1 Introduction
Enhanced rock weathering (ERW), as a negative emissions technology for climate change mitigation, has received far more public, governmental, and academic attention (according to the authors’ account of engagement with such actors) in the past year than in the many years since its first mention in the literature. The term ERW was conceived by Beerling (2017), but the field of research referred to as “enhanced weathering” (EW) can see its origins, by this name, at least as far back as the works of Power and Southam (2005) and Lenton and Britton (2006). This opinion piece provides learnings and thoughts from the GeoRewind group at the University of Guelph (Canada) on what we have defined as “pathways,” “roundabouts,” “roadblocks,” and “shortcuts” to the safe and sustainable deployment of ERW in agriculture.
2 Current status of ERW research and deployment
In the academic space, the pace of publications and the diversification of countries and institutions involved in EW research have undoubtedly accelerated in recent years (Figure 1A), but it still feels like this is the tip of the iceberg (e.g., unequal global reach), considering the many lingering questions to be addressed. For example, the carbon removal knowledge gaps listed in the Frontiersgaps.com (2023) website include, as of April 2023, questions on: 1) how the choice of mineral feedstock (e.g., virgin vs. residual and basalt vs. other silicates) affects ecosystem co-benefits and/or environmental impacts; 2) how mineral feedstock should be characterized for safe use; 3) what an optimal mineral application (and re-application) rate is and its timing, considering desired carbon sequestration rates, costs, and farming practices; 4) in what ways silicate minerals interact with other soil amendments and with other soil acids; and 5) the longstanding (and at times debatable as in Buckingham et al. (2023) versus West et al. (2023)) geochemistry topic of determining realistic weathering rates under field conditions. Such open questions about the feasibility, practicality, and efficiency of ERW inspire discussions that follow.
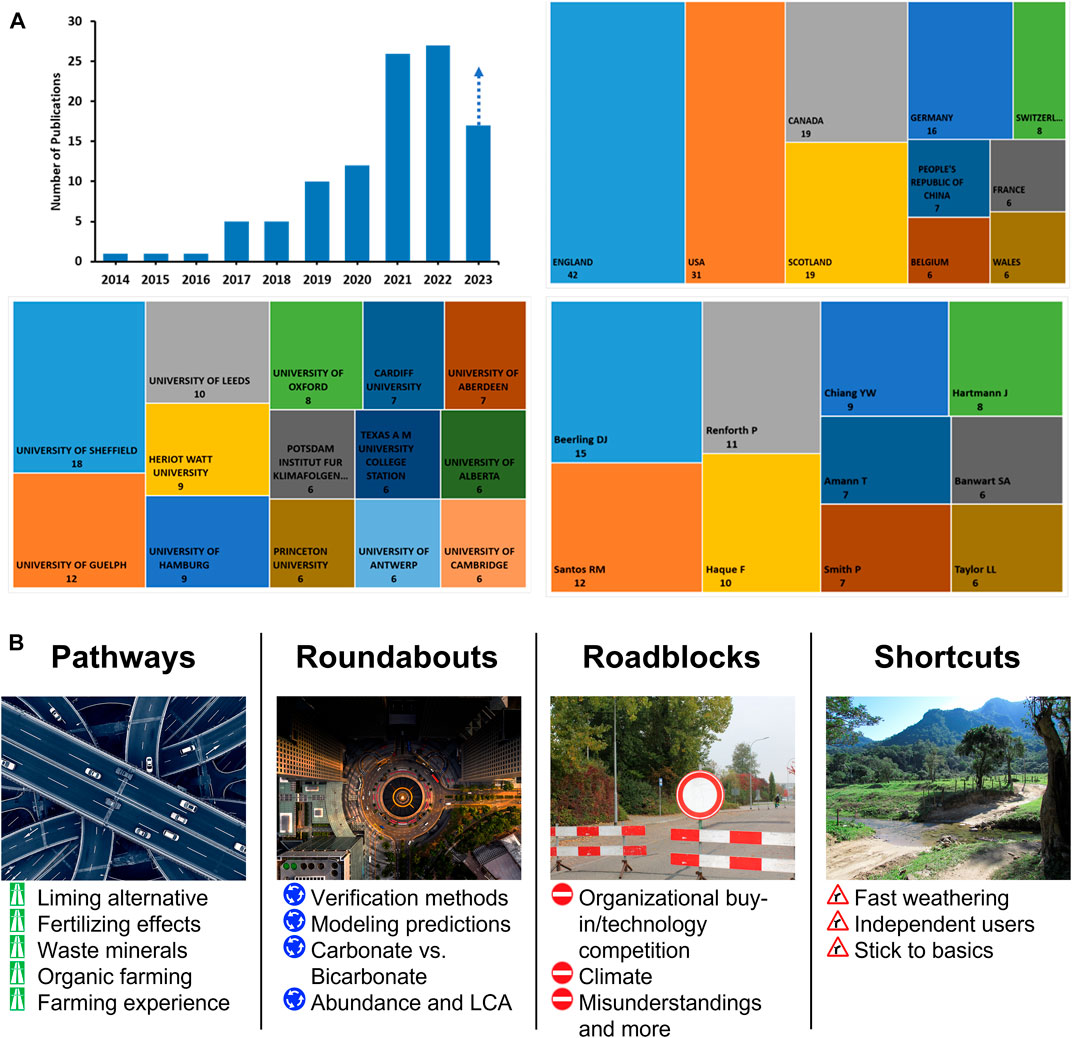
FIGURE 1. (A) Bibliometric analysis of ERW research publications (search string: {“enhanced rock weathering” or [“enhanced weathering” and (“sequestr*” or “capture” or “removal” or “drawdown”) and (“soil*” or “agricultur*” or “crop*” or “plant*”)]}; data source: Web of Science as of 10 July 2023) showing article publications per year since 2014 (top left), top 10 countries (top right), top 10 institutions where in there is a six-way tie for the ninth place (bottom left), and top 10 authors (bottom right). (B) Traffic-themed structure and discussion points of this opinion article (the shortcut image is reproduced from Valadão (2017) CC-BY-SA; other images and symbols are in the public domain).
