- 1Curt-Engelhorn-Zentrum Archäometrie, Mannheim, Germany
- 2Department of Cultural Studies and Antiquities, Ludwig Maximilians University, München, Germany
- 3Institute of Prehistory, Early History and Medieval Archaeology, Eberhard Karls University, Tübingen, Germany
Tin was a crucial commodity in prehistory to produce bronze, and knowledge of the origins of this metal is important for understanding cultural relations and the complexity and extent of trade. However, many aspects of the provenance of tin are still not resolved. A recent study in Science Advances 8(48) examined the historically significant tin ingots from the Uluburun shipwreck, which are key to the economy and long-distance trade of tin in the Late Bronze Age Mediterranean and beyond. Isotopic and chemical data of the objects was collected, from which a tin origin from Central Asia, particularly Mushiston in Tajikistan, and Anatolia was reconstructed. The study thereby proposed a solution to the long-standing riddle of tin provenance via scientific reasoning and comparative data. While this avenue of investigation is intriguing, this article maintains that the authors’ arguments do not support their far-reaching conclusions. Instead, it emphasises the similarities with Late and Middle Bronze Age tin ingots from Israel and Britain, and alternatively suggests a common origin of part of the Uluburun cargo with these items. South-west England is considered a very likely source region, but other tin ingots of the Uluburun wreck could also originate from Afghanistan and perhaps somewhere else.
Introduction
Bronze was the earliest alloy to dominate an entire era of human history. It consists of two metals: Copper and tin. Both components occur as ores in natural deposits, but compared to the almost ubiquitous copper, tin is much rarer and inhomogeneously distributed throughout Eurasia. Regions rich in tin ores contrast with regions devoid of them (Taylor, 1982; Penhallurick, 1986). These inequalities in the availability of highly coveted raw tin stimulated extensive communication between human populations since the Bronze Age, resulting in the establishment of far-reaching trade networks over hundreds, and even thousands, of kilometres. Yet, current understandings of the underlying processes are hindered by a crucial problem: Although tin deposits are known to us, we have little evidence nor robust proof of exactly which sources were used to produce Bronze Age tin and bronze. This is the core of the “tin mystery” as it has been discussed for more than 150 years (von Baer, 1876; Muhly, 1985; Maddin, 1998).
A solution for the “tin mystery” has been long sought out by historians, archaeologists, and natural scientists alike. Generations of scholars from the various disciplines have tried to illuminate the origin of tin from different perspectives. The Middle East in particular was a focal point of investigation, where both the earliest widespread use of bronze is attested in the late 4th and 3rd millennia BCE, and cuneiform texts from Mesopotamia and Anatolia (Mari, Kültepe) offer detailed insight into the mechanisms of metal trade (Dercksen, 2001; Dercksen, 2005; Barjamovic et al., 2012; Pigott, 2021). Early 2nd millennium BCE economic documents make clear that large amounts of tin were traded from south-western Iran (Elam) through Mesopotamia to central Anatolia during this period, one of the earliest interregional trade routes known. Yet, neither archaeologists, philologists, nor natural scientists, have succeded in pinpointing the ultimate origin of Bronze Age tin, and thus, a lively discussion has continued for more than three decades, with source areas suggested from Anatolia, the central Zagros, all the way to Middle Asia (Muhly, 1985; Stech and Pigott, 1986; Reiter, 1997; Yener, 2009). It is noteworthy in this regard that scientific reasoning has been so far inconclusive and the available analytical proxies have not yet provided satisfactory answers.
Great hope was thus pinned on tin isotope analysis as a provenance tool since it was believed that the isotopic signatures of tin could accurately determine where the metal was once mined. However, extensive research over the past decade has revealed that tin isotopes are not a magic bullet to solve the problem (Haustein et al., 2010; Haustein, 2013; Mason et al., 2016; Berger et al., 2019b; Nessel et al., 2019). The variations in the isotopic values of tin ores in tin deposits are large, but what matters more is the large overlap between deposits. This means that there is never an unambiguous answer to the question of tin provenance using tin isotopes alone. Yet, by combining the tin isotope composition with the isotopic composition of lead and the concentration of trace elements, the sources of tin can be narrowed down with much higher probability. Adapting this approach from previous studies (Rapp et al., 1999; Molofsky et al., 2014; Berger et al., 2019b; Berger et al., 2022b), Powell et al., (2022) recently investigated the famous tin ingots from the Uluburun shipwreck, which sank off the Turkish coast in the Late Bronze Age (LBA) shortly before 1,320 BCE (Pulak, 1998; Pulak, 2009; Stos-Gale et al., 1998; Yalçin et al., 2005). It carried by far the largest assemblage of metallic tin from Bronze Age contexts, exported together with copper, glass and other commodities to an unknown destination. In view of the sociocultural importance of the find, there has been no lack of attempts to uncover the origin of the tin with scientific methods, yet so far with unsatisfactory results (Stos-Gale et al., 1998; Pulak, 2005; Berger et al., 2019b). Now, Powell et al. (2022) suggest to have found the solution to the “tin mystery”. On the basis of scientific data and archaeological evidence, the authors postulate an origin of the Uluburun tin from Central Asian tin deposits on the one hand (e.g., Mushiston) and from Anatolian ores on the other (e.g., Kestel and Hisarcık). However, the authors’ argumentation is built on a number of misunderstandings, misinterpretations, unbalanced arguments and unsubstantiated claims, unfortunately leading the reader to the misbelief that the origin of the tin was unequivocally identified. This paper aims to point out the shortcomings of Powell et al.’s reasoning, and to provide a more balanced and alternative interpretation of the tin provenance.
Tin from Central Asian tin sources
Original materials and methods
A total of 105 tin ingots from Uluburun were included in the study of Powell et al. (2022) representing almost 91% of the ship’s tin cargo (Pulak, 1998; Pulak, 2005; Yalçin et al., 2005). This set of objects comprised ingots of different morphologies: complete or fragmented oxhide ingots, bun ingots, rectanguloid or slab ingots, anchor-shaped ingots, as well as loaf-shaped ingots. In addition to these artefacts, the authors examined eleven cassiterite crystals from the Pamir Mountains of Pakistan and Afghanistan, and a further six samples from Kestel and Hisarcık in Anatolia for comparative purposes. The cassiterite was analysed by tin isotope analysis after thermal decomposition, while tin isotope and trace element analysis were used to characterise the artefacts. Lead isotope analysis was also applied to the tin, the data of which was partly obtained in Powell et al. (2022) and Powell et al. (2021a) by MC-ICP-MS, but mainly much earlier by Stos-Gale et al. (1998) using less accurate thermal ionisation mass spectrometry, TIMS (Stos-Gale and Gale, 2009). The latter (Stos-Gale et al., 1998) is not cited by Powell et al. (2022), even though the data is used by them and represents the basis of their reasoning. 206Pb/204Pb, 208Pb/204Pb, δ124Sn (=δ124Sn/116Sn relative to NIST SRM 3161a) and the lead concentrations of the tin were subjected to statistical evaluation with a t-SNE test (t-distributed stochastic neighbour embedding). Trace elements other than lead were omitted from the statistical evaluation.
Lead isotope ratios
Based on these statistics, two compositional groups, MC and P, with eight sub-groups were defined. The main groups are based on the isotope ratios of the lead in the tin, which the authors attribute to Late Paleozoic tin deposits on the one hand (group P), and to Mesozoic and Cenozoic (group MC) deposits on the other (Powell et al., 2021a; Powell et al., 2022). The resulting “Paleozoic ores group” encompasses 35 ingots with lead concentrations of up to 0.55% that distribute over the three sub-groups P1, P2A and P2B.
The age determination of the used tin ores by Powell et al. relies solely on 208Pb/204Pb. They write “[…] tin ingots analyzed yield 208Pb/204Pb values that are consistent with Late Paleozoic […] ores […]” (Powell et al., 2022, 2). Concerning tin deposits with cassiterite, the isotopic composition of lead can in fact be used for determining the formation age of the tin source [e.g., during the Paleozoic (541–252 Ma) or Mesozoic era (252–66 Ma)] when the cassiterite is dominated by radiogenic lead. In this case, either uranium/thorium-lead or lead-lead data allows the calculation of a so-called model age that relates to the formation age of the cassiterite in tin deposits (Faure and Mensing, 2005; Molofsky et al., 2014). The radiogenic signature can be transferred from the ore to the tin metal during smelting, which offers the possibility of linking metallic tin with tin sources. However, unlike tin ores, only lead-lead data is available for metal due to the exclusion of uranium and thorium from tin as they partition to the slag on smelting. The use of lead-lead data with radiogenic character for dating the parent tin ore of archaeological tin objects is meanwhile an established method in archaeometallurgy. It was first applied to prehistoric tin from South Africa (Molofsky et al., 2014), and in a multi-analytical approach to LBA and Middle Bronze Age (MBA) tin ingots from Israel and Salcombe, United Kingdom (Berger et al., 2019b; Berger et al., 2022b). The method rests upon isochrones of the uranogenic ratios 206Pb/204Pb and 207Pb/204Pb, from which the model age is calculated (Faure and Mensing, 2005; Molofsky et al., 2014). These isochrones are straight lines of all tin items that were made with tin from geologically coeval tin mineralisation. In case of the South African objects, a model age of ca. 2,050 Ma was obtained that matched the Proterozoic (2,500–541 Ma) tin deposits in South Africa (Molofsky et al., 2014), whereas the calculated 291 Ma of a set of ingots from Israel actually pointed to a Variscan (i.e., Late Paleozoic, 359–252 Ma) tin source (Berger et al., 2019b; Berger et al., 2022b). All tin objects that were produced from Late Paleozoic cassiterites should lie on the same or a close-by isochrone, since the method does not allow for discrimination between tin mineralisation of similar age. Thus, distinguishing Late Paleozoic tin deposits in Central Asia from Late Paleozoic deposits in Europe (Cornwall, Erzgebirge, Iberian Peninsula etc.) or elsewhere is principally not possible by means of 206Pb/204Pb and 207Pb/204Pb.
The ingots of group P1 by Powell et al. (2022) are actually characterised by portions of radiogenic lead (206Pb/204Pb > 19), but using this for inferring a Late Paleozoic formation age of the parental tin deposit is not feasible for the ingots in question based on the currently available data. Apart from three pieces (KW 0515, KW 1932; KW 4388), the lead isotope ratios of the majority of the Uluburun ingots of group P1 deviate from the “Variscan” isochrone of other LBA tin ingots (Figure 1A). As the data points of the Uluburun artefacts also do not establish a clear isochrone on their own, a model age calculation for these ingots and the conclusion of the formation age of the tin ore is not possible (Molofsky et al., 2014; Artioli et al., 2020). The deviation from the isochrone may have several reasons: First, the uranium concentration of the original and maybe Paleozoic cassiterites was too low to produce enough radiogenic lead (Artioli et al., 2020), or second, the lead isotope composition is a mixture of radiogenic and common lead, e.g., caused by contamination. Given the objects’ lead content of up to 0.22% (group P1), there is some probability for contamination, but the lower accuracy of the ingots’ TIMS data must also be kept in mind. Recent re-analysis of several Uluburun ingots with low lead concentrations (<100 μg g–1) using more precise MC-ICP-MS has refined the earlier results, revealing a good correlation with the “Variscan” isochrone (https://www.facebook.com/Matextv/videos/festival-della-civilt%C3%A0-nuragica-10-11-settembre-comune-di-orroli-parte-2/255356313131449; lecture by M. Jansen). Even though these new results are not yet published, it is expected that at least part of the P1 ingots can actually be traced to Variscan tin deposits, and the findings of Powell et al. (2022) might principally be true. However, their interpretation of the formation age and the tin origin relies on 208Pb/204Pb vs. 206Pb/204Pb instead of 206Pb/204Pb vs. 207Pb/204Pb, which is problematic in several respects. No isochrones are obtained in this combination of ratios, but particularly, their comparison of the Uluburun tin with sulphide ores of copper, lead and silver from Eurasia [in Powell et al. (2021a)] is not at all useful, as the lead isotope composition of sulphides is not readily comparable with that of cassiterite. As suggested by Artioli et al. (2020), 208Pb/204Pb can eventually be used for the discrimination of different source regions of tin, but there is still not enough data from cassiterites to substantiate this argument on statistical grounds. For all these reasons, chronological statements and the inference of a distinct Late Paleozoic source region based on 208Pb/204Pb alone are problematic or not feasible.
