- 1PetroChina Research Institute of Petroleum Exploration & Development, Beijing, China
- 2PetroChina Hangzhou Research Institute of Geology, Hangzhou, China
- 3College of Resources and Environment, Yangtze University, Wuhan, China
- 4Laboratory of Reservoir Microstructure Evolution and Digital Characterization, Yangtze University, Wuhan, China
- 5Institute of Geology and Paleontology, Linyi University, Linyi, China
Marine-continental transitional shale, as an important shale type, gains less attention than marine and continental shale, which restricts the exploration and development process of marine-continental transitional shale gas. In this study, the Upper Permian Longtan Formation shale in the southern Sichuan Basin was taken as the research object, and the organic matter development characteristics, hydrocarbon generation ability, mineral composition, physical properties, and gas bearing characteristics of the Longtan Formation shale were systematically analyzed. In addition, the effects of organic matter abundance, maturity, and mineral components on shale gas adsorption capacity have been discussed in detail. The results show that the abundance of organic matter in the marine continental transitional shale of the Longtan Formation in southern Sichuan varies greatly, with the TOC value of the vast majority of shale being greater than 2.0%, with the carbon shale (TOC>12%) accounting for about 5%. The main type of organic matter is Type III, with part of Type II2. The maturity of organic matter is in the stage of high maturity to over maturity, which is conducive to the generation of dry gas. There is a good positive linear correlation between the reflectance of vitrinite (Ro) and the maximum thermal decomposition peak temperature (Tmax) of rock. The higher the abundance of organic matter, the greater the hydrocarbon generation potential of shale, and the carbonaceous shale shows good shale gas generation potential. The shale of the Longtan Formation is rich in clay minerals, with the highest content of the illite/smectite mixed layer. The abundance and maturity of organic matter jointlypromote the enrichment of Longtan shale gas. The enrichment of clay minerals is beneficial to shale gas adsorption, but poses a challenge to production fracturing.
Highlights
• Abundance, type and maturity of organic matters in Longtan shales were revealed.
• Clay enrichment of Longtan shales challenges production fracturing.
• The enrichment of organic matters and clays is conducive to pore structures.
• Shale gas content is controlled by abundance and maturity organic matter, clays and porosity.
1 Introduction
With the rapid growth of clean energy demand such as natural gas and the continuous development of the energy industry, the global natural gas production has been steadily increasing, and the composition of the world energy structure is undergoing dramatic changes (Curtis, 2002; Pollastro et al., 2007; Wang et al., 2009; Selley, 2012; Johnson and Boersma, 2013; Fu, 2014; Liang et al., 2014). China has multiple types of shale gas resources, which are widely distributed and have great resource potential (Fu, 2014; Yuanjiang et al., 2014; Feng et al., 2015; Cao and Zhou, 2015; Wen, 2016). In recent years, following the shale gas revolution in the United States, the marine Wufeng-Longmaxi shales of the Ordovician Silurian System in the Sichuan Basin facies took the lead in achieving breakthrough in industrial exploration and development of shale gas (Fu, 2014; Liang et al., 2014; Ma et al., 2018). In addition, the exploration and development of lacustrine shale gas has also made important progress in Chang 7 member in the Yanchang exploration area (Wang et al., 2014a; Yan et al., 2017).
The Permian marine–continental transitional shale is widely distributed in China. However, compared with the progress made in marine and continental facies, the research on marine–continental transitional shale gas in China is relatively weak (Dong et al., 2014; 2016; Liang et al., 2014; Luo et al., 2018). Whether it has industrial value or not, the industry has different understandings. There are many research results on shale reservoir characteristics and reservoir formation rules of Wufeng-Longmaxi Formation in southern Sichuan Basin (Wang, 2010; Jiu et al., 2013; Tan et al., 2014; Tang et al., 2014; Wang, 2016b). Comparatively, few studies on shale gas reservoir and gas bearing characteristics of Longtan Formation was reported, especially on the characteristics of transitional facies shale reservoir and the difference between shale gas bearing properties and marine shale (Liang et al., 2014; Tan et al., 2014; Wang et al., 2016b; Dong et al., 2016). A study by Luo et al. (2018) shows that the Upper Permian Longtan shale in northwest Guizhou has great potential as a shale gas resource. It is urgent to reveal whether Longtan Formation shale also has shale gas resource potential in the southern Sichuan Basin, as a neighboring area. In this work, the Upper Permian Longtan Formation shale of marine–continental transitional facies in southern Sichuan was selected and geochemical, mineralogical and gas bearing analysis experiments were conducted, in order to analyze the lithostratigraphic and geochemical characteristics, reservoir characteristics and gas bearing characteristics of Longtan Formation in the study area. The relevant research will provide important scientific support and guidance for the theoretical research of marine terrestrial transitional shale gas accumulation and the actual exploration and development in Sichuan Basin.
2 Geological setting
The Sichuan basin, covering an area of about 180,000 square kilometers (Figure 1), is located in southern China and the northwest edge of the Yangtze Plate in South China (Cao et al., 2014; Wang et al., 2016b; Dong et al., 2016). Multiple sets of source rocks are developed in the basin, which is an important natural gas production area in China (Chen et al., 2015; Wang et al., 2015). The study area in the south of Sichuan Basin is mainly located in the tail of the southwest composite broom low anticline group of Huangshan fault fold belt. Affected by the Daloushan fault fold belt, it belongs to the “Sichuan Guizhou Right Depression” of the southern Sichuan low steep fold belt (Chen et al., 2015; Wang et al., 2015). The south Sichuan low steep fold belt is a brush like echelon low anticline group, which extends southwest of Huaying Mountain fault fold belt and develops three groups of structures in NE, EW and SN directions (Sun et al., 2013; Cao et al., 2014; Chen et al., 2015; Wang et al., 2015). The structures of each group interact with each other and are in reverse or oblique connection. It is a depression area in Caledonian, the main part of Liuzhou ancient uplift in Indosinian, and the uplift area since Mesozoic (Sun et al., 2013; Cao et al., 2014; Chen et al., 2015; Wang et al., 2015).
