- 1College of Transportation Science and Engineering, Jilin Jianzhu University, Changchun, China
- 2College of Civil Engineering, Jilin Jianzhu University, Changchun, China
- 3School of Civil Engineering, Changchun Institute of Technology, Changchun, China
Among the methods of landslide treatment, anti-slide piles are an effective measure. The soil arching effect cannot be ignored in the design of anti-slide piles, which has a significant impact on the supporting. Based on the field investigation, this paper selects the Xinyan landslide in Yanbian area of Jilin Province and uses Flac-3D to build a numerical model to simulate its soil arching effect with different pile arrangements. The simulation results show that the pile arrangement has a significant influence on the soil arching effect. When arranging piles in parallel, the soil arches between the front and rear rows of piles are distributed parallel to each other. When arranging piles in quincunx-shape, the soil arches between the rear rows of piles are the same as when laying piles in parallel. The soil between the piles flows around once when the piles are laid out in parallel, while it flows around twice when the piles are laid out in a quincunx-shape. The latter has better support than the former.
Introduction
Landslide is a natural phenomenon in which the shear strength of the rock-soil mass on a slope is reduced by environmental influence and slides along a soft surface or weak zone towards the front of the slope as a whole or in pieces under the action of gravity. With the continuous growth of global population and the gradual extension of human activities (Zhang et al., 2023a), landslide disasters are occurring more frequently and causing more serious loss of life and property. Therefore, according to the engineering geological characteristics of rock-soil mass in landslide areas, it is of great practical significance for the safety of people and properties to study the genetic mechanism of landslides and develop reasonable support design to improve the stability of slopes (Ukleja, 2020; Zhu et al., 2021; Zhang et al., 2023b).
Several principles of landslide management were summarized through years of engineering experience: Several principles of landslide management were summarized through years of engineering experience: 1) find out the history of landslide occurrence, the current situation and engineering geological characteristics (Jimenez-Peralvarez, 2018); 2) try to avoid landslide areas or pre-reinforcement (Zhang et al., 2021); 3) eliminate landslide hazards at once to avoid repeated treatment (Tiranti and Cremonini, 2019); 4) if the scale of landslides is large, the overall planning should be done; 5) timely management in the early development period of landslide (Yin et al., 2014); 6) to ensure the premise of safety to minimize the cost of the project (Martin et al., 2021); 7) to take effective design solutions and scientific and efficient construction (Cui et al., 2022); 8) Form a dynamic response mechanism to ensure dynamic design and dynamic construction (He et al., 2008; Wang et al., 2022b); 9) focus on inspection, repair and maintenance of facilities (Liao et al., 2022).
The main methods of landslide management include bypassing (Bhasin and Aarset, 2020), drainage (Haugen, 2017; Wang G. et al., 2022) and mechanical balance (Du et al., 2021). The Xinyan landslide is an existing road slide, which cannot be rerouted. The cost of using culvert or bridge solution is too high, and the method of chemical improvement is technically complex, difficult and costly. Therefore, a combination of drainage and mechanical balance is chosen to ensure complete management of the Xinyan landslide.
Among the methods of landslide treatment, anti-slide piles are an effective measure (Zhang, 2000). Earlier, when calculating the load distribution of anti-slide pile, the pile group is usually simplified to single pile for force analysis. However, the anti-slide pile interacts with the soil between the piles, i.e., there is an arching effect, which makes the stress migrate from the soil to the pile, so that the anti-slip pile acts as an obstacle to sliding, and the corresponding stress characteristics of pile become more complex (Xia et al., 2008). Therefore, the formation mechanism and influencing factors of soil arching effect must be studied in depth in order to improve the supporting of anti-slide pile on landslide and to obtain more accurate load distribution characteristics of pile (Zheng, 2005).
The current research focuses on the influence factors of soil arch, such as pile spacing and material parameters between piles and soil, but not enough research on the arrangement of piles (Liang et al., 2020). Therefore, this paper selects the Xinyan landslide in Yanbian area of Jilin Province, which is an expansive rock slope, establishes the three-dimensional model of the Xinyan landslide, and simulates the effect of pile arrangements of anti-slide piles on the soil arching effect between piles. The overall three-dimensional model avoids the disadvantages of the two-dimensional plane model and the partial three-dimensional model of insufficient consideration of spatial and boundary effects, making the simulation study results more accurate and reliable.
