- Department of Geology of Oil and Gas, Saint-Petersburg Mining University, Saint-Petersburg, Russia
A large number of oil and gas accumulations at deep and ultra-deep depths, recently revealed in the world due to the development of drilling technologies, forces a revision of theoretical ideas on many issues, including conditions of oil and gas formation and hydrocarbon conservation in zones of high pressures and temperatures, as well as evaluating the possibility of conservation and formation of reservoirs. Among the most discussed, in this regard, are the issues determining the processes and rate of oil and gas formation at deep and ultra-deep depths, which differ significantly from those at small and medium depths: Thermobaric conditions responsible for the possibility of formation, phase distribution and conservation of hydrocarbons, geochemical indicators—type of kerogen, and its transformation, responsible for the phase distribution and staging of generation peaks—lithological composition of kerogen-bearing strata—the rate and scale of dip and uplift and the timing of exposure to high temperatures—the formation of overlapping saline strata and the presence of deep conductive faults that significantly affect temperature and pressure gradients. Analysis of the influence of the above factors on the prospects of deep-lying complexes taking into account new studies and achievements, mainly in the sedimentary basins of China (Tarim, Jungar, Ordos, Sichuan), the Gulf of Mexico and offshore Brazil (Santos) allowed typifying the sedimentary basins and major depressions of Russia, with the allocation of areas with high and ultra-low depth potential for hydrocarbon studies. Thermobaric and historical and geological criteria have been developed and a methodology for detailed study of promising areas has been proposed, including a complex of geochemical studies of hydrocarbons, extracts of bitumoids (pyrolytic, chromatographic) and lithological and petrophysical core studies aimed at assessing the prospects of oil and gas content of deep complexes in Russian sedimentary basins and the subsequent localization of the most promising areas for deep drilling.
1 Introduction
A number of global events in the field of prospecting and exploration of oil and gas accumulations are attracting more and more attention to the theoretical issues of oil and gas formation, taking into account the possibility of using the scientific results obtained in practice, reinforcing them with actual discoveries replacing the raw material base of traditional objects that is being eliminated as a result of extraction.
Among the most significant events that have substantially affected the state of the raw material base of hydrocarbons in the world, it is necessary to include such as:
- Scientific foundation of the oil and gas potential and development of technologies for the development of the raw material base of low-permeable and “shale” oil and gas-saturated reservoirs (which made it possible to radically change the energy balance and, for example, let the United States became the gas exporter due to the share of its production of more than 70% of such facilities (Alekseev et al., 2017);
- Development of deep drilling technologies and obtaining numerous facts of detecting accumulations of oil and gas at great depths, as well as new data that significantly clarify ideas about the thermobaric conditions of formation and the possibility of preservation of hydrocarbons (Dvoynikov et al., 2022).
The identification of oil and gas deposits at deep and ultra-deep depths (5–8 km or more) allowed us to establish that the boundary values of the thermobaric conditions for the formation and preservation of hydrocarbon deposits differ significantly from those assumed (a priori) earlier and justified experimentally (according to the postulates of the sedimentary migration (organic) theory of the origin of oil and gas. Some assumptions previously used as key ones directly in the practice of geological exploration have ceased to be relevant. First of all, this concerns the temperature, and, consequently, the depth interval of the processes of generation of hydrocarbons of various phase composition and their intensive transformation, up to complete destruction, as well as the influence of abnormally high and abnormally low pressure on the preservation of formed accumulations of oil and gas. The second important aspect determining the possibility of the presence of hydrocarbon accumulations at deep depths is the possibility of preserving the primary void space of reservoir rocks or the formation of fractured and secondary reservoirs, as well as the influence of epigenetic transformations on their formation (Gubin, 2004; Belozerov and Gubaydullin, 2020; Khafizov et al., 2020; Kosenkova et al., 2022). At the same time, an alternative and effective approach that could replace the traditionally used and proven to be highly predictive is basin modeling, which is based on algorithms of balance equations and models for the conversion of organic matter into hydrocarbons, developed in the USSR by the efforts of Sokolov V.A., Wassoevich N.B., Neruchev S.G., Rogozina E.A. and others (Dobrjanski and Andrejev, 1954; Neruchev et al., 1976; Sokolov V.A., 1980) and European and American researchers (Tissot and Welte, 1981; Peters et al., 1994) have not been proposed. Despite a huge number of experiments to study the effects of temperatures and pressures on inorganic compounds, to study the effect of methane inflow on the transformation of organic matter in order to substantiate the production of complex hydrocarbon compounds, to substantiate possible forms (radicals) of finding hydrocarbon compounds at deep and ultra-deep depths (Kudryavtsev, 1951; Kudryavtsev, 1971; Porfiriev, 1987; Ermolkin et al., 2008) a significant practical contribution to the search for oil accumulations and they did not have, because they were not adapted into any technology of search and exploration, which proved its effectiveness. Of course, the impossibility of obtaining direct observations at ultra-deep depths and conducting experiments that fully simulate natural thermobaric conditions, and most importantly the influence of time, continues to “excite” the minds of some researchers in the direction of the “deep origin” of oil and gas today. The most important facts obtained in recent years, which must be taken into account, along with the timing of hydrocarbon deposits at deep and ultra-deep depths to certain geological conditions, were the systematic identification in “special” conditions of deep depths of very rich in organic matter of oil and gas source formations that for one reason or another did not realize their full potential, or the identification of anomalous zones of high pressures, ensuring the preservation of liquid hydrocarbons (not complete cracking) in sedimentary basins with high immersion rates and “avalanche-like” sedimentation.
The concept of “deep origin” considering the possibility of synthesis of hydrocarbons from inorganic substances (carbonates, CO2 and other carbon-containing substances) at deep depths at high temperatures and pressure allows the formation of accumulations of oil and gas at much deeper depths than those assumed by organic theory and, no less importantly, in which there are no oil and gas producing strata, primarily enriched with organic substance.
The problem of theoretical research in the direction of studying “deep oil”, which was developed in particular by N.A. Kudryavtsev Kudryavtsev, (1951); Kudryavtsev, (1971) and V.B. Porfiriev Porfiriev, (1961); Porfiriev, (1987), was the impossibility of using the models of oil and gas formation proposed by them to solve practical problems, which led to a damping of interest in these developments and, conversely, high efficiency and the possibility of provability (experimental) of oil and gas formation processes using balance equations developed by N.B.Vassojevich, S.G. Neruchev, E.A. Rogozina et al. Neruchev et al., (1976), the quantitative forecast, the forecast of the phase composition and the “explainability” of the formation of most of the identified deposits allowed “organics” to dominate in the study of standard depths.
Dobrjanski and Andrejev, 1954). noted that despite the huge scale of accumulated and buried organic matter (according to Ammosov, and then Neruchev S.G. (Neruchev et al., 1986). exceeding hundreds of billions of times the volume of all accumulations of oil and gas already discovered on Earth), the ambiguity of the accumulation mechanisms does not allow us to draw conclusions “how much oil will be collected”. S.G. Neruchev himself drew attention to the ambiguity of the accumulation coefficients in his calculations. According to V.B. Porfiriev the presence of areas of discrepancy between the scales and types of hydrocarbons to the potential of the oil and gas producing strata is noted, and the presence of deposits at depths greater than the depths of the oil and gas source formations is established, which determines the need to search for the causes of oil and gas potential in such areas and depth intervals (Aguilera et al., 2014; Guliyev et al., 2017; Liu et al., 2017).
It is established that the processes of oil and gas formation at deep depths differ significantly from the processes modeled in surface conditions (DeCelles and Giles, 1996; Eberth et al., 2001; Egorov, 2015; Egorov et al., 2021). Ultra-deep hydrocarbon systems are characterized by specific features of fluid migration (Samvelov, 1995; Cao and Bai, 2014; Kerimov et al., 2018), which affects the development of accumulation zones and the preservation of hydrocarbon accumulations due to the dominance of such mechanisms as migration along the planes of conducting disjunctiva, zones of increased fracturing of decompression, contacts of diapir embeddings, eruptives of mud volcanoes (DeCelles and Giles, 1996; Kerimov and Osipov, 2016; Ganguli et al., 2018; Li et al., 2020).
Currently, the contradiction between “theory” and “experiment” (i.e., between science and practice) has worsened due to the discovery of a number of oil fields below (deeper) the so–called “oil window”, where oil can be generated from organic residues (an oil window of 50°C–150°C, i.e., depths, as a rule, from 2.3 to 4.6 km; immediately below—a “gas window”, 150°C–200°C).
A large number of drilled deep and ultra-deep wells in the oil and gas basins of the world testify to a wide range of temperatures and pressures, as well as a complex multi-stage history of the thermal evolution of deep-submerged horizons. Temperatures, thermal evolution and pressure are the determining factors of the formation of hydrocarbons, differences in their phase composition, the possibility of their preservation, and these parameters are especially significant at deep and ultra-deep depths, which today are considered as one of the most important areas of theoretical and practical study of many oil and gas basins in China, the shelf of Brazil, the Gulf of Mexico and other sedimentary basins with a large thickness of the cover.
Based on a large amount of new data on the influence of temperatures, pressures, and the history of diving to deep depths, the concepts of the factors determining the formation, phase composition and preservation of hydrocarbons have been significantly refined. Thus, the phase composition of hydrocarbons in the deep layers of sedimentary basins of different types varies significantly and is determined by temperature, heating rate, pressure and types of the initial oil and gas source rocks containing organic matter deposited with the rock. At the same time, temperature is the most important factor controlling the formation of hydrocarbons and their phase state. Along with the temperature, factors that are almost ignored at shallow depths have a significant impact—this is the time (exposure) and the rate of temperature increase (Neruchev et al., 1986).
Thus, according to the nature of changes in the temperature regime of deep and ultra-deep horizons, sedimentary basins can be distinguished (Ren et al., 2020):
1- With late (young in age) rapid deflection, low geothermal gradient and, accordingly, low temperatures;
2- With late rapid deflection, high geothermal gradient, and, accordingly, high (but short-lived exposure time) temperatures;
3- With moderate (gradual) deflection, average geothermal gradient and late rise and, accordingly, cooling;
4- With early (ancient) intense deflection, rapid heating and subsequent lifting and cooling.
It has been established that under conditions of rapid temperature rise (type 1, 2, and 4), but a short heating time in deep and ultra-deep horizons, the conditions for the existence of oil and gas condensate deposits persist (Ren et al., 2020).
The most important thing for deep horizons is the close correlation between temperature and reservoir pressure, which determines the conditions of oil and gas formation and preservation of oil and condensate.
By the nature of the correlation between temperature and pressure at deep depths, all sedimentary basins can be divided into three groups:
I - high temperature and high pressure;
II - low average temperature and high pressure;
III - average temperature and low pressure (Ren et al., 2020).
It has also been revealed that excessive pressure prevents both the formation of hydrocarbons and the cracking of previously formed (reformation of liquid hydrocarbons into gaseous and simple compounds), which have fallen into zones of high pressures and temperatures for the second time. For all these types and combinations of thermodynamic regimes, the prospects for the oil and gas potential of deep and ultra-deep horizons differ significantly.
The temperature regime of deep horizons is determined by the endogenous regime (the influence of processes occurring at deep depths) and a whole group of processes and phenomena occurring at significantly lower depths, directly in the sedimentary part of the section, often associated with epigenetic transformations.
Endogenous regimes are the subject of extensive study of sedimentary basins at the early stages of regional studies. But with a more detailed study, the significance of the processes occurring at deep depths becomes less important, declarative and is rarely used for practical conclusion and determining the directions of further exploratory research.
Since the influence of processes occurring at ultra-deep depths is more noticeable for the base of sedimentary basins and section intervals located at deep depths, the influence of endogenous phenomena and processes is also one of the subjects of research. At the same time, the possibilities of studying the deep structure are extremely limited both by the depths of wells and by obtaining only indirect information when using geophysical observation methods and conducting laboratory experimental studies with modeling of natural processes. Such restrictions, on the one hand, generated a huge number of assumptions, including about the influence of deep processes on oil and gas formation, and on the other hand, due to the impossibility of establishing reliable links between the actual oil and gas potential and purely assumed “deep factors”, they did not allow them to be used in practice.
Approaches to the assessment of the oil and gas potential based on the concepts of the deep tectonic structure and processes occurring at significant depths according to studies (Sokolov, 1980; Malyshev, 2000; Malyshev, 2004; Egorov et al., 2021) are reduced to the use of the “evolutionary-genetic method, when modern sedimentary basins are considered as a system of conjugate paleobasins, consisting of their relics related to a certain tectonotype, formed in certain geodynamic regimes and those that have undergone a long geological evolution” (Egorov et al., 2021). At each stage of the development of paleobasins, favorable geological, geochemical and thermobaric conditions arose, affecting the processes of oil and gas formation, migration and accumulation and further redistribution of hydrocarbons between different complexes. Crucial importance in the modern distribution of hydrocarbon accumulations is attached to the latest stage of the formation of the basin structure.
According to the research of B.A.Sokolov (Sokolov, 1980): “each of the basins of the primary evolutionary and genetic series is characterized by a certain oil and gas geological regime, which includes the conditions for the development of the basin due to the mobility and direction of tectonic movements, their contrast, the type of the Earth’s crust and the rate of accumulation of sediments, as well as heat flow.”
As noted by most researchers, oil and gas geological regimes are not the same in sedimentary basins located within continents, oceans and transition zones. Within the continents, sedimentary basins of platforms and mountain-folded regions have fundamentally different regimes (Malyshev, 2000; Egorov et al., 2021).
The scales of oil and gas formation differ significantly, which vary from high and very high within the margins of young plates and folded-platform basins in the marginal parts of cratons and to low in post-orogenic depressions on Precambrian median massifs and very low (less than 1%) in orogenic intermountain depressions on the Epivariscian folded base (Malyshev, N.A. (2004).
According to modern concepts, in some sedimentary basins, due to the presence of wide salt layers in the section, relatively low geostatic pressure is provided (due to their relatively low density) and a “cooling” effect of the thermal field is observed (due to their relatively high thermal conductivity), which, despite the deep depths, leads to a delay in the transformation of organic matter in primary enriched (producing) rocks.
