- 1Research Institute of Exploration and Development, Xinjiang Oilfield Company, PetroChina, Karamay, China
- 2Institute of Energy, Peking University, Beijing, China
- 3State Key Laboratory of Shale Oil and Gas Enrichment Mechanisms and Effective Development, Beijing, China
- 4College of Geosciences, China University of Petroleum (Beijing), Beijing, China
The Lower Permian Fengcheng Formation in the Mahu Sag develops a set of organic-rich alkaline lacustrine shale strata, which is a key area for shale oil exploration and development. As an important storage space and seepage channel for shale reservoirs, natural fractures have an impact on shale oil enrichment, production and development effect. In this study, the types and characteristics of natural fractures were first analyzed using core, thin section and imaging logging data. On this basis, combined with the distribution of fractures in single wells, the vertical distribution law of fractures is discussed. Finally, the planar distribution of fractures is evaluated using different seismic attributes such as coherence, curvature, likelihood, and AVAz. The results showed that three types of fractures are existed, including transformational shear fractures, intraformational open fractures and bed-parallel shear fractures, with intraformational open fractures being the most developed. The development degree of fractures in different layers has obvious differences, mainly controlled by lithology and brittle mineral content. The basalt and tuff are developed in the Feng 1 Member, with low carbonate mineral content, resulting in a relatively low degree of fracture development. The dolomite and argillaceous dolomite are developed in the Feng 2 Member and the Feng 3 Member, with high carbonate mineral content and brittleness, resulting in a high degree of fracture development. Additionally, the closer to the fault, the higher the degree of fracture development. On the plane, the fracture zone develops near the main and secondary faults, with the trend mainly oriented in the E-W direction and approximately parallel to the direction of the faults. The width of the fracture zone is largest in the central and southern part of the study area. These fractures are fault-related and are caused by regional stress fields resulting from the activity of the main-secondary faults.
1 Introduction
Due to the great success of the shale revolution in North America, unconventional oil and gas resources such as shale oil have gradually become the focus of global hydrocarbon exploration and development (Jarvie et al., 2007; Zou et al., 2013; He et al., 2016; 2017; Ghosh et al., 2018; Wang et al., 2019; Jin et al., 2021; Tao et al., 2021). Shale oil refers to petroleum that is stored in organic-rich shale strata, generally requiring special techniques such as horizontal wells and hydraulic fracturing to obtain industrial oil production (Gale et al., 2007; Jin et al., 2021; Tang et al., 2021). Exploration practices have confirmed that multiple sets of organic-rich shale strata exist in the lacustrine basins in China, including the Cretaceous in the Songliao Basin, the Permian in the Junggar Basin, the Triassic in the Ordos Basin, the Jurassic in the Sichuan Basin, and the Paleogene in the Bohai Bay Basin (Zou et al., 2013; Liu et al., 2018; Zhao et al., 2020; Jin et al., 2021; Zhang et al., 2022). Lacustrine shales are characterized by strong heterogeneity, low matrix permeability, high clay mineral content, and large compressibility changes, which constrain the deployment and large-scale development of lacustrine shale oil (Jin et al., 2021).
Previous studies have found that lacustrine shale strata have generally experienced multiple stages of tectonic movement, and the content of brittle minerals can reach over 40%, resulting in the widespread development of natural fractures in shale oil reservoirs (Zou et al., 2013; Jin et al., 2021; Tang et al., 2021). Shale oil reservoirs typically have extremely low matrix porosity and permeability, making it difficult to form an effective fluid flow system and to provide industrial oil flow in natural conditions (Gong et al., 2021; Zhang et al., 2022). Natural fractures, serving as fluid conduits for oil and gas transport from the matrix to the wellbore, can significantly improve reservoir properties and affect shale oil enrichment, production, and the development plan (Gale et al., 2007; Ding et al., 2012; Jarvie, 2012; Mastalerz et al., 2013; Zeng et al., 2016; Wang et al., 2018; Liu et al., 2022b; Yang et al., 2022). Previous researchers have conducted numerous works on the development characteristics, formation mechanisms, and controlling factors of natural fractures in conventional reservoirs, but few have focused on the distribution rules of lacustrine shale reservoirs (Laubach et al., 2004; Laubach and Ward, 2006; Laubach et al., 2019; Gale et al., 2010, 2014; Zeng et al., 2013; Wang et al., 2016a; Wang et al., 2016b; Lyu et al., 2019; Liu et al., 2020a; b; Liu et al., 2021a; Liu et al., 2022a).
In recent years, a set of organic-rich alkaline lake shale strata has developed in the Lower Permian Fengcheng Formation of the Mahu Sag, making it an important exploration area in the Junggar Basin (Tang et al., 2021; Zhi et al., 2021; Xia et al., 2022; Lu et al., 2023). Industrial oil flows from multiple drilling wells have displayed good exploration and development prospects. Previous studies have studied the regional structure, sedimentary background, reservoir lithology, and pore structure of the Fengcheng Formation, indicating that natural fractures are important reservoir spaces in the Fengcheng Formation (Tang et al., 2021; Wang et al., 2021; Zhi et al., 2021). Since the deposition in Permian, the Fengcheng Formation has experienced multi-stage tectonic movements such as Hercynian movement, Indosinian movement, Yanshanian movement and Himalayan movement (Zhou et al., 2019; Wang et al., 2022). In addition, the mixed deposition of terrigenous clast, endogenous carbonate and volcanic materials makes the lithology of the Fengcheng Formation extremely complex (Zhi et al., 2021). A variety of factors lead to the unclear distribution of natural fractures in the Fengcheng Formation, which has strong heterogeneity in the vertical and horizontal directions. Therefore, the study on the distribution pattern of natural fractures in the lacustrine shales of the Fengcheng Formation has important guiding significance for the formulation of subsequent exploration and development plans in the study area.
In this study, the Fengcheng Formation in the Mahu Sag was taken as the research object. Firstly, the fracture development characteristics of the Fengcheng Formation were described using core, imaging logs and thin section data. Then, XRD tests were used to analyze the differences of mineral composition in the Feng 1 Member, Feng 2 Member and Feng 3 Member. On this basis, the vertical pattern of fractures was discussed by characterizing the fracture distribution characteristics of single wells. Finally, the natural fractures identified by different attributes were stacked based on different seismic attributes, including curvature, coherence, likelihood and pre-stack AVAz, and the planar distribution pattern of natural fractures was evaluated. The research results will provide a geological basis for the next exploration and development of shale oil reservoirs in the Mahu Sag.
