common chaîne opératoire due to a consistent tin concentration of ca. 3.5% and identical chemical and stable isotope systematics (Figure 8B, 10D–F). Only the slightly varying lead isotope composition seems to contradict this interpretation. The lead isotope ratios themselves are inconclusive in terms of copper provenance, since they show no convincing match with a distinct ore deposit (Figures 11C–D). The proximity of the ratios to those of Mushiston is evident, but a deficiency in uranogenic lead (207Pb) makes a direct relationship with ores from there unlikely. In addition, the consistent high δ65Cu of 0.62–0.64‰ and the likewise uniform but extreme negative δSncorr of –0.22‰ u−1 are clues against the Mushiston ores. From the high δ65Cu, an oxidised ore base such as malachite may be deduced (supported by the low impurity pattern), while the very negative δSncorr points to tin sources in the Hindukush. From nowhere else than Afghanistan are tin ores with such negative compositions known (Figure 9). If this is true, it would be the first indirect evidence for the use of Afghan tin ores.
On the other hand, the remaining two bronze beads from Vuadil’ with 5.4 and 7.2% tin, respectively, are in tune with Mushiston concerning stable isotope compositions. One of them (FG-850238) additionally has matching chemical characteristics, even regarding the 0.16% of zinc (Figures 8B, 10D–F). Unfortunately, it is exactly the bead that has the least compatible lead isotope composition with the Tajik deposit (Figures 11C–D). However, if the Mushiston ores were smelted using extraneous fluxes, as indicated by the Mushiston slag (see above), or the metal was mixed in any way with metal from another source, then the result could probably be explained. The same would apply for the fifth bead from the site (FG-850259).
3.2.2.4 Uzgen
A different origin of the copper is inferred for the pair of horned bracelets from Uzgen having medium amounts of trace elements (Supplementary Table S1). Their lead isotope ratios are almost identical and agree well with copper from the Chatkal Mountains (cf. Figure 1A), whereas the tin isotope values of 0.050 and 0.052‰ u−1 δSncorr are rather unspecific. Comparable values are known from the Mardjanai ore cluster in the southern Pamirs, but also from Kazakhstan and Kyrgyzstan as well as the Hindukush (Figure 9; Supplementary Table S2). However, Afghan cassiterites have mainly lower values and are therefore statistically less likely. Regardless of the origin of the tin, one must assume the same cassiterite or even tin batch for the bracelets because of their very similar δSncorr (Figure 8B; Supplementary Table S1). Moreover, due to their identical δ65Cu of –0.21 to –0.22‰ and chemical composition, a production of the bracelets in the same workshop within a short time span is almost certain, underlined by typological similarities (Figure 5D). Interestingly, a typologically close bracelet excavated at Dasht-i Kozi (FG-850316) (Figure 5F) was most likely also produced in this workshop. It resembles the Uzgen finds in all analytical proxies (only slight deviations in the chemical composition), which cannot be coincidental (Figure 8B; Figures 10, 11). As with the bronze beads of the study, this finding allows important conclusions about the organisation of metallurgy of the Andronovo Culture, as it shows that workshops did not only meet a regional demand, but also produced for wider distribution. Between Dasht-i Kozi in the Zeravshan valley and Uzgen in the eastern Ferghana valley (Figure 1A), a distance of 500 km had to be bridged, either directly or through down-the-line exchange.
3.2.2.5 Iskandar and Arsif
Even though the bronze bracelet from Iskandar (FG-850105) has the same style as the items from Uzgen (Figure 5E), it was most likely not produced in the workshop considered above. The lead isotope signature could also document the use of copper deposits in the Chatkal Mountains, where LBA mining was considered possible for example at Aktashkan (Garner, 2021), but it must originate from another mineralisation or at least another zone of mineralisation within the same deposit. The copper ore used to produce this bracelet was probably oxide-dominated due to the high δ65Cu value of 0.62‰, while the copper of the Uzgen bracelets with slightly negative δ65Cu derives from primary copper sulphides (e.g. chalcopyrite). In addition, the tin isotope value is negative at –0.025‰ u−1 δSncorr and points to Mushiston (Figure 8B). Yet, a clear link with the latter is difficult to establish for Iskandar. Not only do the lead and copper isotope compositions differ, but also the trace element pattern with low arsenic could be a diluted Mushiston signature at best. Metal mixing—e.g. of tin metal from Mushiston after smelting tin-rich ores (cf. Berger et al., 2022a)—with copper from the Chatkal Mountains is possible, but this cannot be verified. That is why an alternative origin of the tin has to be considered, probably from other, smaller tin-bearing polymetallic mineralisation, which are numerous in the region (Sverchkov, 2010; Garner, 2021).
For their part, the chemical and isotopic signatures of the mirror from Arsif are difficult to interpret with reference to ore origin. The tin isotope composition of the find (0.060‰ u−1 δSncorr) is within the same range as the bracelets from Uzgen and Dasht-i Kozi, and the copper certainly originates from deposits in the Tian Shan (Figures 10A–C; 11A, B). However, narrowing down the metal sources more accurately is not possible without tangible point of contacts to other Andronovo artefacts.
3.2.3 Bronzes from the developed Late Bronze Age Andronovo
3.2.3.1 Brichmulla
Covering the time from 1550 to 1300 BCE, fifteen well-preserved bronzes from two sites were analysed from the developed phase of LBA Andronovo. Six of them derive from a hoard at Brichmulla, which contained three decorative buttons (only two analysed) and four arrowheads (Figure 1A; Figure 5J). Even though very similar in shape and style, each of the arrowheads was cast from a separate batch of metal. This is demonstrated by the varying tin contents from 6 to 16%, but also by the variable trace element and lead isotope compositions (Figures 10D–F, 11C, D). One of these objects (FG-850081) has a—by one to two orders of magnitude—higher antimony concentration of 5.4% than other bronzes in the hoard, indicating that the parental copper ore contained appreciable amounts of antimony, e.g. from fahlore minerals such as tetrahedrite. According to Sverchkov (2010), fahlores are known from Koksu in the Chatkal Mountains, not far away from Brichmulla. The same conclusion will apply to one of the buttons (FG-850079) with a comparable antimony concentration (5% Sb). Since the object is also quite similar in the remaining trace elements and the lead isotope ratios, the craftsman most likely accessed the same copper in both cases (Supplementary Table S1). Nevertheless, the button was again produced with an individual batch of metal just like the second decorative button (FG-850078).
Despite the heterogeneous chemical and lead isotope data of the Brichmulla bronzes, their homogenous stable isotope composition with 0.001–0.012‰ u−1 δSncorr and –0.10 to –0.06‰ δ65Cu must be stressed (Figure 8B; Supplementary Table S1). These results suggest tin and copper from the same source and copper most likely originating from the same mineral species. As stated above, δ65Cu values slightly below 0 are typical for primary copper sulphides like chalcopyrite or tetrahedrite (Markl et al., 2006; Jansen et al., 2018). In contrast, from the diversity of the lead isotope ratios of the bronzes, one would rather infer different origins of the copper. This is possible; nevertheless, the homogenous stable isotope values along with the comparable impurity patterns could also indicate the use of copper from a common source or deposit, which has variable lead isotope compositions, or the intentional addition of lead or a lead-bearing component (cf. the elevated lead contents). Due to an insufficient database of copper ores, this can currently not be verified. At least, ores from the Tian Shan are very likely, but a contribution of Kazakh ores, especially for one of the buttons (FG-850078), cannot be excluded (Figures 11C, D).