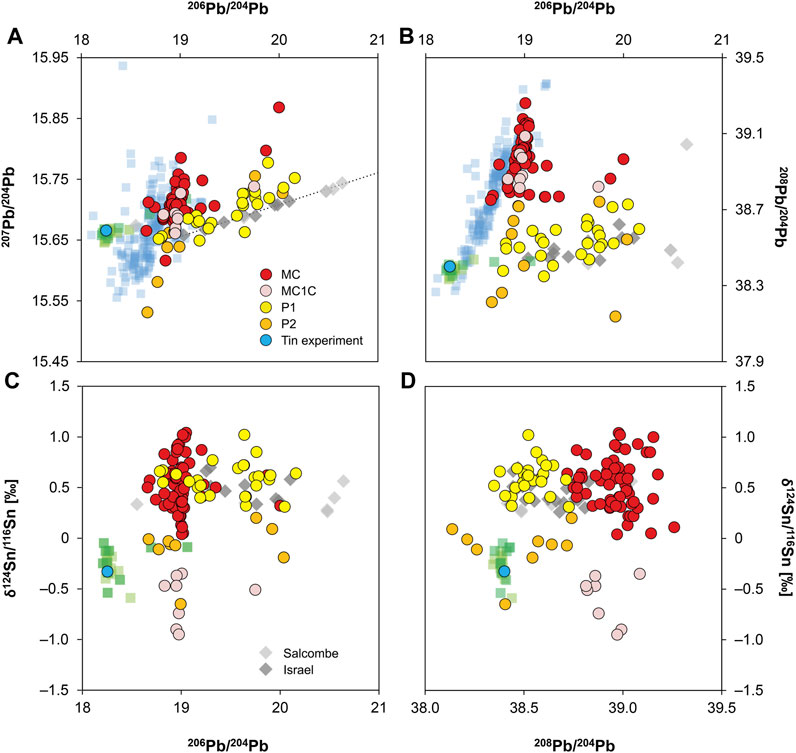
FIGURE 1. Lead and tin isotope systematics of the tin ingots from Uluburun, Salcombe and Israel. (A) 206Pb/204Pb vs. 207Pb/204Pb with isochrone (dotted line) of tin ingots from Israel and Salcombe; (B) 206Pb/204Pb vs. 208Pb/204Pb; (C) 206Pb/204Pb vs. δ124Sn/116Sn; (D) 208Pb/204Pb vs. δ124Sn/116Sn. Data from lead and copper ores from Anatolia (blue squares) and copper-tin ores from Mushiston (light green squares = secondary ores; dark green squares = stannite) for comparison. Data from the experimentally smelted tin from Mushiston as blue circle. Data of the Uluburun ingots from Stos-Gale et al. (1998), Powell et al. (2021a), Powell et al. (2022); data of other ingots from Berger et al. (2019b), Berger et al. (2022b) and Supplementary Table S1; data of experimentally smelted tin and Mushiston ores from Berger et al. (2022a) and Table 2; Anatolian ore data compiled from Seeliger et al. (1985), Wagner et al. (1989), Yener et al. (1991), Sayre et al. (2001). δ124Sn/116Sn values of artefacts and experimentally smelted tin have been corrected for fractionation on smelting by −0.2‰ (Berger et al., 2018) (diagrams: D. Berger).
The Mushiston deposit
Similar concerns apply to the ingots of the sub-groups P2A and P2B with up to 0.55% lead. These groups stand out by fractionation-corrected δ124Sn/116Sn values between −0.65 and +0.20‰, which amongst others are attributed to the tin ores of the Tajik Mushiston deposit by the statement: “The only documented Late Paleozoic tin sources with comparably low δ124Sn [=δ124Sn/116Sn] are the stannite and secondary cassiterite-bearing Mushiston deposit in Tajikistan and deposits in the eastern Altai region of Kazakhstan” (Powell et al., 2022, 4). However, this is only part of the story: The statement neglects the fact that Late Paleozoic tin deposits with comparably low tin isotope compositions also exist in Europe: in the Erzgebirge province, in Cornwall/Devon, and on the Iberian Peninsula (Figure 2) (Berger et al., 2019b; Berger et al., 2022b). The latter tin province was not even mentioned by Powell et al. although it is one of the most likely Variscan ore sources for prehistoric tin along with the Erzgebirge and the British Isles. Moreover, the consideration of the tin-bearing deposit of Mushiston in this context is somewhat surprising due to its polymetallic character with a paragenesis of copper and tin minerals. Stannite [Cu2Sn(Fe,Zn)S4] is the main primary ore, malachite, azurite, hydrostannates such as mushistonite [(Cu,Zn,Fe)Sn(OH)6], varlamoffite [(Sn,Fe)(O,OH)2] and vismirnovite [ZnSn(OH)6] are the dominating weathering products of stannite in the deposit’s huge oxidation zone (Ivanov et al., 1993; Alimov et al., 1998; Konopelko et al., 2022). Exploitation has so far been proven exclusively for the secondary ores by mining archaeological investigations for a time period from the end of the 3rd until the second half of the 2nd millennium BCE (Alimov et al., 1998; Garner, 2013; Garner, 2015). A slag from the second quarter of the 2nd millennium BCE documents the smelting of the ores in the immediate vicinity of the deposit and the direct production of bronze. The latter has been correlated with the Mushiston ores based on the isotopic and chemical compositions of the slag matrix and the enclosed bronze prills (Berger et al., 2022a; Berger et al., 2023). Furthermore, smelting experiments with the secondary ores showed that either copper, bronze or tin metal could be obtained (Figure 3), depending on the mineral mixture of the ore assemblage (Berger et al., 2022a). The extraction of tin metal from tin-dominated ores (cf. Figures 3B, C) is of particular interest for the argumentation of Powell et al., yet the authors overlooked the fact that no pure metal can be produced from the Mushiston ores, only impure tin. It collects all the elements present in the secondary ore. Hence, Mushiston tin contains iron, copper and arsenic in the percentage range and up to several thousand μg g–1 of zinc, silver, antimony, lead and bismuth (Table 1). Comparing the experimentally smelted tin with the Uluburun ingots of group P2, it becomes obvious that the concentrations of the same elements are three to five orders of magnitude lower in the ingots (Figures 4A–C). Thus, the trace elements of the P2 tin ingots, and also of all other Uluburun ingots, are by no means compatible with Mushiston.
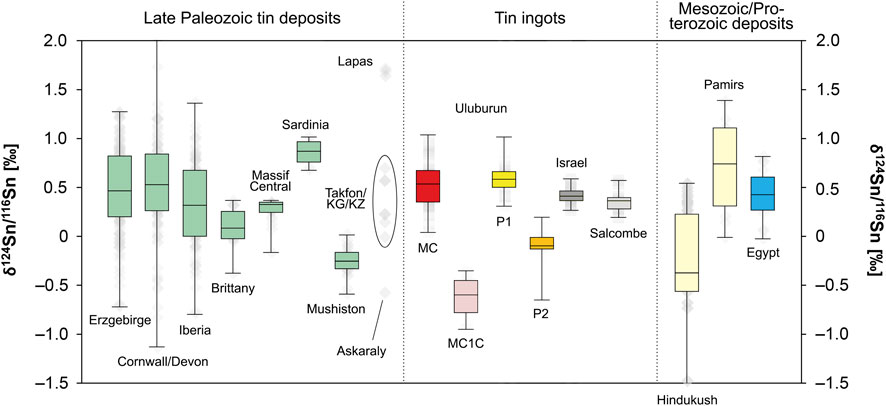
FIGURE 2. Comparison of δ124Sn/116Sn values of the tin ingots from Uluburun, Salcombe and Israel with tin ores from Europe (Erzgebirge, Cornwall/Devon, Iberia, Brittany, Massif Central, Sardinia), Central Asia [Mushiston, Lapas, Takfon, Afghanistan, Pamirs, Kyrgyzstan (KG), Askaraly, Kazakhstan (KZ)] and Egypt. Box-whisker-plots were calculated from published data of Mason et al. (2020), Berger et al. (2022a), Berger et al. (2023), data shown in Berger et al. (2019b) and Supplementary Table S1. Late Paleozoic ores are green, Mesozoic-Cenozoic are light yellow and Proterozoic are blue. δ124Sn/116Sn values of the artefacts from Berger et al. (2019b), Berger et al. (2022b), Powell et al. (2022) have been corrected for fractionation on smelting by −0.2‰ (Berger et al., 2018). Ingot group MC does contain ingots from group MC1C (diagram: D. Berger).
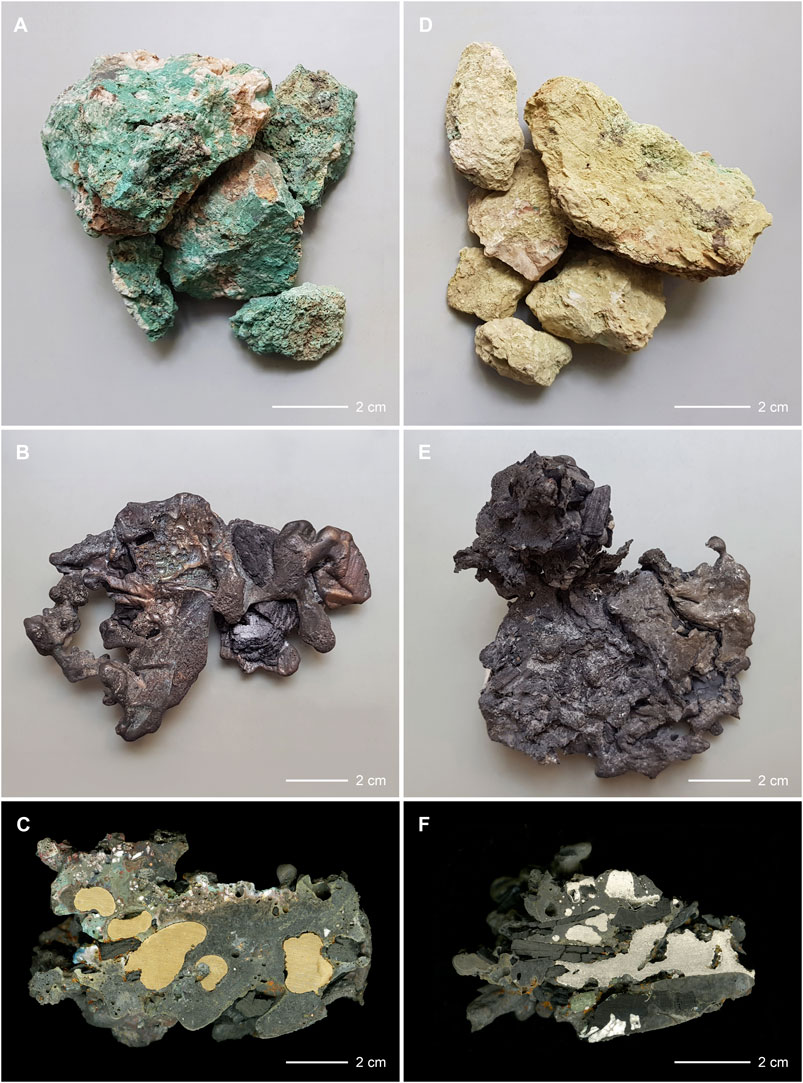
FIGURE 3. Raw materials and products from the smelting experiments carried out with the secondary ores from Mushiston. (A) Copper ore and (B) tin ore (MA-185278); (B, C) Bronze lump and corresponding slag from experimental co-smelting of copper and tin ores; (E, F) Tin and slag from smelting experiment with tin ore (photos: D. Berger).