Evidence of ERW as a technology moving from the academic side to the deployment side (also known as crossing the so-called “valley of death” of innovation (Ellwood et al., 2022)) includes new companies and business models that are supporting the mineral supply, the creation and management of monitoring, reporting, and verification (MRV) methodologies, and the issuance of credits for carbon removal/avoidance/offset (André and Valenciano-Salazar, 2022). A highlight of 2022 was the public release of the Puro Standard Enhanced Rock Weathering Methodology (Puro.earth, 2022) to be used in the voluntary carbon markets to enable the issuance of CO2 removal certificates (CORCs) for ERW activities of certified suppliers. In the same line of thought as Finland-based Puro.earth, US-based VERRA also set out in 2022 to establish its own methodology (VERRA, 2022). US-based CarbonPlan also released in 2022 a framework to help guide research and MRV efforts on ERW (CarbonPlan, 2022).
On the suppliers’ front, several companies that recently emerged have made critical strides. UK-based UNDO celebrated in 2022 its first commercial-scale deployment of basalt (UNDO, 2022), Ireland-based Silicate Carbon was a Shell GameChanger Winner in the THRIVE | Shell Climate-Smart Agriculture Challenge (THRIVE, 2023), and US-based Lithos Carbon has reportedly spread over 11,000 imperial tons of basalt (Wire, 2022). Here, it is notable that these companies have been founded by, or have close relationships with, the most active academic research groups on ERW, which speaks of the need for a scientific direction on the deployment of ERW, given the aforementioned lingering questions.
3 Discussion of pathways, roundabouts, roadblocks, and shortcuts to safe and sustainable deployment of ERW in agriculture
The GeoRewind team has categorized over 5 years of ERW experiences into the following traffic-inspired (Figure 1B) sub-sections. To be clear, these are not meant to be comprehensive or exhaustive discussions but food for thought for future ERW efforts.
3.1 Pathways for ERW
Pathways are low-resistance directions in ERW research and deployment that help accelerate hypothesis testing, knowledge accumulation, and the creation of feasible and trustable practices.
3.1.1 Liming alternative
The common use of liming agents in agriculture (wherein liming with limestone/dolomite is typically a net CO2 emitter (West and McBride, 2005)) is the most direct path for silicate amendments, considering the available supply chain, equipment for spreading, budget for soil amendment, scheduling opportunity, and long-term experience with mineral amendments. Notably, there are clear grower recommendations on the amendment rate of liming agents based on their liming index (Reid et al., 2006), yet the liming index of silicate amendments is unknown, and available studies on the use of silicates as liming agents are often inconclusive about their liming effects and effects on soil carbon (Amoakwah et al., 2023), wherein overliming with silicates (Yan et al., 2023) can cause the same short-term loss of soil organic carbon as for conventional liming (Paradelo et al., 2015). This calls for measured amendment approaches when considering the need for a sufficient silicate amendment to enable MRV and correct dosage to maximize agronomic benefits and total soil carbon storage.