2 Geological setting
The Junggar Basin is a large superimposed petroliferous basin in Northwest China, located in the eastern part of the Kazakhstani Plate, with an area of approximately 13 × 104 km2 (Xiao et al., 2008; Cao et al., 2020; Tang et al., 2021; Wu et al., 2022). The Mahu Sag is a secondary tectonic unit in the basin, which is the hydrocarbon generation sag with the highest degree of oil and gas enrichment. (Liu et al., 2016; Guo et al., 2021; Tang et al., 2021; Zhi et al., 2021; Wu et al., 2022). The Mahu sag is a Carboniferous-Quaternary sag formed on the pre-Carboniferous fold basement and controlled by multi-period peripheral thrust activities. It is surrounded by the Wuxia Fault Zone, Kebai Fault Zone, and Zhongguai Uplift in the west and the Shiyingtan Uplift, Yingxi Sag, Xiayan Uplift, and Dabasong Uplift in the east (Zhang et al., 2019; Li et al., 2021; Lu et al., 2023). The sag is distributed in the northeast-southwest direction, with an E-W length of about 50 km, a N-S length of about 120 km, and an area of about 5,000 km2 (Figure 1) (Xia et al., 2020; Zhi et al., 2021). The Mahu 131 Well Block is located in the northern part of the sag, and multiple wells drilled in the area have shown industrial oil flows. The Well B2 achieved a maximum daily oil production of 50 tons from a depth of 4,581–4,850 m, indicating abundant shale oil resources (Zhi et al., 2021; Tang et al., 2023).
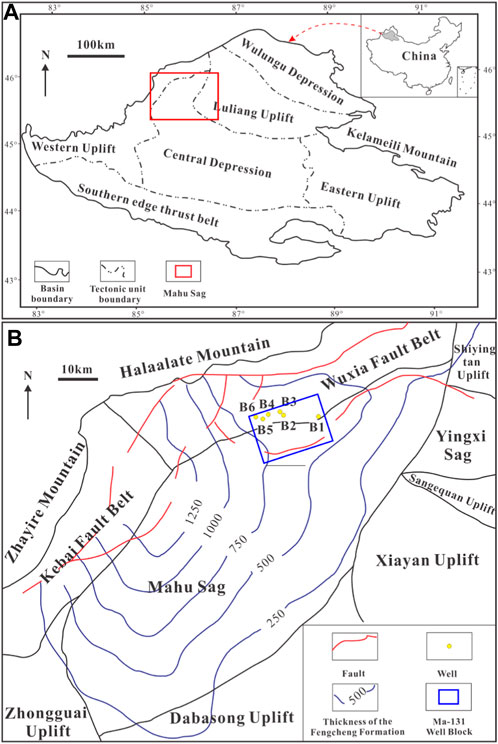
FIGURE 1. (A) Tectonic unit of Junggar Basin. (B) Location of the Mahu Sag and Ma-131 Well Block (Modified from Wang et al., 2022).
The Carboniferous to Cretaceous strata is developed from bottom to top in the sag, among which the Lower Permian Fengcheng Formation develops a set of fan delta-lake sedimentary system, which is a significant regional hydrocarbon source rock (Song et al., 2022; tang et al., 2023). The rock types of the Fengcheng Formation are diverse, including carbonate rocks, clastic rocks and volcanic rocks, reflecting the characteristics of mixed deposition, with a total depositional thickness of 800–1800 m (Zhi et al., 2021; Song et al., 2022). It can be subdivided into three members based on lithologic and electrical characteristics: Feng 1 Member (P1f1), Feng 2 Member (P1f2), and Feng 3 Member (P1f3), each with distinct sedimentary facies (Figure 2 Figure Fig3) (Tang et al., 2021; Wang et al., 2021; Zhi et al., 2021). During the deposition of the Feng 1 Member, the volcanic activity was intense and the supply of terrestrial material was limited, resulting in the development of volcanic clastic rocks. During the Feng 2 Member period, the climate became arid and the salinity of the lake basin increased, leading to the development of dolomitic rocks, dolomitic mudstones, and evaporites. During the sedimentary period of Feng 3 Member, the salinity of the basin decreased, and dolomitic rocks dominated with clastic rocks at the top (Zhang et al., 2018). The various lithologies exhibit a mutually exclusive relationship in different areas, with carbonate sedimentation dominating in the center of the basin, reflecting typical characteristics of a saline lake (Zhang et al., 2018; Zhi et al., 2021).
3 Methods
3.1 X-ray diffraction analysis
XRD was conducted using a German Bruker D8 ADVANCE X-ray diffractometer, completed at the SINOPEC Wuxi Petroleum Geology Research Institute in China. Prior to XRD testing, rock samples were ground into powder with a particle size of 200 mesh. Approximately 10 g of powder was mixed thoroughly with ethanol, and the sample was prepared using a pressing method for testing. Mineral types and mass percentages were calculated by comparing the diffraction peak intensities of standard samples and measuring the area under the curve of the main peaks of each mineral (Gou et al., 2021). All testing procedures followed the China Oil and Gas Industry Standard SY/T 6010-2018.
3.2 Fracture characterization
In this study, natural fractures of the Permian Fengcheng Formation in the Mahu Sag were studied based on different databases, including cores, thin sections, and imaging logs. The investigated wells are drilled vertically and located on the slope in the northern part of the sag, as shown in Figure 1B. Core observations were conducted at the core library of the PetroChina Xinjiang Oilfield Company. Additionally, thin sections (with a thickness of 30 μm) were prepared to observe the micro-features of the fractures, using a Nikon LV100N POL microscope at the School of Earth and Space Sciences at Peking University. Furthermore, fracture analysis was performed using imaging logs collected by a full-borehole micro-scanner imaging tool (FMI). The drilling employed conductive mud, hence unfilled fractures penetrated by drilling fluids are displayed as dark curves, while fractures filled with minerals are displayed as bright curves due to their high electrical resistivity (Lyu et al., 2019).
3.3 Seismic attribute extraction
Seismic waves propagating in fractured media exhibit anisotropic characteristics in terms of attributes such as amplitude, velocity, attenuation, and travel time, which can be used to predict the development intensity of fractures by analyzing their azimuthal variation (Shen et al., 2002; Liu et al., 2021b). Various seismic attributes have different resolutions and different ability to identify fractures (Wang et al., 2022). Post-stack seismic attributes such as curvature, dip angle, variance, coherence, and azimuth have good identification effects on large-scale fractures, and can identify fractures larger than 1/4 wavelength. Compared to post-stack seismic attributes, likelihood attributes contain dip angle and azimuth information, and pre-stack AVAz retains shot-receiver offset and azimuth information, which have good identification effects on medium and small-scale fractures. In this study, large-scale fractures were first identified using curvature, coherence and likelihood attributes, and then medium and small-scale fractures were identified using pre-stack AVAz attributes. The comprehensive identification result of the Fengcheng Formation fractures in the Mahu sag was obtained by overlaying the results of the two identification methods. In addition, the dominant frequency and bandwidth of the 3D seismic data in the Ma131 Well Block are 25 Hz and 50 Hz, respectively.