3.2.3.2 Kashkarchi
The bronze beads, awls/pins, and the spatula from burials of the cemetery at Kashkarchi (Figure 5I) share a common impurity pattern with medium levels of trace elements (0.8–1.5%) and have tin concentrations between 3 and 8% (Figures 10D–F; Supplementary Table S1). Two chemical groups stand out with arsenic as the main distinguishing parameter: One group has elevated arsenic contents between 0.7 and 0.8%, while the second group has 0.2–0.3%. The other trace elements (Ag, Sb, Pb, Bi) are more evenly distributed across the objects. The grouping is not readily reflected in object typology, but three items (beads FG-850232 and -250, awl MA-185685) with higher arsenic contents show very similar lead isotope, stable isotope and chemical compositions, while a fourth one (FG-850235) differs significantly from these three only in its lead isotope ratios (Figures 8B, 11C, D; Supplementary Table S1). Thus, they were most likely produced with copper and tin from the same source and partly from the same ore batch. Because of incompatible lead isotope ratios, and because the stable isotope composition, in particular the more negative δSncorr values (–0.096 to –0.078‰ u−1), does not match well with Mushiston (Figures 8B, 11C, D), either another polymetallic deposit or copper and tin from different sources must be considered. For tin, Afghan cassiterites are again possible due to the low δSncorr values (Figure 9), but Askaraly in the distant Altai Mountains of Kazakhstan with comparably low tin isotope values (one sample!) could also be an option. More data is needed here.
A second cluster of five artefacts is also defined by uniform stable isotope compositions with δSncorr values from 0.013 to 0.040‰ u−1 and δ65Cu from –0.26 to –0.02‰ (Figure 8B). With the exception of one awl (FG-850273), all objects belong to the arsenic-poor chemical group, but their highly variable lead isotope ratios are conspicuous (Figures 11C, D). Two beads (FG-850248, -251) are identical in terms of the lead isotope ratios and could be products from primary sulphidic copper ores (due to δ65Cu slightly below 0) of a common location in the Tien Shan, while for the remaining three artefacts (FG-850247, -273, MA-185686) primary copper sulphides from other locations were likely used. However, as with the bronzes from Brichmulla, exact statements regarding copper provenance are impossible, because the lead isotope ratios either do not match specific ore deposits or were likely subject to mixing or recycling processes.
4 Synthesis and conclusion
This paper has presented results of the archaeometallurgical investigation of 91 bronzes from the Central Asian Bronze Age, providing the most comprehensive chemical and isotopic dataset to date from the region. In addition, copper and tin isotopes are combined in the study of Central Asian metal artefacts for the first time, which complement the more common analysis with lead isotopes and trace elements. In this way, we significantly expand knowledge about the metal procurement and metallurgical practices of the Bactria-Margiana Archaeological Complex (BMAC) and the Andronovo phenomenon, and shed light on their respective technological and economic relationships. In particular, a major advance was possible in the quest for ancient tin sources, while the knowledge about the provenance of copper is refined significantly.
Overall, the analysed bronzes encompass a time frame from the late 3rd to the second half of the 2nd millennium BCE, with the oldest artefacts coming from Gonur Depe and Gelot. These early MBA bronzes of the BMAC, as well as the slightly later ones from the LBA I from Sapalli Tepe and Dzharkutan, contain predominantly copper from polymetallic deposits in Iran. Deh Hosein in the Astaneh-Sarband area and Bagh Gorogh in the Anarak region are the most likely suppliers. However, a provenance of copper from Afghanistan is also possible for several objects due to the overlap of ore data with that of Iran. As such, the collected data supports the archaeological evidence. The material culture of the MBA and LBA I of the BMAC is strongly linked to the cultural traditions of Iran and Afghanistan, whereas no sustained relationships with the Andronovo complex in the north are noticeable in the material culture at this time. The copper travelled along the same pathways as the templates for the objects manufactured from it or the objects themselves. Evidence for metal production and working has been documented e.g. at Gonur Depe, Sapalli Tepe and Dzarkutan (Kraus, 2016; Kraus, 2021). The origin of the tin must probably be understood differently. Even though tin in low and medium concentrations (≤5%) might have been introduced occasionally to the metal from tin-bearing ores (e.g. from Deh Hosein) along with the copper, deliberate alloying with tin has to be assumed for the majority of the MBA and LBA I bronzes with higher tin concentrations and made from copper ore deposits without tin (e.g. Bagh Gorogh). The absence of noticeable quantities of bronzes in the late 3rd and the early 2nd millennium BCE in northern, central and eastern Iran (Stech and Pigott, 1986; Helwing, 2009), and the lack of discrete tin deposits in Iran speak against the transport of tin metal from the southwest. Instead, our data indicates that deposits in the Pamir Mountains and the Hindukush could have been exploited, while there is no clear evidence for any use of tin from mineralisation framing the Zeravshan valley (Mushiston, Karnab, Lapas). The frequent occurrence of lapis lazuli objects in the sites of Margiana and northern Bactria would be in line with this interpretation. Significant deposits of the precious stone are known in the Pamirs and the Hindukush (Steiniger, 2019) not far away from the tin mineralisation, so that a combined procurement is not unreasonable. Even though the same sources for tin can be reconstructed from the rather uniform tin isotope composition of the MBA and LBA I bronzes, the ultimate origin of the tin used in the early Central Asian bronzes cannot be considered solved conclusively. As long as archaeological evidence and additional isotopic data, including tin-bearing polymetallic copper deposits such as Deh Hosein, is missing, no clearer insights into the pathways of the earliest tin can be provided.
The situation has become more clear for the LBA II thanks to our investigations. An influx of copper from Iran or, alternatively, recycling of older copper alloy objects, is noticeable for a further 200–250 years, but the metal bases of the period changed fundamentally: Copper ores from the Tian Shan Mountain range now became available to the people of the BMAC and provided the raw material for the majority of objects. Specifically, the copper-tin deposit of Mushiston, situated close to the BMAC territory (Figure 1A), played a central role. Exploited exclusively by Andronovo miners (Garner, 2013; Garner, 2015; Garner, 2021), the oxidised ores from Mushiston yielded natural bronzes, whose tin concentrations could be more or less well controlled by selecting ore assemblages of specific colours (Berger et al., 2022a, Online Resource 5). The smelting of the ores is proven by a Bronze Age slag found close to the deposit (MA-171261) (Figure 3F), and the isotopic and chemical data collected in this study now additionally demonstrates that almost one third of the analysed bronze artefacts from 1750 to 1400 BCE was produced with metal from Mushiston. This applies to both the BMAC and Andronovo bronzes. Thus, we face a situation of shared mineral sources, which necessitated tight communication and interchange between the two cultural entities and which finds expression in culturally hybrid contexts such as the Kumsay or Tandyryul cemeteries. Both are significant results: Never before could the tin in metal artefacts be traced to its ore sources more precisely, and never before were the economic relations between the BMAC and Andronovo more tangible.