3.1.2 Fertilizing effects
The field of soil remineralization is being developed alongside the technology of ERW and has a longer existence, considering that rock dusts have historically been used worldwide as a locally available low-cost fertilizing amendment (van Straaten, 2007). Many silicate rocks contain appreciable amounts of plant nutrients such as P and K, which can be mobilized during weathering; hence, their use to reduce (at least partially) the use of artificial fertilizers can have additional emission reduction consequences. An often-overlooked non-essential plant nutrient is silica, which is also released in a reactive form (colloidal silica or silicic acid) during weathering and can be beneficial for crops that take up silica for tissue strength (Jariwala et al., 2022; Swoboda et al., 2022).
3.1.3 Waste minerals
A continued concern about the scalability of ERW is the sourcing of suitable minerals and the added environmental impact of new mining operations. There are global stockpiles of mine waste, from quarrying activities, ore mining activities, and metallurgical activities, that have weathering potential and suitable safe composition (Paulo et al., 2021), and those are low-hanging fruits, apart from possible regulatory barriers on waste use in food production, to accelerate ERW deployment.
3.1.4 Organic farming
Despite representing a small fraction of global farming operations, organic farming calls for the use of natural soil amendments. Silicate minerals, as liming agents, fertilizing agents, or solely for carbon sequestration, can qualify for organic use. For example, wollastonite used in the GeoRewind work is organic-certified (Organic Materials Review Institute, 2015).
3.1.5 Farming experience
Many farmers are used to liming; hence, there is a low barrier to entry for ERW if the same conventional logistics are used to deliver and spread minerals. Notably, farming experience should trump scientific advice that, at times, propose very large amendment rates (Kelland et al., 2020). According to the Ontario Agricultural College Dean Prof. Rene Van Acker, “If the technology does not take into account the practical reality of farming, that’s a big mistake” (Grip Workshop, 2023).
3.2 Roundabouts of ERW
Roundabouts are topics that generate certain levels of uncertainty and that can lead to taking a better path when an evidence-based decision is made. On the spectrum of technology development, they consume time and energy but often help thoughts mature and become well-grounded.
3.2.1 Verification methods
It remains unclear if ERW verification should rely on soil water analysis (e.g., for alkalinity, electrical conductivity, isotope, or trace metal analysis), soil solid analysis (e.g., calcimetry, thermogravimetry, X-ray diffraction, and X-ray fluorescence), gas analysis (e.g., soil PCO2 and eddy covariance), or even remote sensing (Almaraz et al., 2022). Scientifically, an all-of-the-above approach is useful for building confidence in the mechanisms of ERW and the fate of weathering products and residues, but practically, it remains to be determined what a feasible, scalable, and equitable form of verifying ERW rates and extent is. Eventually, as with other farming practices, ERW may become a guideline-based practice rather than verification-based technology.
3.2.2 Modeling predictions (global and site-specific)
Efforts have been made to model ERW from the scale of a single mineral grain (Georgakopoulos et al., 2016) to a finite portion of soil (column or a field) (Kelland et al., 2020), or to a watershed and beyond (Zhang et al., 2022). All of these have drawbacks, limitations, and uncertainties. Yet modeling is the backbone of MRV methodologies. As with verification, distinctions are needed for what models are meant for scientific use and what models serve to constrain carbon sequestration estimates (i.e., net CO2 removal after accounting for carbonate precipitation (partial or complete), CO2 off-gassing, loss of alkaline earth metals to sorption and plant uptake, etc.) and be connected to carbon markets.
3.2.3 Carbonate vs. bicarbonate
Some studies have assumed that solid carbonates are the products of ERW (Manning et al., 2013; Haque et al., 2020), and some have assumed that soluble bicarbonates are the products (Amann and Hartmann, 2022; Buckingham et al., 2022). Either can be, and at any point in time, they depend on the soil, climate, soil saturation, and other factors (Khalidy et al., 2022). The carbonate assumption is conservative (and geologically long-term), while the bicarbonate assumption is maximal (and geologically short-term). It, thus, remains an MRV uncertainty as to what alkaline earth metal-to-CO2 ratio to use in the carbon accounting of ERW. An equitable solution may be to use a constant global value of such ratios (within reason as to locations/conditions that make sense for ERW) as it is not at the farmer’s control if the ratio in one land is lower than in another, yet farmers incur similar costs for silicate spreading.
3.2.4 Abundance and LCA
The availability of abundant minerals regionally for any given point of application is intricately tied to the life cycle carbon footprint of an ERW implementation (Eufrasio et al., 2022). Mineral reserves of suitable silicates for ERW are not well-recorded as geological surveys normally focus on ores of commercial value for other applications. As such, a renewed consideration of minerals stocks is needed to inform LCA models of ERW deployment in different regions of the world. Some efforts have been made for mine tailings (Bullock et al., 2021; Bullock et al., 2022).
3.3 Roadblocks to ERW
Roadblocks put a stop to a certain research direction or idea. However, roadblocks eventually clear, so they are instances where unforeseen work is needed to overcome deployment barriers.