Data and method
Date
The Xinyan slide is located within pile number K58 + 750 to K59 + 030 of the under-construction Wangyan highway, and the filling subgrade at the bottom of the highway is cut through by the Xinyan slide body (location and overall topography of the landslide is shown in Figure 1). The plane morphology of the slide body is spindle-shaped, with a long axis length of about 230 m and a short axis length of about 110 m. The intersection angle between the main sliding direction (113°) and the extension direction of Wangyan highway is about 60°. The landslide plane area is about 1.7×104 m2, the slope height is about 15 m, the average thickness of the slide body is about 8 m, and the total volume of the slide body is about 1.36×105 m3. The slide material composition from top to bottom is fill, silty clay, fully weathered mudstone and sandstone respectively.
Method
For the soil arch effect, the theory that the residual sliding force at the rear of the pile is borne by the soil arch behind the pile was propose by Chang (1998). On this basis, Zhou et al. (2004) derived the mechanical model of the soil arch behind the pile by considering the static balance of shear strength and sliding force of the soil in the triangular compression zone behind the piles (Zhou et al., 2009). The calculation model is shown in Figure 2.
In the Figure 2, S is the net distance between adjacent anti-slide piles, t is the section thickness of the soil arch, r is the height of the soil arch, q is the residual sliding force (assumed as uniform force) at the rear of the pile, Fx and Fy are the reaction forces at the end of the soil arch, and the equation of the soil arch axis is:
In Equation 1,
In Equation 2,
In Equation 3,
In Equation 4,
The following conditions should be satisfied during the model calculation:
(1) The model should satisfy the static balance, and the side friction of the pile should not be less than the pressure on the soil arch.
(2) The model should satisfy the strength conditions of the soil arch on dangerous section, and the maximum value of sectional stress should not be greater than the allowable value.
(3) The model should satisfy the triangular compression zone is not damaged, and anti-slide piles need to provide the arch foot (Figure 3).
According to the geometric characteristics of the triangular compression zone at the arch foot, the calculated expression of the pile spacing can be obtained as:
where b is the width of the pile, c is the cohesion, φ is the internal friction angle, α is the base angle of the triangle.
Results
According to the genetic mechanism of the Xinyan landslide, anchor cable, anti-slide pile and pressure foot treatment measures were developed for the influencing factors of the landslide, and combined with the drainage of the slope surface and slope body. All three methods can improve the landslide stability. There are many methods for predicting slope stability (Wang et al., 2022a), and the highest values of 39.79%, 43.13%, and 85.28% are improved respectively by using Janbu, Sarma, and Morgenstern-Price method to calculate the safety coefficients of the three known profiles after treatment in this paper (Shanguan, 2014). Anti-slide piles have the advantages of large anti-slide capacity, good support effect, flexible setting and small disturbance to the slide, which is one of the common methods for landslide management.
This paper mainly studies the soil arching effect of anti-slide pile, so the modeling process is based on the geological characteristics of the Xinyan landslide area, and the Flac-3D software is used to build the landslide 3D model (Figure 4). The model is used to study the soil arching effect between piles at different positions, and focuses on the influence of double-row piles arrangements on soil arching effect. The values of material parameters in the model are shown in Table 1.
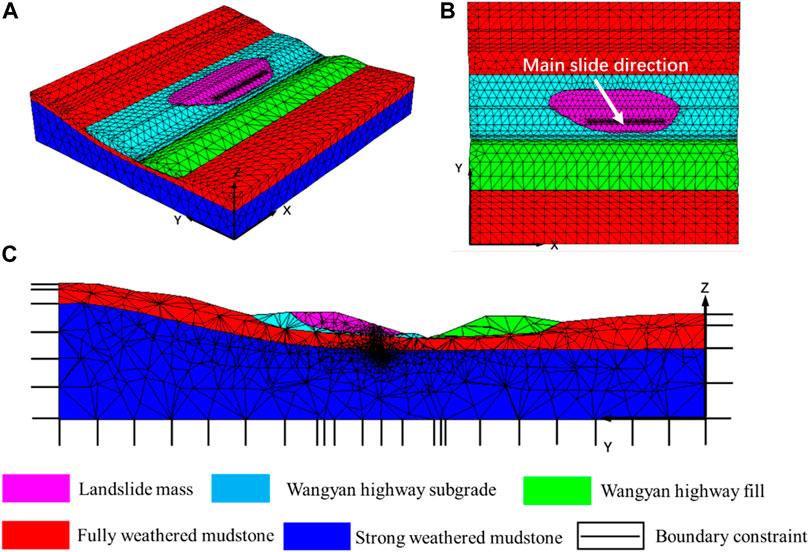
FIGURE 4. Illustration of 3D model and mesh subdivision: (A) Side view; (B) Plan view; (C) Cutaway view.