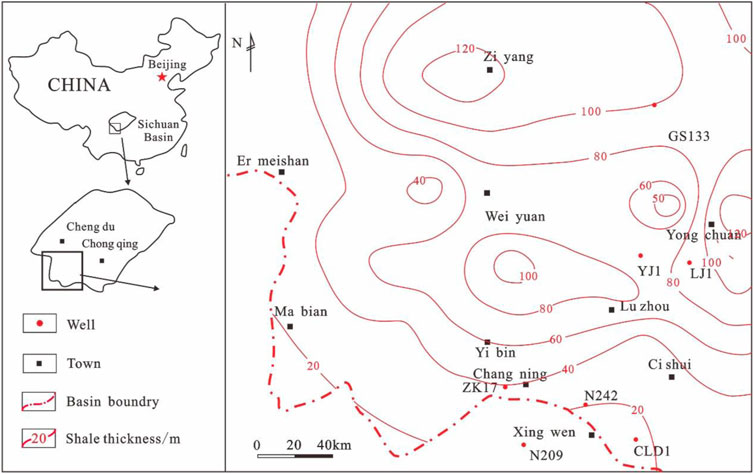
FIGURE 1. Sampling location in Sichuan Basin from South China and the thickness distribution of organic rich Longtan shales (modified after Zhang et al., 2017a).
Due to the Caledonian movement, a set of marine continental transitional facies coal bearing rock series dominated by carbonaceous shale and dark shale was widely deposited in the Longtan period of the late Permian in southern Sichuan, with a thickness ranging between 20 and 120 m (Figure 1) (Sun et al., 2013; Wang et al., 2015; Zhang et al., 2017a; Zhang et al., 2017b). It is in conformity with the overlying Dalong Formation of the Upper Permian and unconformable contact with the underlying strata of the Maokou Formation of the Lower Permian (Figure 2) (Sun et al., 2013; Wang et al., 2015; Zhang et al., 2017a; b). It belongs to coastal swamp facies deposits, rich in organic matter (OM), and is conducive to the generation and enrichment of shale gas.
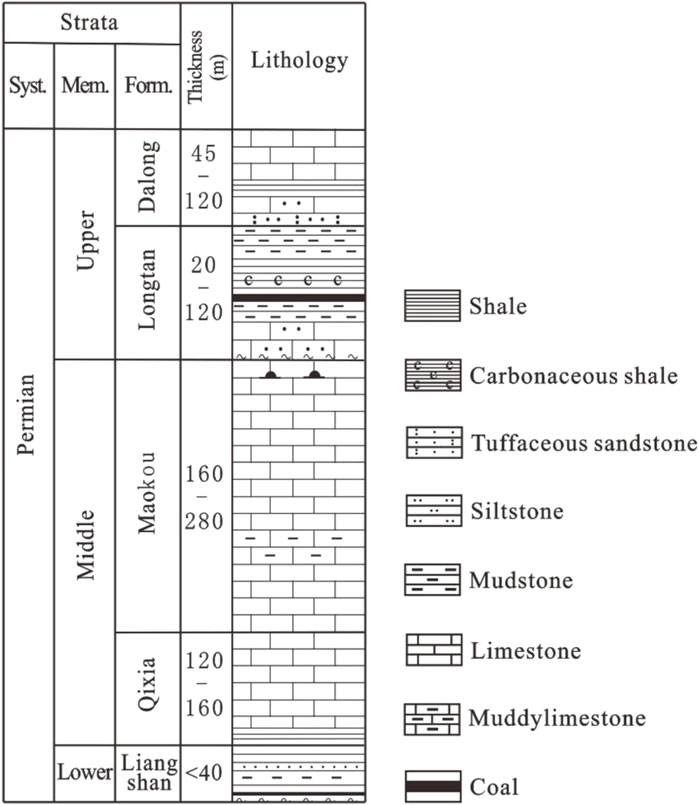
FIGURE 2. Comprehensive histogram of permian strata in sichuan basin (modified after Zhang et al., 2017b).
3 Materials and methods
A total of 366 drilling core samples with the burial depth of 1,650–3,910 m were collected for experiments form seven wells in the southern Sichuan, South China. TOC content analysis were carried out by a LECO–230 carbon-sulfur analyzer (Cornerstone)™, with an accuracy within 0.5%, by adopting the Chinese national standard GB/T 19145-2022. Rock-eval experiments were completed using a OGE-Ⅵ type high temperature rock pyrolysis analyzer, following the Chinese national standard GB/T 18602-2012. A Picarro CM-CRDS carbon isotope analyzer were used for the determination of stable carbon isotopes of Oms in shales, on the basis of Chinese oil and natural gas industry standard SY/T 5238-2019. X-ray diffraction (XRD) experiments were performed using a Japan Shimadzu X-ray diffractometer XRD-7000 S/L, on the basis of Chinese oil and natural gas industry standard SY/T 5163–2018. Methane isothermal adsorption experiment is conducted with a high pressure gas adsorption instrument BSD-PH690, based on the Chinese national standard GB/T 35210.1-2017.
4 Results and discussion
4.1 Bulk geochemical characteristics
Abundant OM is the material basis for shale gas accumulation, which has a significant impact on the pore characteristics of shale (Bowker, 2007; Ghanizadeh et al., 2015a; Ghanizadeh et al., 2015b). Therefore, the study of shale organic matter abundance plays a crucial role in shale reservoir evaluation. Organic carbon content (TOC) is the most commonly used evaluation index to characterize the abundance of organic matter (Bowker, 2007; Ghanizadeh et al., 2015a; Ghanizadeh et al., 2015b). The mass fraction of organic carbon has an important influence on the hydrocarbon generation capacity and gas content of shale (Bowker, 2007; Ghanizadeh et al., 2015a; b; Li et al., 2016). High organic carbon content is an important guarantee for high hydrocarbon generation potential per unit volume of shale. At the same time, shale organic matter has a certain adsorption on gas, which is conducive to the occurrence and enrichment of shale gas (Bowker, 2007). The organic carbon (TOC) content of shale samples from Longtan Formation in southern Sichuan ranges between 0.25% and 39.3%, with an average of 2.61% (Figure 3). According to the statistics of 374 fresh core samples, 75% of Longtan Formation shale samples are rich in OMs, with the TOC value of >2%. In all samples, the carbonaceous shale accounts for about 5%, with the TOC value ranging between 12.5% and 39.3% (average 27.8%). 62% shale sample developed with the TOC value of 2%–4%. Shale samples of Longtan Formation in southern Sichuan are commonly rich in OMs, and shale samples with TOC>2% are distributed at different depths of Longtan Formation. After TOC analysis of 366 samples, we selected samples for further OM type and OM thermal maturity, XRD mineral analysis based on the difference in TOC content to avoid selecting too many samples with similar TOC content from the same well.