3.3.1 Organizational buy-in/technology competition
Government agencies and industrial organizations (e.g., grower associations) have been slow to notice and take up interest in ERW. Examples of this include the absence of ERW mention in agricultural funding calls, the usage of the term “soil carbon sequestration” to signify only those approaches that lead to organic carbon accumulation (Bai and Cotrufo, 2022), and the lack of ERW research occurring within such agencies and organizations or with involvement of their members (from the bibliometric data on Figure 1A, the few exceptions include Haque et al. (2019), Gomez-Casanovas et al. (2021)). This shows that academic groups should perform more of what is known as “knowledge translation and transfer,” “getting research into practice,” and “extension research” (Grip workshop, 2023).
3.3.2 Climate
Conceptual reviews on ERW have largely told a story that ERW is best suited for deployment in warmer regions, at times termed the “Global South” (Institute for Carbon Removal Law and Policy, 2023), in view of higher weathering rates and more acidic soils (Taylor et al., 2016). This has led some studies to omit the deployment of ERW in the vast farmland regions of wealthier nations located in colder or dried climates, such as Canada and the Northern Plains of the US (Hicks et al., 2022). Considering mineral sources and financing resources, ERW should be pursued in most regions of the world as a means to accelerate the development of logistics, guidelines, regulations, and MRV methodologies, and to identify risks and limits before it is deployed at large scales in regions where impacts may be less likely to be studied.
3.3.3 Misunderstandings and more
Historically, certain misunderstandings and disagreements have either slowed the progress on ERW until additional studies could move the science forward, or created conditions that could discourage large-scale ERW adoption. Examples include the following:
(i) In the early days of EW research, it was posed that silicic acid accumulation in natural waters would hinder the weathering reaction progress based on the principles of reaction equilibrium (Köhler et al., 2011), but a debate with Schuiling et al. (2011) showed that silicon removal via the formation of solid phases and biological sinks is the more plausible mechanism, and one that remains well-grounded by experimental observations and is used in reactive ERW models.
(ii) It has been suggested that metal leaching and accumulation can be significant risks of ERW, but one study that posed this concern was focused on the use of metallurgical slags (Choi et al., 2021), while others have spoken of Ni and Cr present in olivine (Dupla et al., 2023). Yet, many of the minerals tested in the ERW field and laboratory experiments are free (i.e., below regulated levels) of metals of concern (Dudhaiya et al., 2019; Lewis et al., 2021). Evidently, this is a point that deserves caution, accounting, and ecotoxicity assessment (Vienne et al., 2022; Vink and Knops, 2023) but not a technological roadblock.
(iii) A recent study (Buckingham et al., 2022) suggested that a large extent of the UK’s croplands is too dry for ERW deployment, and hence, estimates for carbon drawdown potential for the UK should be lower than earlier estimates. West et al. (2023) suggested that the study’s conclusions were affected by large evapotranspiration from the rooftop soil columns and the omission of pedogenic carbonate detection (as also pointed to by Ali and Santos (2023)). In response, Buckingham et al. (2023) refuted these assertions but did not address some specific concerns raised by West et al. (2023) about evapotranspiration and did not provide calcimetry data on the soils to accurately quantify soil inorganic carbon accumulation. This exchange exemplifies how inconsistencies in experimental design and the lack of more distributed studies can act as roadblocks when the reported data are extrapolated to making general recommendations, calling for careful attention to experimental design and data processing.
3.4 Shortcuts for ERW
Shortcuts serve many purposes: they shorten the journey; they help circumvent roadblocks; and when in the form of a bridge, they help overcome a gap.
3.4.1 Fast weathering
A couple of ways to overcome roadblocks 3.3.1 and 3.3.2 have been: 1) to use faster-weathering minerals (such as wollastonite, slags, or concrete residues) (Renforth et al., 2009) and 2) to perform experiments in more amiable climates (e.g., Brazil and Malaysia) (Silva et al., 2021; Larkin et al., 2022). Such strategies have worked and should remain a key means of accelerating ERW understanding, deployment, and risk and impact analysis. However, shortcuts can also create traffic jams, themselves becoming roadblocks (e.g., exclusive focus on 2), so other pathways should be explored alongside.
3.4.2 Independent users
Farmers have used rock dust and other mineral amendments for decades and, in some cases, have used the same rocks and minerals being tested for ERW. An evident shortcut to generating more robust multi-year data, previewing long-term impacts, and looking for evidence of past weathering is to visit these locations to collect soil and water samples for study. Few publications have touched on this strategy (Haque et al., 2020; Taylor et al., 2021), possibly due to the challenge of forensic MRV versus forward-looking MRV, so opportunities remain.