4 Results
4.1 Mineral composition
All samples of the Fengcheng Formation are rich in carbonate minerals, quartz, and feldspar, with average contents of 31.6%, 32.6%, and 18.1%, respectively, while the content of clay minerals and pyrite is relatively low, with average contents of 10.5% and 4.6%, respectively. The mineral content in different layers varies to some extent in the vertical direction. In the Feng 1 Member, the content of quartz is the highest, with an average content of 33.0%, followed by feldspar and carbonate minerals, with average contents of 28.1% and 18.6%, respectively. The mineral composition of the Feng 2 Member and the Feng 3 Member is similar, with carbonate minerals being the highest, averaging 38.8% and 33.5%, respectively. The content of quartz is lower with an average of 33.9% and 30.7% respectively. Feldspar is the least abundant, averaging 13.6% and 15.3%, respectively. Therefore, the Feng 2 Member and the Feng 3 Member have similar mechanical properties, which are significantly different from those of the Feng 1 Member.
4.2 Fracture characteristics
Core and thin section observations and imaging log interpretation results show that natural fractures in the Fengcheng Formation are widely developed in the study area. Natural fractures can be classified into three types according to their origin: transformational shear fractures, intraformational open fractures and bed-parallel shear fractures. The transformational shear fractures are generally large in size with a straight fracture surface and a dip angle of nearly 90°. They traverse one or more rock strata boundaries vertically and extend a long distance. In Figure 4A, the height of the transformational shear fractures exceeds 40 cm. The intraformational open fractures develop within the rock strata and are relatively small in size. Their longitudinal extension is significantly restricted by the rock strata boundary, and their vertical extension is short and intersects the rock strata boundary at a high angle. In Figure 4B, the height of the intraformational open fractures is less than 15cm, and it is possible to observe oil seeping out along the fractures. The bed-parallel shear fractures are parallel to the bedding plane with a relatively low dip angle, and their fracture surfaces show scratch marks, steps, and mirror surfaces (Figure 4C). Among the tectonic fractures in the Fengcheng Formation, the intraformational open fractures are the most developed, while transformational shear fractures and bed-parallel shear fractures are relatively less.
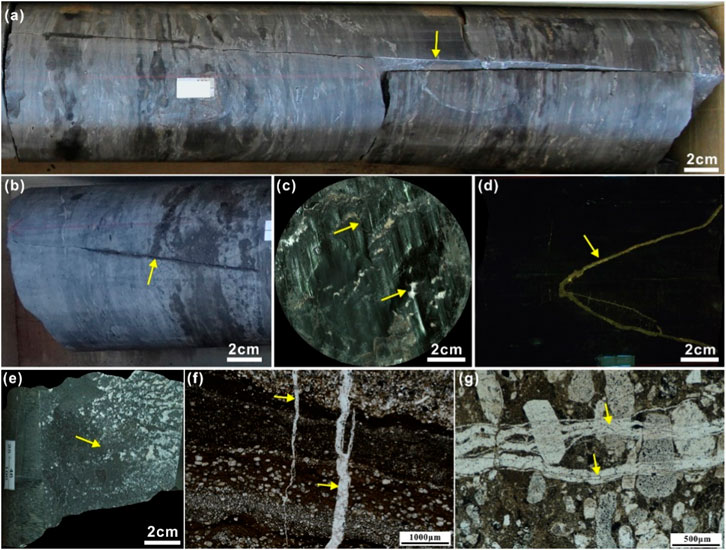
FIGURE 4. Tectonic fractures in the Fengcheng Formation in cores and thin sections in the Mahu Sag. (A) Transformational shear fracture, Well B2, 4,752.37 m. (B) Intraformational open fracture contains oil, Well B2, 4,722.1 m. (C) Bed-parallel shear fracture, Well B2, 4,713.9 m. (D) Fluorescence image indicates that fracture contains oil, Well B2, 4,746.47 m. (E) High-angle fracture surface is partially filled with mineral, Well B2, 4,872.5 m. (F) High-angle fracture is filled with mineral, Well B2, 4,650.3 m. (G) High-angle fracture is filled with mineral, Well B2, 4,616.14 m.
According to the degree of mineral cement filling in the fractures, natural fractures can be divided into three types: unfilled, partially filled, and fully filled fractures, and the effectiveness of fractures decreases in that order (Zeng et al., 2013). Most of the fractures observed in the cores are effective fractures, including unfilled fractures (Figures 4B,D) and partially filled fractures (Figure 4E), which can serve as storage space and migration channels for shale oil. Oil is visible in the fractures observed in the cores, and the fluorescence of the fractures under UV light indicates the presence of residual hydrocarbons (Figures 4B,D). The fractures observed in thin sections are mostly filled with minerals, such as quartz, calcite, and reedmergnerite (Figures 4F,G), showing features of cutting through lamination interfaces or mineral particles.
A large number of induced fractures, conductive fractures, resistive fractures and a small number of small faults can be identified in the imaging log images (Figure 5). The induced fractures are a group of parallel and 180° symmetrical high-angle fractures, and their direction is mainly near E-W, reflecting that the present maximum horizontal principal stress direction is near E-W (Lyu et al., 2019). The conductive fractures appear as dark sinusoidal curves as the open fractures are invaded by drilling mud (Figure 5A). The direction of the conductive fractures is mainly NE-SW, with low dip angles being dominant. Because their orientation is closer to the maximum horizontal principal stress direction, the conductive fractures have large aperture and good effectiveness. The resistive fractures in the Fengcheng Formation are relatively developed, showing bright sinusoidal curves, which are completely filled and having no contribution to the reservoir (Figure 5B). They are mainly in the N-S direction with medium and high dip angles, perpendicular to the direction of the maximum horizontal principal stress, and exhibiting a closure feature. Furthermore, the imaging characteristics of small faults are similar to those of conductive or resistive fractures, with obvious lithological changes or displacement visible (Figure 5C).
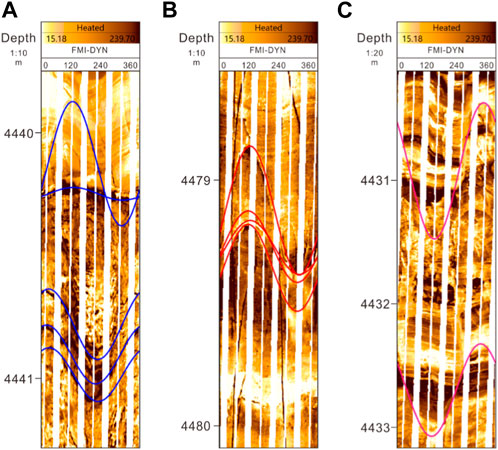
FIGURE 5. Natural fractures in the Fengcheng Formation detected by the borehole image logs in Well B6 in the Mahu Sag. (A) Conductive fractures. (B) Resistive fractures. (C) Small faults.