4.2 OM type
The organic matter type of shale is the quality index in organic geochemical evaluation. Different types of primitive organic matter have different original provenance, depositional preservation environment, chemical composition and structure, so they have different hydrocarbon generation potential (Tissot and Welte, 1984; Hakimi et al., 2016). Therefore, the determination of organic matter type is the key link of shale gas evaluation. There are many evaluation methods for the type of organic matter (Tissot and Welte, 1984; Golyshev et al., 1991). The combination of multiple methods is conducive to accurate determination. As shown in Figure 4, the organic matter types of the shale in the Longtan Formation of the Upper Permian System are poorly displayed. The hydrogen index of most shale samples is very low, and the peak temperature of thermal decomposition is relatively high, which is basically above 500°C, indicating that most shale samples have experienced thermal evolution of over-mature or over-mature stage (Tissot and Welte, 1984; Golyshev et al., 1991). The carbon isotope content of kerogen during thermal degradation does not change much. Therefore, the carbon isotope value of shale can also be used to effectively determine its organic matter type. Figure 4 shows the organic matter type of shale in Longtan Formation, Upper Permian, identified by stable isotope data of kerogen. The figure shows kerogen of shale sample from Longtan Formation (P3l) in southern Sichuan δ 13C ranges between −24.3 and −21.9‰, the organic matter type is mainly type III, and some are type II2. In addition, according to the identification method of kerogen morphology and carbon isotope, and a ternary diagram of the maceral composition derived from Wang et al. (2015) shows vitrinite is the main type of macerals in Longtan shales, which demonstrates that the organic matter type of shale in Longtan Formation of Upper Permian is mainly of type III, and part of type II2.
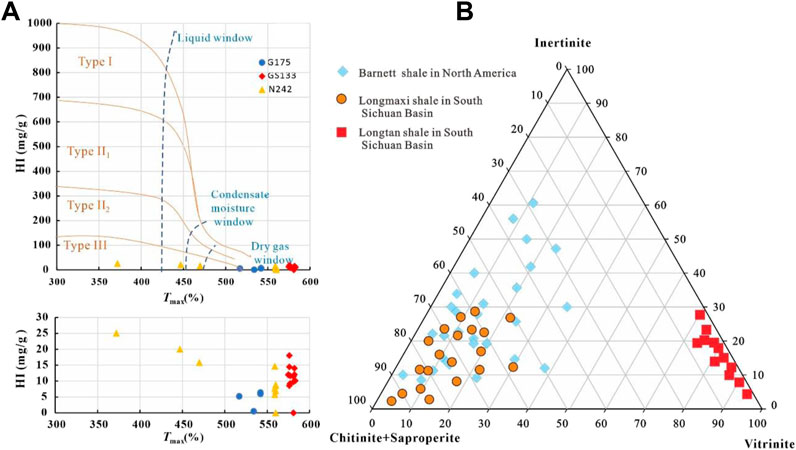
FIGURE 4. Determination of shale organic matter types of Longtan Formation in southern Sichuan based on pyrolysis parameters (A) and macerals (B) (modified from Tissot and Welte, 1984; Wang et al., 2015).
4.3 OM thermal maturity
The abundance and type of organic matter is the material basis of hydrocarbon generation in source rocks, but only when the organic matter reaches a certain maturity can it generate a large amount of hydrocarbon (Zhao et al., 2017). Therefore, the maturity of organic matter is an important content of source rock research. The maturity of organic matter indicates the degree of thermal evolution of organic matter in sedimentary rocks into oil and gas (Xie et al., 2014; Adegoke et al., 2015). During the thermal evolution of hydrocarbon source rocks, some physical or chemical properties of organic matter will change irreversibly, so the change characteristics of physical or chemical composition of these organic matter can be used to determine the maturity of organic matter (Volk et al., 2005; Xie et al., 2014; Adegoke et al., 2015). At present, vitrinite reflectance (Ro) and rock pyrolysis peak temperature (Tmax) are widely used to evaluate the maturity parameters of organic matter. The maturity of shale samples from Longtan Formation in southern Sichuan is generally between 1.74% and 3.57% (average 2.54%), which is in the stage of high maturity and over-maturity, which is conducive to dry gas generation. The maturity of the Longtan shales in southern Sichuan can be quantitatively characterized by both Ro and Tmax, which have a good linear positive correlation (Figure 5).
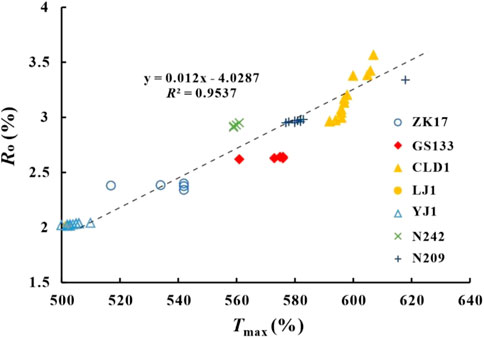
FIGURE 5. Correlation between maximum thermal peak temperature and vitrinite reflectance of the Longtan shales in southern Sichuan basin.