3.4.3 Stick to basics
A closing thought on the complexities of MRV is whether there is the absolute need for such complexities and to what extent. It is inherently difficult to quantify the ERW rate and extent under field conditions, considering the workload involved in collecting representative soils, waters (and even gases), and the uncertainties involved in their analyses and data interpretation. Sticking to basics is a reminder that at a very fundamental level, ERW should work on most croplands, to an extent, as it consists of exposing silicate minerals to conditions in which they are likely to weather much faster than if left to natural geological processes. The following questions are raised:
• Should farmers be rewarded for participating in ERW based on mineral application (respecting agronomic limits that farmers should self-impose), or based on carbon drawdown? One of these is bound to be more equitable and the other more profitable.
• Is the complexity of MRV based on physical sampling worth it, or are models and even simpler guidelines sufficient to regulate the practice of ERW and issue carbon certificates?
• At what point does sampling-based MRV give way to trust in ERW as a conventional agricultural practice: 1) when climate change models signal that ERW is doing its job; 2) when the rest of the economy is sufficiently decarbonized; 3) or will MRV become such a profitable endeavor that it outlives its need?
It is important to answer these questions from the point of view of the global and multi-decadal potential that ERW has and not only what scientists and early startups need to confirm the science. Agriculture has been historically based on trust in science to a great extent, so it remains to be seen if ERW will find its place as a mainstream agricultural technology in addition to being a geoscience technology.
Author contributions
RS, FH, and YC contributed to the conception and design of the study. RS wrote the first draft of the manuscript. FA, HJ, and RK wrote sections of the manuscript. All authors contributed to the article and approved the submitted version.
Funding
The authors acknowledge the financial support provided by the Ontario Agri-Food Innovation Alliance (Gryphon’s LAAIR Product Development grant UG-GLPD-2021-101200).
Conflict of interest
The research conducted at the University of Guelph on ERW from 2017 to 2023 was conducted in the absence of any commercial or financial relationships that could be construed as a potential conflict of interest. Since December 2022, RMS is non-remunerated Scientific Advisor of Everest Carbon, a company commercializing ERW technologies and carbon credits; this opinion article does not report any information linked to this company and is not meant in any way to exclusively benefit this company.
Publisher’s note
All claims expressed in this article are solely those of the authors and do not necessarily represent those of their affiliated organizations, or those of the publisher, the editors, and the reviewers. Any product that may be evaluated in this article, or claim that may be made by its manufacturer, is not guaranteed or endorsed by the publisher.
References
Ali, A., and Santos, R. (2023). Review of: "Soil core study indicates limited CO2 removal by enhanced weathering in dry croplands in the UK. Qeios. doi:10.32388/LMT1BU
Almaraz, M., Bingham, N. L., Holzer, I. O., Geoghegan, E. K., Goertzen, H., Sohng, J., et al. (2022). Methods for determining the CO2 removal capacity of enhanced weathering in agronomic settings. Front. Clim. 4, 970429. doi:10.3389/fclim.2022.970429
Amann, T., and Hartmann, J. (2022). Carbon accounting for enhanced weathering. Front. Clim. 4, 849948. doi:10.3389/fclim.2022.849948
Amoakwah, E., Shim, J., Kim, S., Lee, Y., Kwon, S., Sangho, J., et al. (2023). Impact of silicate and lime application on soil fertility and temporal changes in soil properties and carbon stocks in a temperate ecosystem. Geoderma 433, 116431. doi:10.1016/j.geoderma.2023.116431
André, F. J., and Valenciano-Salazar, J. A. (2022). Voluntary carbon neutral programs. Adoption and firms’ strategies. J. Clean. Prod. 381 (1), 135191. doi:10.1016/j.jclepro.2022.135191
Wire, B. (2022). Lithos decarbonizes farmland, the Foundation of the World’s Food Supply, While Scaling Carbon Removal and Improving Soil Health and Crop Yield. Available online: https://www.businesswire.com/news/home/20221020005311/en/Lithos-Decarbonizes-Farmland-the-Foundation-of-the-World%E2%80%99s-Food-Supply-While-Scaling-Carbon-Removal-and-Improving-Soil-Health-and-Crop-Yield (accessed 2023-March-02).