4.3 Seismic attribute characteristics
The pink area of the predicted results of the coherence attribute is the low value area, which is the large-scale fracture development zone, distributed in the western, eastern and southern parts of the study area, closer to Well B1 and further away from Wells B2-B5 (Figure 6A). The curvature attribute has a good effect on identifying secondary fractures, with the red area being the area of medium to high values of the curvature attribute, representing secondary fractures, and the blue area being the area of relatively low values, representing fault-related fracture development zones (Figure 6B). The secondary faults are mainly in the near E-W direction, distributed around the primary faults, located in the south and middle of the study area. Wells B1 and B2 are located in the area of high curvature properties, indicating the proximity of Wells B1 and B2 to the secondary fractures and the development of fault-related fractures.
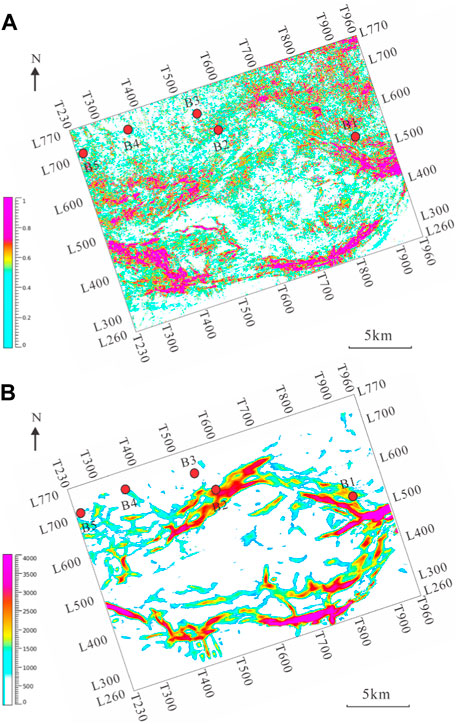
FIGURE 6. Plane distribution of fractue identification results of coherent and curvature seismic attributes. (A) Plane distribution of coherent attributes. (B) Plane distribution of curvature attributes.
The Likelihood attribute contains information such as azimuth and dip, and can clearly depict the development of large-scale fractures in the region and accurately detect the boundaries of fracture zones. In the prediction results of the likelihood attribute, the deeper the color (blue), the greater the probability of fractures, and the lighter the color (green, yellow), the smaller the probability of fractures. Because the Likelihood attribute can predict the abnormal information that is not detected in the coherence and curvature attributes, the ability to identify factures is stronger. The results show that large-scale fractures are mainly distributed in the central and northern parts of the study area, as well as the southernmost part, and are more developed near Wells B2 and B1 (Figure 7A).
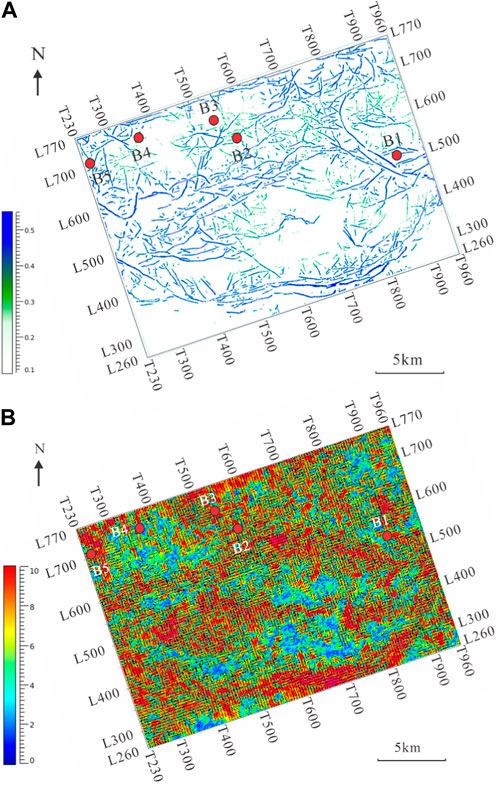
FIGURE 7. Plane distribution of likelihood seismic attributes and AVAZ fracture intensity. (A) Plane distribution of likelihood attributes. (B) Plane Distribution of AVAZ fracture intensity.
Small and medium scale fractures are relatively smaller in size but more numerous. In this study, the pre-stack AVAz method was used to predict small and medium scale fractures. The red area near Wells B2 and B3 represents the area with the highest fracture intensity, while the yellow and blue areas represent areas with lower fracture intensity (Figure 7B). Small and medium scale fractures in the Fengcheng Formation have a relatively strong overall development intensity, especially in the northern and central regions, and in the southern part near the main fault, with a predominant orientation parallel to the main fault in the near E-W direction. The northwest direction of Well B2 is the high fracture development area, the area between Wells B2 and B3 is the moderate fracture development area, and the area near Well B4 is the weak fracture development area.
5 Discussion
5.1 Vertical distribution law of fracture
The differences in lithology and mineral composition of shale reservoirs lead to different rock mechanical properties and brittleness, which in turn affects the development of natural fractures in shale (Zeng et al., 2022). According to the imaging log interpretation results of four wells in the study area, including Well B2, Well B3, Well B4, and Well B6, the development of natural fractures in the Fengcheng Formation is obviously controlled by the lithology and mineral composition, and the development degree of fractures varies greatly in different layers. The Feng 1 Member, with a thickness exceeding 100 m and high content of feldspar and quartz., mainly consists of basalt and tuff, as well as small amounts of conglomerate, fine sandstone, siltstone, and shale (Figure 2). The Feng 1 Member high-angle fractures is dominated by fractures with high dip angles, and the development degree of fractures with low and medium dip angles is relatively low (Figure 8). The fracture density of the Feng 1 Member in Well B2 is 1.16 m−1. The Feng 2 Member, with a thickness of about 220 m, is mainly composed of argillaceous limestone and shale. The content of carbonate minerals in the Feng 2 Member is high, thus the rock is brittle, and fractures with high and medium dip angles are both developed. The fracture density of the Feng 2 Member in Well B2 is 1.82 m−1. The lithology of the Feng 3 Member is similar to that of the Feng 2 Member, and fractures with high and medium dip angles are also developed. Moreover, a small number of fractures with low dip angles are developed in clastic rocks at the top of Feng 2 Member. The fracture density of the Feng 1 Member in Well B2 is 2.31 m−1 (Figure 9). In summary, the Feng 1 Member is mainly composed of volcanic rocks, with low content of carbonate minerals and low brittleness, which leads to low development degree of fracture. The Feng 2 Member and Feng 3 Member are mainly composed of dolomite and argillaceous limestone, with high content of carbonate minerals and higher brittleness, which result in high development degree of fracture (Figure 9). This is because the higher the content of brittle minerals, the greater the brittleness, and fractures will be formed in shale reservoirs when small strains occur under the same tectonic stress (Zeng et al., 2022).