4.4 Hydrocarbon generating potential
The organic matter abundance and hydrocarbon generation potential of source rocks are direct and important indicators to measure the quality of source rocks (Zhang et al., 2016). Whether a sedimentary rock in a sedimentary block can become a source rock depends on its organic matter abundance, which determines the amount of hydrocarbon generation and expulsion of the rock (Xie et al., 2014). When the abundance is too small, the hydrocarbon generation is insufficient and cannot be discharged. Only when the abundance reaches a certain value can the generated hydrocarbon be sufficient to saturate the rock and discharge a part of it (Liang et al., 2014). Therefore, the lower limit of organic matter abundance of source rock refers to the organic matter abundance value corresponding to the source rock when the hydrocarbon generated by the source rock is just saturated but not discharged. This value is the standard for dividing the source rock and non-source rock.
As one of the important parameters of rock pyrolysis, hydrocarbon generation potential (S1+S2) can also be used to evaluate the abundance of organic matter, which is composed of two parts, one is the generated hydrocarbon, namely, soluble hydrocarbon (S1), and the other is pyrolysis hydrocarbon (S2). The analysis and test results show that the hydrocarbon generation potential S1+S2 of shale samples from Longtan Formation in southern Sichuan is generally between 0.05 and 1.25 mg/g (average of 0.28 mg/g), and the difference between different well locations is relatively small. The hydrocarbon generation potential of coal is higher than that of shale, and its hydrocarbon generation potential is generally between 5.5 and 7.0 mg/g. There is a good linear positive correlation between TOC and hydrocarbon generation potential of Longtan shale in southern Sichuan, showing that the higher the abundance of organic matter, the greater the hydrocarbon generation potential of shale. Coal-bearing shales are rich in components such as algae, sporophytes, keratinizes, vitrinite, and matrix vitrinite, with different hydrocarbon generation abilities. The outlier of the shale from the LJ1 in Figure 6 could be attribute to the relative enrichment of sporophytes.
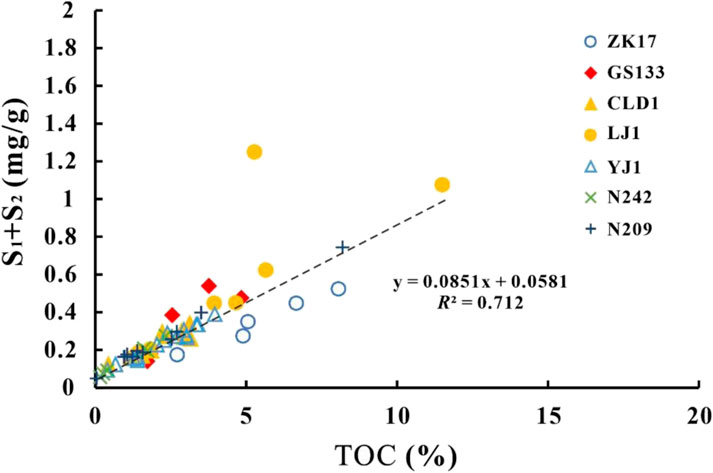
FIGURE 6. Correlation between hydrocarbon generation potential S1+S2 and TOC of the Longtan shales in southern Sichuan basin.
4.5 Mineralogy
The whole rock XRD analysis shows that the mineral composition of Longtan Formation shale samples in southern Sichuan is relatively complex (Kamei et al., 2005). Quartz, feldspar and other clastic minerals, calcite, dolomite, siderite and other carbonate minerals, as well as clay minerals, including illite, kaolinite, chlorite, illite/smectite mixed layer, are detected in shale, of which quartz, clay and carbonate are the main mineral components. In the shale samples of Longtan Formation in southern Sichuan, the main mineral composition is clay and quartz, followed by calcite and feldspar. The clay mineral content is 17.0-72.0 wt% (mean of 54.2 wt%), quartz content is 17.3%-47.7% (mean of 27.2 wt%), calcite content is 0.4-41.37 wt% (mean of 12.5 wt%), feldspar content is 0%–14.6% (mean of 2.9 wt%), and other mineral content generally accounts for 5%–10% (Figure 7). The clay minerals in the shale samples of Longtan Formation in southern Sichuan are mainly composed of illite/smectite mixed layer and kaolinite, followed by chlorite and illite. The content of illite/smectite mixed layer is 5.6-37.7 wt%, with an average of 19.3 wt%, the content of kaolinite is 3.3-25.0 wt%, with an average of 14.0 wt%, the content of chlorite is 1.7-27.5 wt%, with an average of 13.0 wt%, and the content of illite is 0.8-14.2 wt%, with an average of 8.02 wt%.
4.6 Pore development characteristics
Rock porosity is the main space of shale gas occurrence and the key parameter to determine the content of free gas (Yan et al., 2017). With the deepening of shale gas exploration and research, it is found that the vast majority of developable shale gas is free gas, which is similar to conventional reservoirs. The free gas volume directly controls the initial production and final recoverable gas volume of shale gas development. Therefore, systematic analysis of shale porosity is crucial to shale gas content and recoverable reserves. The porosity of Longtan Formation coal-bearing shale in southern Sichuan is between 2.55% and 9.96% (average of 5.1%), with the dominant frequency (accounting for 70.7%) range of 3%–5%. The permeability of Longtan Formation coal-bearing shale in southern Sichuan is between 0.06 and 11.5mD, with an average of 0.57mD, showing a good exponential correlation with porosity (Figure 8).
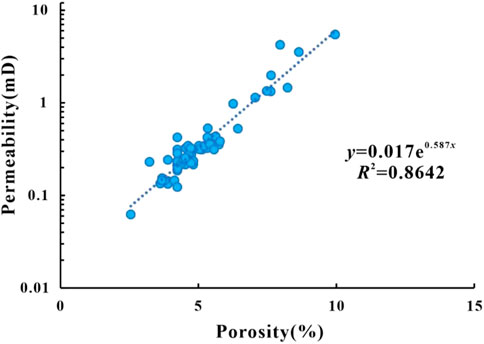
FIGURE 8. Correlation between porosity and permeability of Longtan shale samples in southern Sichuan basin.