Bai, Y., and Cotrufo, M. F. (2022). Grassland soil carbon sequestration: Current understanding, challenges, and solutions. Science 377, 603–608. doi:10.1126/science.abo2380
Beerling, D. J. (2017). Enhanced rock weathering: Biological climate change mitigation with co-benefits for food security? Biol. Lett. 13, 20170149. doi:10.1098/rsbl.2017.0149
Buckingham, F. L., Henderson, G. M., Holdship, P., and Renforth, P. (2022). Soil core study indicates limited CO2 removal by enhanced weathering in dry croplands in the UK. Appl. Geochem. 147, 105482. doi:10.1016/j.apgeochem.2022.105482
Buckingham, F. L., Henderson, G. M., and Renforth, P. (2023). Response to Comment from West et al. on, Soil core study indicates limited CO2 removal by enhanced weathering in dry croplands in the UK. Appl. Geochem. 152, 105622. doi:10.1016/j.apgeochem.2023.105622
Bullock, L. A., James, R. H., Matter, J., Renforth, P., and Teagle, D. A. H. (2021). Global carbon dioxide removal potential of waste materials from metal and diamond mining. Front. Clim. 3, 694175. doi:10.3389/fclim.2021.694175
Bullock, L. A., Yang, A., and Darton, R. C. (2022). Kinetics-informed global assessment of mine tailings for CO2 removal. Sci. Total Environ. 808, 152111. doi:10.1016/j.scitotenv.2021.152111
CarbonPlan (2022). CDR verification network – enhanced Weathering v1.1. Available online: https://carbonplan.org/research/cdr-verification/enhanced-weathering (accessed March 2023, 20).
Choi, W.-J., Park, H.-J., Cai, Y., and Chang, S. X. (2021). Environmental risks in atmospheric CO2 removal using enhanced rock weathering are overlooked. Environ. Sci. Technol. 55 (14), 9627–9629. doi:10.1021/acs.est.1c02505
Dudhaiya, A., Haque, F., Fantucci, H., and Santos, R. M. (2019). Characterization of physically fractionated wollastonite-amended agricultural soils. Minerals 9 (10), 635. doi:10.3390/min9100635
Dupla, X., Möller, B., Baveye, P. C., and Grand, S. (2023). Potential accumulation of toxic trace elements in soils during enhanced rock weathering. Eur. J. Soil Sci. 74 (1), e13343. doi:10.1111/ejss.13343
Ellwood, P., Williams, C., and Egan, J. (2022). Crossing the valley of death: Five underlying innovation processes. Technovation 109, 102162. doi:10.1016/j.technovation.2020.102162
Eufrasio, R. M., Kantzas, E. P., Edwards, N. R., Holden, P. B., Pollitt, H., Mercure, J.-F., et al. (2022). Environmental and health impacts of atmospheric CO2 removal by enhanced rock weathering depend on nations’ energy mix. Commun. Earth Environ. 3, 106. doi:10.1038/s43247-022-00436-3
Frontiersgaps.com (2023). Carbon removal knowledge gaps. Available online: https://frontiergaps.softr.app/ (accessed 2023-March-15)
Georgakopoulos, E., Santos, R. M., Chiang, Y. W., and Manovic, V. (2016). Influence of process parameters on carbonation rate and conversion of steelmaking slags – introduction of the ‘carbonation weathering rate. Greenh. Gases Sci. Technol. 6 (4), 470–491. doi:10.1002/ghg.1608
Gomez-Casanovas, N., Blanc-Betes, E., Moore, C. E., Bernacchi, C. J., Kantola, I., and DeLucia, E. H. (2021). A review of transformative strategies for climate mitigation by grasslands. Sci. Total Environ. 799, 149466. doi:10.1016/j.scitotenv.2021.149466
Grip workshop (2023). Livestock meeting focuses on getting research from the lab to the farm. https://livestockresearch.ca/article/livestock_meeting_focuses_on_getting_research_from_the_lab_to_the_farm (accessed March 2023, 20).
Haque, F., Santos, R. M., and Chiang, Y. W. (2020). CO2 sequestration by wollastonite-amended agricultural soils – An Ontario field study. Int. J. Greenh. Gas Control 97, 103017. doi:10.1016/j.ijggc.2020.103017
Haque, F., Santos, R. M., Dutta, A., Thimmanagari, M., and Chiang, Y. W. (2019). Co-Benefits of wollastonite weathering in agriculture: CO2 sequestration and promoted plant growth. ACS Omega 4 (1), 1425–1433. doi:10.1021/acsomega.8b02477
Hicks, A., Dholabhai, P. P., Ali, A., and Santos, R. M. (2022). Nutrient-doped synthetic silicates for enhanced weathering, remineralization and fertilization on agricultural lands of global cold regions – A perspective on the research ahead. iScience 25 (12), 105556. doi:10.1016/j.isci.2022.105556
Institute for Carbon Removal Law and Policy (2023). Enhanced rock weathering in the global South. Available online: https://youtu.be/kWdT7dlrpQ8 (accessed March 2023, 02).