Large tectonic discontinuous disturbances, which serve as active conductors of deep heat and which, like salt layers, provide a “cooling” effect have an important effect on the temperature regime. This phenomenon has been little studied, but it is important along with understanding the contribution of the role of faults in the formation of secondary fracturing at deep depths, providing the possibility of reservoir formation, in addition to the preserved primary porosity (Prishchepa et al., 2022).
Separately, it should be noted the influence of high sedimentation rates (the so-called “avalanche” deposition) with variations in the accumulation of large capacities only in the Cenozoic, observed, for example, in the inland basins of China (Tarim, Dzungarian, Sichuan, Ordos) with massive identified deposits at deep depths and established oil and gas source rocks at deeper depths than at low sedimentation rates, which probably lead to stretching of the zones of transformation of organic matter (catagenesis), and the effect of the presence of zones of abnormally high reservoir pressures, which, in turn, have a significant impact on the catagenetic transformation of organic matter and the stability of the liquid hydrocarbon phase by inhibiting and significantly slowing down the processes of thermal destruction of organic components (Khafizov et al., 2020).
It is noted that (Kerimov and Osipov, 2016; Kerimov et al., 2018; Khafizov et al., 2020; Ren et al., 2020; Aleksandrova et al., 2023) the key factors of the transformation of OM into oil and the intervals of the location of the “oil window” are the rates of sedimentation and deflection (lowering) of the basin. The rate of deflection of the basin or the rate of elevation of its side structures determines the nature of sedimentation in the basin, due, in turn, to the size of negative relief forms, the rate of introduction of addition of material, its composition and mass. Depending on the individual contribution of these factors at each stage of evolution, the basin may be characterized by uncompensated, compensated and hypercompensated sedimentation (Kerimov et al., 2018).
The sedimentation rate is generally defined as the ratio of the sediment thickness (for the rates of deflection of the bottom of the basin, the sediment thickness together with the thickness of the water layer) and the absolute time of their formation, respectively, in meters per million years; the dimension of the resulting value is m/million years. Standard speeds are 5–10 m/1 million years. High and ultra-high sedimentation rates exceeding 100–1000 m/1 million years, respectively, characterize “avalanche-like” sedimentation (Kerimov et al., 2018).
A certain correlation has been established between the rate of deflection in the sedimentation basin, the rate, gradients of precipitation accumulation rates, on the one hand, and the facies-geochemical situation in the basin and the scale of hydrocarbon generation by oil and gas source rocks, on the other. In the catagenetic evolution (transformation) of organic matter, which begins with its accumulation and burial, as in all sedimentary processes, the sedimentation rate plays a leading role. Qualitative observations and quantitative analysis show that the concentration of OM in sediments increases with an increase in the rate of fossilization, but, reaching some optimum, then decreases (Fomin, 2011; Kerimov et al., 2018; Kontorovich et al., 2021).
In the Russian Federation, despite the rather high geological and geophysical study of oil and gas-bearing areas with a long history of exploration and development (North Pre-Caucasus, Volga-Ural, Pre-Caspian, Timan-Pechora and West Siberian, Lena-Tunguska oil and gas provinces), it can be stated that the number of discoveries at deep and ultra-deep depths (more than 5 km) is estimated units (Osipov, 2021) (Figure 1).
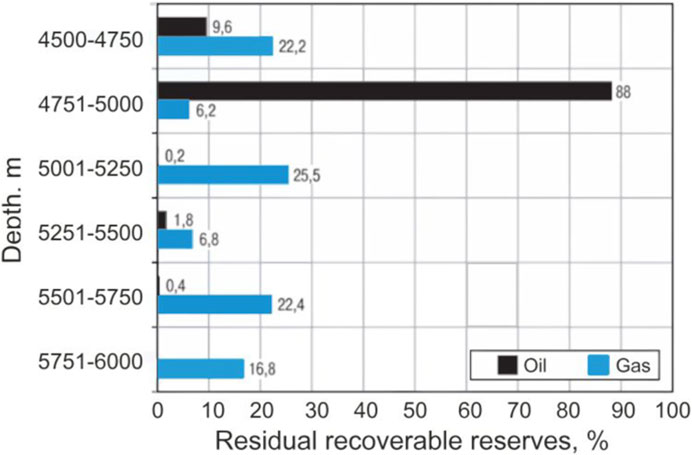
FIGURE 1. Distribution of residual recoverable oil and gas reserves of deep-lying deposits by depth intervals (Osipov, 2021).
At the same time, in sedimentary basins, a fairly significant volume of rocks is located at deep and ultra-deep depths.
If we consider regions with developed infrastructure and a long history of study, the undisputed leader in the volume of sedimentary cover located deeper than 5 km is the Pre-Caspian oil and gas province (hereinafter referred to as “OGP”) the eastern part of which is located in the Republic of Kazakhstan. Within the West Siberian basin, the deepest part of the sedimentary cover is developed in the central part and in the northern (aquatic), plunging towards the North Kara Depression, but in general, the proportion of deep-lying horizons is only 10%–15% (Figure 2).
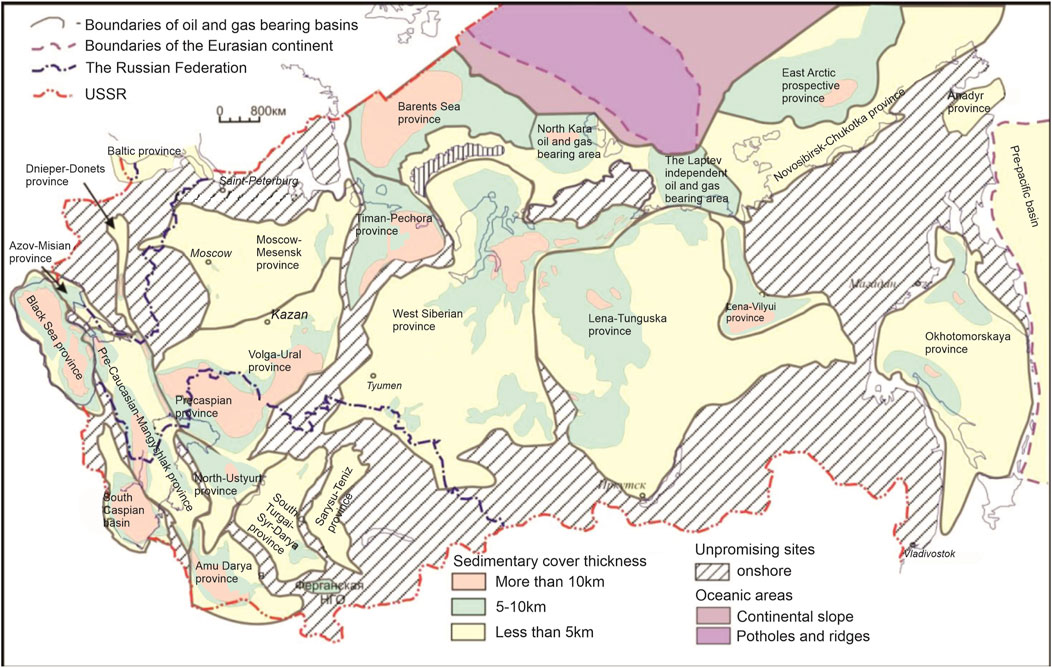
FIGURE 2. Map of oil and gas geological zoning of Russia and adjacent countries with selected areas with sediments at deep depths (Leonov et al., 2015).
Most of the sedimentary asymmetric basins have significant volumes of deep–lying rocks, mainly accumulated in the marginal parts of the platforms (foothill deflections—Pre-Ural, Pre-Caucasian, Pre-Verkhoyansky marginal deflections) along the mountain structures and characterized in that they are blocked by powerful and often filled with a high rate of accumulation (“avalanche”) sediments associated with the time of formation in adjacent areas of the corresponding orogenic structures (Samvelov, 1995; Kontorovich et al., 1998; Leonov et al., 2015; Litvinenko et al., 2017; Abukova and Volozh, 2021; Egorov et al., 2021) (Tables 1, 2).
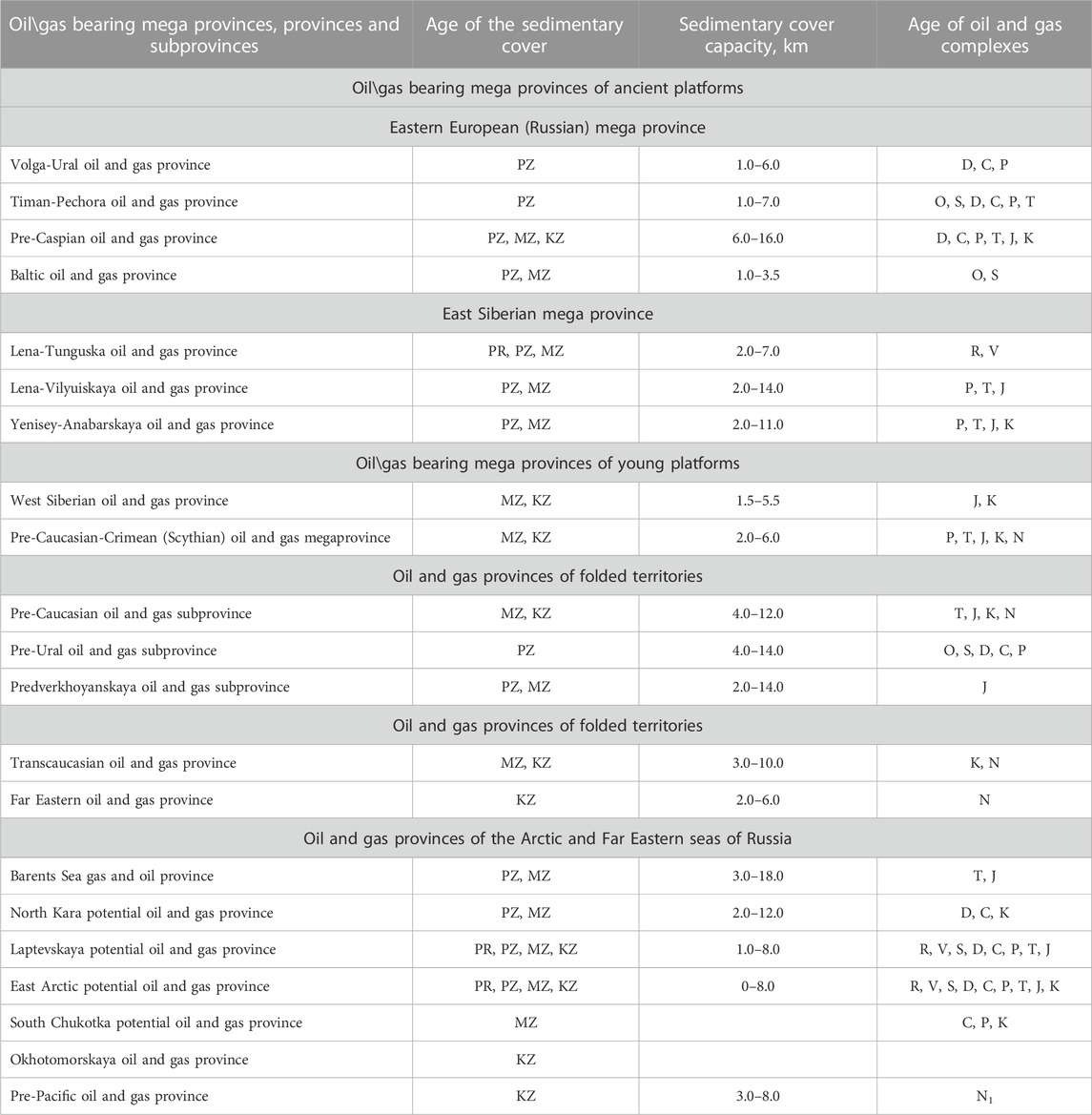
TABLE 2. Oil and gas province of the Russian Federation with the development of sedimentary complexes at deep depths.
The areas of development of deep-submerged sediments of the second type are mainly associated with ancient avlakogenes and, accordingly, the phases of their active deflection up to the beginning of inversion (Pre-Timansky, Pechora-Kolva, Vyatka, Kamsko-Belsky, Srednerussky, Pachelma, Moscowsky, Pripyat-Dnieper-Donetsk avlakogenes). Such areas, in general, are often characterized by an increased heat flow, since they are located above deep active structures and a wide development of tectonic discontinuities. The influence of faults as “cooling” channels on deep horizons has been established, for example, when comparing the temperatures of deep depths in neighboring areas of the TPP, in particular wells drilled within the Vuktyl shaft in the fault zone (West-Vuktyl No. 1 at a depth of 5,604 m, temperature—98.5°C, in the North-Vuktyl No. 214—5,025 m—90°C, Vuktylskaya No.58–6244 m—127°C, respectively, the geothermal gradient was 17°C–18°C/km), and in the Pechora-Kolva avlakogene in the Kolva ultra-deep well at a depth of 6,840 m, the temperature was 165°C, at a depth of 6,900 m—200°C, respectively, the geothermal gradient was 2.5°C–2.9°C/100m, i.e., significantly higher than in the fault zone. The deepest deposits represented by condensate and gas were found at a depth of 4,800–5,000 m. At the same time, within the Paleozoic part of the Pechora-Kolva avlakogene at depths from 4,500 to 4,800 m, 15 hydrocarbon deposits were identified, with differentiation from oil and gas condensate to oil.
Sedimentary basins such as the Pre-Caspian, Timan-Pechora, and East Barents Sea basins belong to areas with precipitation at deep depth deposited under avalanche sedimentation conditions.
The Pre-Caspian OGP also belongs to the sedimentary basins of the Russian Federation with a wide salt development in the section, followed by the Lena-Tunguska and Yenisei-Khatanga. There are some areas of deep-submerged sediments with the development of salts in the Timan-Pechora OGP.