4.7 Shale gas content
As an important indicator to measure the gas adsorption capacity of shale, the isothermal adsorption characteristics of shale are mainly characterized by isothermal adsorption curves, which show the relationship between the maximum gas adsorption capacity of shale and pressure (Loucks et al., 2012). Because shale gas and coalbed methane have certain similarities in adsorption mechanism, the equipment for testing coalbed methane is often used to analyze the adsorption characteristics of shale gas (Jiao et al., 2012; Li X. Q. et al., 2016; Chen et al., 2016). Ten samples were selected from the study area to conduct the test under the isothermal condition of 40°C. The isothermal adsorption curve is shown in Figure 9. With the increase of pressure, the gas adsorption capacity gradually increases. The adsorption capacity increases rapidly in the pressure range of 0–5 MPa, and increases slowly in the pressure range of 5 MPa–10 MPa. With the pressure of over 10 MPa, the absolute curve is basically in a horizontal state. In this stage, the sample adsorption capacity has reached a saturated state, and the adsorption capacity is large under the action of high pressure, showing an excess adsorption state.
5 Discussion
5.1 Effect of organic matter abundance on gas content
Organic carbon content is the material basis of shale gas generation and the main factor affecting methane adsorption capacity. At the same time, the microporous nature of organic matter indicates that it is also one of the carriers of adsorbed gas and can affect the content of adsorbed gas (Jarvie et al., 2007; Clarkson et al., 2013; Wang et al., 2016a). Under the same temperature and pressure, shale rich in organic matter often has more micropore space and specific surface area than shale poor in organic matter, so it can absorb more natural gas (Zou et al., 2010). Therefore, high organic carbon content often indicates higher hydrocarbon generation potential and gas adsorption capacity. The shale samples of Longtan Formation in southern Sichuan are mainly black shale, black carbonaceous mudstone, black siliceous shale and calcareous shale. Through experimental analysis, it is found that the amount of adsorbed gas generally increases with the increase of organic carbon content (Figure 10). The organic carbon content shows good correlation with the amount of adsorbed gas, which is consistent with the understanding of shale research in North America. It indicates that high organic carbon content corresponds to stronger adsorption capacity. Additionally, the high organic carbon content is generated in anoxic environment. Because of the weak bacterial function, it is conducive to the preservation of organic matter and provides a prerequisite for the uninterrupted generation of adsorbed gas. Among the various factors affecting methane adsorption capacity mentioned above, the clay mineral content has the strongest impact, followed by the maturity and abundance of organic matter.
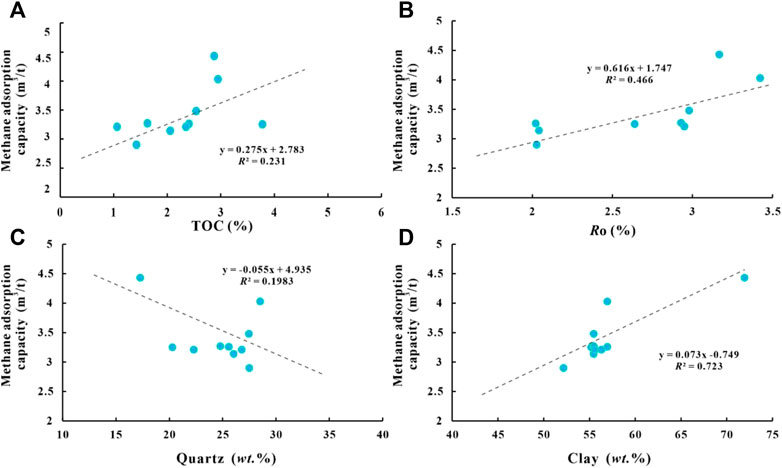
FIGURE 10. Influence of TOC (A), maturity (B), quartz (C) and clay content (D) on methane adsorption in the Longtan shales from southern Sichuan basin.
5.2 Effect of organic matter maturity on gas content
The adsorption capacity of gas gradually increases with the evolution of maturity. Comparing the vitrinite reflectance values of Longtan shale in south Sichuan basin with the shales in the United States, it is found that there is a certain correlation between the adsorption capacity of shale and vitrinite reflectance (Jarvie et al., 2007; Clarkson et al., 2013). Samples with high vitrinite reflectance values tend to have higher methane adsorption capacity, especially the higher the degree of coalification, the better the correlation, showing that there is a certain correlation between adsorption capacity and maturity (Figure 10). However, compared with the samples with low maturity, some highly mature or over-mature samples have relatively low gas adsorption capacity. Researchers also found that when the maturity is higher than a certain value, the adsorption capacity of shale will not increase but will decrease. This phenomenon is probably attributed to that when the organic matter reaches high maturity or over-maturity, the organic matter has been largely consumed, reducing the adsorption carrier and adsorption space, which is not conducive to gas adsorption (Zou et al., 2010).
5.3 Effect of clay mineral content on gas content
Ross and Bustin (2008) believed that clay minerals in shale have higher micropore volume and larger specific surface area, so a certain amount of clay minerals in shale will increase the adsorption capacity of shale. Therefore, within a certain range, the adsorption capacity of samples with high clay content is often stronger than that of samples with low clay content.
Due to different crystal lattice and chemical structure, clay minerals have different micropore volume and specific surface area, and their adsorption capacity is also different. Ji et al. (2012) point out that the type of clay mineral determines the adsorption capacity of gas. Generally, the adsorption capacity of illite and montmorillonite is significantly stronger than other minerals such as kaolinite.
The illite contained in the shale samples of Longtan Formation in southern Sichuan is mainly twisted and the edge is curly under high power scanning electron microscope. The pore size between particles is mostly 50 nm, and the plates are arranged and staggered into triangles and rhombus. The increase of illite content within a certain range can increase the adsorption capacity of shale, especially in the case of low organic carbon content. The researchers found that the storage space of adsorbed gas can be compensated by methane adsorption on the surface of illite. The shale gas research in the United States shows that the illite content of other clay minerals has a greater impact on the adsorption capacity of shale, and often the high illite content corresponds to the high adsorption capacity of gas (Jarvie et al., 2007; Clarkson et al., 2013; Adegoke et al., 2015). Under the scanning electron microscope, montmorillonite is mainly micro-layered, and its edge is wrapped by silky and wavy protrusions, with the size of 1 μm approximately (Li X. Q. et al., 2016; Zhang et al., 2017b). The wide slit connected pores are formed between the layers, and there are many layers of cavernous pores embedded inside, which have strong adsorption capacity (weaker than illite). In the process of diagenesis, the continuous transformation of montmorillonite to illite could be accompanied by the formation of microfracture pores), which is beneficial to the gas storage and adsorption, and indirectly increases the gas adsorption capacity.