To avoid the effect of boundary constraint, the extent of the model boundary should be two times the extent of the studied landslide. Therefore, the model area is 445 m long in the y-direction and 500 m long in the x-direction. The boundary of the mechanical model is constrained on the left and right sides (x-direction), with horizontal constraints on the front and rear sides (y-direction), vertical constraints on the undersurface (z-direction), and no constraints on the superface. Considering that the neotectonic movement of the tectonic stress at the location of the Xinyan landslide is not active, and that the stratum there is mainly composed of fill, fully weathered fine sandstone and clay rock, such rock-soil mass is not enough to store the in-situ stress. Therefore, when considering the initial stress field of the model, only the effect of the gravity field is considered, and the tectonic stress field is not considered (Shanguan, 2014). All the constitutive models of rock-soil mass are used the Moore-Coulomb model, and the elasticity model is used for the anti-slide piles. The subdivision elements are tetrahedral elements, and the elements get larger gradually from top to bottom.
The cross-sectional form of double-row anti-slide piles is circular, which is divided into parallel arrangement and quincunx arrangement. Pile diameter d) is 2.5 m, pile distance (L=8d) is 20 m, and pile row distance (L’=3d) is 7.5 m, as shown in Figure 5.
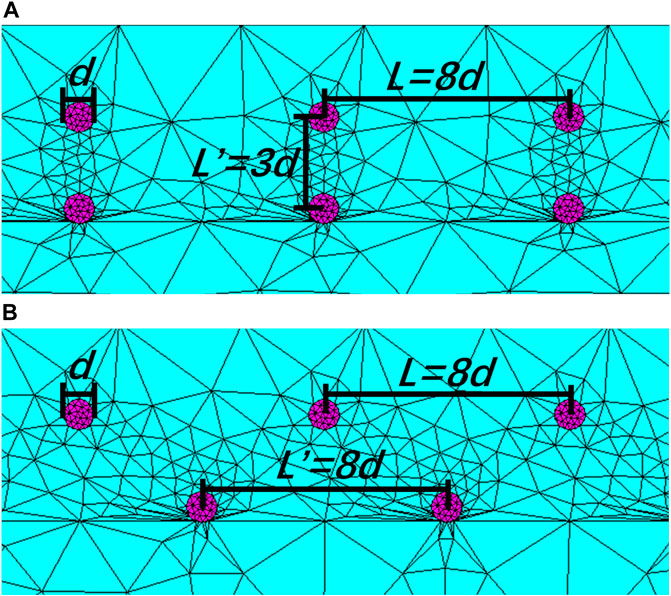