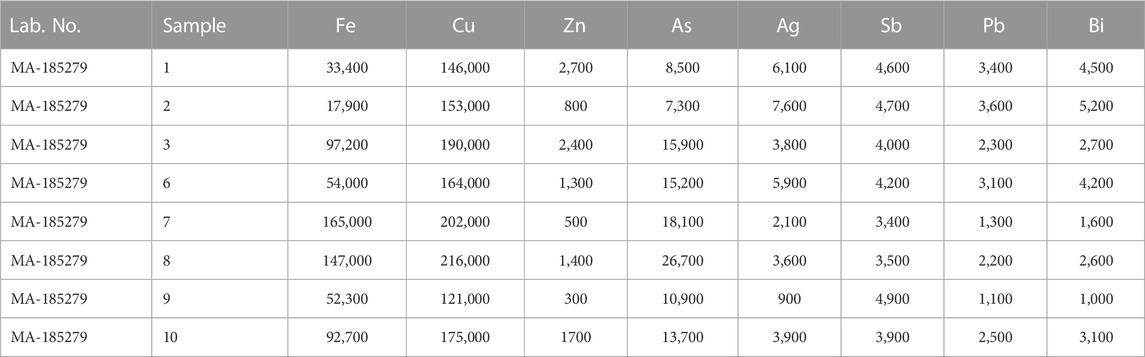
TABLE 1. Chemical composition by EDXRF in μg g–1 of the tin metal that was experimentally smelted from tin-dominated secondary ores from Mushiston (MA-185278); full data of results from Berger et al. (2022a).
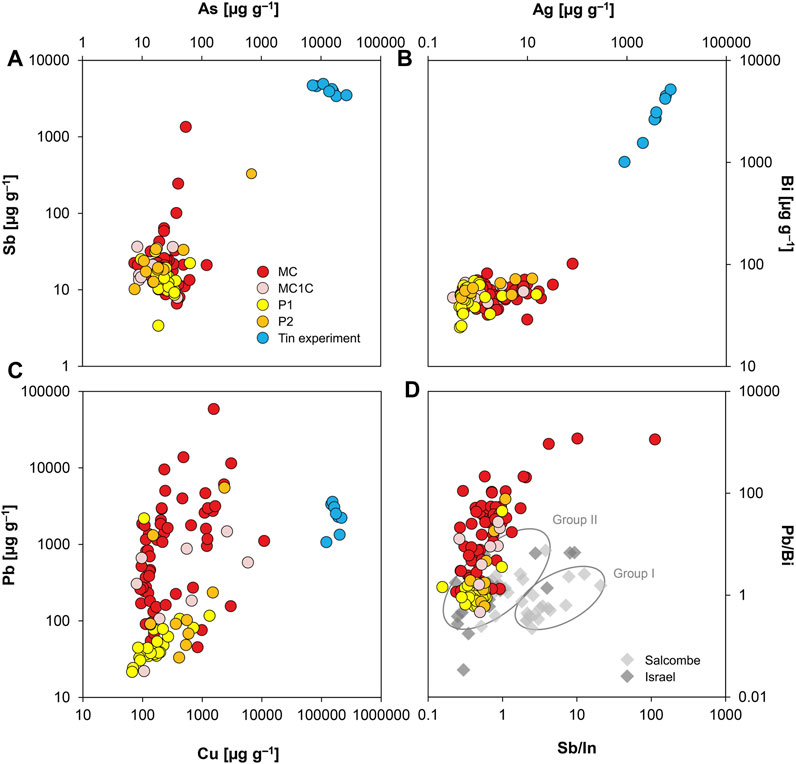
FIGURE 4. Chemical characteristics of the Uluburun tin ingots of the different groupings MC, MC1C, P1 and P2 as defined by Powell et al. (2022). (A) As–Sb; (B) Ag–Bi; (C) Cu–Pb. Shown as blue symbols is the chemical composition of the experimentally smelted tin from the secondary tin ores from Mushiston; (D) Comparison of Sb/In and Pb/Bi ratios of the Uluburun ingots and other LBA ingots from Salcombe, United Kingdom (Groups I and II), and Israel. Data of the Uluburun ingots from Powell et al. (2022); data of the other ingots from Berger et al. (2019b), Berger et al. (2022b); data of the experimentally smelted tin from Berger et al. (2022a) and Table 1 (diagrams: D. Berger).
Similar differences appear in the lead isotope ratios. Figures 1A, B illustrate that the secondary and primary ores from Mushiston are dominated by common lead signatures of Late Paleozoic age with significantly less radiogenic ratios than the Uluburun ingots, including those which were assigned to the “Paleozoic” ores group. Even by assuming dilution of the Mushiston signature by mixing with tin from other tin sources or the use of flux (see Berger et al., 2023), isotopic signatures similar to those in the Uluburun ingots could not be obtained with the ores from the polymetallic mine. The ore’s lead contents are too high [lead is enriched in the secondary tin ores (Berger et al., 2022a)] to have been overprinted by the signature of another tin source. Therefore, Mushiston must also be excluded due to the lead isotope composition. Both the lead isotope data and the results of the smelting experiments (see also Table 2; Figure 1) were published before the work of Powell et al. (Begemann and Schmitt-Strecker, 2009; Berger et al., 2022a), so the data was available. Nevertheless, both publications were ignored in the citation even though the tin isotope data of Mushiston ores from Berger et al. (2022a) was obviously used to calculate the box-whisker-plot in Figure 5 in Powell et al. (2022). Numerical values of the tin isotope composition of Mushiston have not been previously published.
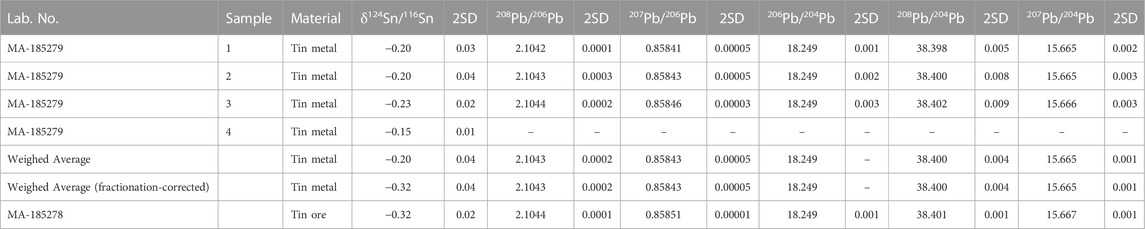
TABLE 2. Isotopic composition of the experimentally produced tin metal from Table 1 (MA-185279) and the tin ore used (MA-185278); full data of results from Berger et al. (2022a) supplemented by new lead isotope analyses of the tin and ore. δ124Sn/116Sn is reported relative to NIST SRM 3161a (data: G. Brügmann and B. Höppner, CEZA).
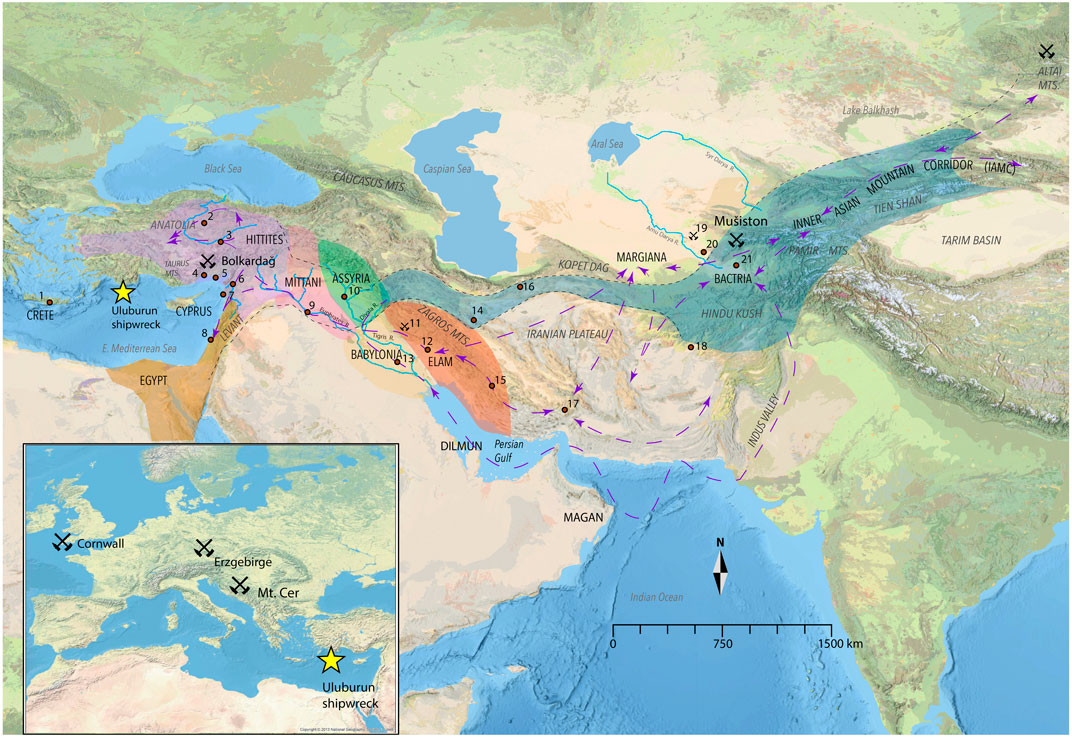
FIGURE 5. Original map of the Near East and Central Asia from Powell et al. (2022), (Figure 1) with an inset of Europe and the original caption: “Regional geography and main sites. 1, Hagia Triada; 2, Hattusa; 3, Hisarcik; 4, Mersin; 5, Tarsus; 6, Alalakh; 7, Ugarit; 8, Haifa; 9, Mari; 10, Assur; 11, Deh Hosein; 12, Susa; 13, Ur; 14, Arisman; 15, Tal-e Malyan; 16, Tepe Hissar; 17, Tepe Yahya; 18, Mundigak; 19, Karnab/Sichkonchi; 20, Sapalli; 21, Shortugai. Purple dashed arrows depict documented trade networks ca. 2200-1700 BCE. Blue shaded region reflects the corridor connecting the Anatolian and Central Asian/Middle Eastern tin trade (in blue), ca. 1600-1000 BCE. Other shaded areas represent key LBA polities. Inset map illustrates the location of ancient tin sources in Europe”.
Other sources—conclusion from tin isotopes and trace elements
The ingots of the “Paleozoic ores group” P1 are also interpreted as originating from Central Asian tin ores, again based on the lead isotope (208Pb/204Pb) signatures. δ124Sn/116Sn of the ingots show higher corrected values of 0.32‰–1.02‰ than group P2 (Figure 2), but within this range there is a match with a number of ore deposits, as the tin isotope composition is not a unique identifier: Late Paleozoic tin ores from Cornwall, the Erzgebirge, but also the Iberian Peninsula have been found with such values, as well as Mesozoic cassiterites in the Tajik Pamir Mountains (Figure 2). Powell et al. additionally present tin isotope data from the Tian Shan Mountain range with a similar variation; however, it is not clear from the sources cited where this data comes from. In Powell et al.’s (2022) study only data for Mesozoic cassiterites from Pakistan and Afghanistan was reported, complementing the isotopic dataset of Afghan and Tajik cassiterites in Berger et al. (2019b). To our knowledge, apart from a few analyses of tin ores from Lapas, Uzbekistan and Kyrgyzstan by our group (Berger et al., 2019b), no other analyses of Central Asian Paleozoic and Mesozoic tin ores have yet been published. Thus, building an argumentation on the few existing data sources lacks a sound statistical basis. Nevertheless, the authors attempt to corroborate their hypothesis by taking into account the trace element composition of the tin as an additional proxy. This approach has been used before (Berger et al., 2019b; Berger et al., 2022b) using lead, bismuth, indium and antimony as potential diagnostic elements (Rapp, 1978; Grant, 1991; Rapp et al., 1999; Haustein and Pernicka, 2011). Powell et al. (2022) use copper, silver, gold and tellurium in addition, the latter being taken as an argument for the higher probability for Central Asian ores. They refer to the elevated tellurium contents of the tin ingots, which should be consistent with mineralisation depths typical of Tajik ore deposits, and which make tin deposits in Europe, and Cornwall in particular, a less likely source for the Uluburun tin. Aside from insufficient records for this statement, it was shown by Haustein and Pernicka (2011) that cassiterite and smelted tin from the Erzgebirge contain appreciable amounts of tellurium. Thus, without systematically measuring the concentrations of tellurium and other trace elements in cassiterites from across Eurasia [only some datasets without tellurium are available for Central Asia (Pavlova et al., 2015)], neither exclusion of sources nor a verification of a distinct region is possible.