Jariwala, H., Haque, F., Vanderburgt, S., Santos, R. M., and Chiang, Y. W. (2022). Mineral–soil–plant–nutrient synergisms of enhanced weathering for agriculture: Short-term investigations using fast-weathering wollastonite skarn. Front. Plant Sci. 13, 929457. doi:10.3389/fpls.2022.929457
Kelland, M. E., Wade, P. W., Lewis, A. L., Taylor, L. L., Sarkar, B., Andrews, M. G., et al. (2020). Increased yield and CO2 sequestration potential with the C4 cereal Sorghum bicolor cultivated in basaltic rock dust-amended agricultural soil. Glob. Change Biol. 26, 3658–3676. doi:10.1111/gcb.15089
Khalidy, R., Arnaud, E., and Santos, R. M. (2022). Natural and human-induced factors on the accumulation and migration of pedogenic carbonate in soil: A review. Land 11 (9), 1448. doi:10.3390/land11091448
Köhler, P., Hartmann, J., and Wolf-Gladrow, D. A. (2011). Reply to Schuiling et al.: Different processes at work. Proc. Natl. Acad. Sci. 108 (12), E42. doi:10.1073/pnas.1019468108
Larkin, C. S., Andrews, M. G., Pearce, C. R., Yeong, K. L., Beerling, D. J., Bellamy, J., et al. (2022). Quantification of CO2 removal in a large-scale enhanced weathering field trial on an oil palm plantation in Sabah, Malaysia. Front. Clim. 4, 959229. doi:10.3389/fclim.2022.959229
Lenton, T. M., and Britton, C. (2006). Enhanced carbonate and silicate weathering accelerates recovery from fossil fuel CO2 perturbations. Glob. Biogeochem. Cycles 20, GB3009. doi:10.1029/2005GB002678
Lewis, A. L., Sarkar, B., Wade, P., Kemp, S. J., Hodson, M. E., Taylor, L. L., et al. (2021). Effects of mineralogy, chemistry and physical properties of basalts on carbon capture potential and plant-nutrient element release via enhanced weathering. Appl. Geochem. 132, 105023. doi:10.1016/j.apgeochem.2021.105023
Manning, D. A. C., Renforth, P., Lopez-Capel, E., Robertson, S., and Ghazireh, N. (2013). Carbonate precipitation in artificial soils produced from basaltic quarry fines and composts: An opportunity for passive carbon sequestration. Int. J. Greenh. Gas Control 17, 309–317. doi:10.1016/j.ijggc.2013.05.012
Organic Materials Review Institute (2015). Canadian wollastonite calcium silicate soil amendment. Available online: https://www.omri.org/mfg/cwt/certificate/5601 (accessed March 2023, 02).
Paradelo, R., Virto, I., and Chenu, C. (2015). Net effect of liming on soil organic carbon stocks: A review. Agric. Ecosyst. Environ. 202, 98–107. doi:10.1016/j.agee.2015.01.005
Paulo, C., Power, I. M., Stubbs, A. R., Wang, B., Zeyen, N., and Wilson, S. A. (2021). Evaluating feedstocks for carbon dioxide removal by enhanced rock weathering and CO2 mineralization. Appl. Geochem. 129, 104955. doi:10.1016/j.apgeochem.2021.104955
Power, I., and Southam, G. (2005). Carbon dioxide sequestration through enhanced weathering of chrysotile mine tailings and subsequent microbial precipitation of magnesium carbonates. Geochimica Cosmochimica Acta 69 (S10), A834. doi:10.1016/j.gca.2005.03.038
Puro.earth (2022). Enhanced rock weathering methodology. Available online: https://puro.earth/articles/enhanced-rock-weathering-in-soil-methodology-public-consulta-788 (accessed March 2023, 02).
Reid, K., Bruulsema, T., Verhallen, A., and Kessel, C. (2006). Soil fertility handbook. OMAFRA Publication 611. Available online: https://files.ontario.ca/omafra-soil-fertility-handbook-en-2022-10-13.pdf (accessed March 2023, 02).