FIGURE 5. Different pile arrangements of double-row anti-slide pile: (A) Parallel arrangement; (B) Quincunx arrangement.
Discussion
Stress distribution of soil between piles
When the anti-slide pile passes through the landslide layer, the sliding displacement of the soil between the piles is larger and the soil arch effect is significant, which bears most of the load. Therefore, the plane at a depth of 22.6 m is chosen to observe the soil stress distribution between the piles. Stress distribution (
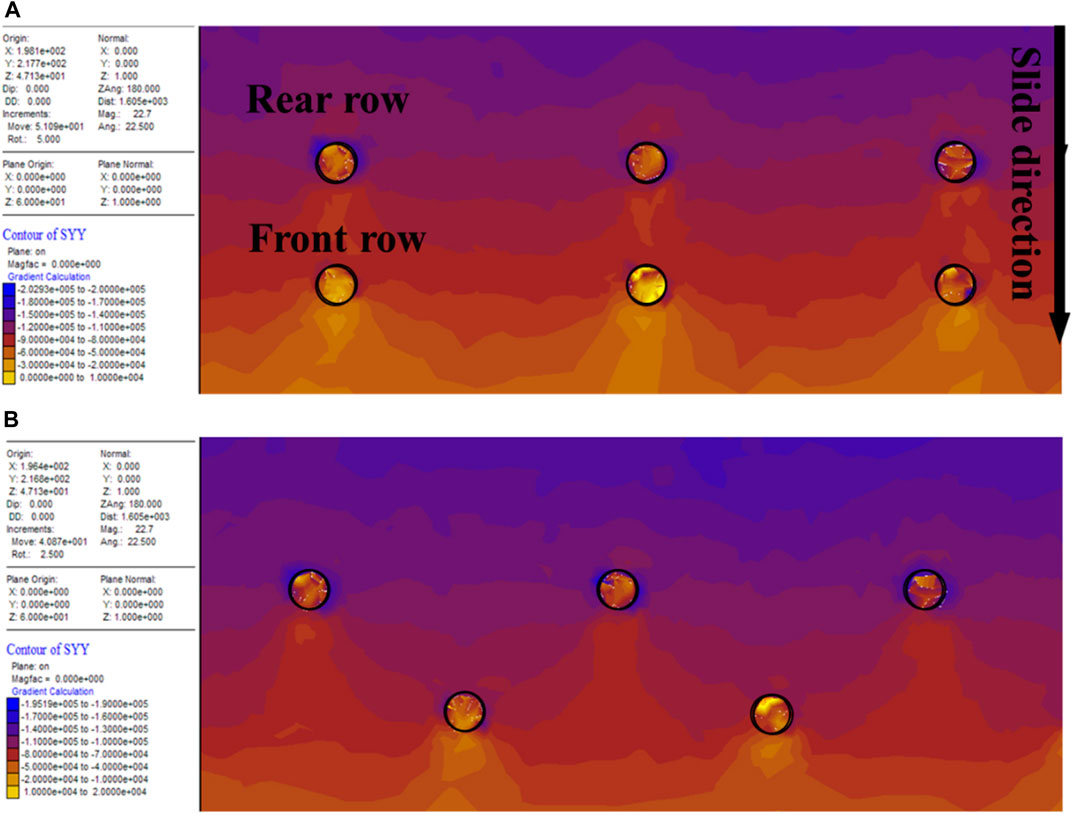
FIGURE 6. Stress distribution with different pile arrangements: (A) Parallel arrangement; (B) Quincunx arrangement.
Displacement distribution of soil between piles
Figure 7 shows the horizontal (y-direction) displacement distribution of soil between piles under different pile arrangements. The blue area in the graph indicates smaller displacement and the red area indicates larger displacement. The displacement of the soil from the rear piles to the front piles gradually decreases, and the displacement in the middle is large and small on both sides. The shape is distributed in an arch convex to the front of the pile, which is the displacement arch. Compared with the parallel arrangement, the soil displacement between piles is smaller in quincunx arrangement, and the soil displacement between piles decreases faster from the rear row piles to the front row piles.
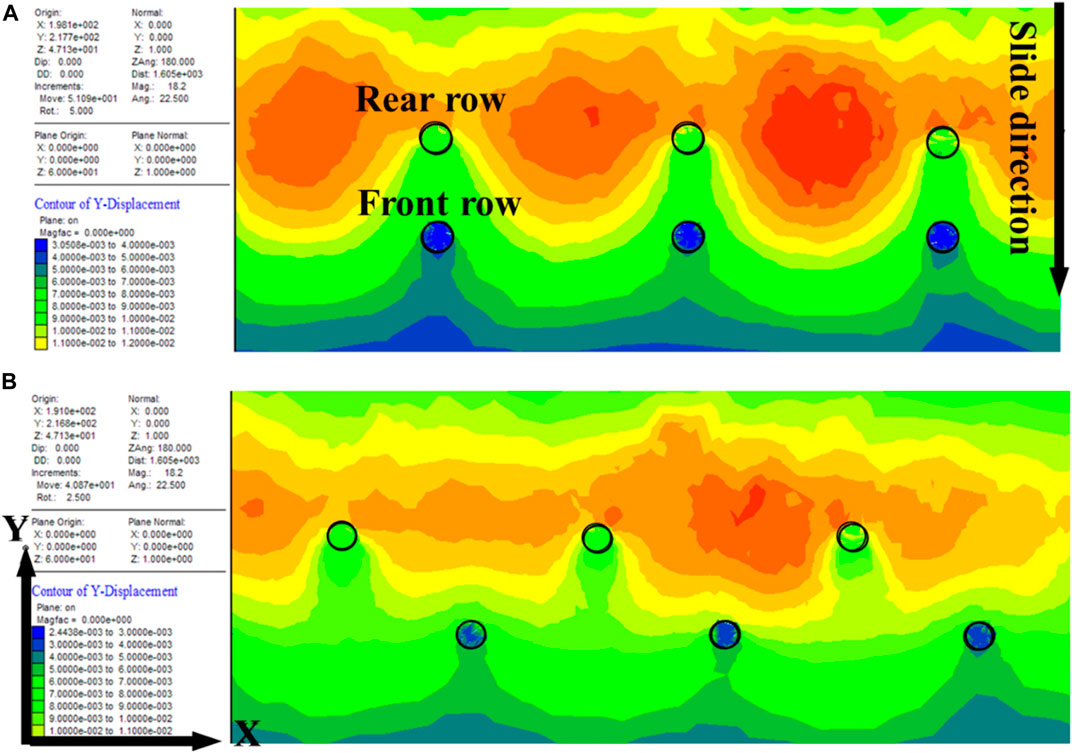
FIGURE 7. Displacement distribution in y-direction with different pile arrangements: (A) Parallel arrangement; (B) Quincunx arrangement.