However, the procurement of tin and copper was certainly a more complex affair. On the one hand, there are clues that Andronovo metallurgists either mixed Mushiston metal with metal from other locations in the Tian Shan, or smelted Mushiston ores using fluxes. The resulting changes in the lead isotope signatures regularly prevent a proper reconstruction of metal provenance, even if other proxies (tin isotopes, copper isotopes, trace elements) clearly point to Mushiston. On the other hand, copper and tin from elsewhere were used for the production of bronzes discovered in southern Central Asia. For copper, sources in the Chatkal and Karamazar Mountains in northern Uzbekistan are an option, but also those in the Kugitangtau Mountains in the south of the country (Figure 1A). All these regions are known for their polymetallic mineralisations, which are occasionally tin-bearing (Sverchkov, 2010; Garner, 2021). According to the lead isotope signatures, it is conceivable that these polymetallic sources were used to produce copper and even bronze, but due to the lack of tin isotope data it can currently not be clarified whether these small deposits provide a realistic alternative for the origin of copper and tin. The situation is similarly uncertain for the important tin deposits of Karnab and Lapas in the Zirabulak Mountains or others further northeast in the Kazakh Altai Mountains (e.g. Askaraly). All these sources were exploited in the 2nd millennium BCE (Stöllner et al., 2011; Garner, 2013), but the sparse data available does not yet allow to draw any reliable conclusions about their significance for LBA metallurgy. To collect more isotopic and chemical data of these and other as yet uncharacterised mineralisation therefore remains a pressing task for future studies, which should go hand in hand with the analysis of potential copper ore deposits in Central Asia. There are still many regions with an insufficient ore database. Thanks to intensive research in the past and the ores’ continued availability at the site, the situation for Mushiston is much better. The deposit is well characterised in geological, archaeological, and archaeometric terms (Alimov et al., 1998; Garner, 2013; Berger et al., 2022a; Konopelko et al., 2022), which helps identifying the signatures of the ores in metal objects. At the current state of research, Mushiston was an important metal supplier for the region in the first half of the 2nd millennium to around 1400 BCE with an apogee between 1800 and 1500 BCE. After that, evidence for mining drops significantly (Garner, 2013, 234–235) and Mushiston ores were not used in later bronzes analysed so far. In addition, it is important here to point out that no traces of Mushiston bronzes have been identified from West Asia (Frank, Forthcoming; Pernicka and Frank, Forthcoming), and certainly not in the tin ingots from Uluburun (cf. Powell et al., 2022; Berger et al., 2023). Therefore, it seems that the deposit was a significant source of copper and tin only on a regional scale. Further analyses of copper-tin alloys are required to quantify the extent to which Andronovo populations have exploited Mushiston, and to understand if Mushiston bronze was traded to more easterly locations in Asia. Further investigations should also help to understand whether Andronovo people potentially accessed tin deposits in Afghanistan or Kazakhstan. First indications for cassiterite likely originating from the Hindukush are presented in this study (cf. the extremely low tin isotope values for Vuadil’). Overall, the 2nd millennium was a period of dynamic change and intensified interregional interaction in the southern parts of Central Asia. Only now does the region turn into the hub of cultures of Silk Road fame. Metallurgy provides one of the clearest examples for these processes, and the quest for natural resources may have been one of its driving forces.
Data availability statement
The original contributions presented in the study are included in the article/Supplementary Material, further inquiries can be directed to the corresponding authors.
Author contributions
Conceptualisation: DB Methodology: DB, GB, JL Investigation: DB, GB, JL, AW, KK, SK Visualisation: DB, SK Supervision: DB, EP Writing—original draft: DB, KK, NB Writing—review and editing: DB, NB, KK, GB, SK, JL, MT, AW, EP. All authors contributed to the article and approved the submitted version.
Funding
Funding for this research was received from an Advanced Grant of the European Research Council (Grant No. 323861) awarded to EP, from the project “ROXIANA” of the Agence Nationale de la Recherche and the Deutsche Forschungsgemeinschaft to H.-P. Francfort and NB (Project no. 11-FRAL-016-ROXIANA-01/BO 379371-1), from RESAF of the Deutsche Forschungsgemeinschaft to NB (Project no. BO 3793_4-1 AOBJ 639517), and from the project “PMU” of the Deutsche Forschungsgemeinschaft (Project no. 315344689) to SK. Open Access was supported by Open Access Publishing Fund of University of Tübingen.
Acknowledgments
We thank Thomas Stöllner and Jennifer Garner, Deutsches Bergbau-Museum Bochum, and Zofia Stos-Gale for providing the cassiterite from Askaraly and cassiterite samples from Kazakhstan, Kyrgyzstan and Tajikistan. The authors would further like to express their thanks to Bernd Höppner, Sigrid Klaus, CEZA, and Janeta Marahrens, Eurofins WEJ Contaminants GmbH, who assisted the sample preparation and the isotopic analyses in the laboratory. Many thanks to Brittany Kucera for editing the English language. We further acknowledge financial support by Open Access Publishing Fund of University of Tübingen.
Conflict of interest
The authors declare that the research was conducted in the absence of any commercial or financial relationships that could be construed as a potential conflict of interest.
Publisher’s note
All claims expressed in this article are solely those of the authors and do not necessarily represent those of their affiliated organizations, or those of the publisher, the editors and the reviewers. Any product that may be evaluated in this article, or claim that may be made by its manufacturer, is not guaranteed or endorsed by the publisher.
Supplementary material
The Supplementary Material for this article can be found online at: https://www.frontiersin.org/articles/10.3389/feart.2023.1224873/full#supplementary-material
References
Alimov, K., Boroffka, N., Bubnova, M., Burjakov, J., Cierny, J., Jakubov, J., et al. (1998). Prähistorischer Zinnbergbau in Mittelasien – Vorbericht der Kampagne 1997. Eurasia Antiq. 4, 137–199.
Askarov, A. A. (1977). Drevnezemledel´cheskaya kul´tura epokhi bronzy yuga uzbekistana. Fan: Tashkent.
Askarov, A. A., and Abdullaev, B. N. (1983). Dzharkutan (K probleme protogorodskoi tsivilizatsii na yuge Uzbekistana). Tashkent: Fan.
Askarov, А. А., and Shirinov, Т. S. (1993). Rannaja gorodskaja kultura epokhi bronzy juga srednej azii. Samarkand: URFA.
Avanesova, N. A. (1991). Kul´tura pastusheskikh plemen epokhi bronzy aziatskoy chasti SSSR (po metallicheskim izdeliyam). Tashkent: Fan.
Balliana, E., Aramendía, M., Resano, M., Barbante, C., and Vanhaecke, F. (2012). Copper and tin isotopic analysis of ancient bronzes for archaeological investigation: Development and validation of a suitable analytical methodology. Anal. Bioanal. Chem. 405, 2973–2986. doi:10.1007/s00216-012-6542-1
Baratov, S. R. (2001). “Fergana und das Syr-Darja-Gebiet im späten 2. und frühen 1. Jahrtausend v. Chr.,” in Migration und Kulturtransfer: Der Wandel vorder- und zentralasiatischer Kulturen im Umbruch vom 2. zum 1. vorchristlichen Jahrtausend: Akten des Internationalen Kolloquiums Berlin, 23. bis 26. November 1999. Kolloquien zur Vor-und Frühgeschichte, Vol. 6. Editors R. Eichmann, and H. Parzinger (Bonn: Rudolf Habelt), 161–180.
Begemann, F., and Schmitt-Strecker, S. (2009). Über das frühe Kupfer Mesopotamiens. Iran. Antiq. 44, 1–45. doi:10.2143/ia.44.0.2034374
Belyaeva, T. V., and Khakimov, Z. A. (1973). “Drevnebaktriiskie pamyatniki mirshade,” in Iz istorya kul´tury Uzbekistan. Editor G. A. Pugachenkova (Tashkent: Izdatel´stvo Literatury i Iskusstva im. Gafura Guljama), 35–55.
Bendezu-Sarmiento, J., and Lhuillier, J. (2019). “Habitat and occupancy during the bronze age in central Asia: Recent work at the sites of ulug-depe (Turkmenistan) and Dzharkutan (Uzbekistan),” in Urban cultures of central Asia from the Bronze Age to the Karakhanids: Learnings and conclusions from new archaeological investigations and discoveries. Proceedings of the first international congress on central asian archaeology held at the University of Bern, 4–6 February 2016. Editors C. Baumer, and M. Novák (Wiesbaden: Harrassowitz), 97–114.