6 Conclusion
In this work, the geochemical and gas bearing characteristics of marine-continental transitional shales from the Upper Permian Longtan Formation, collected from the south Sichuan Basin of China, were discussed, and the following conclusions have been achieved. Shale samples from the Longtan Formation in southern Sichuan are generally rich in organic matters, with the TOC value of >2.0% for most samples. TOC value of 62% of samples ranges between 2% and 4%. Carbonaceous shale (TOC>12%) accounts about 5% of all samples. Hydrocarbon generation potential shows a good linear positive correlation with TOC, with the value of S1+S2 ranging of 0.05-1.25 mg/g δ13C value of the Longtan shales ranges between −24.3 and −21.9‰, and vitrinite is the most abundant organic macerals, showing that the main type of organic matter is Type III, with part of Type II2. Longtan shales are rich in clay minerals, with the content of 17.0%-72.0% (mean of 54.2%), and illite content dominates in clays. High clay minerals reduce the compressibility of shale. Porosity and permeability are 2.55%–9.96% and 0.06mD-11.5mD, respectively. The increase of the abundance and maturity of organic matter and the content of clay minerals contributes to adsorption and enrichment of shale gas.
Data availability statement
The original contributions presented in the study are included in the article/supplementary material, further inquiries can be directed to the corresponding author.
Author contributions
YC, Data summary and processing; XP, Data processing and analysis; JZ, Article writing and organization; NW, Data processing and analysis; DH, Article review and modification; JuW, Data processing and analysis; XX, Experiment and data organization; JiW, Experiment and data organization. All authors contributed to the article and approved the submitted version.
Acknowledgments
The authors would like to thank the financial support from the National Natural Science Foundation of China (Grant No. 42202141), transverse project from Petro China Southwest Oil and Gas field Company: Study on Geological Conditions of shale gas in Longtan Formation of Permian in Sichuan Basin and Optimization of Favorable Exploration Targets (2022-N/G-47631), and Technological Development Project of Petro China: Main controlling factors of high production and beneficial development technology of deep shale gas in western Chongqing (2022KT1205). All the editors and reviewers are gratefully acknowledged.
Conflict of interest
The authors YC, XP, and NW were employed by PetroChina Research Institute of Petroleum Exploration & Development, China. The author YC was employed by PetroChina Hangzhou Research Institute of Geology, China.
The remaining authors declare that the research was conducted in the absence of any commercial or financial relationships that could be construed as a potential conflict of interest.
Publisher’s note
All claims expressed in this article are solely those of the authors and do not necessarily represent those of their affiliated organizations, or those of the publisher, the editors and the reviewers. Any product that may be evaluated in this article, or claim that may be made by its manufacturer, is not guaranteed or endorsed by the publisher.
Abbreviations
OM, organic matter; TOC, total organic carbon; Ro, vitrinite reflectance; Fm, Formation; XRD, X–ray diffraction; S1, volatile hydrocarbon content; S2, remaining hydrocarbon generative potential; Tmax, temperature of maximum pyrolysis yield.
References
Adegoke, A. K., Abdullah, W. H., and Hakimi, M. H. (2015). Geochemical and petrographic characterisation of organic matter from the Upper Cretaceous Fika shale succession in the Chad (Borne) Basin, northeastern Nigeria: Origin and hydrocarbon generation potential. Mar. Pet. Geol. 61, 95–110. doi:10.1016/j.marpetgeo.2014.12.008
Bowker, K. A. (2007). Barnett shale gas production, fort worth basin: Issues and discussion. AAPG Bull. 91 (4), 523–533. doi:10.1306/06190606018
Cao, T. T., Song, Z. G., Wang, S. B., Cao, X. X., Li, Y., and Xia, J. (2014). Characterizing the pore structure in the silurian and permian shales of the Sichuan Basin, China. Mar. Pet. Geol. 61, 140–150. doi:10.1016/j.marpetgeo.2014.12.007
Chen, Q., Zhang, J. C., Tang, X., Li, W. J., and Li, Z. M. (2016). Relationship between pore type and pore size of marine shale: An example from the Sinian–Cambrian formation, upper Yangtze region, South China. Int. J. Coal Geol. 158, 13–28. doi:10.1016/j.coal.2016.03.001
Clarkson, C. R., Solano, N., Bustin, R. M., Bustin, A. M., Chalmers, G. R., He, L., et al. (2013). Pore structure characterization of North American shale gas reservoirs using USANS/SANS, gas adsorption, and mercury intrusion. Fuel 103, 606–616. doi:10.1016/j.fuel.2012.06.119
Curtis, J. B. (2002). Fractured shale–gas systems. AAPG Bull. 86, 1921–1938. doi:10.1306/61EEDDBE-173E-11D7-8645000102C1865D
Dong, D. Z., Gao, S. K., Huang, J. L., Guan, Q. Z., Wang, S. F., and Wang, Y. M. (2014). A discussion on the shale gas exploration and development prospect in the Sichuan Basin. Nat. Gas. Ind. 34 (12), 1–15. doi:10.1016/j.ngib.2015.02.002