In some areas of the ancient East European platform, in the areas of development of deep-submerged horizons—the Pre-Ural regional trough, the Dnieper–Donetsk depression—gas deposits were formed under conditions of immersion rates from 25 to 60 m/million years, occasionally more, and the gradients of the deflection rates exceeded 50 m/million years. At the same time, the main role in gas accumulation was played by the degassing of reservoir waters during inversion tectonic movements and the destruction of oil from previously formed oil and gas deposits. Thus, in the Timan-Pechora sedimentary basin, areas of deep depths are associated, first of all, with the marginal part of the plate—the Pre-Ural marginal trough and the ancient (Riphean) Pechora-Kolva avlakogene (separating from the Paleo-Ural orogen and the younger (Early Hercynian) Varandei-Adzva, where the depths of the Lower Paleozoic part of the section exceed 8,000 m (and in deflection and more (up to 10,000 m) (Figure 3).
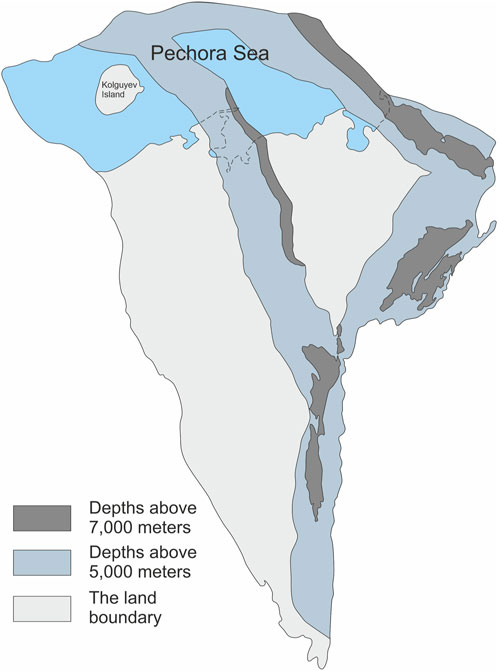
FIGURE 3. Structural map of the top of the TPP foundation with areas of sediments development (A) at a depth of more than 5000 m, (B) more than 7000 m.
Analysis of sedimentation rates within the depressions of the Timan-Pechora sedimentary basin (ancient platform) shows a change from 15–30 m/million years in the gas-bearing Verkhnepechorskaya and Denisovskaya depressions, up to 60–70 m/million years in the oil-bearing Khoreyverskaya.
The study and development of the Arctic shelf, at least in areas remote from the coast, is postponed for a long period, probably beyond the middle of the current century, and therefore one can hardly expect much interest in their study in the next 30 years, despite the huge potential of deep horizons and their widespread development in provinces such as the Barents Sea, North Kara, Laptev Sea and East Siberian Sea (Dvoynikov and Leusheva E.L. 2022). At the same time, the deep-submerged horizons of the water extensions of the Timan-Pechora, West Siberian and Pre-Caspian provinces will certainly be the subject of close study, especially when substantiating the prospects of oil-bearing capacity or the possibility of identifying condensate-enriched gas deposits.
Today deep horizons of some sedimentary basins (Ajdukiewicz et al., 2010; Gu et al., 2019; Huang et al., 2016; Lee and Geological Survey (U.S.), 1985; Li et al., 2021; Liu et al., 2012; Liu et al., 2015; Li et al., 2020; Adriano et al., 2022; Smith, 2018; Wang et al., 2014; Wei et al., 2019; Yang et al., 2021; Zhang et al., 2015; Zhu et al., 2021) are considered as the main directions of geological exploration for oil and gas within their limits.
From the point of view of the tectonic position, it should be noted that in the Russian Federation there are several internal basins very similar to the Tarim and Dzungarian depressions of China, where deep-submerged sediments with identified accumulations of oil and gas are widely developed (Figure 4). There are known works comparing the geological structure of the depressions of China with the Zaisan, Zee-Bureinskaya and other depressions of the east of the Russian Federation. The problem is that in these depressions, even judging by relatively outdated seismic surveys, a large thickness of the sedimentary cover and, accordingly, a wide development of deep horizons are not expected. In this direction, it is possible to compare and consider, first of all, the prospects of such depressions as the Amur.
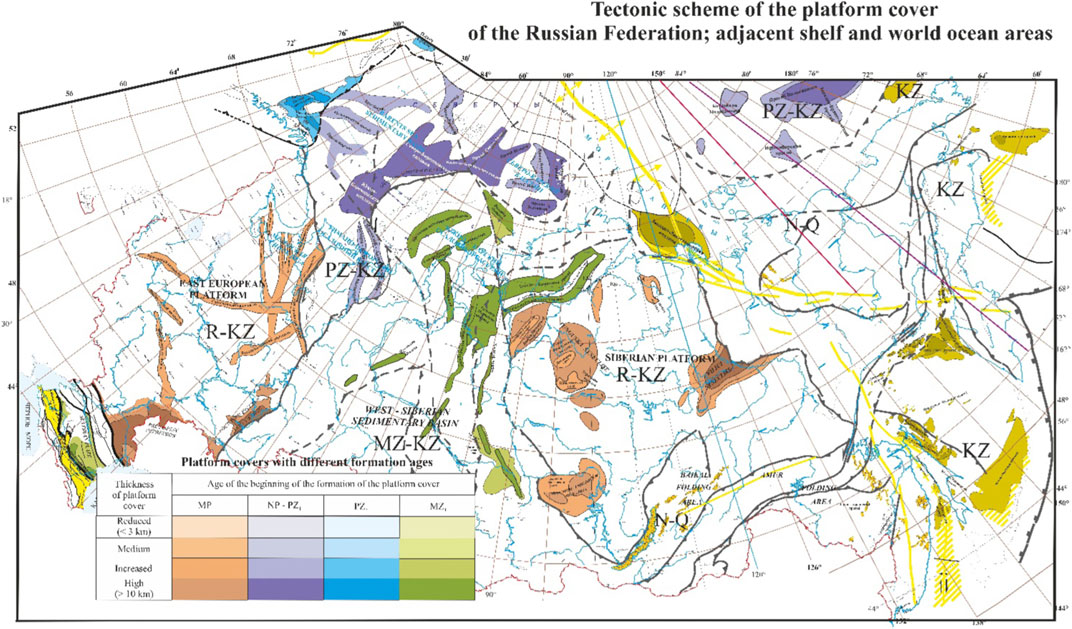
FIGURE 4. Zones of paleorifts and piedmont deflections with deep and ultra-deep sediment depths (Egorov et al, 2021).
To develop criteria for the prospects of deep-submerged horizons, it is necessary to solve a number of theoretical issues, among the priority of which it is necessary to include such as:
- Conditions of formation (mechanisms of accumulation) and preservation of oil deposits at deep depths;
- Conditions of formation and preservation of reservoir rocks;
- Forms of migration and evolution (epigenetic processes) of hydrocarbons at deep depths;
- Geochemical conditions of formation, accumulation and preservation of hydrocarbons at deep depths.
The practice of geological exploration in the world and especially in China has proved the existence of liquid hydrocarbons in deep and ultra-deep horizons, including at high temperature and high pressure (Meihejef, 1993). Oil reservoirs preserved at temperatures above 200°C were found in the deep horizons of the Gulf of Mexico basin (Wang, 1992). In recent years, significant practical results have been achieved in the study of deep and ultra-deep horizons within the superimposed basins of inner China (Sun et al., 2013; Jia and Pang, 2015). Commercial inflows of oil and gas from reservoirs at depths of more than 7,000 m have been identified in the Sichuan, Tarim and Dzungarian basins. The most impressive results were obtained when studying ultra-deep horizons in the Shunbei region of the Tarim basin (Gu et al., 2019), where temperature and pressure were recognized as the most important factors affecting the conditions of formation, accumulation and preservation of hydrocarbons (Hao et al., 2002; Ren et al., 2008; Zhao et al., 2011; Liu et al., 2012; Zhang et al., 2015). However, for a wider range of sedimentary basins, including the Russian Federation, the influence of temperatures and thermal evolution on the phase state of hydrocarbons and the conditions of formation in deep and ultra-deep horizons has yet to be clarified.
The analysis of the revealed mechanisms of accumulation and conservation of hydrocarbons in deep and ultra-deep horizons of sedimentary basins of the world facilitates approaches to the development of a methodology for determining the areas of prospecting and exploration of deep-lying deposits.
At the regional level, in order to determine the prospects for deep and ultra-deep horizons, along with traditional basin analysis, the study of the history of immersion and the nature of temperature changes, an analysis and the correlation of paleotemperature with pressure should be carried out (Kosenkova et al., 2022; Petrakov et al., 2022; Zakharov et al., 2022).
2 Dependence of the phase composition of hydrocarbons on the history of immersion and the influence of temperatures
Temperature is the most important factor determining the evolution of organic matter, the formation (generation) of hydrocarbons and the distribution of their phase state after accumulation (Wassoevich, 1967; Kontorovich et al., 2021).
With an increase in the depth of burial and temperature, the maturity of rocks and organic matter increases systematically.
According to researches (Wassoevich, 1967; Neruchev et al., 1976; Tissot and Welte, 1984; Kontorovich et al., 1991; Peters et al., 1994; Kontorovich et al., 1998; Bazhenova et al., 2008; Dakhnova et al., 2011; Jarvie, 2012; Kontorovich et al., 2021) the boundary temperature conditions were determined, as well as the pressures at which liquid and gaseous hydrocarbons are formed. At the same time, the actual depths of their formation were determined by the temperature gradient determined by the thermal conductivity of rocks and the geothermal background, and, no less importantly, by the type of organic matter (sapropel, humus and mixed types according to researchers of the “Soviet” geochemical school or types I-II or III according to Peters et al., 1994). Thus, in the well–known work of N.B. Wassoevich in 1967, two scales are given - minimum and maximum depths (Figure 5) (the second is often not mentioned by representatives of the school of abiogenic origin of oil) from 2 to 8 km and gas formation from 4 to 12 km or more. Accordingly, without idealizing the tools and methods that were in the arsenal of researchers more than 50 years ago, it is necessary to note the great intuition and foresight of researchers who state, mainly, the possibility of reaching the stage of highly mature and super-mature OM (dry gas formation) at deep and ultra-deep depths, but do not deny the possibility, under certain temperature conditions and for of a certain type of OM, preservation of residual oil and condensate formation at these depths during full-scale gas formation.
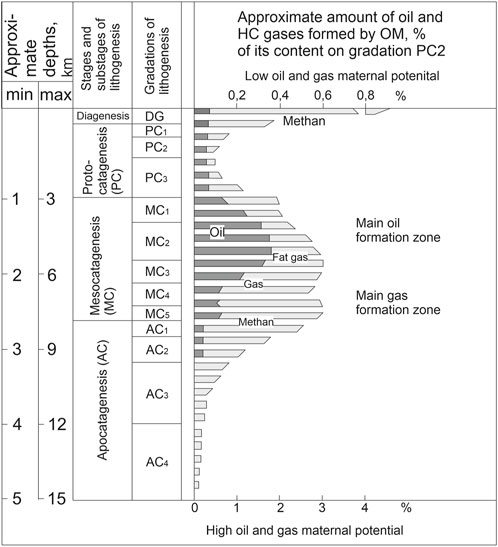
FIGURE 5. Depth intervals corresponding to the main zone of oil and gas formation (Wassoevich, 1967).
According to the sedimentary migration theory (SMT), the “temperature window” of the formation of liquid hydrocarbons varies in the range of 60–90—120°C–150°C, the degree of transformation of OM, determined by the reflectivity of vitrinite (Ro) varies in the range of 0.60%–1.35% (Tissot and Welte, 1984) and according to the stage of catagenesis (used in the Russian Federation) is in the zone of mesocatagenesis of stages MK1-MK3. At the same time, most of the identified oil accumulations in the world are located in the temperature range of 65.5°C–149.0°C (Pusey, 1973). According to the SMT, hydrocarbons should not exist in a liquid state, even despite differences in the type of organic matter, in formations with temperatures above 150°C, i.e., at a standard temperature gradient at depths over 5 km!! Below this mark, liquid hydrocarbons should be subjected to cracking—thermal decomposition with the formation of hydrocarbon gases, hydrogen, and carbon dioxide and sulfur compounds. And when temperatures reach 200°C (on average at a depth of more than 6 km), liquid hydrocarbons should completely decompose (Lu et al., 2002). At the same time, the lower boundary of the main gas formation zone is determined by depths of 6–8 km (or up to 9 km according to N.B. Wassoevich), i.e., in the temperature range up to 200°C–250°C.
However, some new geological discoveries that were made recently make it necessary to be more careful about determining the maximum temperatures and depths of oil and gas formation. For example, liquid hydrocarbons have been found in reservoirs with temperatures exceeding 200°C in China in the Wumishan formation in the Jizhong Basin in the Bohai Gulf Basin, in the United States at the Puckett field in the Valorville Basin and the Gomez field in the Delaware Basin, at the Marun field in the Persian Gulf (Ren et al., 2020). The temperature in the productive horizon of the Marun oil field exceeded 230°C (Sun et al., 2013). The temperature range of the distribution of liquid hydrocarbons in deep and ultra-deep horizons is very different from the temperatures considered by the classical SMT, as evidenced by the existence of liquid hydrocarbons at a very high temperature. The results of the studies show that traces of oil saturation with liquid hydrocarbons were detected in formations with a depth of 7,544–9,600 m, with catagenesis estimated higher than AK2-3, Ro reaching 3.0% and a reservoir temperature of 200°C–250°C (Price, 1993).
Statistics on Chinese basins over the past 40 years show a steady increase in depths estimated as the lower limit of the existence of oil deposits, and sometimes even faster than theoretical assumptions (Kosenkova et al., 2022) (Figure 6).
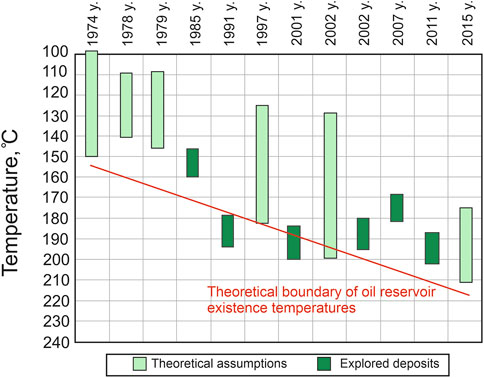
FIGURE 6. Prerequisites for the reassessment of the temperature of oil destruction and the discovery of deep deposits (Zou et al., 2014; Kosenkova et al., 2022).