Figure 8 shows the x-direction displacement distribution of soil between piles under different pile arrangements. The blue area indicates larger displacement in the x-negative direction, the red area indicates larger displacement in the x-positive direction, and the green area indicates smaller displacement and close to zero in the x-direction. the x-direction displacement of the soil on the side of the pile is larger while the x-direction displacement of the soil between the piles is small and close to zero with parallel arrangement. The soil displacement is mainly in the y-direction of the vertical pile center line, while the x-direction displacement at the pile side of the front row piles is significantly reduced compared with the rear row piles. Large x-directional displacements occur in the soil on the side of both the front and rear rows piles with the quincunx arrangement.
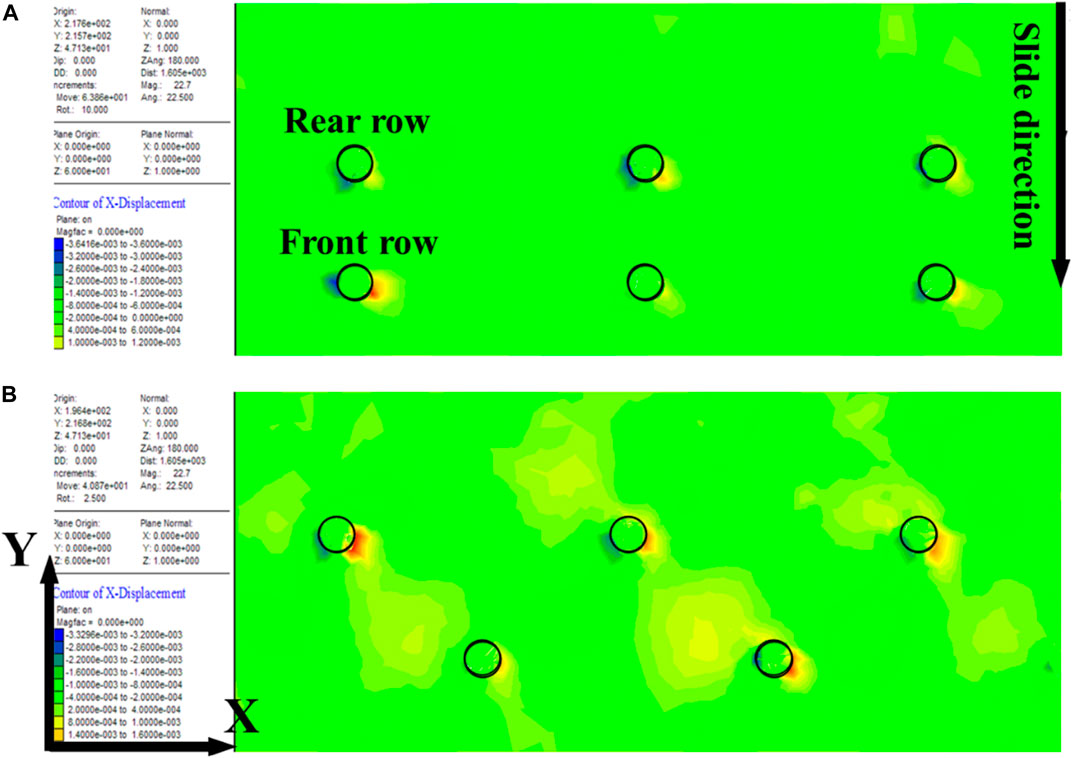
FIGURE 8. Displacement distribution in x-direction with different pile arrangements: (A) Parallel arrangement; (B) Quincunx arrangement.
Anti-slide piles are arranged in vertical sliding direction, when the soil is prevented from moving behind the piles to the front of the piles by the anti-slide piles, it will flow around both sides. The soil mainly flows around the rear row piles, and most of the soil only flows around once in the process of moving to the front slope with parallel arrangement, while the soil flows around the front and rear rows piles, and most of the soil needs to flow around twice to reach the front slope with quincunx arrangement. Therefore, the quincunx pile arrangement is more conducive to stopping the forward creep slipping of the soil between the piles.