Berger, D., Brügmann, G., and Pernicka, E. (2018a). “Fraktionierung von Zinnisotopen bei pyrometallurgischen Prozessen und bei Korrosion von Metallartefakten,” in Archäometrie und Denkmalpflege 2018: Jahrestagung am Deutschen Elektronen-Synchrotron Hamburg 20.–24. März 2018. Editor L. Glaser (Hamburg), 60–63.
Berger, D., Figueiredo, E., Brügmann, G., and Pernicka, E. (2018b). Tin isotope fractionation during experimental cassiterite smelting and its implication for tracing the tin sources of prehistoric metal artefacts. J. Archaeol. Sci. 92, 73–86. doi:10.1016/j.jas.2018.02.006
Berger, D., Brügmann, G., and Pernicka, E. (2019a). On smelting cassiterite in geological and archaeological samples: Preparation and implications for provenance studies on metal artefacts with tin isotopes. Archaeol. Anthropol. Sci. 11, 293–319. doi:10.1007/s12520-017-0544-z
Berger, D., Soles, J. S., Giumlia-Mair, A. R., Brügmann, G., Galili, E., Lockhoff, N., et al. (2019b). Isotope systematics and chemical composition of tin ingots from Mochlos (Crete) and other Late Bronze Age sites in the eastern Mediterranean Sea: An ultimate key to tin provenance? PLOS ONE 14, e0218326. doi:10.1371/journal.pone.0218326
Berger, D., Brügmann, G., Friedrich, R., Lutz, J., Meyer, H.-P., and Pernicka, E. (2022a). Shiny bronze in glassy matter: An inconspicuous piece of slag from the bronze age mining site of Mušiston (Tajikistan) and its significance for the development of tin metallurgy in Central Asia. Archaeol. Anthropol. Sci. 14, 150. doi:10.1007/s12520-022-01606-2
Berger, D., Wang, Q., Brügmann, G., Lockhoff, N., Roberts, B. W., and Pernicka, E. (2022b). The Salcombe metal cargoes: New light on the provenance and circulation of tin and copper in Later Bronze Age Europe provided by trace elements and isotopes. J. Archaeol. Sci. 138, 105543. doi:10.1016/j.jas.2022.105543
Berger, D., Kaniuth, K., Brügmann, G., and Pernicka, E. (2023). Why Central Asia’s Mushiston is not a source for the Late Bronze Age tin ingots from the Uluburun shipwreck. Front. Earth Sci. 11. doi:10.3389/feart.2023.1211478
Berger, D., Brügmann, G., Figueiredo, E., Lutz, J., and Pernicka, E. (Forthcoming). “Pyrometallurgy, corrosion and tin isotopes: An experimental and analytical study into the impact of fractionation on provenance research in archaeology,” in Bronze Age Tin: Geological sources, production, and distribution of tin in Bronze Age Eurasia. Proceedings of the international workshop held at the Reiss-Engelhorn-Museen in Mannheim, Germany, 14–16 March 2018. Forschungen zur Archäometrie und Altertumswissenschaft. Editors E. Pernicka, D. Berger, G. Brügmann, C. Frank, J. Marahrens, and B. Nessel (Rahden/Westf.).
Berthoud, T., Besenval, R., Carbonnel, J. P., Cesbron, F., and Liszak-Hours, J. (1978). Les anciennes mines d’Afghanistan: Rapport Preliminaire. Paris: Prospection 1977.
Bostongukhar, S. (1998). Verkhovja Zarafshana vo II tysjachiletii do n.e. Duschanbe: Institut Istorii, Arkheologii i Etnografii im. A. Donisha, Akademii Nauk Respubliki Tadzhikistan.
Bower, N. W., Lundstrom, C. C., and Hendin, D. B. (2019). MC-ICP-MS analyses of tin isotopes in Roman-era bronze coins reveal temporal and spatial variation. Archaeometry 61, 891–905. doi:10.1111/arcm.12459
Brill, R. H., Felker-Dennis, C., Shirahata, H., and Joel, E. C. (1997). “Lead isotope analyses of some Chinese and Central Asian pigments,” in Conservation and ancient sites of the Silk Road. Editor N. Agnew (Los Angeles: The Getty Conservation Institute), 369–378.
Brügmann, G., Berger, D., Frank, C., Marahrens, J., Nessel, B., and Pernicka, E. (2017a). “Tin isotope fingerprints of ore deposits and ancient bronze,” in The tinworking landscape of Dartmoor in a European context: Prehistory to 20th century: Papers presented at a conference in Tavistock, Devon, 6–11 May 2016 to celebrate the 25th anniversary of the DTRG. Editor P. Newman (Exeter: Dartmoor Tinworking Research Group), 103–114.
Brügmann, G., Berger, D., and Pernicka, E. (2017b). Determination of the tin stable isotopic composition in tin-bearing metals and minerals by MC-ICP-MS. Geostand. Geoanalytical Res. 41, 437–448. doi:10.1111/ggr.12166
Cao, C., Shen, P., Pan, H., Li, C., and Eleonora, S. (2018). Fluid evolution and mineralization mechanism of the East Kounrad porphyry Mo-W deposit in the Balkhash metallogenic belt, Central Kazakhstan. J. Asian Earth Sci. 165, 175–191. doi:10.1016/j.jseaes.2018.07.013
Chernykh, E. N. (1992). Ancient metallurgy in the USSR: The early metal age. New York: Cambridge University Press.
Chernyshev, I. V., Golubev, V. N., and Chugaev, A. V. (2017). Anomalous lead isotopic composition of galena and age of altered uranium minerals: A case study of Chauli deposits, Chatkal–Qurama district, Uzbekistan. Geol. Ore Deposits 59, 551–560. doi:10.1134/S107570151706006X
Chiaradia, M., Seltmann, K., Seltmann, R., and Cliff, R. A. (2006). Lead isotope variations across terrane boundaries of the Tien Shan and Chinese Altay. Miner. Deposita 41, 411–428. doi:10.1007/s00126-006-0070-x
Chorievna, G. K. (2021). The lapis lazuli beads in Sapalli culture and ancient Near East. Am. J. Soc. Sci. Educ. Innovations 3, 539–551. doi:10.37547/tajssei/Volume03Issue01-95
Cierny, J. (1995). Die Gruben von Muschiston in Tadschikistan: Stand die Wiege der Zinnbronze in Mittelasien? Der Anschnitt 47, 68–69.
Cierny, J., and Weisgerber, G. (2003). “Bronze Age tin mines in central Asia,” in Le problème de l’étain à l’origine de la métallurgie: Actes du XIVème congrès UISPP, université de Liège, belgique, 2–8 septembre 2001. BAR International Series, Vol. 1199. Editors A. Giumlia-Mair, and F. Lo Schiavo (Oxford: Archaeopress), 23–31.
Cleuziou, S., and Berthoud, T. (1982). Early tin in the Near East: A reassessment in the light of new evidence from western Afghanistan. Expedition 25, 14–19.
Creech, J. B., Moynier, F., and Badullovich, N. (2017). Tin stable isotope analysis of geological materials by double-spike MC-ICPMS. Chem. Geol. 457, 61–67. doi:10.1016/j.chemgeo.2017.03.013
Dubova, N. A. (2019). “Gonur Depe – City of kings and gods, and the capital of Margush country (Modern Turkmenistan): Its discovery by Viktor Sarianidi and recent finds,” in Urban cultures of central Asia from the Bronze Age to the Karakhanids: Learnings and conclusions from new archaeological investigations and discoveries. Proceedings of the first international congress on central Asian archaeology held at the University of Bern, 4–6 February 2016. Editors C. Baumer, and M. Novák (Wiesbaden: Harrassowitz), 29–53.