Thus, the solution to the question of the prospects of oil and gas potential of deep horizons lies in the field of explaining the established fact of a significant difference in the temperature of completion of oil and gas formation, varying in different basins and regions, depending on the time (speed) of heating, pressure, types of OM and other factors.
Some works (Kosenkova et al., 2022) note important differences in the conditions of formation of oil and gas deposits and the factors of their control between basins developed on ancient and young platforms at deep and ultra-deep depths. On ancient platforms, the boundaries of the distribution of liquid hydrocarbons are much higher in section and in intervals with lower temperatures than in the basins of young platforms or Mesozoic-Cenozoic folded and transitional regions. In the latter, oil deposits can sink to a depth of 7 km. The zone of accumulation of exclusively methane deposits in young basins has not yet been reached; it is assumed that it can be developed at a depth of 9–10 km (Gu et al., 2019; Kosenkova et al., 2022). At the same time, the marginal parts of the ancient platforms in the area of articulation with folded systems, due to the high rates of deflection and immersion in the orogenic stage of development, differ significantly in the paleotemperature conditions of deep depths, which is observed almost along the entire length of the Pre-Ural marginal deflection framing the Eastern European platform in three independent sedimentary basins at once (Timan-Pechora, Volga-Ural and the Pre-Caspian region).
The temperature and depth of completion of oil formation vary greatly in different types of basins (Figure 6).
2.1 The influence of paleotemperature on the phase state of hydrocarbons in ancient basins
At deep depths of the basins of ancient platforms, a significant number of deposits of liquid hydrocarbons—oil and\or gas condensate—have been revealed. Such accumulations are established, first of all, in zones of extremely low temperature gradients determined by geological conditions, the first of which include the presence of salts, late and rapid deflection, where Paleozoic sediments were at low temperatures for a long time.
Such areas include the Pre-Caspian bowl, where the geothermal gradient is only 16°C/km (Gulieyu et al., 2017; Khafizov et al., 2020), and the lower boundary of oil formation drops to a depth of 8–9 km, the Pre-Ural deflection of the marginal part of the Pechora Plate (the geothermal gradient is only 14°C–17°C/km. In the Tarim basin of China, the reservoir temperature at a depth of 8,000 m is only 160°C–170°C, and oil detected at a depth of more than 7 km (Liu et al., 2015; Huang et al., 2016; Ma, 2016) (7,200–7,863 m) in the Ordovician formation in the Shunbei region has no signs of degradation (Sun et al., 2013) (Figure 7). Ordovician sediments in the Shunbei area of the Tarim basin have been in low temperature conditions for a long time, which was crucial for the preservation of the light part of liquid hydrocarbons.
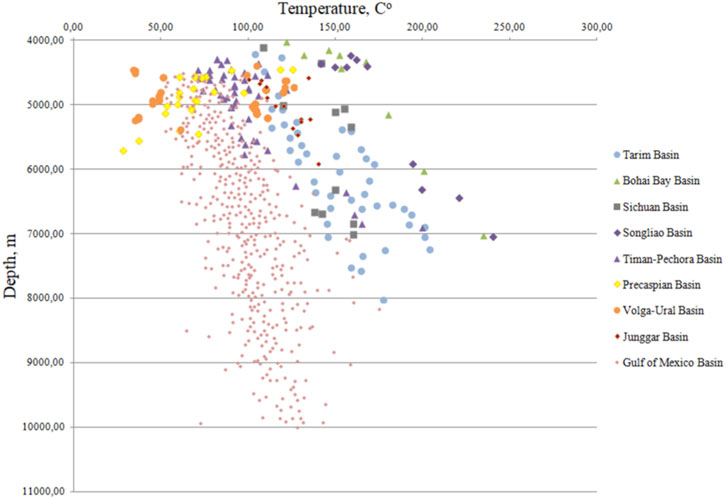
FIGURE 7. The dependence of temperature on depth in the oil and gas basins of the world, opened by lls at deep depths (Wang et al, 2014; Ma, 2016, Liu et al, 2017, HPHT 2020).
In the Dzungarian basin, a fairly low geothermal gradient is observed in a number of wells in the central part of the basin (2.0°C–2.3°C/100 m), and accordingly, conditions for the formation of oil have been preserved at depths up to 6 km, and condensate at deeper depths. Thus, in the work (Prischepa et al., 2022), a pattern of decreasing the density of crude oil with depth was revealed (at a depth of <3,100 m—average density oil 0.84–0.9 g/cm3, at a depth of 3,100–3,900 m—light oil 0.8–0.82 g/cm3, and below 4,400 m—mainly condensate. An important pattern is noted in the distribution of gas in the central part of the Dzungarian basin. Thus, the methane content varies from 60%–95% with a decrease in it at deep depths (below 4,500 m <80%). The content of ethane and propane, on the contrary, gradually increases with increasing depth (Prischepa et al., 2022). The dryness coefficient of natural gas in the study area is 0.7–1.0 and it gradually decreases with increasing depth.
On the other hand, along with geochemical conditions, an extremely important positive factor for hydrocarbons of deep depths is a significant expansion of the reservoir conservation depth range in conditions of a low geothermal gradient.
In one of the most representative (in terms of the number of wells) that have opened deep and ultra-deep horizons, in the sedimentary basin of the Gulf of Mexico (HPHT, 2020; Iskaziev et al., 2021) out of more than 30,000 wells drilled in the period of 2000–2016. 31 wells reached a depth of 9,000 m and more than 60 wells reached a depth of 8,000 m (the total of the sea and rocks, with most of these wells drilled at a sea depth of more than 3,000 m) (Figure 3). For wells with a depth of more than 6,000 m, the average geothermal gradient differs significantly in wells drilled in a zone of relatively shallow sea depths (up to 3,000 m), but with a small proportion of salts in the section or their absence from 24°C to 26°C/km to 12°C–12.8°C/km for wells drilled at a deeper depth of the sea (more than 3,000 m) and with a significant development of salts in the section. Very low downhole temperatures were detected in the deepest 8 wells (9,700–10,000 m) from 93°C to 138°C, which indicates the conditions for finding such horizons in the conditions of the completion of the “oil window”. One of the important factors determining the preservation of oil from cracking at high temperatures is pressure. The deep horizons of the Gulf of Mexico basin are characterized by abnormally high reservoir pressures with a coefficient of 1.6–2.0 (Figure 8).
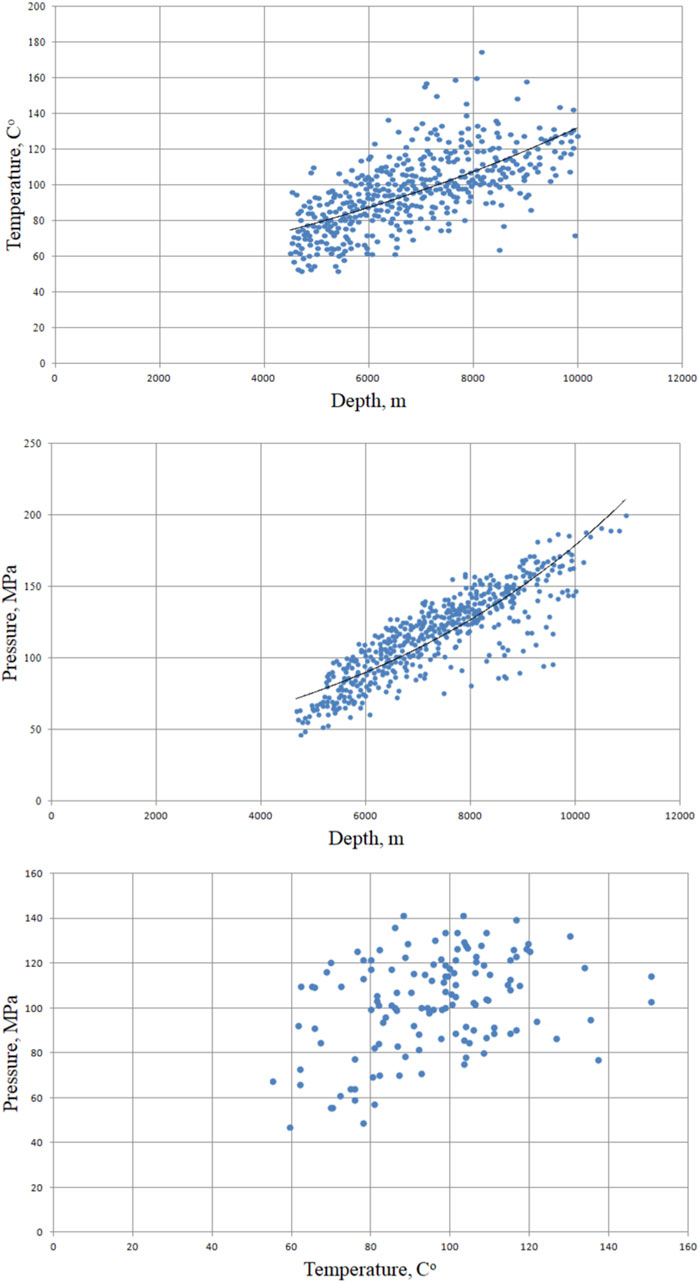
FIGURE 8. Thermodynamic conditions at deep depth in wells of the Gulf of Mexico. Based on materials (HPHT, 2020).
For Paleozoic basins, such as the Pre-Caspian bowl, a low geothermal gradient (1.2–2.2 C/100 m) determined the reservoir temperature no more than 200°C even for the deepest horizons (14–16 km), which can be considered as the main factor in the possibility of the existence of deep-lying oil and gas deposits in the basin in the Paleozoic and possibly in the Upper Proterozoic deposits. The key factor is not the achievement of temperatures at which crude oil is completely cracked, and the oil and gas producing strata remain in the range of oil generation.
2.2 Influence of paleotemperature on the phase state of hydrocarbons in young basins
For the basins of young platforms, it was found that under conditions of rapid temperature increase and even with a high geothermal gradient, deposits of crude oil and gas condensate are formed and preserved at deep depth. For example, in the basin of the Bohai Bay, which belongs to the Cenozoic basins, where a high geothermal gradient is established, rapid deflection and, accordingly, rapid temperature rise in the late Cenozoic led to thermal transformation of the source rocks, causing a rapid transition from oil formation to gas formation. Thus, in the basin of the Bohai Bay, a condensate-saturated reservoir was detected at a depth of 5,641–6,027 m with a reservoir temperature of 201°C (Zhao et al., 2011). According to modern data, at a depth below 4,500 m, the reflectivity coefficient of vitrinite Ro exceeded 1.5%, which indicates the stage of intense gas formation. At a depth of 5,141 m, the temperature reached 180°C, but due to the short duration of heating—less than 10 million years (Zhou et al., 2017), the condensate did not degrade.
The cracking of crude oil is controlled by the properties of crude oil and the history of thermal evolution. Experiments show that the boundaries of the beginning of oil degradation differ greatly in temperature. So, if under standard conditions this occurs in the range from 165°C to 190°C, then under certain conditions (extremely high pressure) oil is preserved at higher temperatures in the range from 230°C to 240°C (Sun et al., 2013; Liu et al., 2017). Under the condition of rapid burial and low temperature due to the low geothermal gradient in the Cenozoic basins, the depth of oil formation is in a very wide range, with a maximum of more than 8,000 m or even more than 10,000 m (Sun et al., 2013; Liu et al., 2017; Ren et al., 2020).
For intermountain basins and foothill depressions, such as the southern part of the Pre-Caspian basin, the Panon basin, due to rapid immersion and short heating time (<10–15 million years), the temperature of the “oil window” is significantly higher than in other basins at comparable depths.
For Mesozoic basins (for example, the Barents Sea, West Siberian) with a low rate of deflection in the Cenozoic, or subjected to uplift and denudation since the Cenozoic, the effective heating time is stretched, and the temperature of the “oil window” and “gas window” decreases, and the depth of the location of oil and gas deposits is extremely limited.
2.3 Influence of heating time on the phase state of hydrocarbons
The heating time also has an important influence on the temperature range and phase state of hydrocarbons. Temperature and heating time are the key reasons for the difference in temperature ranges of phase states of hydrocarbons in different basins. In the case of a short heating time, the oil is stored at a very high temperature. Liquid hydrocarbons can be stored at a maximum depth of up to 8,000 m and reservoir temperature >200°C; gas deposits—at a maximum depth of 10,000–12000 m and reservoir temperature up to 300°C–350°C (Tuo, 2002). Oil in a basin with a short heating time and a temperature in the range from 300°C to 315°C was discovered in a deep ocean basin in the northeast Pacific Ocean (Wang, 1992).
For basins with a high deflection rate in the Cenozoic, the effective heating time is very short, and crude oil can exist at very high temperatures. For example, in China, condensate gas deposits were found in the Bohai Bay basin at a temperature of 201°C at a depth of 6,027 m (Sun et al., 2013); liquid hydrocarbons were detected at a depth of 5,011 m in the Inghehai Basin at a reservoir temperature of 240°C and Ro of only 1.20% (Hao et al., 2002). With the same rate of increase in reservoir temperature, the temperature of the initial cracking of oil varies greatly from region to region, and the temperature of the complete cracking of oil also differs slightly.
As a rule, the temperature at which the liquid phase undergoes thermal cracking is at least 170°C, and the thermal stability largely depends on the composition of the crude oil, in addition to the temperature and heating time. The temperature of the formation of the oil phase is 178°C–214°C (Liu et al., 2017).
The heating rate also has an obvious effect on the temperature range of the liquid phase. An increase in the heating rate from 1°C/Ma to 10°C/Ma can increase the temperature of the independent oil phase by 17°C–27°C. With an increase in the heating rate in conditions of deep depth and high temperature, hydrocarbons can exist in the oil phase, and the temperature limit for storing condensate in a supercritical state is about 240°C (Kerimov and Osipov, 2016; Liu et al., 2017; Kerimov et al., 2018; Ren et al., 2020).