The discrepancy of the lead, bismuth, indium and antimony concentrations in the Uluburun tin with those in MBA/LBA tin ingots from Salcombe, for which English tin sources were suggested (Berger et al., 2022b), is another indication against a European source for Powell et al. (2022). However, this is again only part of the story: The Salcombe tin ingots can be divided into two groups. Plotting the elemental data of the Salcombe tin together with the Uluburun ingot groups P1 and P2, a very good match with Salcombe group II as defined in Berger et al. (2022b) is observed (Figure 4D). Strikingly, most of the LBA tin ingots found off the Israeli coast can be assigned to this group (Figure 4D) (Berger et al., 2019b). Based on the chemical data of the Uluburun material analysed by Powell et al., there seem thus to be more similarities with these MBA/LBA tin ingots than differences, not least as there is also no fundamental difference regarding the tin isotope composition (Figures 1C, D; Figure 2): Salcombe: 0.18‰–0.56‰; Israel: 0.26‰–0.58‰ corrected δ124Sn/116Sn (Berger et al., 2019b; Berger et al., 2022b) (Supplementary Table S1). So far, only the lead isotope ratios of most of the Uluburun ingots of group P1 deviate from the isochrone established for Salcombe and Israel (Berger et al., 2019b; Berger et al., 2022b) (Figure 1A). However, as mentioned above, unpublished results of the re-analysis of a series of Uluburun ingots suggest an allocation to the Variscan 206Pb/204Pb-207Pb/204Pb isochrone, and 208Pb/204Pb is anyway very similar to the ratios of the ingots from Salcombe and Israel (Figure 1B). Thus, it is not too far-fetched to assume an origin of part of the Uluburun cargo and the other ingots from the same region.
If the trace element patterns of the Uluburun tin ingots should indeed suggest a placer origin, as the authors claim without any reference [“High Au, low Cu, Ag, and Pb and incorporation of radiogenic 206Pb suggest a placer origin” (Powell et al., 2022, 4)], then this would not be an argument for, but rather against the least distant Central Asian Late Paleozoic ores. The only Variscan and demonstrably prehistorically exploited tin deposits of Uzbekistan at Karnab, Lapas and Changali are not alluvial deposits (Alimov et al., 1998; Weisgerber, 2009; Garner, 2013, 20), and neither is the Late Paleozoic Mushiston (Konopelko et al., 2022). For these sources, only eluvial parts are possible because of strong erosion. Accordingly, the ingots of group P2, for which an alluvial source is considered by Powell et al. (2022), cannot be related to the non-alluvial deposit Mushiston if their assumption would be correct. Finally, gold is no useful discriminator for placer tin since gold is ubiquitous in cassiterite mineralisation, whether primary or alluvial (Rapp, 1978; Dube, 2006). This was explicitly pointed out in another study by the authors, where the complex and non-alluvial iron-arsenic-tin mineralisation of Kestel was found to contain traces of gold (Powell et al., 2021b). In light of all these points, European tin deposits can certainly not be ruled out analytically as sources for the tin ingots of group P from Uluburun, as Powell et al. claim. In fact, given the available data and the similarities with other tin ingots [also highlighted by (Stos-Gale et al., 1998)], European deposits are currently the more likely sources, although an origin from eastern tin deposits cannot be excluded given the to date rudimentary database of cassiterite analyses. To resolve this uncertainty, chemical and lead isotope data of tin ores across Eurasia is urgently needed. ‘Project Tin’, initiated by British researchers, is currently making progress, at least for European tin deposits (https://projectancienttin.wordpress.com/).
Anatolian tin sources
Significance of the lead isotope ratios
The majority of the Uluburun cargo, consisting of 68 ingots, belongs to the lead-rich group MC (with sub-groups MC1A, MC1B, MC1C, MC2A, MC2B), which is interpreted as “Mesozoic-Cenozoic ore group”. It is linked by Powell et al., (2022) to Anatolia and to the tin mineralisation at Kestel and Hisarcık in particular. This, again based upon the lead isotope composition of the tin, appears to be correct at first glance. Most of the artefacts of ingot groups MC1 and MC2 are comparable to Anatolian copper and lead ores, and correlate best with the lead isotope ratios of ores from the Bolkardağ range in the Taurus Mountains (Figures 1A, B). The tin ingots thus contain lead that came from the Bolkardağ range. Strikingly, many of the ingots are enriched in lead (Figure 4C). The contents range from 45 to 130,000 μg g–1 with a median of 1,090 μg g–1 and an average of 6,800 μg g–1 (Powell et al., 2022). This clearly indicates incorporation of extraneous lead because cassiterite is usually lead-poor (Begemann et al., 1999; Pavlova et al., 2015; Moscati and Neymark, 2020). Powell et al. are aware of this issue and write “The Uluburun tin cargo includes a morphologically diverse (oxhide, slab, bun, and stone anchor shaped; Figure 4) group of Pb-enriched ingots (MC1) that were produced from ores collected in the Taurus region and subsequently pooled and smelted at the nearby Pb-Ag mining center of Bolkardağ where extraneous Pb was added” (Powell et al., 2022, 2). If this statement is indeed true, it raises a fundamental problem that recurs with the interpretation of lead isotope ratios in archaeological metal objects. In the case that extraneous lead was either intentionally added or entered as (probably unrecognised) contamination, then the lead isotope signature of the original metal would be altered. For example, many bronzes of the LBA show high lead concentrations and, accordingly, their lead isotope signatures relate predominantly or exclusively to the source of the lead (e.g., Rohl and Northover, 1994). The same happens when tin metal is alloyed or contaminated with foreign lead (e.g., from the smelting structures, fuel), and since cassiterite is generally extremely poor in lead (<10 μg g–1), the isotopic signature of lead in the tin is partly or completely overwritten. Strictly speaking, inferring the tin deposits by means of lead isotope analysis is not possible given the composition of the majority of the MC1 and MC2 ingots. It is also impossible to determine when and where the lead entered the tin. Therefore, it is equally likely that the tin derived from Late Paleozoic tin deposits such as those in the Iberian Peninsula, the British Isles, or from Mesozoic deposits in the Pamirs. It would then be conceivable, for example, that tin was transported from far distances to the eastern Mediterranean, where it was pooled and cast in suitable and familiar forms (such as oxhide and rectanguloid ingots), and there lead may have been added or entered as impurity. The fragments of what appears to have been a lead bar fused to one of the Uluburun ingots (Powell et al., 2021a) show that tin or tin ingots from other locations were processed at a location where lead ingots were present.
Conclusion from the tin isotope values
Despite these pitfalls, Powell et al. use the tin isotope composition of the ingots of all sub-groups (except MC1C) as additional evidence for the use of tin ores from Kestel and Hisarcık. A total of six cassiterite samples from the two mineralisations were isotopically characterised and found to represent the entire range of the fractionation-corrected δ124Sn/116Sn values of the ingots (0.04‰–1.04‰). Apart from the fact that six mineral samples are not statistically representative in geochemical terms, it is questionable whether the presented tin isotope values are reliable. We do not principally doubt the tin isotope data of Powell et al., however, for the cassiterites from Kestel and Hisarcık, we would expect difficulties in the thermal reduction procedure used by the authors due to their mineralogical peculiarities and associations. The mineralisations of Kestel and Hisarcık are without question tin-bearing, but cassiterite is present in complex intergrowth with hematite, arsenates and clay minerals (Yalçin and Özbal, 2009; Yazgan, 2015; Johnson et al., 2017; Powell et al., 2021b). Our group at CEZA Mannheim reduced more than one thousand samples of different tin-bearing minerals thermally, and from our own experience with iron-rich ore samples, thermal reduction of such cassiterite by the method described in Mathur et al. (2017) and Berger et al. (2019a), results in the evaporation of some tin, despite the use of potassium cyanide (the latter is used to prevent evaporation). The evaporation of tin species can lead to measurable fractionation and false isotopic values, just as large amounts of siliceous minerals can result in the formation of substantial slag that takes up part of the tin (Berger et al., 2019a). Thus, it is possible that the authors did not notice evaporation during reduction of their samples. If this was the case we would recommend to reduce the samples again with another method or at least to disclose preparative details such as the composition of the original unreduced samples. We found that reduction of complex ores (also those from Mushiston) is easier and works without evaporative loss of tin when the impure cassiterite is covered by copper powder and above potassium cyanide. Reduction at 1,100°C results in bronze prills that absorb all the tin, while iron and other impurities settle on top or nearby the prill or are bound in a matte phase. This method is better known in archaeometallurgy as cementation.
Notwithstanding our concerns about the sample preparation, the tin isotope composition of the Anatolian cassiterites provides again no unique fingerprint. On the one hand, the isotopic range of cassiterite from Kestel and Hisarcık of about 0.2‰–1.2‰ δ124Sn/116Sn (Powell et al., 2022 Figure 5) is quite large. On the other, there are other tin mineralisation or deposits having similar, overlapping or identical isotopic variation (Figure 2). Because the lead isotope signature does not necessarily trace the tin, the spectrum of potential supply regions is therefore much broader than described by Powell et al., as also Variscan tin ores (whose original signature is masked by extraneous lead) must be taken into account. At the current stage of research, the only regions that can be excluded with high statistical probability are the pegmatitic and Mesozoic tin deposits in the Hindukush in eastern Afghanistan. They have lower δ124Sn/116Sn values than most of the ingots of all MC sub-groups (except MC1C) ranging down to negative compositions as observed for Mushiston or even lower (Berger et al., 2019b; Berger et al., 2021; Berger et al., 2023). Hence, contrary to the suggestions of Powell et al. (2022), pinpointing the source area of all these ingots is not possible by means of tin isotopes. It should anyway be standard scientific practice to emphasise that a determination of provenance by analytical means is basically never possible, but that origin can only be narrowed down to a certain degree of probability.
Relevance of the trace elements
Further clues can be obtained from the chemical composition of the tin ingots. As can be seen in Figure 4A, the ingots of the Uluburun MC groups contain less than 50 μg g–1 arsenic, which is within the range observed for other LBA tin ingots (Wang et al., 2016; Berger et al., 2019a). The primary mineralisation of Kestel, Hisarcık and comparable tin-bearing occurrences in the Taurus region are rich in arsenic compounds in addition to iron oxides (Yalçin and Özbal, 2009; Yazgan, 2015; Johnson et al., 2017; Powell et al., 2021b). Therefore, one would expect a significant arsenic content in the smelted tin when such primary ores were used (Craddock et al., 2007). The same applies to iron minerals, which were not easy to separate from similar-looking cassiterite with ancient methods. Even if beneficiation of ore concentrates is assumed (Earl and Yener, 1995; Earl and Özbal, 1996; Yener, 2009), neither iron minerals nor firmly intergrown arsenic compounds will have been completely separated. The smelted tin from such mineral assemblages should thus have contained much iron and a considerable amount of arsenic, just like the tin from the Mushiston ores. Unfortunately, Powell et al. do not specify any iron concentration for the Uluburun ingots although this would be important information. However, judging from previous chemical analyses of part of the cargo (Hauptmann et al., 2002), the iron concentrations are consistently very low with values below 100 μg g–1 in 25 of 32 ingots analysed. This concentration would not be expected if iron-rich cassiterite concentrates were smelted, a result emphasised by smelting experiments carried out with the cassiterite from Kestel, Hisarcık and a nearby mineralisation (Earl and Özbal, 1996; Johnson et al., 2017). The authors of one of these studies were aware of the so-called “hardhead problem”, addressing an intermetallic compound of tin and iron (FeSn, Fe2Sn etc.) that forms upon smelting ferruginous cassiterite concentrates (Wright, 1982; Smith, 1996; Johnson et al., 2017). Hardheads make the smelted tin very brittle and difficult to melt, and if the iron content of the charge is too high, it could ultimately be impossible to obtain pure tin (Tafel, 1929). In addition, the high proportion of siliceous components at Kestel and Hisarcık further reduces tin yield, as much tin enters the slag, which can be lost depending on the process parameters. If tin metal was obtained, the iron content could be largely eliminated by re-melting (Craddock and Timberlake, 2005; Craddock et al., 2007), but more than 100 μg g–1 iron is still to be expected. In contrast, it is not possible to remove arsenic from tin by re-melting (Craddock and Timberlake, 2005; Craddock et al., 2007), and so is the case with the pyrometallurgically similarly behaving antimony, which seems to be present in the mineralisations as well (Powell et al., 2021b). All these arguments considered, the tin from Uluburun is not compatible with Anatolian primary iron-tin mineralisation, besides the fact that the Kestel mine was apparently not operating in the LBA. In view of the results of the smelting experiments with the mineral assemblages in question (Earl and Özbal, 1996; Johnson et al., 2017), the extraction of adequate quantities of metallic tin with low impurities seems to be quite complicated. Therefore, one might pose the question if not metallic tin but rather bronze was produced from the material of Kestel and Hisarcık via cementation with metallic copper or co-smelting with copper ores?