Renforth, P., Manning, D. A. C., and Lopez-Capel, E. (2009). Carbonate precipitation in artificial soils as a sink for atmospheric carbon dioxide. Appl. Geochem. 24, 1757–1764. doi:10.1016/j.apgeochem.2009.05.005
Schuiling, R. D., Wilson, S. A., and Power, I. M. (2011). Enhanced silicate weathering is not limited by silicic acid saturation. Proc. Natl. Acad. Sci. U. S. A. 108, E41–E42. doi:10.1073/pnas.1019024108
Silva, R. C., Ferreira, E. P., and de Azevedo, A. C. (2021). Weathering features of a remineralizer in soil under different land uses. Pesqui. Agropecuária Bras. 56, e01442. doi:10.1590/S1678-3921.pab2021.v56.01442
Swoboda, P., Döring, T. F., and Hamer, M. (2022). Remineralizing soils? The agricultural usage of silicate rock powders: A review. Sci. Total Environ. 807 (3), 150976. doi:10.1016/j.scitotenv.2021.150976
Taylor, L. L., Driscoll, C. T., Groffman, P. M., Rau, G. H., Blum, J. D., and Beerling, D. J. (2021). Increased carbon capture by a silicate-treated forested watershed affected by acid deposition. Biogeosciences 18, 169–188. doi:10.5194/bg-18-169-2021
Taylor, L., Quirk, J., Thorley, R., Kharecha, P. A., Hansen, J., Ridgwell, A., et al. (2016). Enhanced weathering strategies for stabilizing climate and averting ocean acidification. Nat. Clim. Change 6, 402–406. doi:10.1038/nclimate2882
THRIVE (2023). THRIVE | Shell – climate-smart agriculture challenge. Available online: https://thriveagrifood.com/shell-challenge/ (accessed March 2023, 02).
UNDO (2022). Chris Zair’s post. Available online: https://www.linkedin.com/posts/chris-zair-80515829_as-the-ancient-chinese-proverb-goes-the-activity-6971066284541116416-yP6x?utm_source=share&utm_medium=member_desktop (accessed March 2023, 02).
Valadão, W. S. (2017). Estrada do Atalho, riacho. Available online: https://commons.wikimedia.org/wiki/File:Estrada_do_Atalho,_riacho.jpg (accessed July 2023, 10).
van Straaten, P. (2007). Agrogeology: The use of rocks for crops. Available online: https://www.researchgate.net/publication/328392285 (accessed March 2023, 02).
VERRA (2022). Enhanced weathering and mineralization advisory group—invitation to apply. Available online: https://verra.org/enhanced-weathering-and-mineralization-advisory-group-invitation-to-apply/ (accessed March 2023, 02).
Vienne, A., Poblador, S., Portillo-Estrada, M., Hartmann, J., Ijiehon, S., Wade, P., et al. (2022). Enhanced weathering using basalt rock powder: Carbon sequestration, Co-benefits and risks in a mesocosm study with Solanum tuberosum. Front. Clim. 4, 869456. doi:10.3389/fclim.2022.869456
Vink, J. P. M., and Knops, P. (2023). Size-Fractionated weathering of olivine, its CO2-sequestration rate, and ecotoxicological risk assessment of nickel release. Minerals 13, 235. doi:10.3390/min13020235
West, L. J., Banwart, S. A., Martin, M. V., Kantzas, E., and Beerling, D. J. (2023). Making mistakes in estimating the CO2 sequestration potential of UK croplands with enhanced weathering. Appl. Geochem. 151, 105591. doi:10.1016/j.apgeochem.2023.105591
West, T. O., and McBride, A. C. (2005). The contribution of agricultural lime to carbon dioxide emissions in the United States: Dissolution, transport, and net emissions. Agric. Ecosyst. Environ. 108 (2), 145–154. doi:10.1016/j.agee.2005.01.002
Yan, Y., Dong, X., Li, R., Zhang, Y., Yan, S., Guan, X., et al. (2023). Wollastonite addition stimulates soil organic carbon mineralization: Evidences from 12 land-use types in subtropical China. Catena 225 (3), 107031. doi:10.1016/j.catena.2023.107031
Keywords: enhanced weathering, soil amendment, silicate minerals, carbonates, alkalinity, crop health, carbon sequestration, soil inorganic carbon
Citation: Santos RM, Araujo F, Jariwala H, Khalidy R, Haque F and Chiang YW (2023) Pathways, roundabouts, roadblocks, and shortcuts to safe and sustainable deployment of enhanced rock weathering in agriculture. Front. Earth Sci. 11:1215930. doi: 10.3389/feart.2023.1215930
Received: 02 May 2023; Accepted: 17 July 2023;
Published: 01 August 2023.
Edited by:
Shuang Zhang, Texas A and M University, United StatesReviewed by:
Phil Renforth, Heriot-Watt University, United KingdomSalvatore Calabrese, Texas A and M University, United States
Copyright © 2023 Santos, Araujo, Jariwala, Khalidy, Haque and Chiang. This is an open-access article distributed under the terms of the Creative Commons Attribution License (CC BY). The use, distribution or reproduction in other forums is permitted, provided the original author(s) and the copyright owner(s) are credited and that the original publication in this journal is cited, in accordance with accepted academic practice. No use, distribution or reproduction is permitted which does not comply with these terms.
*Correspondence: Rafael M. Santos, c2FudG9zckB1b2d1ZWxwaC5jYQ==; Yi Wai Chiang, Y2hpYW5nZUB1b2d1ZWxwaC5jYQ==