3 The relationship between pressure and temperature
An important role in the control of the phase state of hydrocarbons and the distribution of oil and gas reserves depending on the ratio of temperature and pressure is revealed. These two factors are closely related and interact with each other.
According to data analysis, the correlation between temperature and pressure at deep depths is complex and varies significantly in different types of basins and even in different structural units of the same basin. This ratio can be divided into three types: the type of high temperature and high pressure, the type of low average temperature and high pressure, and the type of medium temperature and low-medium pressure (Ren et al., 2020) (Figure 9).
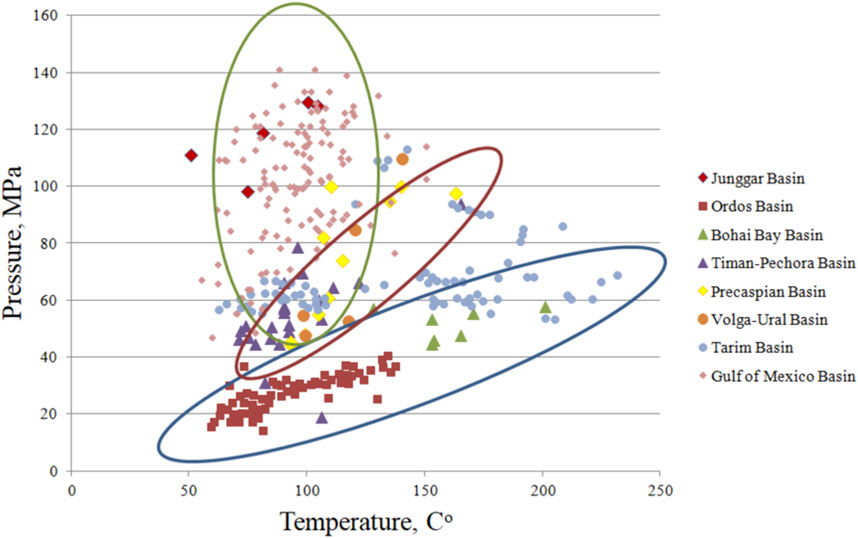
FIGURE 9. Correlation between reservoir temperature and reservoir pressure in deep oil and gas deposits.
3.1 Basin type with high temperature and high pressure
High reservoir temperatures (>150°C) and high reservoir pressures (>60 MPa) in deep and ultra-deep depths are the most typical and characteristic of both ancient and young basins. According to classical concepts, the degree of transformation of organic matter should be extremely high and oil can only be located at a relatively shallow depth. But high pressure prevents the conversion of hydrocarbons into oil and gas producing strata, which is why the temperature does not reach the threshold value of cracking crude oil. (Gu et al., 2019). At the same time, another extremely important positive moment was revealed, associated with the possibility of formation of fracture zones in such conditions, as well as the formation of an additional capacity due to a large driving force due to pressure drop during migration from the oil and gas producing strata. Such examples are known in the Timan-Pechora, Pre-Caspian and Lena-Tunguska basins (Cherdantsev and Zharkov, 2021; Zhukov and Kuzmin, 2021; Prischepa et al., 2022).
The influence of abnormally high pressure plays an important role in controlling the thermal evolution of deep-lying oil and gas source formations. Some researchers as Price and Wenger (1992) believe that an increase in pressure can prevent the thermal release of organic matter and the formation of oil and gas. At the same time, the correlation between the reflectivity of vitrinite and the depth in the overpressure range (2,400–4,500 m) in the northern part of the Green River basin in the United States did not reveal any significant effect of overpressure on the reflectivity of vitrinite (Law, 2002). The study of Domanik deposits of the TPP (Prischepa et al., 2013) showed, with large pressure variations, significant variations in determining the reflectivity of vitrinite and the degree of transformation of organic matter in neighboring wells and even within the section of one well, depending more on the lithological composition. Excessive pressure prevents thermal degradation and formation of hydrocarbons from kerogens, as well as high-temperature cracking of hydrocarbons (Helgeson et al., 2009; Gu et al., 2019).
In sedimentary basins with a low geothermal gradient and a deep depth of occurrence of oil and gas producing strata, high pressure affects the thermal evolution and the degree of transformation. Thus, in the study (Gu et al., 2019), Ro was observed to be more than 0.5% lower than the normal value. High pressure helps to prolong the formation time of liquid hydrocarbons in the strata at deep and ultra-deep depths and prevents the conversion of liquid hydrocarbons into gaseous hydrocarbons.
The presence of excessive pressure leads to the fact that even deep-lying oil and gas producing strata (hereinafter referred to as “OGPS”) that have entered the stage of metamorphism in a sedimentary basin with a high geothermal gradient remain in a favorable stage of hydrocarbon formation and accumulation of oil and gas. At the Lake Washington oil field in the Gulf of Mexico basin, tertiary formations with a depth of more than 6,540 m have a reservoir temperature of more than 200°C, but hydrocarbons remain in the liquid phase due to abnormally high pressure (130 MPa) (Ajdukiewicz et al., 2010).
Typical examples are the Shunbei, Tabei, and Anyue gas fields in the Tarim Basin. In the Shunbei region, for deep-lying (more than 8,000 m) productive sediments of the Ordovician, the geothermal gradient is 2.12°C/100 m, the reservoir temperature is 160°C–170°C, and the reservoir pressure is more than 80 MPa. At the Anyue gas field, the gas reservoir of the Lunwangmiao formation at a depth of 4,600–5,400 m is characterized by a reservoir temperature of 140°C–161°C, a reservoir pressure of 56–59 MPa and a pressure anomaly coefficient of 1.06–1.65 (Wang et al., 2014).
3.2 Basin type with low temperature and abnormally high pressure
In the most common case, both temperature and pressure are a function of the depth of occurrence and, accordingly, at deep and ultra-deep depths, high pressures must correspond to high temperatures. The causes of temperature anomalies were discussed above, mainly in the direction of their lowered values, caused by both a reduced geothermal background and a “cooling” effect in the case of the presence of salts in the section, a powerful layer of overlapping water in the water areas and zones of tectonic disturbances. Accordingly, in such areas, a lower temperature will be shown relatively to the “standard” at the appropriate depths. A feature of sedimentary basins with oil deposits at deep depths is often abnormally high pressures with an anomaly coefficient of 1.5 or more.
This type of basins (structures) is characterized by a low reservoir temperature (usually up to 150°C) and increased reservoir pressure (usually >80 MPa) than most basins at comparable depths of 5,000–8,000 m.
Since pressure can suppress the degree of thermal transformation of hydrocarbons for this type of structures, the generation stages and the possibility of preserving liquid and gaseous hydrocarbons occur later and at deeper depths. Abnormally high pressure can also slow down the compaction of overlying sediments, which contributes to the formation and preservation of deep and ultra-deep zones with high residual porosity (Ren et al., 2020; Volozh et al., 2021, Zakharov et al., 2022). Typical examples are the Keshen gas fields of the Tarim basin, Kelasu and Dabey in the Kukainsky depression of the Dzungarian basin (Lu et al., 2018).
The Kela-Keshen gas field (Tarim basin) is an ultra-deep accumulation in the zone of abnormally high pressures at a depth of 6,500–8,000 m, with a reservoir pressure of 90–136 MPa, an anomaly coefficient of 1.60–1.85 and a low reservoir temperature of less than 150°C. The same is noted for the neighboring Keshen 2 block, where the initial reservoir pressure was 116 MPa, the pressure anomaly coefficient is 1.79, and the reservoir temperature is 168°C. The Keshen gas field was formed due to the Jurassic coal-bearing gas-producing strata of the Baicheng depression. The maturity coefficient of natural gas at the gas field is 2.28%–3.68%, on average 3.10%, which indicates the overripe nature of natural gas.
Similar thermobaric conditions were found in the central part of the Dzungarian basin located to the north. So, at depths from 4,600 to 5,900 m, the reservoir pressure was 67–115 MPa, the anomaly coefficient was 1.45–2.04, and the reservoir temperatures varied in the range of 100°C–140°C, i.e., they were low enough for such depths. The reservoir, according to the researchers, was formed under the influence of rapid burial and rapid formation of hydrocarbons, as well as a significant difference in pressure in oil and gas producing strata and reservoirs with a correspondingly strong driving force of migration and accumulation of hydrocarbons (Wei et al., 2019).
3.3 Medium temperature and low pressure type
Sedimentary basins with low pressure (<50 MPa) and moderate temperature at deep depths (<150°C) are specific, first of all, for ancient platforms or their marginal parts, but are also noted on young platforms. They have extremely limited opportunities for vertical and lateral redistribution of hydrocarbons due to the low pressure drop. The most favorable conditions for the preservation of oil and gas accumulations at deep depths are provided by relatively young uplift and erosion. In such conditions, a significant potential is associated with the oil and gas producing strata from which previously generated hydrocarbons did not migrate. Typical examples are the Lena-Tunguska OGP of the Siberian Platform with a large burial depth in the Proterozoic and Paleozoic periods and a relatively small depth at present, limited areas of the northern part of the West Siberian OGP, and, for example, the Ordos basin in China. The Ordos basin was subjected to severe uplift and erosion, which let the residual potential of the OGPS to be preserved.
Despite the similarity of temperature–pressure relations, when comparing the sedimentary basins of the Siberian Platform and the Ordos basin, they differ significantly in the hydrocarbon potential of the deep-lying horizons. Thus, in the Lena-Tunguska OGP, the widely developed phenomena of uplift and erosion in the period after the Early Ordovician, when the basin reached the maximum depth of immersion and maximum paleotemperature, which allowed the formation of hydrocarbons from black shales of the Riphean and Vendian age, led mainly to unsatisfactory conditions for the preservation of deposits at deep depths, since the ancient (Late Riphean-Vendian) reservoirs were violated (Bochkarev and Krinochkin, 1988). At the same time, due to the low geothermal gradient, the ancient OGPS were still at the stage of oil formation at the time of the beginning of the inversion. Later, when the basin experienced slow uplift and was largely eroded, the reservoir temperature decreased. A positive factor for deep horizons was the widespread distribution of salts in the lower Cambrian of the East Siberian Platform, which in this case did not work as a subsurface cooling system, but created good conditions for the preservation of ancient oil and gas deposits, which is confirmed by numerous discoveries at moderate depths and are assumed to be within the entire Riphean-Vendian (Precambrian) part section (Bochkarev and Krinochkin, 1988; Qiu et al., 2018) (Figure 10).
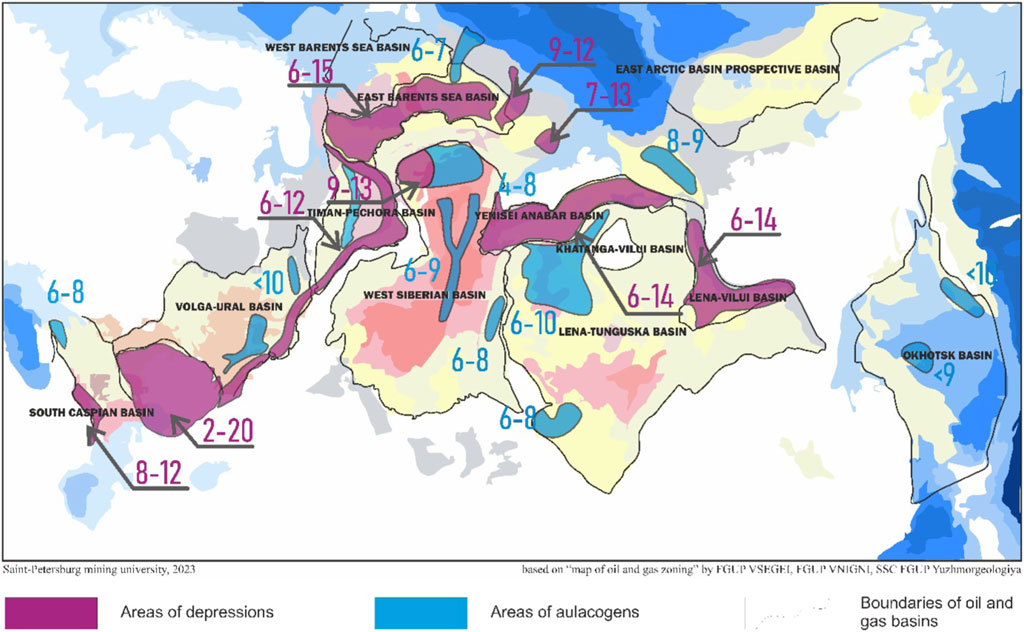
FIGURE 10. Schematic distribution of deep-lying sedimentary complexes in oil and gas basins of the Russian Federation.
Inversion movements and the influence of erosion processes are noted as important factors that cause the formation of reservoirs in ancient (Paleozoic) strata filled with hydrocarbons before their immersion to deep depth in subsequent historical periods. The possibility of preserving deposits is provided, as already discussed earlier, by a high rate of immersion and high pressures that do not let cracking to begin. Thus, the influence of erosion processes with an emphasis on carbonate rocks is noted for many formations and sedimentary basins where oil deposits have been identified at deep depths. The influence of stratigraphic inconsistencies on the improvement of reservoir properties as a result of leaching by meteoric waters is noted in works.
Discussing the criteria of oil and gas potential along with the effect of “cooling” of the depths, due to the presence of conducting (through) faults, it is impossible not to say about their role in the formation and distribution of reservoirs. Thus, at deep depths, control of the distribution of oil-saturated reservoirs by faults is noted, which is used as a search sign (Figure 10). Based on the analysis of the factors of hydrocarbon accumulation in the deep reservoirs of the oil and gas fields of the Dzungarian basin (Shiksi, Mobei, and Mosuovanan), it was concluded that the oil and gas source rocks were connected by faults with the reservoirs (Prischepa et al., 2022), which let hydrocarbons to migrate. Consequently, faults not only contribute to the formation of fractures at deep depths (Prischepa et al., 2022), but also to the flow of oil and gas through them.