Ingot group MC1C
Seven ingots from Uluburun belong to the MC1C cluster and stand out due to very negative fractionation-corrected δ124Sn/116Sn values of −0.95 to −0.35‰ (Figure 2). This is interpreted by Powell et al. as indication that stannite or hydrostannates were the most likely starting materials. The impurity levels are slightly elevated in relation to other ingots of the cargo, especially that of copper. Nevertheless, the seven ingots are not fundamentally different from cassiterite-derived ingots in terms of chemistry (Figure 4). It is therefore unlikely that this group of tin ingots was produced from iron- and copper-rich stannite, as their copper contents are low (cf. Hauptmann et al., 2002). If stannite would have been used, then the iron, but especially the copper contents should be much higher, as was to be expected from smelting the secondary ores from Mushiston (Berger et al., 2022a). Without a roasting process, to remove part of the sulphur, smelting of stannite to yield tin was not possible anyway, and it is questionable whether this really happened in prehistory. What remains unclear from Powell et al.’s argumentation is why they assume an alluvial source. The authors suppose that “[…] hydrostannates such as mushistonite {[Cu, Zn, Fe]Sn(OH)6} and cassiterite (SnO2) […] may have accumulated in nearby river sediments” Powell et al., (2022), 3) without providing any evidence or reference. They claim that “[…] the most likely known source for group MC1C is the Maden stream, which flows below the polymetallic mineralization of Bolkardağ” Powell et al., (2022), 3). If this speculation is based on the analysis of the single stannite sample included in the study, it is weak evidence indeed. First, stannite is a common yet not abundant mineral in polymetallic environments across Eurasia (Ramdohr, 1975; Anthony et al., 1990), which is as unstable under oxidative alluvial conditions as hydrostannates (Haase et al., 2022). And second, it is characterised by consistently low but non-diagnostic tin isotope values (Yao et al., 2018; Berger et al., 2022a). Accordingly, a specific source cannot be identified with these characteristics. It may well be conceivable that cassiterite with comparably low δ124Sn/116Sn values was used for producing the seven ingots. In this context, it might be worth considering the Mesozoic cassiterite mineralisation of the Hindukush in eastern Afghanistan or Late Paleozoic deposits in the Iberian Peninsula with comparably low isotopic compositions (Figure 2) (Berger et al., 2019b; Berger et al., 2021; Berger et al., 2023), rather than the construction of a rather unlikely scenario.
The Mediterranean–Central Asian link
Archaeological data is inherently more difficult to interpret than data generated by the natural sciences. Nonetheless, there is a number of relevant points bearing on the proposal of Powell et al. from the perspective of the historical disciplines.
The first is a statement, namely, that the authors prudently do not build a case from archaeological or textual data to support the analytically based assumption of a late-2nd millennium BCE tin procurement route from Central Asia to the eastern Mediterranean. For the MBA (ca. 2,000–1,600 BCE), an eastern origin for a large part of the tin consumed in Mesopotamia and Anatolia is generally accepted, thanks to the abundant documentation from Kültepe and Mari cuneiform archives (Reiter, 1997; Dercksen, 2001; Veenhof and Eidem, 2008). This trade was, however, highly volatile. Already in the mid-19th century BCE a decline in the volume of traded tin is noticeable for Kültepe (Dercksen, 2001; Barjamovic et al., 2012). Other centres, such as Mari, continued to source from Elam, and shipped part of the metal to Syria and the Levant in the 18th century BCE (Joannès, 1991). From how far east (the Zagros or Central Asia) the tin came is still a matter of debate (Helwing, 2009; Cuénod et al., 2015), but the Elam-Anatolia/Levant axis, i.e., a westward movement of goods, can be considered a given. From the 17th century BCE onwards, our sources fall silent on the issue, and during the LBA (16th−13th century BCE) the few texts in existence suggest a re-configuration of the tin trade network. Tin was ubiquitous in the LBA (with the possible exception of Babylonia), to the degree that we can only rarely observe a directionality in trade, let alone a dominant source region. More specifically, Elamite texts (Stolper, 1984) deal in different quantities of tin but fail to mention its provenance, while Assyrian sources are more commensurate with a north-west to south-east movement of tin (Faist, 2001a; Faist, 2001b; Postgate, 2014). This is explicitly stated by Powell et al., but immediately qualified to the effect that “[…] no textual evidence definitively identifies localities […]” (Powell et al., 2022, 4), at the same time passing over the fact that the documentation is no better for a Middle Asian origin of tin in the MBA, to say nothing of the LBA. On current evidence, the latest textual indicator for an eastern origin of tin in the Near East predates the Uluburun wreck by 400 years. Powell et al. (2022) repeatedly stress that the same (potential, not proven) MBA tin trade routes from Middle Asia to the eastern Mediterranean could have been operational in the second half of the 2nd millennium BCE, but all textual sources available for the 14th century BCE can be more easily accommodated with an eastbound trade of tin from the Mediterranean.
The most conspicuous support for an LBA tin trade linking Central Asia and the Mediterranean in Powell et al. (2022) is Figure 5, a map of the area discussed, from the eastern Mediterranean to the Tarim Basin, with an inset covering Europe. This map is problematic in a number of respects: Already the map section is suggestive, emphasising the eastern source regions of tin, while relegating the large European deposits to the map inset. As for the latter, Mt. Cer (only a small occurrence of cassiterite) and the Erzgebirge are displaced from their correct positions by several hundred kilometres, and the Mt. Cer mining symbol suggests a significant and secured tin source for which there is as yet no archaeological or analytical evidence (Huska et al., 2014; Mason et al., 2020; Powell et al., 2020). More importantly, the information included stresses the relevance of these eastern connections to the point of misrepresentation. Apart from the uncontested locations of the Uluburun wreck and the tin deposits discussed, the map operates with three major graphical elements (Figure 5). Points represent the “main sites”, colour shading the “key LBA polities”, i.e., states roughly contemporary with the Uluburun wreck, and dashed arrows “[…] documented trade networks ca. 2200 to 1700 BCE” (Powell et al., 2022, 2). A blue shading extends the coloured territories eastward, across northern Iran and far into the Central Asian mountains, where “Bactria”, the Mushiston deposit, and the “Inner Asian Mountain Corridor” serve as its eastern anchors. This blue shading represents “[…] the corridor connecting the Anatolian and Central Asian/Middle Eastern tin trade (in blue) ca. 1600 to 1000 BCE” (Powell et al., 2022, 2). The reader is presented with a visually compelling and seemingly convincing illustration of the eastern procurement theory. In reality, not one of the sites mapped to the east of the Zagros chain is contemporary with the polities of the Amarna age illustrating the LBA networks to the west. The synchronicity easily read into the map is only imaginative. The equally suggestive purple dashed arrows are correctly labelled as referring to MBA trade networks, but it is not acknowledged that none of the documented trade is in tin, but in lapislazuli, carnelian, and similar prestige goods. Most importantly, the blue-shaded area extends the “Inner Asian Mountain Corridor”, an immensely fruitful concept developed by co-author M. Frachetti, into northern Iran claiming that it “[…] entangled local communities from the northern edges of the Iranian Plateau through the Pamir and Tien Shan foothills eastward toward China and northward to the Altai mountains in shared networks of resource exchange” and that “similarly structured, small-scale communities [as in Central Asia] may also have been engaged in tin trade from Mushiston (and nearby sources) southward and westward across the Iranian Plateau” (Powell et al., 2022, 4–5, 6). The basis for this statement remains elusive, since sites dating between 1,600 and 1,200 BCE are virtually unknown in northern Iran (Matthews and Fazeli Nashli, 2022). Only with the Early Iron Age (Marlik, from 1,250 BCE) does bronze appear in larger quantities in northern or north-eastern Iran (Oudbashi and Hessari, 2017). Contrary to the preceding MBA, we cannot point to any contacts between the Central Asian mining zone, dominated by Andronovo communities and their successors, and western Asia after 1,600 BCE at all. Figure 5 therefore amalgamates an MBA (early 2nd millennium BCE) Middle Asian production zone with the LBA (late 2nd millennium BCE) environment of a western Asian tin consumption zone, and links the two by a connecting corridor that is not backed by any material or textual evidence.
Given this dearth of archaeological, textual, and analytical data in support of a Mushiston or even a Central Asian provenance for some Uluburun tin, other source regions must be considered. In particular, the economic networks connecting Central Europe to the Mediterranean World have received much renewed attention during the past decade, which is not reflected in the paper under discussion. Originally argued on the basis of contact finds (Gerloff, 1993), considerable evidence now demonstrates that much of the Central European landmass formed part of a single Bronze Age economic sphere linked to the Mediterranean along the riverine corridors of Danube, Rhine, and Rhône (Gerloff, 2010; Earle et al., 2015; Kristiansen and Suchowska-Ducke, 2015; Vandkilde, 2016). Even when the scale and economic impact of this trade prior to 1,500 BCE may be argued, there can be no doubt that by the time of the Uluburun ship (ca. 1,320 BCE), tin and bronze formed an important part of this trans-continental economic activity. The finds from the wreck have in the past correctly been viewed in this context. Oxhide ingots, the hallmark of the LBA metals trade, are known from the Eastern Mediterranean shores, Crete, and Sardinia (Jones, 2007), the Balkans (Athanassov et al., 2020), and from Central Europe (Sabatini, 2016); depictions were found on petroglyphs in Scandinavia (Ling et al., 2019). The origin of most of the oxhide ingot copper—as has been analytically verified also for the Uluburun assemblage (Stos-Gale et al., 1998)—is undisputedly Cyprus, which was integrated in a large network of trade routes from all directions in the LBA. Connections to the west reached as far as Sardinia, where most of the oxhide ingots known to date were found, except Cyprus and the Uluburun wreck (Blake, 2015; Gradoli et al., 2020; Sabatini and Lo Schiavo, 2020; Knapp et al., 2022). Sardinia itself has been recently considered a potential supplier for some copper and bronze found in northern and north-western Europe, and LBA lead ingots from Israel (Ling et al., 2019; Berger et al., 2022b; Yahalom-Mack et al., 2022). It has even been labelled a market place for the metals trade within the LBA Mediterranean interaction sphere, connected via trade routes to Iberia, Atlantic and Continental Europe, and the Eastern Mediterranean (Iacono et al., 2022; Matta and Vandkilde, 2023; Perra and Lo Schiavo, 2023). In the light of emerging evidence, it is conceivable that tin from Iberia or Cornwall reached Sardinia, and was traded further to the East. Cyprus could have been another important entrepôt, where tin from different locations was pooled, re-melted and subsequently traded on. The latter assumption finds support in tin ingots from Israel bearing symbols of “Cypro-Minoan” origin, indicating that Cypriot agents were likely involved in the trade of tin (Galili et al., 2013). Interestingly, that is what has previously been suggested for marked ingots of Sardinian lead from the Israeli coast (Wachsmann, 2020; Yahalom-Mack et al., 2022), and even one third of the Uluburun copper and tin ingots were marked in a comparable way (Pulak, 1998; Jones, 2007). In addition to the metal, amber beads discovered in the Uluburun ship can be connected with the Baltic shores (Pulak, 2005), and traffic in raw Baltic amber has been analytically proven to have reached as far as the Levant (Mukherjee et al., 2008). All these strands of information demonstrate that the cargo of the Uluburun ship (including many more materials) was the result of intensive trade connections within a prehistoric globalised world encompassing Europe and the Near East. The various materials and artefacts on the ship originated from different parts of the Old World, which is at odds with the unidirectional model for tin as suggested by Powell et al. (2022). In Europe, the LBA metal trade was most likely based on the tin deposits of Cornwall, Iberia and the Erzgebirge, thus Powell et al.’s laconic remark that “[…] Erzgebirge tin trade did not extend to the Mediterranean or Black Sea […], making it an unlikely contributor to the Uluburun shipwreck tin ingot assemblage” (Powell et al., 2022, 4) does not adequately reflect the amount of material evidence pointing in this direction (Penhallurick, 1986; Tolksdorf et al., 2020).
