- 1Research Institute of Exploration and Development, Xinjiang Oilfield Company, PetroChina, Karamay, China
- 2College of Earth Sciences, Jilin University, Changchun, China
The Carboniferous volcanic reservoirs in the Shixi area of the Junggar Basin are complex and diverse. Identifying the characteristics and main factors controlling high-quality volcanic reservoirs is the key to increasing oil and gas reserves and production in this area. Through core observations, thin section identification, physical property and pore structure analyses, combined with production data, the main controlling factors and development modes of high-quality reservoirs were analysed. The results show that the Carboniferous strata in the Shixi area mainly contain andesite and dacite of overflow facies, followed by volcanic breccia and tuff of explosive facies. Volcanic reservoirs in the study area are high-porosity–low-permeability and medium-porosity–low-permeability reservoirs. Volcanic breccia of explosive facies has the best physical properties, showing the characteristics of high porosity and medium permeability. The reservoir space is mainly composed of gas cavities, corrosion pores and fractures, among which the corrosion pores are the most important reservoir spaces of the Carboniferous volcanic rocks. Lithology and lithofacies, weathering and corrosion, and fractures are the main factors controlling the development of high-quality volcanic reservoirs. Volcanic rocks that had experienced weathering and denudation for a long time developed a large number of secondary corrosion pores due to the corrosion of soluble minerals or volcanic ash. Fractures further improved the physical properties, causing volcanic rocks to eventually develop into weathering crust reservoirs. The physical properties of the volcanic rocks far away from the weathering crust were improved through primary gas cavities and structural fractures, and these volcanic rocks eventually developed into the inner reservoir.
1 Introduction
As a special type of oil and gas reservoir, volcanic reservoirs have become an important new field for global oil and gas exploration (Zhang et al., 2016). At present, more than 300 volcanic oil and gas reservoirs have been discovered in more than 100 countries worldwide, and the potential of oil and gas resources is massive (Wang et al., 2012). At present, onshore volcanic oil and gas exploration has entered the stage of rapid development, and proven geological reserves have increased greatly. Industrial oil and gas flow has been found in Carboniferous and Permian volcanic rocks in the Songliao Basin, Bohai Bay Basin, Junggar Basin and Santanghu Basin (Wang et al., 2021). Since 1998, great breakthroughs have been made in the exploration of Carboniferous volcanic reservoirs in the eastern Junggar Basin. In 2008, the Kelameili gas field was discovered in the Carboniferous volcanic rocks in the eastern Junggar Basin, and this discovery opened a new opportunity for the exploration and development of volcanic oil and gas in the Junggar Basin (Chen et al., 2020). In recent years, large oil reservoirs, such as C210, JL10, and C471, have been discovered in the northwest margin of the basin, and the proven geological petroleum reserves have reached 250 million tons. With the continuous breakthrough in the exploration of Carboniferous volcanic rocks in the eastern and western Junggar Basin, the exploration of Carboniferous rocks in the hinterland of the basin has received attention again. The discovery of Carboniferous oil and gas in the hinterland of the basin began in 1992. Well SX1, which was drilled in the Carboniferous buried hill of the Shixi uplift, yielded high industrial oil flow, which opened the door for the exploration of the Shixi oilfield, with proven geological reserves of 38.94 million tons (Li H et al., 2021). After decades of exploration and research, major breakthroughs have been made in SX16, SX161H, SX101, SX102, and other wells, indicating that the Shixi area has large prospects for oil and gas resources.
The reservoir characteristics and physical properties of volcanic rocks play an important role in the accumulation and distribution of oil and gas in volcanic strata. Volcanic reservoir spaces are of various types. According to their morphological characteristics, volcanic reservoir spaces can be divided into primary pores, secondary pores and fractures. The reservoir spaces of the Carboniferous volcanic rocks in the Shixi area are mainly primary pores, corrosion pores and fractures, which are found in typical pore–fracture reservoirs (Li S B et al., 2021; Wang and Wang, 2021). The types of reservoir spaces in volcanic rocks with different lithologies are different. Some lava has primary gas cavities, while some volcanic breccia and tuff have secondary corrosion pores. Diagenesis controls the preservation of primary pores and the development of secondary pores during the evolution of volcanic rocks (Feng et al., 2015; Lan et al., 2021). Wang et al. (2021) divided the diagenetic stage of deep volcanic rocks in the Songliao Basin into the cooling diagenesis stage, postmagmatic hydrothermal stage, weathering denudation and leaching stage and burial diagenesis stage according to the diagenetic evolutionary sequence and spatiotemporal pore evolutionary process. Qu et al. (2014) divided the diagenesis of the Kelameili gas field in the Junggar Basin into a syngenetic stage, epigenetic stage and burial diagenesis stage. The burial evolution of volcanic rocks determines the diagenetic evolutionary sequence of a region. Previous studies have suggested that volatilization, corrosion, shrinkage, devitrification, and recrystallization play an important role in improving volcanic reservoirs, while hydrothermal precipitation and crystallization, compaction, cementation and filling in the penecontemporaneous period are not conducive to the development of volcanic reservoirs (Guo H et al., 2021). At present, volcanic activity, tectonic movement, lithology, lithofacies, corrosion and weathering are considered to be important factors controlling the development of high-quality volcanic reservoirs. Qin et al. (2012) believed that volcanic reservoirs in the Junggar Basin were mainly distributed in tectonic belts with strong tectonic action and relatively developed fractures. Lithology and lithofacies are the basic influencers of reservoir properties. Weathering and leaching are very important for influencing reservoir properties. Volcanic rocks can become effective reservoirs only after weathering and leaching occur. A large number of primary gas cavities formed by volcanic eruptions are not connected, while weathering and leaching can connect the reservoir pores near the weathering crust to form effective reservoirs.