Conditions for the formation of reservoirs (areas with improved FCS) at deep depths are an important issue along with the formation and preservation of hydrocarbons (Kosenkova et al., 2022; Kuandykov et al., 2022). A major generalization on the distribution of porosity and permeability at deep depths was made in Ehrenberg’s work, in which an extensive database was statistically processed, including more than 35,000 values for terrigenous sections and 13,300 for carbonates (Ehrenberg and Nadeau, 2005). A decrease in porosity with depth was traced in the range up to 3,500–4,500 m, after which a “flattening” of the trend is observed, which allows us extrapolating the values of absolute porosity to more than 5,000–6,000 m at the level of 8% for carbonate reservoirs and 10% for terrigenous ones. Due to the significantly greater chemical activity of carbonate reservoirs, epigenetic processes during immersion pay great attention to them. It is noted that for FCS, the influence of reservoir temperatures (and paleotemperature) is much more important than the absolute values of the depths of occurrence (Kuandykov et al., 2022).
A significant addition to understanding the prospects of deep horizons is the direct study of the geochemistry of oil and the comparison of parameters with the geochemistry of extracted bitumen core samples. Thus, the use of the isomerization parameters of C29 sterane for deep oil and shallow oil deposits makes it possible to determine the similarity of the source of oil and gas formation, and the ratio of βß sterane C29/(βß+αα) characterizes the depth, since it decreases with increasing depth. This is probably influenced by the degree of thermal evolution, which increases with increasing thermal maturity and, according to (Osipov, 2021), significantly affects the migration of hydrocarbons. During the migration of oil and gas, the ratio of βß sterane C29/(βß+αα) increases with the distance of migration. Thus, the ratio of βß sterane C29/(βß+αα) is gradually increasing from deep-lying to shallow oil and indicates oil migration controlled by faults and distance.
Based on the analysis and the proposed classification, a sequence (methodology) for studying deep and ultra-deep horizons of sedimentary basins of the Russian Federation, including two main blocks, has been developed (Figure 11):
1. Study of thermodynamic conditions (temperature, pressure) and assessment of their influence on the possibility of formation and preservation of hydrocarbon accumulations at deep depths.
2. Assessment of geochemical conditions for the formation and preservation of hydrocarbon accumulations at deep depths.
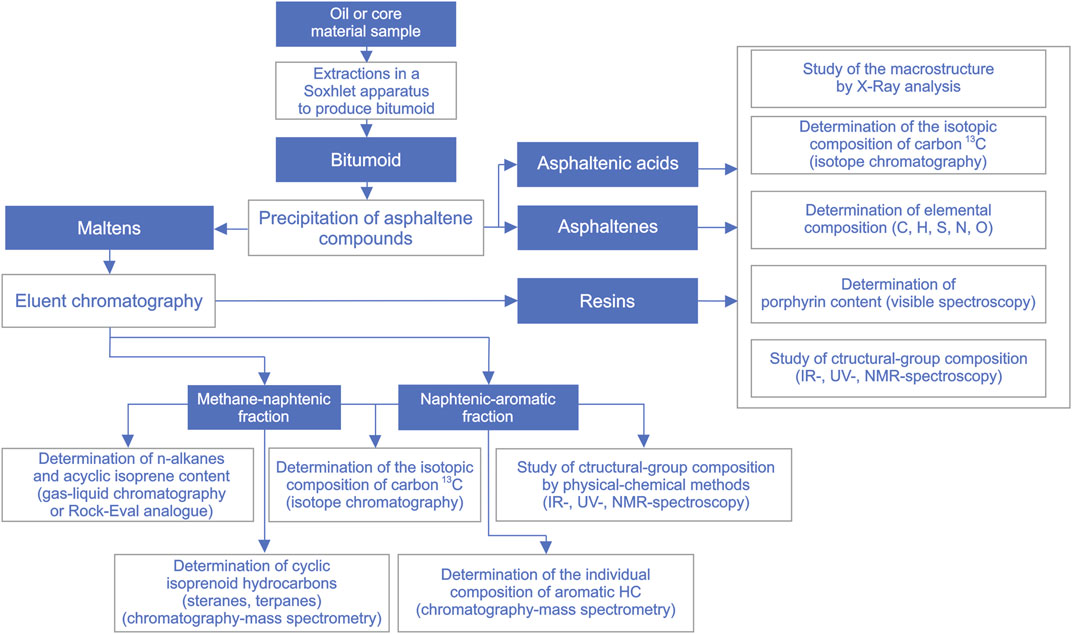
FIGURE 11. Schematic diagram of geochemical analysis of oil and core material to assess the potential of deep horizons.
The first block, in turn, includes the study of:
- Geodynamic characteristics of the sedimentary basin (including tectonic conditions for the formation of underlying sedimentary cover levels);
- Sedimentary basin immersion history (immersion rates and sedimentation rates);
- The nature of the temperature distribution and geothermal gradient with an emphasis on deep depths;
- Paleotemperature history (time of exposure to temperatures);
- Lithological composition of rocks, distribution of depths and areas of development of salts and salt-containing strata;
- Conducting or screening properties of tectonic disturbances penetrating to deep depths;
- Studying the possibility of preserving the primary properties of reservoirs (porosity) at deep depths;
- Studying the time and scale of formation and preservation of fracturing.
The second block of research includes a number of sequential procedures containing clarification of the features of the geological structure of the thickness section intervals that can be diagnosed as oil and gas-producing, the study of geochemical features and biomarkers of oil and gases, oil-saturated reservoirs, bitumoids and kerogen of the core of wells from deep depths and the study of reservoir properties of hydrocarbon-containing strata.
Such sequential steps include:
1. Identification of characteristic features of attribution to high-carbon deposits (study of TOC content).
2. Determination of the stratigraphic interval of the distribution of deposits primarily enriched with organic matter, referred to as OGPS.
3. Determination of lithological composition (microscopic and petrographic studies of sections) and lithotyping of sediments.
4. Mapping of the current concentration of organic carbon and catagenetic maturity of organic matter by bituminological and pyrolytic studies.
5. Assessment of geochemical parameters (laboratory study of extracts of bitumoids and pyrolytic studies of core samples), distribution and degree of transformation of organic matter of high-carbon deposits, their association with different lithotypes and zones differ in facies conditions of formation.
6. Assessment of thermodynamic conditions. Isolation of temperature parameters and pressures of zones of HC manifestation and preservation of different composition.
7. Geochemical characteristics of oil and bitumen extracts (analytical studies, including: pyrolysis (Rock-Eval), study of the kinetics of thermal decomposition of kerogen, one-dimensional (GC-MS), two-dimensional (GC × GC-MS) chromatomass spectrometry and isotope mass spectrometry (EA-IRMS).
8. Biomarker analysis with the identification of the oil-extract connection.
9. Assessment of the possibility of preserving the porosity (petrophysical parameters) of the selected OGPS lithotypes.
10. Evaluation of petrophysical properties with fracturing.
11. Recalculation of the organic carbon content in rocks at the beginning of catagenesis using balance equations for three main types of organic matter.
12. Determination of the movable part of bitumoids (separation into residual kerogen and parautachthonous bitumoids) preserved in Domanik oil and gas bearing deposits after migration of generated hydrocarbons.
13. Assessment of oil and gas resources of deep and ultra-deep horizons according to a set of geological and geochemical indicators.
14. Assessment of the possibility of using the developed approach to the deeply submerged horizons of sedimentary basins of the Russian Federation
Geochemical characteristics of oil and bitumen extracts can be obtained by using a complex of modern analytical studies, including: pyrolysis (Rock-Eval), study of the kinetics of thermal decomposition of kerogen, one-dimensional (GC-MS), two-dimensional (GC × GC-MS) chromatomass spectrometry and isotope mass spectrometry (EA-IRMS).
According to the results of pyrolytic studies, the total content of organic carbon (TOC) in rocks can be estimated. The obtained pyrolytic parameters S1, S2, and Tmax are used to estimate the generation potential.
The Russian Federation has store of considerable experience in studying kerogen-saturated sections of such oil-producing strata as the Bazhenov, Domanik, Kuma, and Khadum formations, located at relatively shallow depths and almost unexplored at deep depths. Meanwhile, even fragmentary data indicate a significant and not fully realized potential of these strata at deep depths. Thus, in the Timan-Pechora basin, “cooled” zones with a geothermal gradient of 15°C–18°C/km and a residual TOC for marls and clay limestones of the Upper Devonian from 0.5% to 1% were identified within the junction zone of the marginal part of the platform and the Pre-Ural marginal deflection. According to the results of GIS, intervals of oil-producing, fluid-saturated rocks are being distinguished.
In connection with the development of ideas about events that contribute to the accumulation of carbon-enriched “high-bituminous” horizons, the information about global anoxic events in the World Ocean is important information for their diagnosis. The isotopic composition measured by the values of isotopic densification δ13Ccarb and δ13Corg. Carbonate and organic carbon of sedimentary rocks allows us to diagnose sedimentation conditions and to isolate biotic and facies conditions for the formation of enriched rocks with OM.
The saturation of reservoirs with movable oil (both natural reservoirs and technically stimulated ones) can be determined by high values of pyrolytic parameters S0 + S1, productivity index (PI), oil saturation index (OSI) and reduced Tmax, relative to the average distribution in the section.
According to the results of kinetic studies of thermal decomposition of kerogen, most samples are characterized by a spectrum characteristic of type II kerogen. As the degree of catagenesis increases, the shape of the spectrum changes and the maximum shifts towards higher activation energies. Information about the facies-genetic type of OM and its thermal maturity can be obtained by studying the composition of biomarkers and isotopic composition data. Based on the values of molecular parameters, characteristic biomarker ratios Pr/Ph and C29/C27, conclusions are drawn about the assessment of the conditions for the OM formation of the studied deep sediment bitumoids.
The second block of the proposed methodology assumes the obtained analytical data, which should be compared with the data of thermodynamic modeling to assess the possibility of the existence of individual components of liquid oil, gaseous reservoir fluid and solid kerogen mass under various thermobaric conditions (Shimansky et al., 1994; Kerimov et al., 2018; Kontorovich et al., 2021).
It is most rational to take the interpretation of equilibrium processes described in R. Helgeson’s work as the basis of the thermodynamic model (Helgeson et al., 2009).
The Helgeson model suggests a sequence of biomass conversion processes with a step-by-step transformation of shales containing bitumoids and kerogen, oil and gas, formed, among other things, in areas of high pressures, temperatures and depths.
For further research, it is important that this equilibrium system of equations will also help to describe the processes postulated within the framework of abiogenic concepts, which are characterized by a reversible dependence.
This case implies a reverse pyrolysis process. At the same time, endogenous deep hydrocarbons, with a decrease in temperature and pressure, form the oil and gas familiar to us, successively passing into carbon shales, shungite and graphite during the ascent.
Thus, the proposed method of thermodynamic modeling, based on the basic chemical reactions associated with chemical and physical transformation in an equilibrium system of solid, liquid, gas and further construction of the dependences of the reduced Gibbs energies on temperature and pressure in the reservoir, taking into account the imperfection of the system, will allow us obtaining an array of results that let not only determine the equilibrium composition of the components of this a three-phase system at various depths, but also to confirm or refute the possibility of regressive metamorphism, characterized by the possibility of phase “solidification” of oil in areas of ultra-high pressures or abnormally low temperatures with the formation of pseudocrystal kerogens.
Thermodynamic calculations are based on the assumption that all the transformations described above proceed in accordance with the basic postulates of Hess’s law, Kirchhoff’s law and the second law of thermodynamics.
At the same time, it is possible to represent oil, gas and solid kerogen in the form of chemical compounds of a given stoichiometry, and, consequently, to bring their thermodynamic state functions for further calculations. For example, light and heavy oils can be represented as compounds of the type—(С10Н22), solid kerogens—(С128Н68O7 and C292H288O12), gases (alkanes (CnH2n+2) (Helgeson et al., 2009). The structural formulas of “mature” and “immature” kerogen are shown in Figures 12, 13.
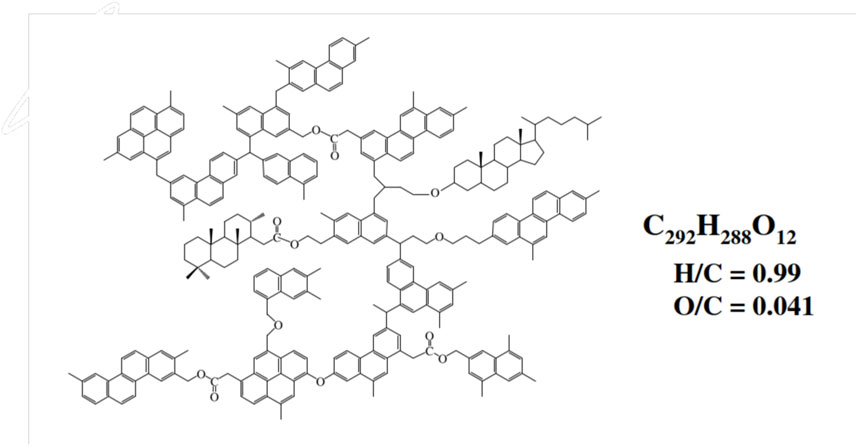
FIGURE 12. Structural formula of “immature” kerogen (Helgeson et al., 2009).
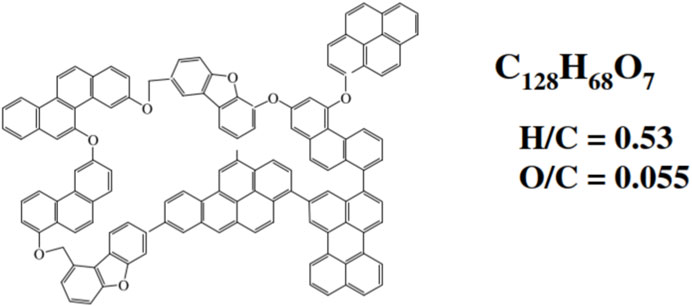
FIGURE 13. Structural formula of “mature” kerogen (Helgeson et al., 2009).
The transformations of sulfur-containing and nitrogen-containing heteroatomic compounds of kerogen will not be considered in the proposed model. This assumption makes the above described system idealized, however, in accordance with the data published in (Helgeson et al., 2009), the possibility of applying such a simplification is shown.