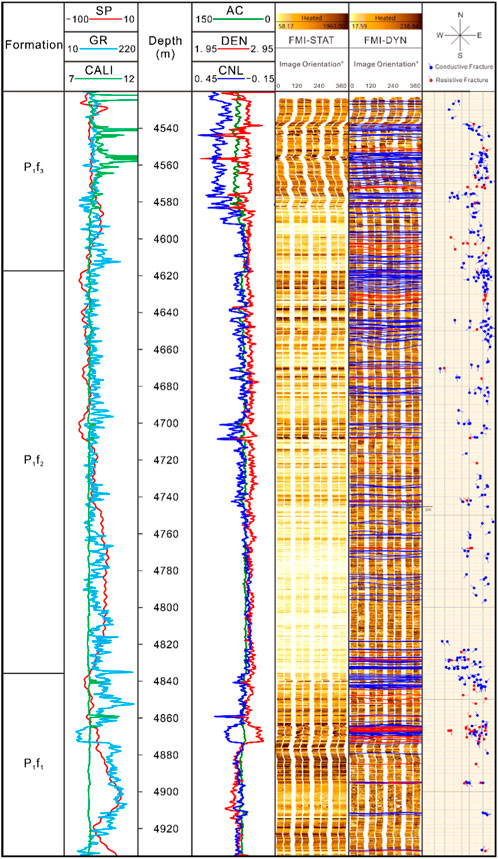
FIGURE 8. Vertical distribution of natural fractures in the Fengcheng Formation in Well B2 in the Mahu Sag.
In addition, different orders of faults in the Mahu Sag influence the development of natural fractures in different single wells. The Well B2 is closest to the fault and has the highest degree of fracture development in the Fengcheng Formation, with a fracture density of 1.69 m−1. The fracture development degree of Well B6 and Well B4 is relatively low, with fracture densities of 1.55 m−1 and 1.36 m−1, respectively. The Well B3 is the furthest from the fault and the fracture development degree is the lowest, with a fracture density of only 0.74 m−1. This is because there is a fault damage zone near the fault, and the development of natural fractures is affected by the local stress field around the fault. Generally, the closer the distance to the fault, the higher the degree of fracture development (Zeng and Li, 2009; Griffith and Prakash, 2015).
5.2 Plane distribution law of fracture
Due to the fact that different seismic attributes can characterize the distribution of fractures at different scales, superimposing seismic attribute maps of the same layer can be used to analyze the similarities and differences in the distribution patterns of fractures at different scales, and to discuss whether there is any correlation between the origin of fractures at different scales.
Overall, the main and secondary faults of the Fengcheng Formation are distributed in the central and southern parts of the study area, with the trend of near E-W direction.
The zone where large-scale fractures develop is widest in the central and southern parts of the study area, with a predominant trend in the E-W direction and a secondary trend in the S-N direction (Figure 10A). Small and medium scale fractures are well developed in the study area, and their trends are mainly in the E-W and NW-SE directions, which are approximately parallel or obliquely intersected with the large-scale fractures and main-secondary faults (Figure 10B). Locally, the northern part of the study area near Well B4 is a zone of weak fracture development, while the area between Well B3 and Well B1 is a zone of fracture development. The plateau area in the central part of the study area and the southern part near the main-secondary fault zone are both areas of large-scale and medium-small-scale fracture development, and the fractures are mostly oriented in the direction of the maximum horizontal principal stress.
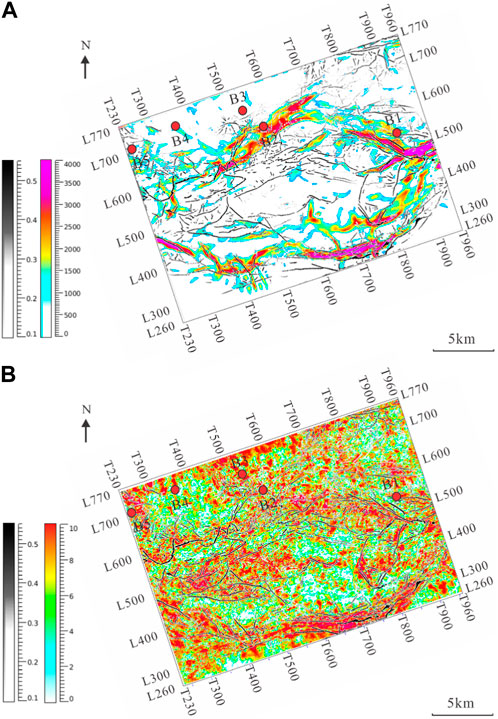
FIGURE 10. Superimposed plane distribution map of pre-stack and post-stack attribute. (A) Superimposed plane distribution map of likelihood attribute and curvature attribute. (B) Superimposed plane distribution map of pre-stack fracture intensity and likelihood attribute.
Based on the above analysis, large-scale fractures mainly develop near the main-secondary faults and trend approximately parallel to them. They are mainly fault-related fractures resulting from the activity of the main-secondary faults and the effects of regional stress field. Small and medium scale fractures are the most developed in the zone where large-scale fractures develop and nearby, indicating that indicating that they are mainly controlled by fault activity and local stress field during the formation of large-scale fractures.
6 Conclusion
(1) Different types of natural fractures are existed in the Fengcheng Formation of the Mahu Sag, including transformational shear fractures, intraformational open fractures and bed-parallel shear fractures, with intraformational open fractures being the most developed. Moreover, induced fractures, conductive fractures, resistive fractures, and small faults are identified by imaging logs, with good effectiveness of conductive fractures oriented close to the direction of present maximum horizontal principal stress.
(2) There are great differences in the development degree of natural fractures in different layers of Fengcheng formation in the vertical direction. The basalt and tuff are developed in the Feng 1 Member, with low carbonate mineral content and low brittleness, thus the degree of fracture development is relatively low. The dolomite and argillaceous dolomite are developed in the Feng 2 Member and the Feng 3 Member, with high carbonate mineral content and high brittleness, and the degree of fracture development is high. In addition, the closer to the fault, the higher the degree of fracture development in different single wells.