The quincunx arrangement can effectively stop the soil between piles from sliding toward the front of the slope, mainly because the quincunx arrangement indirectly shortens the pile distance. According to previous research results (Wang, 2005), the influence of pile distance on soil arching effect is most significant, so any method that can directly or indirectly shorten pile distance can effectively enhance the soil arching effect between piles.
Load sharing ratio of pile with two pile arrangements
The load sharing ratio of piles is an important indicator of the soil arching effect (Cao and Meng, 2012). For the double-row piles, the front and rear rows piles and the soil between the rows form a whole and resist the residual sliding force of the landslide together (Wu and Zhang, 2007). The load sharing ratio among the three is important for the effect of double-row anti-slide piles to reinforce the slope and design safe and economical anti-slide piles. The anti-slide pile within a depth of 6 m is located within the slip body, which bears most of the load. Due to the soil in front of the piles being less at a depth of 2 m and above, the horizontal cross-sections at depth of 3 m, 4 m, 5 m and 6 m are chosen to calculate the load sharing ratio of the front and rear rows piles and soil between piles (Table 2).
Table 3 shows the load sharing ratio of double-row anti-slide piles in parallel and quincunx arrangements. The maximum value of load sharing ratio for quincunx arrangement appears at a depth of 3 m as 76%, the maximum value for parallel arrangement appears at a depth of 4 m as 69%, and the average value for both is 67.25% and 60%. Both the maximum and average values of quincunx arrangement are larger than parallel arrangement, so the quincunx arrangement is conducive to the soil arching effect, which makes more residual sliding force to migrate to the pile body.
From Table 2 and Table 3, the load sharing ratio of front and rear piles is different with increasing depth, the load in depth 3–5 m is mainly borne by rear piles, while the load in depth 5–6 m is mainly borne by front piles. But the load sharing ratio of rear piles is greater than that of front piles, the design should improve the strength of rear piles, increase the reinforcement, or reduce the diameter of front piles appropriately. The load sharing ratio of the row piles is greater than that of the parallel arrangement in the quincunx arrangement, and the load sharing ratio of the front row of piles is similar in both cases. The soil between the rows in the quincunx arrangement bears little load, while the soil between the rows in the parallel arrangement bears a larger load, so that the anti-slide force provided by the soil between the piles can be used as a safety reserve to improve the safety factor of the slope.
Conclusion
Due to the expansibility of the rock-soil mass of the Xinyan landslide, its sliding damage is influenced by a variety of factors. In this paper, based on the anti-slide pile reinforcement scheme of the Xinyan landslide, a three-dimensional model was established by FLAC-3D to simulate the soil arching effect between piles with different arrangements of double-row piles, the following conclusions are drawn:
(1) The soil arch is generated between two adjacent piles in the same row and parallel to each other in parallel arrangement; while it is generated between the front row pile and the adjacent rear piles in quincunx arrangement.
(2) The soil flows around the piles only once in parallel arrangement, while it flows around piles twice in quincunx arrangement, which is more conducive to stopping the creep slipping of the soil between the piles.
(3) When piles are arranged in double rows, the rear row piles bear a greater load than the front row piles. Compared with parallel arrangement, the load sharing ratio of rear pile is greater and the support effect is better with quincunx arrangement.
(4) In this paper, the study of soil arch effect is limited to numerical simulation analysis, which makes the deviation between simulated and real values. The next research will focus on establishing a physical model of the landslide and adjusting the numerical model through physical model tests to making the simulation results closer to the real values.
Data availability statement
The data that support the findings of this study are available from SB but restrictions apply to the availability of these data, which were used under license for the current study, and so are not publicly available. Data are however available from the authors upon reasonable request and with permission of DX. Requests to access the datasets should be directed to DX, MTM5MjE3ODU5OTJAMTYzLmNvbQ==.
Author contributions
YS: Investigation, Resources, Writing–Original Draft DX: Data Curation GW: Methodology, Writing–Review and Editing DL: Data Curation SB: Software WW: Validation. All authors contributed to the article and approved the submitted version.
Funding
This study is supported by the National Natural Science Foundation of China (No. 42207209), Doctoral Research Initial Fund of Jilin Jianzhu University (No. 86122401) and Jilin Provincial Science and Technology Development Project of China (No. YDZJ202201ZYTS647). The work is also supported by Crosswise Tasks of Changchun Jianye Group Corporation (No. 73800801) and Jilin Gongcheng Project Management Limited Company (No. 73800801). The funders were not involved in the study design, collection, analysis, interpretation of data, the writing of this article, or the decision to submit it for publication.