Thus, the equilibrium reactions between the phases can be written as:
Hydrolytic disproportion of kerogen:
Metastable state in the kerogen—water system:
Metastable state in the kerogen—oil—water system:
Metastable state in the kerogen—oil—gas system:
Metastable state in the oil—gas—water system:
Metastable state in the gas—water system:
The obtained isotherms for the equilibrium constants for the proposed reactions are shown in Table 3.
By solving the proposed system of equations for various temperatures and pressures, it is possible to obtain dependences of the quantitative ratio of the components described above on the depth of occurrence, which in turn will allow us predicting the critical boundaries (depth) of the place of possible oil and gas accumulations in atypical geological conditions.
4 Conclusion
1) Deep and ultra-deep horizons of sedimentary basins differ significantly in temperature and paleotemperature conditions, reservoir pressures, the rate of deposition of sediments and the time of exposure to high temperatures from horizons located at medium and shallow depths, where most of the previously discovered hydrocarbon deposits are located (up to 4.5–5 km).
2) Sedimentary basins with widely developed deep and ultra-deep hydrocarbon deposits are fairly confidently typified by their prospects in terms of the ratio of temperature and reservoir pressures, geothermal gradient, presence (or forecast) and richness at great depths of strata, organic matter, and belonging to a certain geodynamic (tectonic) situation.
3) One of the significantly underestimated factors for large depths, and not included in the technology of basin modeling, is the time factor (which refers to both the rate of immersion and the time of exposure to high temperatures and the time of manifestation of peaks in the generation of liquid and gaseous hydrocarbons). The effective heating time significantly affects the phase composition of ultra-deep hydrocarbon accumulations.
4) The phase composition of hydrocarbons is controlled by temperature, heating time, heating rate, pressure and the type of organic matter of the oil and gas producing rock. The possibility of detecting oil deposits at deep and ultra-deep depths of 8–10 km is determined by both a low geothermal gradient and abnormally high pressures. Under the condition of rapid heating in a high-temperature environment, gas condensates may exist. The detection of gas deposits in conditions of progressive cracking of oil or at relatively low temperatures is not limited by ultra-high pressures and is possible up to a depth of 10–12 km.
Data availability statement
The original contributions presented in the study are included in the article/supplementary material, further inquiries can be directed to the corresponding author.
Author contributions
Conception the Research: OP. Methodology: OP, SK, and DL. Design: YN, TK, and NS. Collection and assembly of data: OP, SK, YN, AM, TK, and RX. Data analysis and interpretation: OP, AM, DL, TK, and NS. Writing-original draft: PM. Writing-review and editing: OP, SK, and YN. All authors read and provided significant inputs into all drafts of the editorial, agreed to be accountable for all aspects of the work and approved the final draft of the editorial for publication.
Conflict of interest
The authors declare that the research was conducted in the absence of any commercial or financial relationships that could be construed as a potential conflict of interest.
Publisher’s note
All claims expressed in this article are solely those of the authors and do not necessarily represent those of their affiliated organizations, or those of the publisher, the editors and the reviewers. Any product that may be evaluated in this article, or claim that may be made by its manufacturer, is not guaranteed or endorsed by the publisher.
References
Abukova, L. A., and Volozh, Yu. A. (2021). Geofluidodynamics of deep-submerged zones of oil and gas accumulation of sedimentary basins. Geol. Geophys. 62 (8), 1069–1080. doi:10.15372/gig2021132
Adriano, M. S., Figueiredo, J. P., Coelho, P. H. G. R., and Borghi, L. (2022). Tectonic and stratigraphic evolution of the Santos Basin rift phase: New insights from seismic interpretation on Tupi oil field area. J. S. Am. Earth Sci. 116 (116), 103842. doi:10.1016/j.jsames.2022.103842
Aguilera, R. F., Ripple, R. D., and Aguilera, R. (2014). Link between endowments, economics and environment in conventional and unconventional gas reservoirs. Fuel 126 (3), 224–238. doi:10.1016/j.fuel.2014.02.063
Ajdukiewicz, J. M., Nicholson, P. H., and Esch, W. L. (2010). Prediction of deep reservoir quality using early diagenetic process models in the Jurassic Norphlet Formation, Gulf of Mexico. AAPG Bull. 94 (8), 1189–1227. doi:10.1306/04211009152
Aleksandrova, T., Nikolaeva, N., Afanasova, A., Romashev, A., Aburova, V., and Prokhorova, E. (2023). Extraction of low-dimensional structures of noble and rare metals from carbonaceous ores using low-temperature and energy impacts at succeeding stages of raw material transformation. Minerals 13, 84. doi:10.3390/min13010084
Alekseev, A. D., Zhukov, V. V., Strizhnev, K. V., and Cherevko, S. A. (2017). Research of hard-to-recovery and unconventional oil-bearing formations according to the principle ‘in-situ reservoir fabric. J. Min. Inst. 228, 695–704. doi:10.25515/PMI.2017.6.695
Bazhenova, T. K., Shimansky, V. K., Vasilyeva, V. F., Shapiro, A. I., Gembitskaya, L. A., and Klimova, A. I. (2008). Organic geochemistry of the Timan-Pechora basin. St. Petersburg: VNIGRI.
Belozerov, I. P., and Gubaydullin, M. G. (2020). Concept of technology for determining the permeability and porosity properties of terrigenous reservoirs on a digital rock sample model. J. Min. Inst. 244, 402–407. doi:10.31897/pmi.2020.4.2
Bochkarev, V. S., and Krinochkin, V. G. (1988). “Precambrian and paleozoic formations of western siberia,” in Tectonics of platform areas (Netherlands: Elsevier).
Cao, B., and Bai, G. (2014). “Global distribution of petroleum reserves in deep reservoirs,” in 2014 AAPG Annual Convention and Exhibition At, Houston, Texas, 6-9 April 2014.
Cherdantsev, G. A. A., and Zharkov, A. M. (2021). Prospects for the oil and gas content of the Upper Permian deposits of the southwestern part of the Vilyui syneclise based on the analysis of sedimentary environments and geochemical conditions of oil and gas content. J. Min. Inst. 251, 698–711. doi:10.31897/PMI.2021.5.9
Dakhnova, M. V., Bazhenova, T. K., Lebedev, V. S., and Kiselev, S. M. (2011). Isotopic criteria for predicting the phase composition of hydrocarbons in Riphean and Vendian deposits of the Lena–Tunguska petroleum province. Russ. Geol. Geophys. 52 (8), 945–953. doi:10.1016/j.rgg.2011.07.015
DeCelles, P. G., and Giles, K. A. (1996). Foreland basin systems. Basin Res. 8 (2), 105–123. doi:10.1046/j.1365-2117.1996.01491.x
Dobrjanski, A. F., and Andrejev, P. F. (1954). Thermodynamics of processes of formation and change of oils in nature. Eesti NSV Tead. Akad. Toim. 3 (2), 193–204. doi:10.3176/toimetised.1954.2.03
Dvoynikov, M. V., and Leusheva, E. L. (2022). Modern trends in hydrocarbon resources development. J. Min. Inst. 258, 879–880.
DvoynikovM, V., SidorkinD, I., YurtaevS, L., GrokhotovE, I., and UlyanovD, S. (2022). Drilling of deep and ultra-deep wells for prospecting and exploration of new raw mineral fields. J. Min. Inst. 258, 945–955. doi:10.31897/PMI.2022.55
Eberth, D. A., Brinkman, D. B., Chen, P.-J., Yuan, F.-T., Wu, S.-Z., Li, G., et al. (2001). Sequence stratigraphy, paleoclimate patterns, and vertebrate fossil preservation in jurassic-cretaceous strata of the junggar basin, xinjiang autonomous region, people’s republic of China. Can. J. Earth Sci. 38 (12), 1627–1644. doi:10.1139/e01-067
Egorov, A. S. (2015). Features of the deep structure and composition of the geostructures of the Earth’s crust in the continental part of the Russian territory. Russian: Journal of Mining Institute, Publishing House of the NMSU.
Egorov, A. S., Prischepa, O. M., Nefedov, Y. V., Kontorovich, V. A., and Vinokurov, I. Y. (2021). Deep structure, tectonics and petroleum potential of the western sector of the Russian arctic. J. Mar. Sci. Eng. 9 (3), 258. doi:10.3390/jmse9030258
Ehrenberg, S. N., and Nadeau, P. H. (2005). Sandstone vs. carbonate petroleum reservoirs: A global perspective on porosity-depth and porosity-permeability relationships. AAPG Bull. 89 (4), 435–445. doi:10.1306/11230404071
Ermolkin, V. I., Kočofa, A. G., Sorokova, E. I., and Trunova, M. I. (2008). Phase zonality of hydrocarbons in the ultra-deep horizons of the Earth's crust. Bull. Peoples' Friendsh. Univ. Russ. Ser. Eng. Res. (3), 36–40.
Fomin, A. N. (2011). Catagenesis of organic matter and oil and gas potential of Mesozoic and Paleozoic deposits of the West Siberian basin. Novosibirsk: INGG SO RAN.
Ganguli, S. S., Vedanti, N., Pandey, O. P., and Dimri, V. P. (2018). Deep thermal regime, temperature induced over-pressured zone and implications for hydrocarbon potential in the Ankleshwar oil field, Cambay basin, India. J. Asian Earth Sci. 161, 93–102. doi:10.1016/j.jseaes.2018.05.005
Gu, Y., Wan, Y., Huang, J., Zhuang, X., Wang, B., Li, M., et al. (2019). Mutual correlation attentive factors in dyadic fusion networks for speech emotion recognition. Pet. Geol. Exp. 41 (2), 157–166. doi:10.1145/3343031.3351039
Gubin, I. A. (2004). The problem of assessing the filtration-capacitance properties of the reservoir in connection with epigenetic changes. J. Min. Inst. 159 (2), 7–10.
Guliyev, I. S., Kerimov, V. Yu., Osipov, A. V., and Mustaev, R. N. (2017). Generation and accumulation of hydrocarbons at great depths under the earth’s crust. SOCAR Proc. (1), 4–16. doi:10.5510/ogp20170100302
Hao, F., Zou, H., Ni, J., Zeng, Z., and Wang, M. (2002). Evolution of overpressured systems in sedimentary basins and conditions for deep oil/gas accumulation. Earth Science-Journal China Univ. Geosciences 27 (5), 610–615.
Helgeson, H. C., Richard, L., McKenzie, W. F., Norton, D. L., and Schmitt, A. (2009). A chemical and thermodynamic model of oil generation in hydrocarbon source rocks. Geochimica Cosmochimica Acta 73 (3), 594–695. doi:10.1016/j.gca.2008.03.004
HPHT (2020). HPHT production in the Gulf of Mexico. New Orleans: U.S. Department of the Interior Bureau of Ocean Energy Management New Orleans Office.
Huang, J., Deliao, Y., and Yu, H. (2016). Petoleum geology features and accumulation controls for ultra-deep oil and gas reservoirs. Petroleum Geol. Exp. 38 (5), 635–640. doi:10.11781/sysydz201605635
Iskaziev, K. O., Syngaevsky, P. E., and Khafizov, S. F. (2021). Deep oil. Offshore fields in the Gulf of Mexico in the Norflet Formation. Development history and prospects. Kazakhstan J. oil gas industry 3 (2), 3–26. doi:10.54859/kjogi89466
Jarvie, D. (2012). Shale resource systems for oil and gas: Part 2: Shale-Oil resource systems. AAPG Mem. 97, 89–119. doi:10.1306/13321447M973489
Jia, C., and Pang, X. (2015). Research processes and main development directions of deep hydrocarbon geological theories. Acta Pet. Sin. 36 (12), 1457–1469. doi:10.7623/syxb201512001
Kerimov, V. Yu., Mustaev, R. N., and Osipov, A. V. (2018). Peculiarities of hydrocarbon generation at great depths in the crust. Dokl. Earth Sci. 483 (3), 1413–1417. doi:10.1134/s1028334x18110193
Kerimov, V. Yu., and Osipov, A. V. (2016). Oil and gas content of great depths and promising areas of geological exploration for oil and gas in deeply submerged horizons in the territory of the Russian Federation. RU: Delovoy zhurnal Neftegaz.
Khafizov, S. F., Osipov, A. V., Dantsova, K. I., Monakova, A. S., and Barshin, A. V. (2020). “Factors that determine the formation and preservation of accumulations of liquid hydrocarbons at depths of more than 5 km,”. Geomodel.
Kondrasheva, N. K. (2018). “Evaluation of the effectiveness of using oil shale,” in Innovation-based development of the mineral resources sector: Challenges and prospects - 11th conference of the Russian-German raw materials (Boca Raton, Florida, United States: CRC Press).
Kontorovich, A. E., Danilova, V. P., Kostyreva, E. A., and Stasova, O. F. (1998). Geochemistry and Genesis of paleozoic oilfields of western siberia. Geochemistry 36 (1), 3–17.
Kontorovich, A. E., Ivanov, I. A., Kovešnikov, A. E., Krasnov, V. I., and Perozio, G. N. (1991). “Geological conditions of oil and gas potential of the upper part of the Paleozoic section of Western Siberia,” in Theoretical and regional problems of oil and gas geology (Novosibirsk: Nauka).
Kontorovich, A. E., Burstein, L. M., and Livshits, V. R. (2021). Theory of naphthidogenesis: A quantitative model of the evolution of aquagenic organic matter in catagenesis. Geol. Geophys. 62 (8), 1026–1047. doi:10.15372/gig2021119
Kosenkova, N. N., Syngaevsky, P. E., and Khafizov, S. F. (2022). Review of the modern ideas about the hydrocarbon accumulations formation processes at the great depth. Neft. khozyaystvo - Oil Ind. (5), 6–12. doi:10.24887/0028-2448-2022-5-6-12
Kuandykov, B. M., Syngaevsky, P. E., and Hafizov, S. F. (2022). Formation and preservation of reservoirs at great depths. Bull. oil gas industry Kazakhstan 4 (2), 11–26. doi:10.54859/kjogi100605
Kudryavtsev, N. A. (1951). Against the organic hypothesis of the origin of oil. Oil Ind. (9), 17–24.