Finally, another particularly important line of evidence with direct bearing on the metals trade has been assembled recently: the spread of weighing technology. Standardised systems of weighing would seem to be a prerequisite of long-distance trade in metals (Ialongo et al., 2021; Vandkilde, 2021). After 2,800 BCE, weights dispersed from Mesopotamia and Egypt first to Syria, the Aegean and Anatolia. During the first half of the 2nd millennium BCE they reached northern Italy and by 1,340 BCE scales and weights had crossed over the Alps into Central Europe. This distribution marks the widening of an economic horizon closely aligned with metal technology and procurement. Interestingly, while the Persian Gulf and southern Iran have produced evidence for weighing, this is not the case for northern Iran or Central Asia (Ialongo et al., 2021; Ascalone, 2022). Neither a Mesopotamian, nor an Indus-derived system of weights extended to these regions. While the metrological evidence does not rule out the transport of metals between Central Asia, Mushiston and Uluburun, it does not conform to the trade system proposed by Powell et al. (2022). Quite to the contrary, it lends support to a European tin trade route supplying the eastern Mediterranean in the Late Bronze Age, as does other archaeological and historical evidence.
Conclusion
The Uluburun shipwreck with its unique cargo of raw metal is a key find of Bronze Age archaeology and essential for understanding prehistorical networks. The publication of Powell et al. (2022) brings forward a valuable dataset of the culturally and historically important Bronze Age tin ingots from the ship. For the first time, tin isotope values of ca. 90% of the entire find complex are presented, supplemented by new chemical data and a few analyses of lead isotope ratios by MC-ICP-MS. All the data is assessed in combination with the aim of narrowing down the tin origin, and the authors confidently sketch a picture in which Central Asian tin sources—with Mushiston in particular—and mineralisations in Anatolia, are the lynchpins of tin economy and trade. However, we have described in detail that on the basis of the existing comparative data of Eurasian tin ores and due to the lack of archaeological evidence, no definite statements can be made about the origin of the LBA tin of the Uluburun cargo. We have even shown that the polymetallic tin ores from Mushiston in Central Asian Tajikistan can in no way be a source for the tin ingots from Uluburun, and that tin mineralisation in Anatolia is a less likely option, too. Instead, in view of the chemical and isotopic similarities with the somewhat younger tin ingots from Israel and Salcombe, we suggest a possible common origin for at least part of the Uluburun cargo (group P) with ingots from the other sites, for which tin deposits in south-west England have been identified as the most likely sources. Yet, this interpretation is not incontrovertible as it rests upon a still tenuous base of ore data. It may well be possible that future research will make other deposits more likely or that a diversity of ores (e.g., including Afghan ores) were used to produce the tin traded in the LBA Mediterranean. We do not entirely reject the interpretations of Powell et al. (2022), nor do we claim that they are completely wrong, but the finality of their statements about the origin of LBA tin is justified neither by the data presented nor the archaeological evidence at hand. Only when more lead isotope and chemical analyses of cassiterites across Eurasia are available, will it be possible to more accurately locate potential tin deposits used for the tin from Uluburun and elsewhere. Although considerable progress has been made over the last 20 years, and we are now in the position of excluding non-matching tin deposits with greater certainty than before, tracking down the tin sources of the Bronze Age and beyond remains one of the most challenging problems in Old World archaeology.
Data availability statement
The data presented in the study is included in the article/Supplementary Material or was taken from the referenced literature. Further inquiries can be directed to the corresponding authors.
Author contributions
Conceptualisation: DB. Methodology: DB and GB. Investigation: DB, GB, and KK. Visualisation: DB. Supervision: DB and EP. Writing—original draft: DB and KK. Writing—review and editing: DB, GB, KK, and EP. All authors contributed to the article and approved the submitted version.
Funding
The funding for the research that laid the foundation to write this article was received from an Advanced Grant of the European Research Council (Grant No. 323861) awarded to EP. Open Access was supported by Open Access Publishing Fund of University of Tübingen.
Acknowledgments
We thank Ehud Galili, The Zinman Institute of Archaeology, University of Haifa, Israel, for providing two additional tin ingots from Israel for analysis. We further acknowledge the helpful comments of several colleagues on the original manuscript. Many thanks to Brittany Kucera for editing the English language. We further acknowledge financial support by Open Access Publishing Fund of University of Tübingen.
Conflict of interest
The authors declare that the research was conducted in the absence of any commercial or financial relationships that could be construed as a potential conflict of interest.
Publisher’s note
All claims expressed in this article are solely those of the authors and do not necessarily represent those of their affiliated organizations, or those of the publisher, the editors and the reviewers. Any product that may be evaluated in this article, or claim that may be made by its manufacturer, is not guaranteed or endorsed by the publisher.
Supplementary material
The Supplementary Material for this article can be found online at: https://www.frontiersin.org/articles/10.3389/feart.2023.1211478/full#supplementary-material
Abbreviations
EDXRF, Energy dispersive X-ray fluorescence spectroscopy; LBA, Late Bronze Age; Ma, Billion years, geochronological unit; MBA, Middle Bronze Age; MC-ICP-MS, Multi collector mass spectrometry with inductively coupled plasma ionisation; TIMS, Thermal ionisation mass spectrometry.
References
Alimov, K., Boroffka, N., Bubnova, M., Burjakov, J., Cierny, J., Jakubov, J., et al. (1998). Prähistorischer Zinnbergbau in Mittelasien -Vorbericht der Kampagne 1997. Eurasia Antiq. 4, 137–199.
Anthony, J. W., Bideaux, R. A., Bladh, K. W., and Nichols, M. C. (1990). Handbook of mineralogy. Tucson, Arizona, United States: Mineral Data Publishing.
Artioli, G., Canovaro, C., Nimis, P., and Angelini, I. (2020). LIA of prehistoric metals in the central Mediterranean area: A review. Archaeometry 62, 53–85. doi:10.1111/arcm.12542
Ascalone, E. (2022). Bronze Age weights from Mesopotamia, Iran and greater Indus valley. Kiel, Germany: Wachholtz.
Athanassov, B., Dimitrov, K., Krauß, R., Popov, H., Schwab, R., Slavchev, V., et al. (2020). “A new look at the Late Bronze Age oxhide ingots from the eastern Balkans,” in Objects, ideas and travelers: Contacts between the Balkans, the Aegean and western Anatolia during the Bronze and Early Iron Age. Universitätsforschungen zur prähistorischen Archäologie 350. Editors J. Maran, R. Băjenaru, S.-C. Ailincăi, A.-D. Popescu, and S. Hansen (Bonn, Germany: Verlag Dr. Rudolf Habelt GmbH), 299–356.
Barjamovic, G. J., Hertel, T., and Larsen, M. T. (2012). Ups and downs at Kanesh: Chronology, history and society in the old Assyrian period. Leiden, Netherlands: Nederlands Instituut voor het Nabije Oosten te Leiden.
Begemann, F., Kallas, K., Schmitt-Strecker, S., and Pernicka, E. (1999). “Tracing ancient tin via isotope analyses,” in The beginnings of metallurgy. Der Anschnitt, Beiheft 9. Editors A. Hauptmann, E. Pernicka, T. Rehren, and Ü. Yalcin (Bochum, Germany: Deutsches Bergbau-Museum), 277–284.
Begemann, F., and Schmitt-Strecker, S. (2009). Über das frühe Kupfer Mesopotamiens. Iran. Antiq. 44, 1–45. doi:10.2143/ia.44.0.2034374
Berger, D., Brügmann, G., Friedrich, R., Lutz, J., Meyer, H.-P., and Pernicka, E. (2022a). Shiny bronze in glassy matter: An inconspicuous piece of slag from the bronze age mining site of Mušiston (Tajikistan) and its significance for the development of tin metallurgy in Central Asia. Archaeol. Anthropol. Sci. 14, 150. doi:10.1007/s12520-022-01606-2
Berger, D., Brügmann, G., and Pernicka, E. (2019a). On smelting cassiterite in geological and archaeological samples: Preparation and implications for provenance studies on metal artefacts with tin isotopes. Archaeol. Anthropol. Sci. 11, 293–319. doi:10.1007/s12520-017-0544-z
Berger, D., Brügmann, G., Pernicka, E., and Stolz, J. (2021). “Naturwissenschaftliche Untersuchungen zur Erhaltung, Zusammensetzung und Herkunft der frühbronzezeitlichen Zinnperlen aus Schwabmünchen und Buxheim,” in Eine einmalige Zinnperlentracht der Frühbronzezeit aus Bayern: “Powerdressing” vor 4000 Jahren. Inhalte-Projekte-Dokumentationen 23. Editors S. Berg, and C. Metzner-Nebelsick (Lindenberg im Allgäu, Germany: Kunstverlag Josef Fink), 117–140.
Berger, D., Figueiredo, E., Brügmann, G., and Pernicka, E. (2018). Tin isotope fractionation during experimental cassiterite smelting and its implication for tracing the tin sources of prehistoric metal artefacts. J. Archaeol. Sci. 92, 73–86. doi:10.1016/j.jas.2018.02.006
Berger, D., Kaniuth, K., Boroffka, N., Brügmann, G., Kraus, S., Lutz, J., et al. (2023). The rise of bronze in Central Asia: New evidence for the origin of Bronze Age tin and copper from multi-analytical research. Front. Earth Sci. 11. doi:10.3389/feart.2023.1224873
Berger, D., Soles, J. S., Giumlia-Mair, A. R., Brügmann, G., Galili, E., Lockhoff, N., et al. (2019b). Isotope systematics and chemical composition of tin ingots from Mochlos (Crete) and other Late Bronze Age sites in the eastern Mediterranean Sea: An ultimate key to tin provenance? PLOS ONE 14, e0218326. doi:10.1371/journal.pone.0218326
Berger, D., Wang, Q., Brügmann, G., Lockhoff, N., Roberts, B. W., and Pernicka, E. (2022b). The Salcombe metal cargoes: New light on the provenance and circulation of tin and copper in Later Bronze Age Europe provided by trace elements and isotopes. J. Archaeol. Sci. 138, 105543. doi:10.1016/j.jas.2022.105543
Blake, E. (2015). “Late Bronze Age Sardinia: Acephalous cohesion,” in The Cambridge prehistory of the Bronze and Iron Age Mediterranean. Editors A. B. Knapp, and P. van Dommelen (Cambridge, England: Cambridge University Press), 96–108. doi:10.1017/CHO9781139028387.009
Craddock, P. T., Meeks, N., and Timberlake, S. (2007). “On the edge of success: The scientific examination of the products of the early mines research group smelting experiments,” in Metals and mines: Studies in archaeometallurgy. Editors S. L. Niece, D. Hook, and P. T. Craddock (London, England: Archetype Publications Ltd.), 37–45.
Cuénod, A., Bray, P., and Pollard, A. M. (2015). The “Tin Problem” in the prehistoric Near East: Further insights from a study of chemical datasets on copper alloys from Iran and Mesopotamia. Iran 53, 29–48. doi:10.1080/05786967.2015.11834749
Dercksen, J. G. (2001). “‘When we met in Hattush’. Trade according to old Assyrian texts from Alishar and Bogazkoy,” in Veenhof anniversary volume: Studies presented to Klaas R. Veenhof on the occasion of his sixty-fifth birthday. PIHANS. Editors W. Van Soldt, J. G. Dercksen, N. J. C. Kouwenberg, and T. Krispijn (Leiden, Netherlands: Neederlands Instituut voor het Nabije Oosten), 39–66.
Dercksen, J. G. (2005). “Metals according to documents from Kültepe-Kanish dating to the Old Assyrian colony period,” in Anatolian metal III. Der Anschnitt, Beiheft 18. Editor Ü. Yalcin (Bochum, Germany: Bochum: Deutsches Bergbau-Musem), 17–34.