Fontugne, M., Zajtseva, G. I., Lyonnet, B., Dubova, N. A., and Burova, N. D. (2021). “Radicarbon dates related to the BMAC/Oxus Civilisation,” in The world of the Oxus civilization. Editors B. Lyonnet, and N. A. Dubova (New York: Routledge), 863–911.
Frank, C. (Forthcoming). “The emergence of bronze metallurgy: Bronze artefacts in the ancient Near East during the third millennium BC,” in Bronze Age Tin: Geological sources, production, and distribution of tin in Bronze Age Eurasia. Proceedings of the international workshop held at the Reiss-Engelhorn-Museen in Mannheim, Germany, 14–16 March 2018. Forschungen zur Archäometrie und Altertumswissenschaft. Editors E. Pernicka, D. Berger, G. Brügmann, C. Frank, J. Marahrens, and B. Nessel (Rahden/Westf.).
Friebel, M., Toth, E. R., Fehr, M. A., and Schönbächler, M. (2020). Efficient separation and high-precision analyses of tin and cadmium isotopes in geological materials. J. Anal. Atomic Spectrom. 35, 273–292. doi:10.1039/c9ja00289h
Gamburg, B. Z., and Gorbunova, N. G. (1956). Mogil´nik epokhi bronzy v ferganskoi doline. Kratk. soobshcheniya Instituta Istor. Material´noi Kul´tury 63, 85–93.
Gamburg, B. Z., and Gorbunova, N. G. (1957). Novye dannye o kul´ture epokhi bronzy ferganskoi doliny. Sov. arkheologiya 2, 130–135.
Garner, J. (2013). Das Zinn der Bronzezeit in Mittelasien II: Die montanarchäologischen Forschungen an den Zinnlagerstätten. Archäologie in Iran und Turan, Vol. 194 (Berlin: Deutsches Archäologisches Institut, Eurasien-Abteilung).
Garner, J. (2015). “Bronze Age tin mines in central Asia,” in Archaeometallurgy in Europe III: Proceedings of the 3rd international conference, 29 June to 1 July 2011. Der Anschnitt, Beiheft, Vol. 26. Editors A. Hauptmann, and D. Modaressi-Tehrani (Bochum: Deutsches Bergbau-Museum), 135–142.
Garner, J. (2021). “Metal sources (tin and copper) and the BMAC,” in The world of the Oxus civilization. Editors B. Lyonnet, and N. A. Dubova (New York: Routledge), 799–826.
Grigoriev, S. (2021). Andronovo problem: Studies of cultural Genesis in the Eurasian Bronze Age. Open Archaeol. 7, 3–36. doi:10.1515/opar-2020-0123
Hall, A. (1980). The determination of total tin content of some geological materials by atomic absorption spectrophotometry. Chem. Geol. 30, 135–142. doi:10.1016/0009-2541(80)90120-5
Haustein, M., Gillis, C., and Pernicka, E. (2010). Tin isotopy: A new method for solving old questions. Archaeometry 52, 816–832. doi:10.1111/j.1475-4754.2010.00515.x
Haustein, M. (2013). Isotopengeochemische Untersuchungen zu möglichen Zinnquellen der Bronzezeit Mitteleuropas. Forschungsberichte des Landesmuseums für Vorgeschichte Halle. (Halle (Saale): Landesmuseum für Vorgeschichte Halle) Vol. 3.
Helwing, B. (2009). Rethinking the tin mountains: Patterns of usage and circulation of tin in greater Iran from the 4th to the 1st millennium BC. Türkiye Bilim. Akad. Arkeol. Derg. 12, 209–221. doi:10.22520/tubaar.2009.0017
Isakov, A. I., Kohl, P. L., Lamberg-Karlovsky, C. C., and Maddin, R. (1987). Metallurgical analysis from Sarazm, Tadjikistan SSR. Archaeometry 29, 90–102. doi:10.1111/j.1475-4754.1987.tb00400.x
Isakov, А. I., and Potemkina, Т. М. (1989). Mogilnik plemen epokhi bronzy v Tadzhikistane. Sov. Arkheologija 1, 145–169.
Ivanov, L. (1988). Kashkarchinskij mogilnik – novij pamjatnik epokhi pozdnej bronzy Fergany. Obshchestvennye Nauki Uzb. 10, 44–47.
Jansen, M., Hauptmann, A., Klein, S., and Seitz, H.-M. (2018). The potential of stable Cu isotopes for the identification of Bronze Age ore mineral sources from Cyprus and Faynan: Results from Uluburun and Khirbat Hamra Ifdan. Archaeol. Anthropol. Sci. 10, 1485–1502. doi:10.1007/s12520-017-0465-x
Kaniuth, K. (2006). Metallobjekte der Bronzezeit aus Nordbaktrien. Archäologie in Iran und Turan (Mainz: Philipp von Zabern) Vol. 6.
Kaniuth, K. (2007). The metallurgy of the Late Bronze Age Sapalli culture (Southern Uzbekistan) and its implications for the tin question. Iran. Antiq. 42, 23–40. doi:10.2143/ia.42.0.2017869
Klein, S., Domergue, C., Lahaye, Y., Brey, G. P., and Kaenel, H.-M. V. (2009). The lead and copper isotopic composition of copper ores from the Sierra Morena (Spain). J. Iber. Geol. 35, 59–68.
Konopelko, D. L., Cherny, R. I., Petrov, S. V., Strekopytov, S., Seltmann, R., Vlasenko, N. S., et al. (2022). The Mushiston Sn deposit in Tajik Tien Shan as the type locality for stannite-cassiterite-hydrostannate mineralization: New mineral chemistry data and genetic constraints. J. Geochem. Explor. 239, 107017. doi:10.1016/j.gexplo.2022.107017
Koryakova, L., and Epimakhov, A. V. (2007). The Urals and western Siberia in the Bronze and Iron Ages. Cambridge world archaeology. Cambridge: Cambridge University Press.
Kouznetsova, E. F., Pchenitchnaia, N. F., and Souleimenov, E. N. (1988). Etudes physico-chimiques: Des matières premières et production métallurgique à l’Age du Bronze au Kazakhstan Central. Paléorient 14, 49–55. doi:10.3406/paleo.1988.4438
Kraus, S. (2016). “Metallurgical investigations in Gonur Depe, Turkmenistan,” in Transactions of Margiana archaeological expedition: To the memory of Professor Victor Sarianidi 6. Editors N. A. Dubova, E. B. Antonova, P. M. Kozhin, M. F. Kosarev, R. G. Muradov, R. M. Sataevet al. (Moscow: Stari sad), 257–264.
Kraus, S. (2021). “Archaeometallurgical studies on BMAC artifacts,” in The world of the Oxus civilization. Editors B. Lyonnet, and N. A. Dubova (New York: Routledge), 779–798.
Kraus, S., Ahmedshaev, A. S., Saipov, H. A., and Boroffka, N. (Forthcoming). On the history of research on prehistoric copper mining in Uzbekistan and adjacent regions. Archäologische Mittl. aus Iran Turan.
Kutimov, J. G. (2013). Novye issledovanija mogilnika epokhi pozdnej bronzy Kumsaj (Tadzhikistan). Arkheologicheskie Vesti 19, 75–91.