Dong, D. Z., Wang, Y. M., Li, X. J., Zou, C. N., Guan, Q. Z., Zhang, C. C., et al. (2016). Breakthrough and prospect of shale gas exploration and development in China. Nat. Gas. Ind. 36 (1), 12–26. doi:10.1016/j.ngib.2016.02.002
Fu, C. Y. (2014). China's shale gas and shale oil resources: Opportunities and challenges. Energy explor. exploi. 32 (5), 759–769. doi:10.1260/0144-5987.32.5.759
Ghanizadeh, A., Clarkson, C. R., Aquino, S., Ardakani, O. H., and Sanei, H. (2015a). Petrophysical and geomechanical characteristics of canadian tight oil and liquid-rich gas reservoirs: i pore network and permeability characterization. Fuel 153, 664–681. doi:10.1016/j.fuel.2015.03.020
Ghanizadeh, A., Clarkson, C. R., Aquino, S., Ardakani, O. H., and Sanei, H. (2015b). Petrophysical and geomechanical characteristics of canadian tight oil and liquid-rich gas reservoirs: Ii geomechanical property estimation. Fuel 153, 682–691. doi:10.1016/j.fuel.2015.02.113
Golyshev, S. I., Verkhovskaya, N. A., Burkova, V. N., and Matis, E. Y. (1991). Stable carbon isotopes in source-bed organic matter of West and East Siberia. Org. Geochem. 17 (3), 277–291. doi:10.1016/0146-6380(91)90091-w
Jarvie, D. M., Hill, R. J., Ruble, T. E., and Pollastro, R. M. (2007). Unconventional shale–gas systems: The Mississippian Barnett Shale of north–central Texas as one model for thermogenic shale–gas assessment. AAPG Bull. 91 (4), 475–499. doi:10.1306/12190606068
Ji, L. M., Zhang, T. W., Milliken, K. L., Qu, J. L., and Zhong, X. L. (2012). Experimental in-vestigation of main controls to methane adsorption in clay-rich rocks. Appl. Geochem. 27, 2533–2545. doi:10.1016/j.apgeochem.2012.08.027
Jiao, S. J., Han, H., Weng, Q. P., Yang, F., Jiang, D. Q., and Cui, L. S. (2012). Scanning electron microscope analysis of porosity in shale. J. Chin. Electron Microsc. Soc. 31 (5), 432–436. doi:10.1007/s11783-011-0280-z
Jiu, K., Ding, W. L., Huang, W. H., Zhang, Y. Q., Zhao, S., and Hua, L. J. (2013). Fractures of lacustrine shale reservoirs, the zhanhua depression in the Bohai bay basin, eastern China. Mar. Pet. Geol. 48, 113–123. doi:10.1016/j.marpetgeo.2013.08.009
Johnson, C., and Boersma, T. (2007). Energy (in)security in Poland the case of shale gas. Energy Policy 53, 389–399. doi:10.1016/j.enpol.2012.10.068
Kamei, G., Mitsui, M. S., Futakuchi, K., Hashimoto, S., and Sakuramoto, Y. (2005). Kinetics of long-term illitization of montmorillonite—A natural analogue of thermal alteration of bentonite in the radioactive waste disposal system. J. Phy. Chem. Solids 66 (2), 612–614. doi:10.1016/j.jpcs.2004.06.067
Li, A., Ding, W., He, J., Dai, P., Yin, S., and Xie, F. (2016a). Investigation of pore structure and fractal characteristics of organic-rich shale reservoirs: A case study of lower cambrian qiongzhusi Formation in malong block of eastern yunnan province, south China. Mar. Pet. Geol. 70, 46–57. doi:10.1016/j.marpetgeo.2015.11.004
Li, X. Q., Wang, Y., Zhang, J. Z., Guo, M., Zhao, P., Xu, H. W., et al. (2016b). Pore characteristics of shale gas reservoirs from the Lower Paleozoic in the southern Sichuan Basin, China. J. Nat. Gas. Geosci. 1 (3), 195–202. doi:10.1016/j.jnggs.2016.07.002
Liang, C., Jiang, Z., Zhang, C., Guo, L., Yang, Y., and Li, J. (2014). The shale characteristics and shale gas exploration prospects of the Lower Silurian Longmaxi shale, Sichuan Basin, South China. J. Nat. Gas. Sci. Eng. 21, 636–648. doi:10.1016/j.jngse.2014.09.034
Loucks, R. G., Reed, R. M., Ruppel, S. C., and Hammes, U. (2012). Spectrum of pore types and networks in mudrocks and a descriptive classification for matrix-related mudrock pores. AAPG Bull. 96, 1071–1098. doi:10.1306/08171111061
Luo, W., Hou, M. C., Liu, X. C., Huang, S. G., Chao, H., Zhang, R., et al. (2018). Geological and geochemical characteristics of marine-continental transitional shale from the Upper Permian Longtan formation, Northwestern Guizhou, China. Mar. Pet. Geol. 89, 58–67. doi:10.1016/j.marpetgeo.2017.06.029
Ma, Y. S., Cai, X. Y., and Zhao, P. R. (2018). China's shale gas exploration and development: Understanding and practice. Pet. Explor. Dev. 45 (4), 589–603. doi:10.1016/s1876-3804(18)30065-x
Pollastro, R. M. (2007). Total petroleum system assessment of undiscovered resources in the giant Barnett Shale continuous (unconventional) gas accumulation, Fort Worth Basin, Texas. AAPG Bull. 91, 551–578. doi:10.1306/06200606007
Ross, D., and Bustin, R. M. (2008). Characterizing the shale gas resource potential of devonian–Mississippian strata in the Western Canada sedimentary basin: Application of an integrated formation evaluation. AAPG Bull. 92 (1), 87–125. doi:10.1306/09040707048
Selley, R. C. (2012). UK shale gas: The story so far. Mar. Pet. Geol. 31 (1), 100–109. doi:10.1016/j.marpetgeo.2011.08.017
Sun, M. D., Yu, B. S., Hu, Q. H., Chen, S., Xia, W., and Ye, R. C. (2016). Nanoscale pore characteristics of the lower cambrian niutitang Formation shale: A case study from well yuk #1 in the southeast of chongqing, China. Int. J. Coal Geol. 154-155, 16–29. doi:10.1016/j.coal.2015.11.015