Dube, R. K. (2006). Interrelation between gold and tin: A historical perspective. Gold Bull. 39, 103–113. doi:10.1007/BF03215537
Earl, B., and Yener, K. A. (1995). Tin smelting at the Oriental Institute. Orient. Inst. News Notes 146, 1–5.
Earl, B., and Özbal, H. (1996). Early Bronze Age tin processing at Kestel/Göltepe, Anatolia. Archaeometry 38, 289–303. doi:10.1111/j.1475-4754.1996.tb00777.x
Earle, T., Ling, J., Uhnér, C., Stos-Gale, Z., and Melheim, L. (2015). The political economy and metal trade in Bronze Age Europe: Understanding regional variability in terms of comparative advantages and articulations. Eur. J. Archaeol. 18, 633–657. doi:10.1179/1461957115Y.0000000008
Faist, B. I. (2001a). Der Fernhandel des assyrischen Reiches zwischen dem 14. und 11. Jh. v. Chr. Alter Orient und Altes Testament 265. Münster, Germany: Ugarit-Verlag.
Faist, B. I. (2001b). “Die Handelsbeziehungen zwischen Assyrien und Anatolien in der zweiten Hälfte des 2. Jt.s v. Chr., unter besonderer Berücksichtigung des Metallhandels,” in Anatolien im Lichte kultureller Wechselwirkungen: Akkulturationsphänomene in Kleinasien und seinen Nachbarregionen während des 2. und 1. Jahrtausends v. Chr. Editors H. Klinkott (Tübingen), 53–66. doi:10.11588/PROPYLAEUMDOK.00000951
Faure, G., and Mensing, T. M. (2005). Isotopes: Principles and applications. Hoboken, New Jersey, United States: John Wiley and Sons.
Galili, E., Gale, N., and Rosen, B. (2013). A Late Bronze Age shipwreck with a metal cargo from Hishuley Carmel, Israel. Int. J. Nauctical Archaeol. 42, 2–23. doi:10.1111/j.1095-9270.2012.00344.x
Garner, J. (2013). Das Zinn der Bronzezeit in Mittelasien II: Die montanarchäologischen Forschungen an den Zinnlagerstätten. Archäologie in Iran und Turan 194. Berlin Bochum: Deutsches Archäologisches Institut.
Garner, J. (2015). “Bronze Age tin mines in Central Asia,” in Archaeometallurgy in Europe III. Editors A. Hauptmann, and D Modaressi-Tehrani. Der Anschnitt, Beiheft 26. (Bochum, Germany: Deutsches Bergbau-Museum), 135–142.
Gerloff, S. (1993). Zu Fragen mittelmeerländischer Kontakte und absoluter Chronologie der Frühbronzezeit in Mittel-und Westeuropa. Prähistorische Z. 68, 58–102. doi:10.1515/prhz.1993.68.1.58
Gerloff, S. (2010). “Von Troja an die Saale, von Wessex nach Mykene – Chronologie, Fernverbindungen und Zinnrouten der Frühbronzezeit Mittel- und Westeuropas,” in Der Griff nach den Sternen: Wie Europas Eliten zu Macht und Reichtum kamen. Tagung des Landesmuseums für Vorgeschichte Halle (Saale) 5(II). Editors H. Meller, and F. Bertemes (Halle, Germany), 603–639.
Gradoli, M. G., Waiman-Barak, P., Bürge, T., Dunseth, Z. C., Sterba, J. H., Schiavo, F. L., et al. (2020). Cyprus and Sardinia in the Late Bronze Age: Nuragic table ware at Hala Sultan Tekke. J. Archaeol. Sci. Rep. 33, 102479. doi:10.1016/j.jasrep.2020.102479
Grant, M. R. (1991). “Trace element in southern African artefact tins using NAA,” in Archaeometry ’90: Proceedings of the 27th Symposium on Archaeometry held in Heidelberg. Editors P. E. Wagner, and G. A. Wagner (Heidelberg, Germany: Heidelberg u. a.: Birkhäuser-Verlag), 165–172.
Haase, P., Kiefer, S., Pollok, K., Drahota, P., and Majzlan, J. (2022). Weathering of stannite–kësterite [Cu2(Fe,Zn)SnS4] and the environmental mobility of the released elements. Eur. J. Mineralogy 34, 493–506. doi:10.5194/ejm-34-493-2022
Hauptmann, A., Maddin, R., and Prange, M. (2002). On the structure and composition of copper and tin ingots excavated from the shipwreck of Uluburun. Bull. Am. Sch. Orient. Res. 328, 1–30. doi:10.2307/1357777
Haustein, M. (2013). Isotopengeochemische Untersuchungen zu möglichen Zinnquellen der Bronzezeit Mitteleuropas. Forschungsberichte des Landesmuseums für Vorgeschichte Halle 3. Halle, Germany: Landesmuseum für Vorgeschichte Halle.
Haustein, M., and Pernicka, E. (2011). Die Verfolgung der bronzezeitlichen Zinnquellen Europas durch Zinnisotopie: Eine neue Methode zur Beantwortung einer alten Frage. Jahresschr. für Mitteldtsch. Vorgesch. 92, 387–415.
Haustein, M., Gillis, C., and Pernicka, E. (2010). Tin isotopy: A new method for solving old questions. Archaeometry 52, 816–832. doi:10.1111/j.1475-4754.2010.00515.x
Helwing, B. (2009). Rethinking the tin mountains: Patterns of usage and circulation of tin in greater Iran from the 4th to the 1st millennium BC. Türkiye Bilim. Akad. Arkeol. Derg. 12, 209–221. doi:10.22520/tubaar.2009.0017
Huska, A., Powell, W., Mitrovic, S., Bankoff, H. A., Bulatovic, A., Filipovic, V., et al. (2014). Placer tin ores from Mt. Cer, West Serbia, and their potential exploitation during the Bronze Age. Geoarchaeology 29, 477–493. doi:10.1002/gea.21488
Iacono, F., Borgna, E., Cattani, M., Cavazzuti, C., Dawson, H., Galanakis, Y., et al. (2022). Establishing the Middle Sea: The Late Bronze Age of Mediterranean Europe (1700–900 BC). J. Archaeol. Res. 30, 371–445. doi:10.1007/s10814-021-09165-1
Ialongo, N., Hermann, R., and Rahmstorf, L. (2021). Bronze Age weight systems as a measure of market integration in Western Eurasia. Proc. Natl. Acad. Sci. 118, e2105873118. doi:10.1073/pnas.2105873118
Ivanov, O. P., Yeremenko, L. Y., Voronov, A. I., Zorin, Y. M., Kuzovenko, A. I., Khar’kevich, K. A., et al. (1993). Mineralogy and technology of new economic types of tin ores. Int. Geol. Rev. 35, 603–612. doi:10.1080/00206819309465545
Joannès, F. (1991). “L’étain, de l’Elam à Mari,” in Mesopotamie et Elam: Actes de la XXXVIème Rencontre Assyriologique Internationale, Gand, 10–14 juillet 1989. Editors L. de Meyer, and H. Gasche (Ghent, Belgium: University of Ghent), 67–76.
Johnson, M. A., Kulakoglu, F., Yener, K. A., Dardeniz, G., and Yazgan, E. (2017). “Experimental smelting of tin from Senir Sirti and Hisarcik near Kayseri. Mostly heartbreak, some elation,” in Movenment, resources, interaction: Proceedings of the 2nd Kültepe international meeting, Kültepe, 26–30 July 2015. Studies dedicated to Klaas Veenhof. Subartu 39. Editors F. Kulakoglu, and G. Barjamovic (Turnhout, Belgium: Brepols Publishers), 117–129.
Jones, M. R. (2007). Oxhide ingots, copper production, and the Mediterranean trade in copper and other metals in the Bronze Age. Texas, United States: Bryan, College Station.
Knapp, A. B., Russell, A., and van Dommelen, P. (2022). Cyprus, Sardinia and Sicily: A maritime perspective on interaction, connectivity and imagination in Mediterranean prehistory. CAJ 32, 79–97. doi:10.1017/S0959774321000330
Konopelko, D. L., Cherny, R. I., Petrov, S. V., Strekopytov, S., Seltmann, R., Vlasenko, N. S., et al. (2022). The Mushiston Sn deposit in Tajik Tien Shan as the type locality for stannite-cassiterite-hydrostannate mineralization: New mineral chemistry data and genetic constraints. J. Geochem. Explor. 239, 107017. doi:10.1016/j.gexplo.2022.107017
Kristiansen, K., and Suchowska-Ducke, P. (2015). Connected histories: The dynamics of Bronze Age interaction and trade 1500–1100 BC. Proc. Prehist. Soc. 81, 361–392. doi:10.1017/ppr.2015.17
Ling, J., Hjärthner-Holdar, E., Grandin, L., Stos-Gale, Z., Kristiansen, K., Melheim, A. L., et al. (2019). Moving metals IV: Swords, metal sources and trade networks in Bronze Age Europe. J. Archaeol. Sci. Rep. 26, 101837. doi:10.1016/j.jasrep.2019.05.002
Maddin, R. “Early metallurgy: The tin mystery,” in The fourth international conference on the beginning of the use on metals and alloys (BUMA-IV), Kunibiki Messe, Matsue, Shimane, Japan, May, 1998 (Matsue, Japan), 1–4.
Mason, A. H., Powell, W. G., Bankoff, H. A., Mathur, R., Bulatovi, A., Filipovi, V., et al. (2016). Tin isotope characterization of bronze artifacts of the central Balkans. J. Archaeol. Sci. 69, 110–117. doi:10.1016/j.jas.2016.04.012
Mason, A. H., Powell, W. G., Bankoff, H. A., Mathur, R., Price, M., Bulatovic, A., et al. (2020). Provenance of tin in the Late Bronze Age Balkans based on probabilistic and spatial analysis of Sn isotopes. J. Archaeol. Sci. 122, 105181. doi:10.1016/j.jas.2020.105181
Mathur, R., Powell, W., Mason, A., Godfrey, L., Yao, J., and Baker, Ma. E. (2017). Preparation and measurement of cassiterite for Sn isotope analysis. Geostand. Geoanalytical Res. 41, 701–707. doi:10.1111/ggr.12174
Matta, V., and Vandkilde, H. (2023). The state of the debate: Nuragic metal trade in the Bronze Age and Early Iron Age. Open Archaeol. 9, 20220280. doi:10.1515/opar-2022-0280
Matthews, R., and Fazeli Nashli, H. (2022). The archaeology of Iran from the palaeolithic to the achaemenid empire. London, England: Routledge.
Molofsky, L. J., Killick, D., Ducea, M. N., Macovei, M., Chesley, J. T., Ruiz, J., et al. (2014). A novel approach to lead isotope provenance studies of tin and bronze: Applications to South African, Botswanan and Romanian artifacts. J. Archaeol. Sci. 50, 440–450. doi:10.1016/j.jas.2014.08.006
Moscati, R. J., and Neymark, L. A. (2020). U–Pb geochronology of tin deposits associated with the Cornubian Batholith of southwest England: Direct dating of cassiterite by in situ LA-ICPMS. Miner. Deposita 55, 1–20. doi:10.1007/s00126-019-00870-y
Muhly, J. D. (1985). Sources of tin and the beginnings of bronze metallurgy. Am. J. Archaeol. 89, 275–291. doi:10.2307/504330
Mukherjee, A., Roßberger, E., James, M., Pfälzner, P., Higgitt, C., White, R., et al. (2008). The Qatna lion: Scientific confirmation of Baltic amber in Late Bronze Age Syria. Antiquity 82, 49–59. doi:10.1017/s0003598x00096435
Nessel, B., Brügmann, G., Frank, C., Marahrens, J., and Pernicka, E. (2019). Tin provenance and raw material supply – Considerations about the spread of bronze metallurgy in Europe. METALLA 24, 65–72. doi:10.46586/metalla.v24.2018.i2.65-72
Oudbashi, O., and Hessari, M. (2017). Iron Age tin bronze metallurgy at Marlik, northern Iran: An analytical investigation. Archaeol. Anthropol. Sci. 9, 233–249. doi:10.1007/s12520-015-0280-1
Pavlova, G. G., Palessky, S. V., Borisenko, A. S., Vladimirov, A. G., Seifert, T., and Phane, L. A. (2015). Indium in cassiterite and ores of tin deposits. Ore Geol. Rev. 66, 99–113. doi:10.1016/j.oregeorev.2014.10.009
Penhallurick, R. D. (1986). Tin in antiquity: Its mining and trade throughout the ancient world with particular reference to Cornwall. London, England: The Institute of Metals.