(3) On the plane, the natural fracture development zone is located near the main-secondary faults, with the trend of mainly E-W, approximately parallel to the direction of the faults. The width of the fracture zone is largest in the central and southern part of the study area, and they are fault-related fractures under the action of regional stress fields caused by the activity of the hanging wall and footwall of the main-secondary faults.
Data availability statement
The original contributions presented in the study are included in the article/Supplementary Material, further inquiries can be directed to the corresponding author.
Author contributions
GL, ZJ, and LZ contributed to conception and design of the study. SY and WH organized the database. XD and GL performed the statistical analysis. GL wrote the first draft of the manuscript. XD and GL wrote sections of the manuscript. All authors listed have made a substantial, direct, and intellectual contribution to the work and approved it for publication.
Funding
This research was supported by the National Natural Science Foundation of China (No.42090025), State Key Laboratory of Shale Oil and Gas Enrichment Mechanisms and Effective Development (33550000-22-ZC0613-0336), and CNPC Innovation Found (2023DQ02-0103).
Acknowledgments
We are particularly grateful to the editors and two reviewers for their constructive comments, which will contribute significantly to the improvement of this manuscript.
Conflict of Interest
The authors declare that the research was conducted in the absence of any commercial or financial relationships that could be construed as a potential conflict of interest.
Publisher’s note
All claims expressed in this article are solely those of the authors and do not necessarily represent those of their affiliated organizations, or those of the publisher, the editors and the reviewers. Any product that may be evaluated in this article, or claim that may be made by its manufacturer, is not guaranteed or endorsed by the publisher.
References
Cao, J., Xia, L., Wang, T., Zhi, D., Tang, Y., and Li, W. (2020). An alkaline lake in the late paleozoic ice age (lpia): A review and new insights into paleoenvironment and petroleum geology. Earth Sci. Rev. 202, 103091. doi:10.1016/j.earscirev.2020.103091
Ding, W., Li, C., Li, C., Xu, C., Jiu, K., Zeng, W., et al. (2012). Fracture development in shale and its relationship to gas accumulation. Geosci. Front. 3, 97–105. doi:10.1016/j.gsf.2011.10.001
Gale, J. F. W., Lander, R. H., Reed, R. M., and Laubach, S. E. (2010). Modeling fracture porosity evolution in dolostone. J. Struct. Geol. 32, 1201–1211. doi:10.1016/j.jsg.2009.04.018
Gale, J. F. W., Reed, R. M., and Holder, J. (2007). Natural fractures in the Barnett Shale and their importance for hydraulic fracture treatments. AAPG Bull. 91, 603–622. doi:10.1306/11010606061
Ghosh, S., Galvis-Portilla, H. A., Klockow, C. M., and Slatt, R. M. (2018). An application of outcrop analogues to understanding the origin and abundance of natural fractures in the Woodford Shale. J. Petroleum Sci. Eng. 164, 623–639. doi:10.1016/j.petrol.2017.11.073
Gong, L., Wang, J., Gao, S., Fu, X., Liu, B., Miao, F., et al. (2021). Characterization, controlling factors and evolution of fracture effectiveness in shale oil reservoirs. J. Petroleum Sci. Eng. 203, 108655. doi:10.1016/j.petrol.2021.108655
Gou, Q., Xu, S., Hao, F., Yang, F., Shu, Z., and Liu, R. (2021). The effect of tectonic deformation and preservation condition on the shale pore structure using adsorption-based textural quantification and 3D image observation. Energy 219, 119579. doi:10.1016/j.energy.2020.119579
Griffith, W. A., and Prakash, V. (2015). Integrating field observations and fracture mechanics models to constrain seismic source parameters for ancient earthquakes. Geology 43, 763–766. doi:10.1130/g36773.1
Guo, P., Wen, H., Gibert, L., Jin, J., Jiang, Y., and Wang, G. (2021). Controlling factors of high-quality hydrocarbon source rocks developed in lacustrine shallow-water zone of the Junggar Basin, northwestern China. AAPG Bull. 105, 2063–2092. doi:10.1306/03122119013
He, J., Ding, W., Jiang, Z., Jiu, K., Li, A., and Sun, Y. (2017). Mineralogical and chemical distribution of the Es3L oil shale in the Jiyang Depression, Bohai Bay Basin (E China): Implications for paleoenvironmental reconstruction and organic matter accumulation. Mar. Pet. Geol. 81, 196–219. doi:10.1016/j.marpetgeo.2017.01.007
He, J., Ding, W., Zhang, J., Li, A., Zhao, W., and Dai, P. (2016). Logging identification and characteristic analysis of marine–continental transitional organic-rich shale in the Carboniferous-Permian strata, Bohai Bay Basin. Mar. Pet. Geol. 70, 273–293. doi:10.1016/j.marpetgeo.2015.12.006
Jarvie, D. M., Hill, R. J., Ruble, T. E., and Pollastro, R. M. (2007). Unconventional shale-gas systems: The Mississippian Barnett Shale of north-central Texas as one model for thermogenic shale-gas assessment. AAPG Bull. 91, 475–499. doi:10.1306/12190606068
Jarvie, D. M. (2012). Shale resource systems for oil and gas: Part 2—shale-oil resource systems. AAPG Mem. 97, 89–119. doi:10.1306/13321447M973489