Leonov, Yu.G., Volozh, Yu.A., Antipov, M. P., Bykadorov, V. A., Patina, I. S., and Lodzhevskaya, M. I. (2015). Oil of deep horizons of sedimentary basins of Russia and neighboring countries. Monit. Sci. Technol. Sci. Tech. J. 25 (4), 6–15.
Li, J., Zhang, Z., Zhu, G., Zhao, K., Chi, L., Wang, P., et al. (2021). Geochemical characteristics and the origin of superdeep condensates in Tarim basin, China. ACS Omega 6 (11), 7275–7285. doi:10.1021/acsomega.0c04932
Li, Y., Lobusev, A. V., Bochkarev, A. V., Wang, Q., and Liu, R. (2020). The nature and modeling of the oil reservoir fractures in X field of the Tarim basin (China). Geol. Geophys. Dev. Oil Gas Fields (3), 27–34. doi:10.30713/2413-5011-2020-3(339)-27-34
Litvinenko, V. S., Kozlov, A. V., and Stepanov, V. A. (2017). Hydrocarbon potential of the Ural–African transcontinental oil and gas belt. J. Petroleum Explor. Prod. Technol. 7 (1), 1–9. doi:10.1007/s13202-016-0248-4
Liu, J., Jin, Z., and Ma, A. (2015). Hydrocarbon phase in the deep cambrian of the Tarim Basin. Pet. Geol. Exp. 37 (6), 681–688. doi:10.11781/sysydz201506681
Liu, Y., Tian, Y., Yang, C., Xiao, D., Liao, Q., Shen, C., et al. (2017). Hydrocarbon phase limit and conversion process in the deep formation from Qikou Sag of Bohai Bay rift lacustrine basin, China. J. Nat. Gas Geoscience 2 (4), 229–238. doi:10.1016/j.jnggs.2017.12.003
Liu, Z., Zhu, W., Sun, Q., Jin, B., Xu, X., Zhang, H., et al. (2012). Blockade of autocrine TGF-β signaling inhibits stem cell phenotype, survival, and metastasis of murine breast cancer cells. Shiyou Xuebao/Acta Pet. Sin. 33, 1–8. doi:10.4172/2157-7633.1000116
Lu, S., Xue, H.-T., and Zhong, N.-N. (2002). Chemical kinetic study of the oil preservation threshold. Petroleum Explor. Dev. 29 (6), 1–3.
Lu, X., Zhao, M., Liu, K., Zhuo, Q., Fan, J., Yu, Z., et al. (2018). Forming condition and mechanism of highly effective deep tight sandstone gas reservoir in Kuqa foreland basin. Shiyou Xuebao/Acta Pet. Sin. 39 (4), 365–378. doi:10.7623/syxb201804001
Ma, A. (2016). Kinetics of oil-cracking for different types of marine oils from Tahe Oilfield, Tarim Basin, NW China. J. Nat. Gas Geoscience 1 (1), 35–43. doi:10.1016/j.jnggs.2016.03.001
Malyshev, N. A. (2004). Tectonics, evolution and oil and gas content of sedimentary basins of the European North. Yekaterinburg: Ural Branch of the Russian Academy of Sciences, Komi Scientific Center.
Malyshev, N. A. (2000). Tectonics, evolution and oil and gas potential of sedimentary basins of the European North of Russia. Russia: Doctoral dissertation. MSU.
Meihejef, (1993). The oil bearing temperature conditions on deep strata. Pet. Geol. Form. 14 (3/4), 1–4.
Neruchev, S. G., Rogozina, E. A., Parparova, G. M., Zelichenko, I. A., Silina, N. P., Lebedev, B. A., et al. (1986). Oil and gas formation in Domanik deposits. Leningrad, USSR: Nedra.
Neruchev, S. G., Vassoevich, N. B., and Lopatin, N. V. (1976). On the catagenesis scale in connection with oil and gas formation Combustible Minerals. Moscow, USSR: Nauka.
Osipov, A. V. (2021). Characteristics of hydrocarbon reserves in deep accumulations of oil and gas provinces of the Russian Federation. Neft. khozyaystvo - Oil Ind. (5), 8–10. doi:10.24887/0028-2448-2021-5-8-10
Pang, X.-Q., Jia, C.-Z., and Wang, W.-Y. (2015). Petroleum geology features and research developments of hydrocarbon accumulation in deep petroliferous basins. Petroleum Sci. 12 (1), 1–53. doi:10.1007/s12182-015-0014-0
Peters, K. E., Kontorovic, A. Eh., Huizinga, B. J., Moldowan, J. M., and Lee, C. Y. (1994). Multiple oil families in the West Siberian basin. AAPG Bull. 78 (6), 893–909. doi:10.1306/a25fe3dd-171b-11d7-8645000102c1865d
Petrakov, D. G., Penkov, G. M., and Zolotukhin, A. B. (2022). Experimental study on the effect of rock pressure on sandstone permeability. J. Min. Inst. 254, 244–251. doi:10.31897/PMI.2022.24
Porfiriev, V. B. (1987). The nature of oil, gas and fossil coals: Selected works: In 2 vols. Sciences 1–2, 440.
Price, L. C. (1993). Thermal stability of hydrocarbons in nature: Limits, evidence, characteristics, and possible controls. Geochimica Cosmochimica Acta 57 (14), 3261–3280. doi:10.1016/0016-7037(93)90539-9
Price, L. C., and Wenger, L. M. (1992). The influence of pressure on petroleum generation and maturation as suggested by aqueous pyrolysis. Org. Geochem. 19 (1-3), 141–159. doi:10.1016/0146-6380(92)90033-t
Prischepa, O. M., Averyanova, O. Yu., and Zharkov, A. M. (2013). Oil and gas deposits of domanic type – A reserve to maintain hydrocarbons production in commercially developed areas. Georesursy 54 (4), 18–22. doi:10.18599/grs.54.4.3
Prischepa, O. M., Nefedov, Y., Nikiforova, V., and Ruiming, X. (2022). Raw material base of Russia’s unconventional oil and gas reserves (hydrocarbons shale strata). Front. Earth Sci. 10, 1. doi:10.3389/feart.2022.958315
Qiu, N., Liu, W., Xu, Q., Liu, Y., and Chang, J. (2018). Temperature-pressure field and hydrocarbon accumulation in deep-ancient marine strata. Diqiu Kexue - Zhongguo Dizhi Daxue Xuebao/Earth Sci. - J. China Univ. Geosciences 43 (10), 3511–3525. doi:10.3799/dqkx.2018.286
Ren, Z., Cui, J., Qi, K., Yang, G., Chen, Z., Peng, Y., et al. (2020). Control effects of temperature and thermal evolution history of deep and ultra-deep layers on hydrocarbon phase state and hydrocarbon generation history. Nat. Gas. Ind. B 7 (5), 453–461. doi:10.1016/j.ngib.2020.09.003
Ren, Z., Liu, L., and Cui, J. (2008). Application of tectonic thermal evolution history to hydrocarbon accumulation timing in sedimentary basins. Oil Gas Geol. 29 (4), 502–506. doi:10.11743/ogg20080413
Samvelov, R. (1995). Hydrocarbon deposits at deep depths: Features of formation and placement. Geol. oil gas (9), 5–15.
Shen, Y., Lü, X., Guo, S., Song, X., and Zhao, J. (2017). Effective evaluation of gas migration in deep and ultra-deep tight sandstone reservoirs of Keshen structural belt, Kuqa depression. J. Nat. Gas Sci. Eng. 46 (46), 119–131. doi:10.1016/j.jngse.2017.06.033
Shimansky, V. K., Neruchev, S. G., Bazhenova, T. K., Prischepa, O. M., and Smirnov, S. V. (1994). “Geochemical modelling of Timan-Pechora oil and gas basin on the basis of balance and kinetic models petroleum Exploration and Production in Timan-Pechora Basin and Barents Sea,” in Collected reports of international conference august 15-17, 1994 (St. Petersburg, Russia: VNIGRI).
Sokolov, B. A. (1980). Evolution and oil and gas content of sedimentary basins. Kazakhstan: M. Nauka.
Sun, L., Zou, C., Zhu, R., Zhang, Y., Zhang, S., Zhang, B., et al. (2013). Formation, distribution and potential of deep hydrocarbon resources in China. Petroleum Explor. Dev. 40 (6), 687–695. doi:10.1016/s1876-3804(13)60093-2
Tissot, B. P., and Welte, D. H. (1984). Petroleum Formation and occurrence. Berlin: SpringereVerlag.
Tissot, B., and Welte, D. (1981). Fate of organic matter in sedimentary basins: Oil and gas generation. Moscow, Russia: Oil formation and distribution: Mir.
Torba, D. I., and Nikiforova, V. S. (2019). Proceedings of the XIV international forumcontest of young researchers. St. Petersburg, Russia: Litvinenko V.Oil recovery increase in kerogen of the Bazhenov formation by a thermal treatment technology,” in Topical issues of rational use of natural resources
Tuo, J. (2002). Research status and advances in deep oil and gas exploration. Adv. Earth Sci. 17 (4), 565–571. doi:10.11867/j.issn.1001-8166.2002.04.0565
Volozh, Y. A., Gogonenkov, G. N., Miletenko, N. V., and Petrov, E. I. (2021). Oil resources development from deep horizons in historically oil producing regions. Geol. Oil Gas 6, 7–21. doi:10.31087/0016-7894-2021-6-7-21
Wang, Z., Wang, T., Wen, L., Hua, J., and Baomin, Z. (2014). Basic geological characteristics and accumulation conditions of Anyue giant gas field, sichuan basin. China Offshore Oil Gas 28 (2), 45–52.
Wassoevich, N. B. (1967). Theory of sedimentary-migratory origin of oil (historical review and current state). Izv. USSR Acad. Sci. Ser.geol. (11), 135–156.
Wei, Q., Li, X., and Sun, K. (2019). Geochemical characteristics of deep-seated natural gas accumulation of the Keshen large gas field in the Kuqa depression. Tarim Basin. Nat. Gas. Geosci. 30 (6), 897–907.
Weisberg, L. A., Kameneva, E. E., and Nikiforova, V. S. (2018). Microtomographic studies of the pore space of rocks as a basis for improving the technology of their disintegration. Ob. Rud. 2018 (3), 51–55. doi:10.17580/or.2018.03.09
Yang, P., Liu, K., Liu, J., Yu, S., Yu, B., Hou, M., et al. (2021). Petroleum charge history of deeply buried carbonate reservoirs in the Shuntuoguole Low Uplift, Tarim Basin, west China. Mar. Petroleum Geol. 128, 105063. doi:10.1016/j.marpetgeo.2021.105063
Zakharov, L. А., Martyushev, D. А., and Ponomareva, I. N. (2022). Predicting dynamic formation pressure using artificial intelligence methods. J. Min. Inst. 253, 23–32. doi:10.31897/PMI.2022.11
Zhang, S., Huang, H., Su, J., and Liu, M. (2015). Ultra-deep liquid hydrocarbon exploration potential in cratonic region of the Tarim Basin inferred from gas condensate Genesis. Fuel 160, 583–595. doi:10.1016/j.fuel.2015.08.023
Zhao, X., Jin, F., Wang, Q., Zhang, R., Wang, J., Bai, X., et al. (2011). Niudong 1 ultra-deep and ultra-high temperature subtle buried hill field in Bohai Bay basin: Discovery and significance. Acta Pet. Sin. 32 (6), 915–927. doi:10.7623/syxb201106001
Zhou, X., Zhang, R., Li, H., Wang, B., and Guo, Y. (2017). Major controls on natural gas accumulations in deep-buried hills in Bozhong Depression, Bohai Bay Basin. J. China Univ. Petroleum Ed. Nat. Sci. 41 (1), 42–50.
Zhu, G., Milkov, A. V., Chen, F., Weng, N., Zhang, Z., Yang, H., et al. (2018). Non-cracked oil in ultra-deep high-temperature reservoirs in the Tarim basin, China. Mar. Petroleum Geol. 89, 252–262. doi:10.1016/j.marpetgeo.2017.07.019
Zhu, G., Milkov, A. V., Li, J., Xue, N., Chen, Y., Hu, J., et al. (2021). Deepest oil in Asia: Characteristics of petroleum system in the Tarim basin, China. J. Petroleum Sci. Eng. 199, 108246. doi:10.1016/j.petrol.2020.108246
Zhukov, V. S., and Kuzmin, Y. O. (2021). Experimental evaluation of compressibility coefficients for fractures and intergranular pores of an oil and gas reservoir. J. Min. Inst. 251, 658–666. doi:10.31897/PMI.2021.5.5
Keywords: ultra-deep depths, oil and gas, deep accumulations, deep origin, high temperature, high pressure
Citation: Prischepa OM, Kireev SB, Nefedov YV, Martynov AV, Lutsky DS, Krykova TN, Sinitsa N and Xu R (2023) Theoretical and methodological approaches to identifying deep accumulations of oil and gas in oil and gas basins of the Russian Federation. Front. Earth Sci. 11:1192051. doi: 10.3389/feart.2023.1192051
Received: 22 March 2023; Accepted: 20 April 2023;
Published: 17 May 2023.
Edited by:
Liang Chen, China University of Mining and Technology, ChinaReviewed by:
Andrey Kuranov, Timan-Pechora Research Center, RussiaIlya Vinokurov, A.P. Karpinsky Russian Geological Research Institute, Russia
Oleg Smirnov, All Russian Research Institute of Geology and Mineral Resources of the World Ocean, Russia
Copyright © 2023 Prischepa, Kireev, Nefedov, Martynov, Lutsky, Krykova, Sinitsa and Xu. This is an open-access article distributed under the terms of the Creative Commons Attribution License (CC BY). The use, distribution or reproduction in other forums is permitted, provided the original author(s) and the copyright owner(s) are credited and that the original publication in this journal is cited, in accordance with accepted academic practice. No use, distribution or reproduction is permitted which does not comply with these terms.
*Correspondence: Ruiming Xu, eDQ3Mjc5NDc3M0BnbWFpbC5jb20=