Perra, M., and Lo Schiavo, F. (2023). “Contacts and exchanges between Sardinia, continental Italy and the north-Western Europe in the Bronze Age (18th–11th c. BC): The “copper route”, the “amber route”, the “tin route”,” in Proceedings of the fifth festival of the nuragic civilization (orroli, cagliari). Cagliari, Italy: Arkadia Editore.
Pigott, V. C. (2021). “The acquisition of tin in Bronze Age southwest Asia,” in The world of the Oxus civilization. Editors B. Lyonnet, and N. A. Dubova (New York, NY, USA: Routledge), 827–861.
Postgate, N. (2014). Bronze age bureaucracy: Writing and the practice of government in Assyria. Cambridge, England: Cambridge University Press. doi:10.1017/CBO9781107338937
Powell, W., Frachetti, M., Pulak, C., Bankoff, H. A., Barjamovic, G., Johnson, M., et al. (2022). Tin from Uluburun shipwreck shows small-scale commodity exchange fueled continental tin supply across Late Bronze Age Eurasia. Sci. Adv. 8, eabq3766. doi:10.1126/sciadv.abq3766
Powell, W., Johnson, M., Pulak, C., Yener, K. A., Mathur, R., Bankoff, H. A., et al. (2021a). From peaks to ports: Insights into tin provenance, production, and distribution from adapted applications of lead isotopic analysis of the Uluburun tin ingots. J. Archaeol. Sci. 134, 105455. doi:10.1016/j.jas.2021.105455
Powell, W., Mladenovic, O., Cruse, S., Bankoff, H. A., and Mathur, R. (2020). Revisiting “‘tin in south-eastern Europe?’”. Starinar 70, 85–94. doi:10.2298/sta2070085p
Powell, W., Yazgan, E., Johnson, M., Yener, K. A., and Mathur, R. (2021b). Mineralogical analysis of the Kestel mine: An Early Bronze Age source of tin ore in the Taurus mountains, Turkey. Minerals 11, 91. doi:10.3390/min11010091
Pulak, C. (1998). The Uluburun shipwreck: An overview. Int. J. Naut. Archaeol. 27, 188–224. doi:10.1016/s1057-2414(98)80031-9
Pulak, C. (2005). “Das Schiffswrack von Uluburun,” in Das Schiff von Uluburun: Welthandel vor 3000 Jahren: Katalog der Ausstellung des Deutschen Bergbau-Museums Bochum. Veröffentlichungen aus dem Deutschen Bergbau-Museum Bochum 138. Editors Ü. Yalçin, C. Pulak, and R. Slotta (Bochum, Germany: Bochum: Deutsches Bergbau-Museum), 55–102.
Pulak, C. (2009). The Uluburun tin ingots and the shipment of tin by sea in the Late Bronze Age Mediterranean. TÜBA-AR 12, 189–207. doi:10.22520/tubaar.2009.0016
Rapp, G. J. (1978). “Trace element as a guide to the geographical source of tin ore,” in The search for ancient tin. Editors A. D. Franklin, J. S. Olin, and T. A. Wertime (Washington D.C., United States: Smithsonian Institution), 59–63.
Rapp, G. J., Rothe, R., and Jing, Z. (1999). “Using neutron activation analysis to source ancient tin (cassiterite),” in Metals in antiquity. BAR International Series 792. Editors S. M. M. Young, A. M. Pollard, P. Budd, and R. A. Ixer (Oxford: Archaeopress), 153–162.
Reiter, K. (1997). Die Metalle im Alten Orient: Unter besonderer Berücksichtigung altbabylonischer Quellen. Münster, Germany: Ugarit-Verlag.
Sabatini, S. (2016). Late Bronze Age oxhide and oxhide-like ingots from areas other than the Mediterranean: Problems and challenges. Oxf. J. Archaeol. 35, 29–45. doi:10.1111/ojoa.12077
Sabatini, S., and Lo Schiavo, F. (2020). Late Bronze Age metal exploitation and trade: Sardinia and Cyprus. Mater. Manuf. Process. 35, 1501–1518. doi:10.1080/10426914.2020.1758329
Sayre, E. V., Joel, E. C., Blackman, M. J., Yener, K. A., and Özbal, H. (2001). Stable lead isotope studies of Black Sea Anatolian ore sources and related Bronze Age and Phrygian artefacts from nearby archaeological sites. Appendix: New central Taurus ore data. Archaeometry 43, 77–115. doi:10.1111/1475-4754.00006
Seeliger, T. C., Pernicka, E., Wagner, G. A., Begemann, F., Schmitt-Strecker, S., Eibner, C., et al. (1985). Archaometallurgische Untersuchungen in Nord-und Ostanatolien. Jahrb. RGZM 32, 597–659. doi:10.11588/JRGZM.1985.0.69268
Smith, R. (1996). An analysis of the processes for tin smelting. Bull. Peak Dist. Mines Hist. Soc. 13, 91–99.
Stech, T., and Pigott, V. C. (1986). The metals trade in Southwest Asia in the third millennium B.C. Iraq 48, 39. doi:10.2307/4200250
Stolper, M. W. (1984). Texts from tall-i Malyan 1: Elamite administrative texts. Pennsylvania, United States: Babylonian Fund of the Univ. Museum.
Stos-Gale, Z. A., Gale, N. H., Bass, G. F., Pulak, C., Galili, E., and Sharvit, J. “The copper and tin ingots of the late bronze age mediterranean,” in Proceedings of the fourth international conference on the beginning of the use on metals and alloys (BUMA-IV), Kunibiki Messe, Matsue, Shimane, Japan, May, 1998 (Matsue, Japan), 115–126.
Stos-Gale, Z. A., and Gale, N. H. (2009). Metal provenancing using isotopes and the Oxford archaeological lead isotope database (OXALID). Archaeol. Anthropol. Sci. 1, 195–213. doi:10.1007/s12520-009-0011-6
Tafel, V. (1929). Lehrbuch der Metallhüttenkunde: Wismut, Blei, Zinn, Antimon, Zink, Quecksilber, Nickel, Aluminium. Leipzig, Germany: S. Hirzel.
Tolksdorf, J. F., Schröder, F., Petr, L., Herbig, C., Kaiser, K., Kočár, P., et al. (2020). Evidence for Bronze Age and Medieval tin placer mining in the Erzgebirge mountains, Saxony (Germany). Geoarchaeology 35, 198–216. doi:10.1002/gea.21763
Vandkilde, H. (2016). Bronzization: The Bronze Age as pre-modern globalization. Prähistorische Z. 91, 103–123. doi:10.1515/pz-2016-0005
Vandkilde, H. (2021). Trading and weighing metals in Bronze Age western Eurasia. Proc. Natl. Acad. Sci. 118, e2110552118. doi:10.1073/pnas.2110552118
Veenhof, K. R., and Eidem, J. (2008). Mesopotamia: The old Assyrian period. Cambridge, Massachusetts, United States: Academic Press.
von Baer, K. E. (1876). Von wo das Zinn zu den ganz alten Bronzen gekommen sein mag? Arch. für Anthropol. 9, 263–267.
Wachsmann, S. (2020). “Hahotrim, Israel: A late second-millennium BC group of metal scrap artefacts,” in Shared heritage: Proceedings of the sixth international congress for underwater archaeology. IKUWA 6. Editors J. A. Rodrigues, and A. Traviglia (Oxford: Archaeopress, 218–227.
Wagner, G. A., Begemann, F., Eibner, C., Lutz, J., Öztunali, Ö., Pernicka, E., et al. (1989). Archäometallurgische Untersuchungen an Rohstoffquellen des frühen Kupfers Ostanatoliens. Jahrb. RGZM 36, 637–686.
Wang, Q., Strekopytov, S., Roberts, B. W., and Wilkin, N. (2016). Tin ingots from a probable Bronze Age shipwreck off the coast of Salcombe, Devon: Composition and microstructure. J. Archaeol. Sci. 67, 80–92. doi:10.1016/j.jas.2016.01.018
Weisgerber, G. (2009). Prähistorischer Zinnbergbau in Mittelasien. Türkiye Bilim. Akad. Arkeol. Derg. (TÜBA-AR) 12, 237–258. doi:10.22520/tubaar.2009.0019
Yahalom-Mack, N., Finn, D. M., Erel, Y., Tirosh, O., Galili, E., and Yasur-Landau, A. (2022). Incised Late Bronze Age lead ingots from the southern anchorage of Caesarea. J. Archaeol. Sci. Rep. 41, 103321. doi:10.1016/j.jasrep.2021.103321
Yalçin, Ü., Pulak, C., and Slotta, R. (2005). Das Schiff von Uluburun: Welthandel vor 3000 Jahren: Katalog der Ausstellung des Deutschen Bergbau-Museums Bochum. Veröffentlichungen aus dem Deutschen Bergbau-Museum Bochum 138. Bochum, Germany: Deutsches Bergbau-Museum.
Yalçin, Ü., and Özbal, H. (2009). Ein neues Zinnvorkommen in Kayseri-Hisarcık, Zentralanatolien: Ein Vorbericht. Türkiye Bilim. Akad. Arkeol. Derg. (TÜBA-AR) 12, 117–122. doi:10.22520/tubaar.2009.0009
Yao, J., Mathur, R., Powell, W., Lehmann, B., Tornos, F., Wilson, M., et al. (2018). Sn-isotope fractionation as a record of hydrothermal redox reactions. Am. Mineralogist 103, 1591–1598. doi:10.2138/am-2018-6524
Yazgan, E. (2015). “Cassiterite(tin) mineralization related with Erciyes volcanic activities and the mode of formation of the hematite-cassiterite-yazganite-tridymite paragenesis and its implication for bronze alloys,” in Proceedings of the 1st Kültepe international meeting. Kültepe, 19–23 September, 2013: Studies dedicated to Kutlu Emre. Subartu 35. Editors F. Kulakoğlu, and C. Michel (Turnhout, Belgium: Brepols Publishers, 183–193.
Yener, K. A., Sayre, E. V., Joel, E. C., Özbal, H., Barnes, I. L., and Brill, R. H. (1991). Stable lead isotope studies of central Taurus ore sources and related artifacts from eastern Mediterranean Chalcolithic and Bronze Age sites. J. Archaeol. Sci. 18, 541–577. doi:10.1016/0305-4403(91)90053-r
Keywords: tin ingots, Uluburun shipwreck, Late Bronze Age, tin isotopes, lead isotopes, tin provenance
Citation: Berger D, Kaniuth K, Brügmann G and Pernicka E (2023) Why Central Asia’s Mushiston is not a source for the Late Bronze Age tin ingots from the Uluburun shipwreck. Front. Earth Sci. 11:1211478. doi: 10.3389/feart.2023.1211478
Received: 24 April 2023; Accepted: 17 July 2023;
Published: 04 August 2023.
Edited by:
Kunlong Chen, University of Science and Technology Beijing, ChinaReviewed by:
Alessandra Giumlia-Mair, AGM-Archaeoanalisi, ItalyElena Marrocchino, University of Ferrara, Italy
Copyright © 2023 Berger, Kaniuth, Brügmann and Pernicka. This is an open-access article distributed under the terms of the Creative Commons Attribution License (CC BY). The use, distribution or reproduction in other forums is permitted, provided the original author(s) and the copyright owner(s) are credited and that the original publication in this journal is cited, in accordance with accepted academic practice. No use, distribution or reproduction is permitted which does not comply with these terms.
*Correspondence: Daniel Berger, ZGFuaWVsLmJlcmdlckBjZXphLmRl; Ernst Pernicka, ZXJuc3QucGVybmlja2FAY2V6YS5kZQ==
†ORCID:Daniel Berger, orcid.org/0000-0001-6214-2264; Kai Kaniuth, orcid.org/0000-0002-6892-0917; Gerhard Brügmann, orcid.org/0000-0002-9904-0445; Ernst Pernicka, orcid.org/0000-0003-4746-9239