Jin, Z., Zhu, R., Liang, X., and Shen, Y. (2021). Several issues worthy of attention in current lacustrine shale oil exploration and development. Pet. Explor. Dev. 48, 1471–1484. doi:10.1016/s1876-3804(21)60303-8
Laubach, S. E., Lander, R. H., Criscenti, L. J., Anovitz, L. M., Urai, J. L., Pollyea, R. M., et al. (2019). The role of chemistry in fracture pattern development and opportunities to advance interpretations of geological materials. Rev. Geophys. 57, 1065–1111. doi:10.1029/2019rg000671
Laubach, S. E., Reed, R. M., Olson, J. E., Lander, R. H., and Bonnell, L. M. (2004). Coevolution of crack-seal texture and fracture porosity in sedimentary rocks: Cathodoluminescence observations of regional fractures. J. Struct. Geol. 26, 967–982. doi:10.1016/j.jsg.2003.08.019
Laubach, S. E., and Ward, M. E. (2006). Diagenesis in porosity evolution of opening-mode fractures, middle triassic to lower jurassic La boca formation, NE Mexico. Tectonophysics 419, 75–97. doi:10.1016/j.tecto.2006.03.020
Li, W., Cao, J., Zhi, D., Tang, Y., He, W., Wang, T., et al. (2021). Controls on shale oil accumulation in alkaline lacustrine settings: Late Paleozoic Fengcheng Formation, northwestern Junggar Basin. Mar. Pet. Geol. 129, 105107. doi:10.1016/j.marpetgeo.2021.105107
Liu, G., Chen, Z., Wang, X., Gao, G., Xiang, B., Ren, J., et al. (2016). Migration and accumulation of crude oils from Permian lacustrine source rocks to Triassic reservoirs in the Mahu depression of Junggar Basin, NW China: Constraints from pyrrolic nitrogen compounds and fluid inclusion analysis. Org. Geochem. 101, 82–98. doi:10.1016/j.orggeochem.2016.08.013
Liu, G., Zeng, L., Li, H., Ostadhassan, M., and Rabiei, M. (2020a). Natural fractures in metamorphic basement reservoirs in the Liaohe Basin, China. Mar. Pet. Geol. 119, 104479. doi:10.1016/j.marpetgeo.2020.104479
Liu, G., Zeng, L., Sun, G., Zu, K., Qin, L., Mao, Z., et al. (2020b). Natural fractures in tight gas volcanic reservoirs and their influences on production in the Xujiaweizi depression, Songliao Basin, China. AAPG Bull. 104, 2099–2123. doi:10.1306/05122017169
Liu, J., Chen, P., Xu, K., Yang, H., Liu, H., and Liu, Y. (2022a). Fracture stratigraphy and mechanical stratigraphy in sandstone: A multiscale quantitative analysis. Mar. Pet. Geol. 145, 105891. doi:10.1016/j.marpetgeo.2022.105891
Liu, J., Ding, W., Wang, R., Yang, H., Wang, X., and Li, A. (2018). Methodology for quantitative prediction of fracture sealing with a case study of the lower Cambrian Niutitang Formation in the Cen'gong block in South China. J. Petroleum Sci. Eng. 160, 565–581. doi:10.1016/j.petrol.2017.10.046
Liu, J., Ding, W., Yang, H., and Liu, Y. (2021a). Quantitative multiparameter prediction of fractured tight sandstone reservoirs: A case study of the yanchang Formation of the Ordos Basin, central China. SPE J. 26, 3342–3373. doi:10.2118/205495-pa
Liu, J., Han, L., Shi, L., Chen, S., Lv, W., Zhang, T. G., et al. (2021b). CypB promotes cell proliferation and metastasis in endometrial carcinoma. Oil Gas Geol. 42, 747–754. doi:10.1186/s12885-021-08374-7
Liu, J., Mei, L., Ding, W., Xu, K., Yang, H., and Liu, Y. (2022b). Asymmetric propagation mechanism of hydraulic fracture networks in continental reservoirs. GSA Bull. 135, 678–688. doi:10.1130/b36358.1
Lu, G., Zeng, L., Dong, S., Huang, L., Liu, G., Ostadhassan, M., et al. (2023). Lithology identification using graph neural network in continental shale oil reservoirs: A case study in Mahu sag, Junggar Basin, western China. Mar. Pet. Geol. 150, 106168. doi:10.1016/j.marpetgeo.2023.106168
Lyu, W., Zeng, L., Zhou, S., Du, X., Xia, D., Liu, G., et al. (2019). Natural fractures in tight-oil sandstones: A case study of the upper triassic yanchang Formation in the southwestern Ordos Basin, China. AAPG Bull. 103, 2343–2367. doi:10.1306/0130191608617115
Mastalerz, M., Schimmelmann, A., Drobniak, A., and Chen, Y. (2013). Porosity of Devonian and Mississippian New Albany Shale across a maturation gradient: Insights from organic petrology, gas adsorption, and mercury intrusion. AAPG Bull. 97, 1621–1643. doi:10.1306/04011312194
Shen, F., Sierra, J., Burns, D. R., and Toksöz, M. N. (2002). Azimuthal offset-dependent attributes applied to fracture detection in a carbonate reservoir. Geophysics 67, 355–364. doi:10.1190/1.1468596
Song, Y., Yang, Z., He, W., Gan, R., Zhang, R., Huang, L., et al. (2022). Exploration progress of alkaline lake type shale oil of the permian Fengcheng Formation in Mahu sag, Junggar Basin (in Chinese with English abstract). China Pet. explor. 27, 60–72.
Tang, Y., Cao, J., He, W., Guo, X., Zhao, K., and Li, W. (2021). Discovery of shale oil in alkaline lacustrine basins: The late paleozoic Fengcheng Formation, Mahu sag, Junggar Basin, China. Pet. Sci. 18, 1281–1293. doi:10.1016/j.petsci.2021.04.001
Tang, Y., He, W., Jiang, Y., Fei, L., Shan, X., Zhao, Y., et al. (2023). Enrichment conditions and exploration direction of Permian saline lacustrine shale oil and gas in Junggar Basin (in Chinese with English abstract). Acta Pet. Sin. 44, 125–143.