Kuz’mina, E. E. (1991). Die urgeschichtliche Metallurgie der Andronovo-Kultur: Bergbau, Metallurgie und Metallbearbeitung. Z. für Archäologie 25, 29–48.
Kuz’mina, E. E. (2004). “Historical perspectives on the Andronovo and metal use in eastern Asia,” in Metallurgy in ancient eastern Eurasia from the Urals to the Yellow River. Editor K. M. Linduff (Lewiston: Mellen), 37–84.
Kuz’mina, E. E., and Vinogradova, N. M. (1983). Beziehungen zwischen bronzezeitlichen Steppen-und Oasenkulturen in Mittelasien. Beiträge zur Allg. Vgl. Archäologie 5, 35–55.
Litvinskij, B. А., Okladnikov, A. P., and Ranov, V. A. (1962). Drevnosti kajrak-kumov: Drevnejshaja istorija severnogo tadzhikistana. Duschanbe: Izd. Akad. Nauk Tadzh. SSSR.
Liu, P., Mao, J., Lehmann, B., Weyer, S., Horn, I., Mathur, R., et al. (2021). Tin isotopes via fs-LA-MC-ICP-MS analysis record complex fluid evolution in single cassiterite crystals. Am. Mineralogist 106, 1980–1986. doi:10.2138/am-2021-7558
Luneau, É. (2017). “Transfers and interactions between north and south in central Asia during the Bronze Age,” in Interaction in the Himalayas and central Asia: Processes of transfer, translation and transformation in art, archaeology, religion and polity. Proceedings of the third international SEECHAC colloquium 25–27 Nov. 2013, Austrian Academy of Sciences, Vienna. Denkschriften (Österreichische Akademie der Wissenschaften. Philosophisch-Historische Klasse), Vol. 495. Editors E. Allinger, F. Grenet, C. Jahoda, M.-K. Lang, and A. Vergati (Vienna: Austrian Academy of Sciences Press), 13–27.
Lutz, J., and Pernicka, E. (1996). Energy dispersive X-ray fluorescence analysis of ancient copper alloys: Empirical values for precision and accuracy. Archaeometry 38, 313–323. doi:10.1111/j.1475-4754.1996.tb00779.x
Lyonnet, B., and Dubova, N. A. (Editors) (2021). The world of the Oxus civilization (New York: Routledge).
Maddin, R. (1998). “Early metallurgy: The tin mystery,” in The fourth international conference on the beginning of the use on metals and alloys (BUMA-IV), May 25–27, 1998, Kunibiki Messe, Matsue (Shimane, Japan (Matsue)), 1–4.
Markl, G., Lahaye, Y., and Schwinn, G. (2006). Copper isotopes as monitors of redox processes in hydrothermal mineralization. Geochimica Cosmochimica Acta 70, 4215–4228. doi:10.1016/j.gca.2006.06.1369
Mason, A. H., Powell, W. G., Bankoff, H. A., Mathur, R., Price, M., Bulatovic, A., et al. (2020). Provenance of tin in the Late Bronze Age balkans based on probabilistic and spatial analysis of Sn isotopes. J. Archaeol. Sci. 122, 105181. doi:10.1016/j.jas.2020.105181
Mathur, R., Powell, W., Mason, A., Godfrey, L., Yao, J., and Baker, Ma. E. (2017). Preparation and measurement of cassiterite for Sn isotope analysis. Geostand. Geoanalytical Res. 41, 701–707. doi:10.1111/ggr.12174
Molodin, V., and Polos’mak, N. (2014). “Southern Siberia during the Bronze and Early Iron periods,” in The Cambridge World Prehistory. Editors C. Renfrew, and P. Bahn (Cambridge: Cambridge University Press), 1638–1657.
Muhly, J. D. (1985). Sources of tin and the beginnings of bronze metallurgy. Am. J. Archaeol. 89, 275–291. doi:10.2307/504330
Narasimhan, V. M., Patterson, N., Moorjani, P., Rohland, N., Bernardos, R., Mallick, S., et al. (2019). The formation of human populations in South and Central Asia. Science 365, eaat7487. doi:10.1126/science.aat7487
Nezafati, N. (2006). Au-Sn-W-Cu-mineralization in the Astaneh-Sarband Area, West Central Iran, including a comparison of the ores with ancient bronze artifacts from Western Asia. PhD thesis, University: Tübingen.
Nezafati, N., Pernicka, E., and Momenzadeh, M. (2009). Introduction of the Deh Hosein ancient tin-copper mine, Western Iran. Türkiye Bilim. Akad. Arkeol. Derg. (TÜBA-AR) 12, 221–236.
Nezafati, N., Pernicka, E., and Momenzadeh, M. (2011). “Early tin-copper ore from Iran,” in Anatolian metal V. Der Anschnitt, Beiheft, Vol. 21. Editor Ü. Yalcin (Bochum: Selbstverlag Deutsches Bergbau-Museum), 211–230.
Nezafati, N., and Stöllner, T. (2017). Economic geology, mining archaeological and archaeometric investigations at the Veshnaveh ancient copper mine, Central Iran. Metalla 23, 67–90.
Nezafati, N., Pernicka, E., Helwing, B., and Kirchner, D. (2021). “Reassessment of the prehistoric metallurgy at Arisman, central Iran,” in On salt, copper and gold: The origins of early mining and metallurgy in the Caucasus. Proceedings of the conference held in Tbilisi (Georgia), June 16th-19th 2016. Archéologie(s), Vol. 5. Editors C. Marro, and T. Stöllner (Lyon: Maison de l’Orient et de la Méditerranee), 231–260.
Nokandeh, J., Rekāvandi, H. O., and ‘Abbāsi, Q. (2006). A preliminary report on the discovery of the Bāzgir hoard, Gorgan Plain 1379. Archaeol. Rep. 4, 113–129.
Oudbashi, O., Rademakers, F. W., Vanhaecke, F., Degryse, P., Hasanpour, A., and Bahadori, S. (2021a). An old problem in a new light: Elemental and lead isotopic analysis of Luristan Bronzes. J. Archaeol. Sci. Rep. 39, 103163. doi:10.1016/j.jasrep.2021.103163
Oudbashi, O., Renson, V., and Hasanpour, A. (2021b). Lead isotope analysis of tin bronze objects from the Iron Age site at Baba Jilan, Luristan, Western Iran. Archaeol. Anthropol. Sci. 13, 161. doi:10.1007/s12520-021-01428-8
Parzinger, H., and Boroffka, N. (2001). Woher stammt das Zinn der Bronzezeit in Mittelasien. Archäologie Deutschl. 3, 12–17.
Parzinger, H., and Boroffka, N. (2003). Das Zinn der Bronzezeit in Mittelasien I: Die siedlungsarchäologischen Untersuchungen im Umfeld der Zinnlagerstätten. Archäologie in Iran und Turan. Editors H. Parzinger, and N. Boroffka (Mainz: Philipp von Zabern) Vol. 5.
Parzinger, H., Pernicka, E., and Weisgerber, G. (2003). “Einführung,” in Das Zinn der Bronzezeit in Mittelasien I: Die siedlungsarchäologischen Untersuchungen im Umfeld der Zinnlagerstätten. Archäologie in Iran und Turan, Vol. 5. Editors H. Parzinger, and N. Boroffka (Mainz: Philipp von Zabern), 1–14.