Conflict of interest
The authors declare that the research was conducted in the absence of any commercial or financial relationships that could be construed as a potential conflict of interest.
Publisher’s note
All claims expressed in this article are solely those of the authors and do not necessarily represent those of their affiliated organizations, or those of the publisher, the editors and the reviewers. Any product that may be evaluated in this article, or claim that may be made by its manufacturer, is not guaranteed or endorsed by the publisher.
References
Bhaisin, R., and Aarset, A. (2020). Application of Norwegian Method of Tunnelling (NMT) principles to bypass landslides in mountainous terrain. Geotechnics Sustain. Infrastructure Dev. 62, 273–279. doi:10.1007/978-981-15-2184-3_34
Cao, Q. L., and Meng, Y. K. (2012). Study on the load-sharing ratio of piles and soil about the squared piles composite foundation of sluice. Adv. Hydrology Hydraulic Eng. 212, 963–969. doi:10.4028/www.scientific.net/AMM.212-213.963
Chang, B. P. (1998). A study on soil arch between stabilizing piles and critical span. Proc. Landslides 3, 73–78. doi:10.3969/j.issn.1004-9665.2004.01.018
Cui, P., Ge, Y. G., Li, S. J., Li, Z. H., Xu, X. W., Zhou, G. G. D., et al. (2022). Scientific challenges in disaster risk reduction for the Sichuan-Tibet Railway. Eng. Geol. 309, 106837. doi:10.1016/j.enggeo.2022.106837
Du, Y., Yan, E. C., Gao, X., Mwizerwa, S., Yuan, L. W., and Zhao, S. (2021). Identification of the main control factors and failure modes for the failure of Baiyuzui landslide control project. Geotechnical Geol. Eng. 39 (5), 3499–3516. doi:10.1007/s10706-021-01707-0
Haugen, B. D. (2017). A design method for landslide surface water drainage control. Environ. Eng. Geoscience 23 (4), 275–289. doi:10.2113/gseegeosci.23.4.275
He, K. Q., Wang, S. Q., Du, H., and Wang, S. J. (2008). The dynamic parameter of rainfall: Its importance in the prediction of colluvial landslides. Bull. Eng. Geol. Environ. 67 (3), 345–351. doi:10.1007/s10064-008-0143-4
Jimenez-Peralvarez, J. D. (2018). Landslide-risk mapping in a developing hilly area with limited information on landslide occurrence. Landslides 15 (4), 741–752. doi:10.1007/s10346-017-0903-y
Liang, L. J., Xu, C. J., Chen, Q. Z., and Chen, Q. S. (2020). Experimental and theoretical investigations on evolution of soil-arching effect in 2D trapdoor problem. Int. J. Geomechanics 20, 06020007. doi:10.1061/(ASCE)GM.1943-5622.0001643
Liao, S. F., Ye, M. Z., Yuan, R. C., and Ma, W. Z. (2022). Unmanned aerial vehicle surveying and mapping trajectory scheduling and autonomous control for landslide monitoring. J. Robotics 2022, 1–13. doi:10.1155/2022/2365006
Martin, J. G. C., Scolobig, A., Linnerooth-Bayer, J., Liu, W., and Balsiger, J. (2021). Catalyzing innovation: Governance enablers of nature-based solutions. Sustainability 13 (4), 1971. doi:10.3390/su13041971
Shanguan, Y. L. (2014). “Study on genetic mechanism and pile-soil contact effect of anti-slide piles of Xinyan landslide,”. dissertation/doctor's thesis (Changchun, China: Jilin University).
Tiranti, D., and Cremonini, R. (2019). Editorial: Landslide hazard in a changing environment. Front. Earth Sci. 7, 1–3. doi:10.3389/feart.2019.00003
Ukleja, J. (2020). Stabilization of landslides sliding layer using electrokinetic phenomena and vacuum treatment. Geosciences 10 (8), 284. doi:10.3390/geosciences10080284
Wang, G. J., Zhao, B., Wu, B. S., Wang, M. L., Liu, W. L., Zhou, H. M., et al. (2022a). Research on the macro-mesoscopic response mechanism of multisphere approximated heteromorphic tailing particles. Lithosphere 2022, 1977890. doi:10.2113/2022/1977890
Wang, G. J., Zhao, B., Wu, B. S., Zhang, C., and Liu, W. L. (2022b). Intelligent prediction of slope stability based on visual exploratory data analysis of 77 in situ cases. Int. J. Min. Sci. Technol. 33 (1), 47–59. doi:10.1016/j.ijmst.2022.07.002
Wang, G., Zhao, B., Lan, R., Liu, D. W., Wu, B. S., Li, Y. J., et al. (2022c). Experimental study on failure model of tailing dam overtopping under heavy rainfall. Lithosphere 2022, 5922501. doi:10.2113/2022/5922501
Wang, Q. K. (2005). Discussion on the soil arching effect and the critical spacing between adjacent anti-slide piles. J. Wuhan Univ. Technol. 27 (8), 64–67. doi:10.3321/j.issn:1671-4431.2005.08.019
Wu, C. J., and Zhang, Z. X. (2007). Numerical analysis of soil arching effect in piles in the slope engineering. Chin. J. Undergr. Space Eng. 3 (7), 1305–1309. doi:10.3969/j.issn.1673-0836.2007.z1.027
Xia, Y. Y., Zheng, X. Y., and Rui, R. (2008). “Numerical analysis of soil-arch effect of anti-slide piles,” in Landslides and engineered slopes: From the past to the future (Boca Raton, Florida, United States: CRC Press), 1011–1016.