Tao, K., Cao, J., Hu, W., Zhi, D., Lei, D., Tang, Y., et al. (2021). Petroleum system for the continuous oil play in the lacustrine Lower Triassic, Junggar Basin, China. AAPG Bull. 105, 2349–2380. doi:10.1306/07022119211
Wang, R., Ding, W., Zhang, Y., Wang, Z., Wang, X., He, J., et al. (2016a). Analysis of developmental characteristics and dominant factors of fractures in lower cambrian marine shale reservoirs: A case study of niutitang formation in cen’gong block, southern China. J. Petroleum Sci. Eng. 138, 31–49. doi:10.1016/j.petrol.2015.12.004
Wang, R., Gu, Y., Ding, W., Gong, D., Yin, S., Wang, X., et al. (2016b). Characteristics and dominant controlling factors of organic-rich marine shales with high thermal maturity: A case study of the lower cambrian niutitang Formation in the cen’gong block, southern China. J. Nat. Gas. Sci. Eng. 33, 81–96. doi:10.1016/j.jngse.2016.05.009
Wang, R., Hu, Z., Long, S., Liu, G., Zhao, J., Dong, L., et al. (2019). Differential characteristics of the upper ordovician-lower silurian wufeng-longmaxi shale reservoir and its implications for exploration and development of shale gas in/around the Sichuan Basin. Acta Geol. Sin. - Engl. Ed. 93, 520–535. doi:10.1111/1755-6724.13875
Wang, R., Hu, Z., Sun, C., Liu, Z., Zhang, C., Gao, B., et al. (2018). Comparative analysis of shale reservoir characteristics in the wufeng-longmaxi (O3w-S1l) and niutitang (Є1n) formations: A case study of wells JY1 and TX1 in the southeastern Sichuan Basin and its neighboring areas, southwestern China. Interpretation 6, SN31–SN45. doi:10.1190/int-2018-0024.1
Wang, S., Wang, G., Huang, L., Song, L., Zhang, Y., Li, D., et al. (2021). Logging evaluation of lamina structure and reservoir quality in shale oil reservoir of Fengcheng Formation in Mahu Sag, China. Mar. Pet. Geol. 133, 105299. doi:10.1016/j.marpetgeo.2021.105299
Wang, X., Jin, Z., Chen, G., Peng, M., Huang, L., Wang, Z., et al. (2022). Multi-scale natural fracture prediction in continental shale oil reservoirs: A case study of the Fengcheng Formation in the Mahu sag, Junggar Basin, China. Front. Earth Sci. 10. doi:10.3389/feart.2022.929467
Wu, Y., Liu, C., Jiang, F., Hu, T., Lv, J., Zhang, C., et al. (2022). Geological characteristics and shale oil potential of alkaline lacustrine source rock in Fengcheng Formation of the Mahu Sag, Junggar Basin, Western China. J. Petroleum Sci. Eng. 216, 110823. doi:10.1016/j.petrol.2022.110823
Xia, L., Cao, J., Stüeken, E. E., Zhi, D., Wang, T., and Li, W. (2020). Unsynchronized evolution of salinity and pH of a permian alkaline lake influenced by hydrothermal fluids: A multi-proxy geochemical study. Chem. Geol. 541, 119581. doi:10.1016/j.chemgeo.2020.119581
Xiao, W., Han, C., Yuan, C., Sun, M., Lin, S., Chen, H., et al. (2008). Middle cambrian to permian subduction-related accretionary orogenesis of northern Xinjiang, NW China: Implications for the tectonic evolution of central asia. J. Asian Earth Sci. 32, 102–117. doi:10.1016/j.jseaes.2007.10.008
Yang, Z., Wang, X., Ge, H., Zhu, J., and Wen, Y. (2022). Study on evaluation method of fracture forming ability of shale oil reservoirs in Fengcheng Formation, Mahu sag. J. Petroleum Sci. Eng. 215, 110576. doi:10.1016/j.petrol.2022.110576
Zeng, L., and Li, X. (2009). Fractures in sandstone reservoirs with ultra-low permeability: A case study of the upper triassic yanchang Formation in the Ordos Basin, China. AAPG Bull. 93, 461–477. doi:10.1306/09240808047
Zeng, L., Ma, S., Tian, H., Xue, M., Liu, G., and Lyu, W. (2022). Research progress of natural fractures in organic rich shale (in Chinese with English abstract). Earth Sci., 1–15.
Zeng, L., Su, H., Tang, X., Peng, Y., and Gong, L. (2013). Fractured tight sandstone oil and gas reservoirs: A new play type in the dongpu depression, Bohai Bay Basin, China. AAPG Bull. 97, 363–377. doi:10.1306/09121212057
Zhang, C., Liu, D., Jiang, Z., Song, Y., Luo, Q., and Wang, X. (2022). Mechanism for the formation of natural fractures and their effects on shale oil accumulation in Junggar Basin, NW China. Int. J. Coal Geol. 254, 103973. doi:10.1016/j.coal.2022.103973
Zhang, G., Wang, Z., Guo, X., Sun, Y., Sun, L., and Pan, L. (2019). Characteristics of lacustrine dolomitic rock reservoir and accumulation of tight oil in the Permian Fengcheng Formation, the Western slope of the Mahu Sag, Junggar Basin, NW China. J. Asian Earth Sci. 178, 64–80. doi:10.1016/j.jseaes.2019.01.002
Zhang, Z., Yuan, X., Wang, M., Zhou, C., Tang, Y., Chen, X., et al. (2018). Alkaline-lacustrine deposition and paleoenvironmental evolution in permian Fengcheng Formation at the Mahu sag, Junggar Basin, NW China. Pet. Explor. Dev. 45, 1036–1049. doi:10.1016/s1876-3804(18)30107-1
Zhao, W., Hu, S., Hou, L., Yang, T., Li, X., Guo, B., et al. (2020). Types and resource potential of continental shale oil in China and its boundary with tight oil. Pet. Explor. Dev. 47, 1–11. doi:10.1016/s1876-3804(20)60001-5
Zhi, D., Tang, Y., He, W., Guo, X., Zheng, M., and Huang, L. (2021). Orderly coexistence and accumulation models of conventional and unconventional hydrocarbons in lower permian Fengcheng Formation, Mahu sag, Junggar Basin. Pet. Explor. Dev. 48, 43–59. doi:10.1016/s1876-3804(21)60004-6
Zhou, L., Zhu, J., Song, Y., Lu, P., Qu, J., You, X., et al. (2019). Analysis of fault characteristics and reservoir control in Triassic Baikouquan Formation in central and eastern Mahu depressin (in Chinese with English abstract). Earth Sci. Front. 26, 248–261.
Zou, C., Yang, Z., Cui, J., Zhu, R., Hou, L., Tao, S., et al. (2013). Formation mechanism, geological characteristics and development strategy of nonmarine shale oil in China. Pet. Explor. Dev. 40, 15–27. doi:10.1016/s1876-3804(13)60002-6
Zeng, L., Lyu, W., Li, J., Zhu, L., Weng, J., Yue, F., et al. (2016). Natural fractures and their influence on shale gas enrichment in Sichuan Basin, China. J. Nat. Gas Sci. Eng. 30, 1–9. doi:10.1016/j.jngse.2015.11.048
Keywords: natural fractures, distribution patterns, lacustrine shale, Fengcheng formation, Mahu sag
Citation: Liu G, Jin Z, Yang S, Zeng L, He W, Du X, Lu G and Liu G (2023) Distribution pattern of natural fractures in lacustrine shales: a case study of the Fengcheng formation in the Mahu Sag of the Junggar Basin, China. Front. Earth Sci. 11:1207033. doi: 10.3389/feart.2023.1207033
Received: 17 April 2023; Accepted: 09 May 2023;
Published: 18 May 2023.
Edited by:
Ruyue Wang, Sinopec Petroleum Exploration and Production Research Institute, ChinaReviewed by:
Jingshou Liu, China University of Geosciences Wuhan, ChinaJianhua He, Chengdu University of Technology, China
Copyright © 2023 Liu, Jin, Yang, Zeng, He, Du, Lu and Liu. This is an open-access article distributed under the terms of the Creative Commons Attribution License (CC BY). The use, distribution or reproduction in other forums is permitted, provided the original author(s) and the copyright owner(s) are credited and that the original publication in this journal is cited, in accordance with accepted academic practice. No use, distribution or reproduction is permitted which does not comply with these terms.
*Correspondence: Zhijun Jin, jinzj1957@pku.edu.cn