Pavlova, G. G., and Borisenko, A. S. (2009). The age of Ag-Sb deposits of Central Asia and their correlation with other types of ore systems and magmatism. Ore Geol. Rev. 35, 164–185. doi:10.1016/j.oregeorev.2008.11.006
Pavlova, G. G., Palessky, S. V., Borisenko, A. S., Vladimirov, A. G., Seifert, T., and Phane, L. A. (2015). Indium in cassiterite and ores of tin deposits. Ore Geol. Rev. 66, 99–113. doi:10.1016/j.oregeorev.2014.10.009
Pernicka, E. (1987). Erzlagerstätten in der Ägäis und ihre Ausbeutung im Altertum. Geochemische Untersuchungen zur Herkunftsbestimmung archäologischer Metallobjekte. Jahrb. RGZM 34, 607–714.
Pernicka, E. (1990). Gewinnung und Verbreitung der Metalle in prähistorischer Zeit. Jahrb. RGZM 37, 21–129.
Pernicka, E. (2014). “Provenance determination of archaeological metal objects,” in Archaeometallurgy in global perspective: Methods and syntheses. Editors B. W. Roberts, and C. P. Thornton (New York: Springer), 239–268.
Pernicka, E., Adam, K., Böhme, M., Hezarkhani, Z., Nezafati, N., Schreiner, M., et al. (2011). “Archaeometallurgical research on the western central Iranian plateau,” in Early mining and metallurgy on the western central Iranian plateau. Archäologie in Iran und Turan, Vol. 9. Editors R. Vatandoust, H. Parzinger, and B. Helwing (Mainz: Philipp von Zabern), 631–688.
Pernicka, E., and Frank, C. (Forthcoming). “Lead isotope analysis of bronzes from the third millennium BC,” in Bronze Age Tin: Geological sources, production, and distribution of tin in Bronze Age Eurasia. Proceedings of the international workshop held at the Reiss-Engelhorn-Museen in Mannheim, Germany, 14–16 March 2018. Forschungen zur Archäometrie und Altertumswissenschaft. Editors E. Pernicka, D. Berger, G. Brügmann, C. Frank, J. Marahrens, and B. Nessel (Rahden/Westf.).
Peters, S. G., Ludington, S. D., Orris, G. J., Sutphin, D. M., Bliss, J. D., and Rytuba, J. J. (2007). Preliminary non-fuel mineral resource assessment of Afghanistan 2007.
Piccardo, P., Mille, B., and Robbiola, L. (2007). “Tin and copper oxides in corroded archaeological bronzes,” in Corrosion of metallic heritage artefacts: Investigation, conservation and prediction of long term behaviour. European Federation of Corrosion (EFC) Series, Vol. 48. Editors P. Dillmann, P. Piccardo, H. Matthiesen, and G. Beranger (Norwich, NY: Woodhead Publishing Ltd.), 239–262.
Pigott, V. C. (2020). “The acquisition of tin in Bronze Age southwest Asia,” in The world of the Oxus civilization. Editors B. Lyonnet, and N. A. Dubova (New York: Routledge), 827–861.
Powell, W., Johnson, M., Pulak, C., Yener, K. A., Mathur, R., Bankoff, H. A., et al. (2021). From peaks to ports: Insights into tin provenance, production, and distribution from adapted applications of lead isotopic analysis of the Uluburun tin ingots. J. Archaeol. Sci. 134, 105455. doi:10.1016/j.jas.2021.105455
Powell, W., Frachetti, M., Pulak, C., Bankoff, H. A., Barjamovic, G., Johnson, M., et al. (2022). Tin from Uluburun shipwreck shows small-scale commodity exchange fueled continental tin supply across Late Bronze Age Eurasia. Sci. Adv. 8, eabq3766. doi:10.1126/sciadv.abq3766
Robbiola, L., Blengino, J.-M., and Fiaud, C. (1998). Morphology and mechanisms of formation of natural patinas on archaeological Cu-Sn alloys. Corros. Sci. 40, 2083–2111. doi:10.1016/s0010-938x(98)00096-1
Ruzanov, V. D. (1980). K voprosu o metalloobrabotke u plemen chustskoj kultury. Sov. Arkheologija 4, 55–64.
Ruzanov, V. D. (1982). Istoriya drevney metallurgii i gornogo dela Uzbeksitana v epochu bronzy i rannego zheleza.
Ruzanov, V. (1999). “Zum frühen Auftreten der Zinnbronze in Mittelasien,” in The Beginnings of metallurgy: Proceedings of the international conference “The beginnings of metallurgy”, Bochum 1995. Der Anschnitt, Beiheft, Vol. 9. Editors A. Hauptmann, E. Pernicka, T. Rehren, and Ü. Yalcin (Bochum: Deutsches Bergbau-Museum), 103–105.
Sarianidi, V. I. (2005). Gonurdepe: Shalaryn we hudaylaryn shäheri. Gonur-Depe: City of kings and gods. Aschkhabad: Miras.
Sarianidi, V. I., and Dubova, N. A. (2010). Zadolgo do Zaratushtry: Arkheologicheskie dokazatelʹstva protozoroastrizma v Baktrii i Margiane. Moscow: Staryi sad.
Schulze, J. M. (2018). Bestimmung von Sn-Isotopenverhältnissen mittels fs-LA-ICP-MC-MS und ihre Anwendbarkeit für die Provenienzanalytik an historischen Metallartefakten. PhD thesis, University: Hannover.
Shafiei, B. (2010). Lead isotope signatures of the igneous rocks and porphyry copper deposits from the Kerman Cenozoic magmatic arc (SE Iran), and their magmatic-metallogenetic implications. Ore Geol. Rev. 38, 27–36. doi:10.1016/j.oregeorev.2010.05.004
She, J.-X., Kubik, E., Li, W., and Moynier, F. (2023). Stable Sn isotope signatures of Mid-ocean ridge basalts. Chem. Geol. 622, 121347. doi:10.1016/j.chemgeo.2023.121347
Stech, T., and Pigott, V. C. (1986). The metals trade in Southwest Asia in the third millennium B.C. Iraq 48, 39. doi:10.2307/4200250
Steiniger, D. (2019). “Lapis Lazuli—Raw material sources, provenance studies and prehistoric distribution patterns in Eurasia: An overview,” in Social changes and trade between the east and the west in Eurasia: Archaeological and archaeometrical approaches. Proceedings of the international symposium on ancient Japanese studies, Meiji University, Tokyo, 23.02.2019 (Tokyo: Meiji University), 13–20.
Steiniger, D., and Junker, K. (2020). Pamir-Gebirge, Tadschikistan: Tadschikisch-Deutsche Pamir Expedition. Die Arbeiten des Jahres 2019. e-Forschungsberichte 3, 101–110. doi:10.34780/EFB.V0I3.1011
Stöllner, T., Samaschev, Z., Berdenov, S., Cierny, J., Doll, M., Garner, J., et al. (2011). “Tin from Kazakhstan,” in Anatolian metal V. Der Anschnitt, Beiheft, Vol. 24. Editor Ü. Yalcin (Bochum: Selbstverlag Deutsches Bergbau-Museum), 231–251.
Stöllner, T., Samasev, Z., Berdenov, S., Cierny, J., Doll, M., Garner, J., et al. (2013). “Zinn und Kupfer aus dem Osten Kasachstans,” in Unbekanntes Kasachstan: Archäologie im Herzen Asiens. Editors T. Stöllner, and S. Zajnolla (Bochum: Deutsches Bergbausmuseum), 357–382.
Sun, L., Hu, Y., Sun, W., Gao, Z., and Tian, M. (2017). Selective recovery of mushistonite from gravity tailings of copper-tin minerals in Tajikistan. Minerals 7, 242–253. doi:10.3390/min7120242
Sverchkov, L. M. (2010). A history of research on ancient mining in Uzbekistan. Archäologische Mittl. aus Iran Turan 41, 141–164.