Tan, J., Weniger, P., Krooss, B., Merkel, A., Horsfield, B., Zhang, J. C., et al. (2014). Shale gas potential of the major marine shale formations in the upper Yangtze platform, south China. Part II: Methane sorption capacity. Fuel 129, 204–218. doi:10.1016/j.fuel.2014.03.064
Tang, X., Zhang, J., Wang, X., Yu, B., Ding, W., Xiong, J., et al. (2014). Shale characteristics in the southeastern ordos basin, China: Implications for hydrocarbon accumulation conditions and the potential of continental shales. Int. J. Coal Geol. 128-129, 32–46. doi:10.1016/j.coal.2014.03.005
Tissot, B. P., Deroo, G., and Hood, A. (1978). Geochemical study of the uinta basin: Formation of petroleum from the green river formation. Geochim. Cosmochim. Acta 42, 1469–1485. doi:10.1016/0016-7037(78)90018-2
Volk, H., George, S. C., Middleton, H., and Schofield, S. (2005). Geochemical comparison of fluid inclusion and present-day oil accumulations in the Papuan Foreland – evidence for previously unrecognised petroleum source rocks. Org. Geochem. 36, 29–51. doi:10.1016/j.orggeochem.2004.07.018
Wang, G. C., Ju, Y. W., Yan, Z. F., and Li, Q. G. (2015). Pore structure characteristics of coal–bearing shale using fluid invasion methods: A case study in the Huainan–huaibei coalfield in China. Mar. Petroleum Geol. 62, 1–13. doi:10.1016/j.marpetgeo.2015.01.001
Wang, G., Wang, T. G., Simoneit, B. R. T., Zhang, L., and Zhang, X. (2010). Sulfur rich petroleum derived from lacustrine carbonate source rocks in Bohai Bay Basin, East China. Org. Geochem. 41, 340–354. doi:10.1016/j.orggeochem.2009.12.010
Wang, H. Y., Li, J. M., Zhao, Q., and Lin, Y. J. (2009). Resources and development of new energy in China. Acta Pet. Sin. 30 (3), 469–474. doi:10.3321/j.issn:0253-2697.2009.03.029
Wang, Y., Zhu, Y., Chen, S., and Wu, L. (2014a). Characteristics of the nanoscale pore structure in northwestern hunan shale gas reservoirs using field emission scanning electron microscopy, high-pressure mercury intrusion, and gas adsorption. Energy fuels. 28 (2), 945–955. doi:10.1021/ef402159e
Wang, Y., Zhu, Y. M., Liu, S. M., and Zhang, R. (2016). Pore characterization and its impact on methane adsorption capacity for organic-rich marine shales. Fuel 181, 227–237. doi:10.1016/j.fuel.2016.04.082
Wen, L. (2016). A review on shale reservoirs as an unconventional play—the History,Technology revolution,importance to oil and gas industry, and the development future. Acta geolo. Sin. 90 (5), 1887–1902. doi:10.1111/1755-6724.12823
Xie, Q. F., Zhou, L. F., and Liu, Y. (2014). Geochemistry and geological significant of Permian source rocks in Yili Basin. Acta pet. sin. 35 (1), 50–57. doi:10.7623/syxb201401005
Yan, W., Fan, T. L., Wang, H. Y., Zhu, C., Gao, Z. Q., Meng, X. J., et al. (2017). Micropaleontology and palaeoclimate during the early cretaceous in the lishu depression, Songliao basin, northeast China. Geosci. Front. 01, 93–106. doi:10.1016/j.gsf.2015.12.005
Zhang, H., Zhu, Y. M., Wang, Y., Kang, W., and Chen, S. B. (2016). Comparison of organic matter occurrence and organic nanopore structure within marine and terrestrial shale. J. Nat. Gas. Sci. Eng. 32, 356–363. doi:10.1016/j.jngse.2016.04.040
Zhang, J. Z., Li, X. Q., Wei, Q., Gao, W. J., Liang, W. L., Wang, Z., et al. (2017a). Quantitative characterization of pore–fracture system of organic–rich marine–continental shale reservoirs: A case study of the upper permian Longtan Formation, southern Sichuan Basin, China. Fuel 200, 272–281. doi:10.1016/j.fuel.2017.03.080
Zhang, J. Z., Li, X. Q., Wei, Q., Sun, K. X., Zhang, G. W., and Wang, F. Y. (2017b). Characterization of full-sized pore structure and fractal characteristics of marine−continental transitional Longtan formation shale of Sichuan Basin, South China. Energy fuels. 31 (10), 10490–10504. doi:10.1021/acs.energyfuels.7b01456
Zhao, Z. F., Pang, X. Q., Li, Q. W., Hu, T., Wang, K., Li, W., et al. (2017). Depositional environment and geochemical characteristics of the lower carboniferous source rocks in the marsel area, chu-says basin, southern Kazakhstan. Mar. Pet. Geol. 81, 134–148. doi:10.1016/j.marpetgeo.2016.12.021
Keywords: Longtan formation, Sichuan Basin, shale gas, geochemical characteristics, reservoir
Citation: Chen Y, Pei X, Zhang J, Wang N, Han D, Wang J, Xiao X and Wang J (2023) Geochemical and gas bearing characteristics of the marine-continental transitional Longtan shales in southern Sichuan, China. Front. Earth Sci. 11:1202005. doi: 10.3389/feart.2023.1202005
Received: 07 April 2023; Accepted: 21 June 2023;
Published: 07 July 2023.
Edited by:
Mingtao Li, Linyi University, ChinaReviewed by:
Hongjian Zhu, Yanshan University, ChinaDaye Chen, China University of Geosciences, China
Copyright © 2023 Chen, Pei, Zhang, Wang, Han, Wang, Xiao and Wang. This is an open-access article distributed under the terms of the Creative Commons Attribution License (CC BY). The use, distribution or reproduction in other forums is permitted, provided the original author(s) and the copyright owner(s) are credited and that the original publication in this journal is cited, in accordance with accepted academic practice. No use, distribution or reproduction is permitted which does not comply with these terms.
*Correspondence: Yana Chen, chenyn_hz@petrochina.com.cn; Jizhen Zhang, zhangjz1991@126.com