Yin, Z. Q., Qin, X. G., Yin, Y. P., Zhao, W. J., and Wei, G. (2014). Landslide developmental characteristics and response to climate change since the last glacial in the upper reaches of the Yellow River, NE Tibetan Plateau. ACTA Geol. Sinica-English Ed. 88 (2), 635–646. doi:10.1111/1755-6724.12219
Zhang, C. L., Jiang, J. L., Lei, D., Asghar, A., Su, L. J., and Wang, Z. M. (2021). Large-scale shaking table test on seismic behaviour of anti-slide pile-reinforced bridge foundation and gravel landslide: A case study. Bull. Eng. Geol. Environ. 80 (2), 1303–1316. doi:10.1007/s10064-020-02006-3
Zhang, Z. Q., Li, M., Wang, J., Yin, Z. Q., Yang, Y. Z., Xun, X. Y., et al. (2023a). A calculation model for the spatial distribution and reserves of ground ice - a case study of the Northeast China permafrost area. Eng. Geol. 315, 107022. doi:10.1016/j.enggeo.2023.107022
Zhang, Z. Q., Li, M., Wen, Z., Yin, Z. Q., Tang, Y. F., Gao, S. R., et al. (2023b). Degraded frozen soil and reduced frost heave in China due to climate warming. Sci. Total Environ. 893, 164914. doi:10.1016/j.scitotenv.2023.164914
Zhang, Z. Y. (2000). The present status, technical advance and development trends of landslide remedial measures. J. Geol. Hazards Environ. Preserv. 2, 89–97+181. doi:10.3969/j.issn.1006-4362.2000.02.001
Zheng, X. Y. (2005). “Numerical simulation studies on the sustentation structure of anti-sliding piles,”. dissertation/master's thesis (Wuhan, Hubei: Wuhan University of Technology).
Zhou, D. P., Wang, H. L., and Sun, H. W. (2009). Micro-pile composite structure and its design theory. Chin. J. Rock Mech. Eng. 28 (7), 1353–1362. doi:10.3321/j.issn:1000-6915.2009.07.008
Zhou, D. P., Xiao, S. G., and Xia, X. (2004). Discussion on rational spacing between adjacent anti-slide piles in some cutting slope projects. Chin. J. Geotechnical Eng. 27 (8), 64–67. doi:10.3321/j.issn:1000-4548.2004.01.025
Keywords: anti-slide pile, soil arching effect, landslide, double-row piles, pile arrangements
Citation: Shangguan Y, Xue D, Wang G, Li D, Bao S and Wang W (2023) A study on soil arching effect of anti-slide pile considering different pile arrangements. Front. Earth Sci. 11:1195552. doi: 10.3389/feart.2023.1195552
Received: 28 March 2023; Accepted: 26 June 2023;
Published: 06 July 2023.
Edited by:
Guangjin Wang, Kunming University of Science and Technology, ChinaReviewed by:
Ze Zhang, Northeast Forestry University, ChinaZhongqiong Zhang, Chinese Academy of Sciences (CAS), China
Copyright © 2023 Shangguan, Xue, Wang, Li, Bao and Wang. This is an open-access article distributed under the terms of the Creative Commons Attribution License (CC BY). The use, distribution or reproduction in other forums is permitted, provided the original author(s) and the copyright owner(s) are credited and that the original publication in this journal is cited, in accordance with accepted academic practice. No use, distribution or reproduction is permitted which does not comply with these terms.
*Correspondence: Gang Wang, ZG91YmxlNzI5NDIyQGdtYWlsLmNvbQ==