Sverchkov, L. M., and Boroffka, N. (2015). Materialy poseleniya epokhi pozdney bronzy Molali. Arkhaeologiya Uzb. 1, 75–88.
Tapster, S., and Bright, J. W. G. (2020). High-precision ID-TIMS cassiterite U–Pb systematics using a low-contamination hydrothermal decomposition: Implications for LA-ICP-MS and ore deposit geochronology. Geochronology 2, 425–441. doi:10.5194/gchron-2-425-2020
Terenozhkin, A. I. (1962). Klad andronovskikh bronzovykh predmetov iz s. Brichmulla bliz Tashkenta. Sov. Arkheologiya 3, 279–281.
Teufer, M., Natalja, M., and Kutimov, J. (2014). Der Übergang von der Spätbronze-zur Früheisenzeit in Mittelasien. Das. Altert. 59, 109–148.
Teufer, M. (2015). Spätbronzezeitliche Grabfunde aus Nordbaktrien und benachbaren Regionen: Studien zur Chronologie zwischen Aralsee und Persischem Golf. Berlin: Reimer.
Thomalsky, J., Bräutigam, B., Karaucak, M., and Kraus, S. (2013). Early mining and metal production in Afghanistan: The first year of investigations. Archäologische Mittl. aus Iran Turan 45, 199–230.
Thornton, C. P., Liezers, L.-K., Liezers, C. C., Liezers, M., and Young, S. M. M. (2005). “Stech and Pigott revisited: New evidence concerning the origins of tin bronze in the light of chemical and metallographic analyses of the metal artefacts from Tepe Yahya, Iran,” in Proceedings of the 33rd International Symposium on Archaeometry, 22–26 April 2002. Amsterdam Geoarchaeological and Bioarchaeological Studies, Vol. 3. Editors H. Kars, and E. Burke (Amsterdam: University of Amsterdam), 395–398.
Vinogradova, N. M. (2000). “Issledovanija kontaktov zemledelcheskogo i stepnogo naselenija na juge Srednej Azii (juzhnyj Tadzhikistan) v epokhu bronzy,” in Arkheologija, paleoekologija i paleodemografija Evrazii. Editor V. S. Olkhovskij (Moscow: GEOS), 89–109.
Vinogradova, N. (2004). “The settlement and the cemetery of Kangurttut among the late bronze age sites of north-eastern Bactria (south Tajikistan),” in Annali dell’Università degli studi di Napoli L’Orientale. Rivista del Dipartimento di Studi Asiatici e del Dipartimento di Studi e Ricerche su Africa e Paesi Arabi 64, 75–97.
Vinogradova, N. M., and Pyankova, L. T. (1990). Kumsai cemetery in south Tadjikistan. Inf. Bull. 17, 121–148.
Vinogradova, N. M., Ranov, V. A., and Filimonova, T. G. (2008). Pamjatniki Kangurttuta v jugo-zaoadnom Tadzhikistane (epokha neolita i bronzovogo veba). Moscow: Inst. Vostokovedenija RAN.
Vinogradova, N. M., Kutimova, J., and Teufer, M. (2010). “Pogrebenija bronzovogo veka v okrestnostjah kishlaka “Gelot” na juge Tadzhikistana,” in Na puti otkrytija civilizacii: Sbornik statej, k 80-letiju V. I. Sarianidi. Trudy Margianskoj arheologicheskoj jekspedicii (Sankt-Petersburg: Aletejja), 389–411.
von Baer, K. E. (1876). Von wo das Zinn zu den ganz alten Bronzen gekommen sein mag? Arch. für Anthropol. 9, 263–267.
Voronets, M. E. (1948). “Braslety bronzovoi epokhi muzeya istorii ANUzSSR,” in Trudy instituta istorii i arkheologii, akademiya nauk uzbekskoi SSR. Materialy po arkheologii uzbekistana 1, 65–70.
Wang, X., Amet, Q., Fitoussi, C., and Bourdon, B. (2018). Tin isotope fractionation during magmatic processes and the isotope composition of the bulk silicate Earth. Geochimica Cosmochimica Acta 228, 320–335. doi:10.1016/j.gca.2018.02.014
Wang, X., Fitoussi, C., Bourdon, B., Fegley, B., and Charnoz, S. (2019). Tin isotopes indicative of liquid–vapour equilibration and separation in the Moon-forming disk. Nat. Geosci. 12, 707–711. doi:10.1038/s41561-019-0433-4
Weisgerber, G. (2004). Prähistorischer und historischer Bergbau in Afghanistan. Der Anschnitt 56, 190–211.
Wong, K.-H., Zhou, M.-F., Chen, W. T., O’Brien, H., Lahaye, Y., and Chan, S.-L. J. (2017). Constraints of fluid inclusions and in-situ S-Pb isotopic compositions on the origin of the North Kostobe sediment-hosted gold deposit, eastern Kazakhstan. Ore Geol. Rev. 81, 256–269. doi:10.1016/j.oregeorev.2016.10.004
Yamazaki, E., Nakai, S., Sahoo, Y., Yokoyama, T., Mifune, H., Saito, T., et al. (2014). Feasibility studies of Sn isotope composition for provenancing ancient bronzes. J. Archaeol. Sci. 52, 458–467. doi:10.1016/j.jas.2014.09.014
Zhou, Z.-H., Mao, J.-W., Zhao, J.-Q., Gao, X., Weyer, S., Horn, I., et al. (2022). Tin isotopes as geochemical tracers of ore-forming processes with Sn mineralization. Am. Mineralogist 107, 2111–2127. doi:10.2138/am-2022-8200
Glossary
Keywords: bronze, tin origin, copper origin, Mushiston, Central Asia, Bronze Age, tin isotope analysis, BMAC
Citation: Berger D, Kaniuth K, Boroffka N, Brügmann G, Kraus S, Lutz J, Teufer M, Wittke A and Pernicka E (2023) The rise of bronze in Central Asia: new evidence for the origin of Bronze Age tin and copper from multi-analytical research. Front. Earth Sci. 11:1224873. doi: 10.3389/feart.2023.1224873
Received: 18 May 2023; Accepted: 10 July 2023;
Published: 03 August 2023.
Edited by:
Andrea Dolfini, Newcastle University, United KingdomReviewed by:
Kunlong Chen, University of Science and Technology Beijing, ChinaPura Alfonso, Universitat Politecnica de Catalunya, Spain
Copyright © 2023 Berger, Kaniuth, Boroffka, Brügmann, Kraus, Lutz, Teufer, Wittke and Pernicka. This is an open-access article distributed under the terms of the Creative Commons Attribution License (CC BY). The use, distribution or reproduction in other forums is permitted, provided the original author(s) and the copyright owner(s) are credited and that the original publication in this journal is cited, in accordance with accepted academic practice. No use, distribution or reproduction is permitted which does not comply with these terms.
*Correspondence: Daniel Berger, daniel.berger@ceza.de; Ernst Pernicka, ernst.pernicka@ceza.de
†ORCID:Daniel Berger, orcid.org/0000-0001-6214-2264; Kai Kaniuth, orcid.org/0000-0002-6892-0917; Nikolaus Boroffka, orcid.org/0000-0002-3437-1569; Gerhard Brügmann, orcid.org/0000-0002-9904-0445; Steffen Kraus, orcid.org/0000-0002-8467-4082; Andreas Wittke, orcid.org/0000-0002-4509-9388; Ernst Pernicka, orcid.org/0000-0003-4746-9239