The lithology and lithofacies of the Carboniferous volcanic rocks in the Shixi area are complicated, and the long-term weathering and multistage tectonic transformation cause the volcanic rocks to have strong heterogeneity. Explorations show that there are great differences in the gas content and productivity of wells in the Shixi area. Therefore, it is urgent to identify the types and characteristics of high-quality Carboniferous volcanic reservoirs in the study area and determine the main factors controlling the development of high-quality volcanic reservoirs. Based on a summary of previous research results, combined with the production data and experimental analysis, this paper carried out research on the characteristics of volcanic reservoirs, the main controlling factors and the development model of high-quality reservoirs. The research results are expected to provide a geological basis for predicting the distribution of high-quality volcanic reservoirs and guiding future exploration and development in the Shixi area.
2 Geological setting
The Shixi area is located in the subunits of the Shixi uplift and Mobei uplift in the southern part of the Luliang uplift in the centre of the Junggar Basin and is adjacent to the West sag of the Pen 1 well and the Shinan sag in the north and south, respectively, with superior oil and gas conditions (Sun et al., 2019). The strata in the Shixi area are well developed, ranging from Carboniferous to Neogene. From bottom to top, the strata are as follows: Carboniferous strata, the Permian Wuerhe Formation (P2w); Triassic Baikouquan Formation (T1b), Karamay Formation (T2k) and Baijiantan Formation (T3b); Jurassic Badaowan Formation (J1b), Sangonghe Formation (J1s), Xishanyao Formation (J2x), and Toutunhe Formation (J2t); and Cretaceous strata. The Permian is missing in the main part of the Shixi uplift, forming an angular unconformity. The top of the Jurassic is unconformable with the Cretaceous. In the early Carboniferous period, the Luliang island arc was affected by the subduction of oceanic crust, which resulted in large-scale volcanic eruptions and the development of extremely thick volcanic rocks. The drilling results show that the upper Carboniferous series in the Shixi area mainly contains alternating overflow facies and explosive facies, but mainly overflow facies. The rock types of overflow facies mainly include basalt, andesite, dacite and rhyolite. Volcanic breccia and tuff are the main explosive facies. The upper Carboniferous volcanic rocks can form large-scale oil and gas reservoirs by connecting with the oil- and gas-generating depression through the large convex fault (Figure 1).
3 Samples and experiments
3.1 Samples
In this study, Carboniferous volcanic rock cores from 9 wells in the Shixi area were observed and described, and volcanic rock core samples from 6 wells were collected for experimental analyses of the lithology and reservoir.
3.2 Experiments
An Olympus microscope was used to observe rock thin sections. The minerals, pores, lithologies and other characteristics of volcanic rock samples were analysed. Samples of different lithologies were selected for the subsequent experimental analysis of reservoir physical properties and pore structures.
An Axios-max wavelength dispersive X-ray fluorescence spectrometer was used to analyse the major elements of volcanic rocks. Based on GB/T14506-2010, the test was conducted at 24°C, and the relative humidity was 24%. The instrument used for trace element analysis was a NexION300D plasma mass spectrometer. Based on GB/T14506.30-2010, the test was conducted at 20°C, and the relative humidity was 22%.
Petrophysical properties (porosity and permeability) were completed at Northeast Petroleum University. The experimental equipment was a PoroPDP-200 overburden pressure porosimeter from American Core Company, and the experimental test was carried out according to the SY/T5336-1996 national standard. After the samples were washed and dried, 25 massive rock samples were used under the condition of 25°C. The porosity of each sample was measured and calculated according to Boyle’s law, and the permeability was measured by the unsteady state method.
Nitrogen adsorption experiments were completed at Northeast Petroleum University. The experiment was carried out by the fully automatic gas adsorption instrument ASAP2460 specific surface area and pore analyser of Mack Instruments, which followed the national standards GB/T19587-2004 and GB/T21651.2-2008 of the People’s Republic of China. Nitrogen was used as the adsorbent, and the corresponding time points and different nitrogen adsorption amounts were recorded at −195.8°C for adsorption experiments (Lollar et al., 2014; Hirschmann and Cottrell, 2017).
4 Results
4.1 Volcanic lithology
The division of the lithology and lithofacies of volcanic rocks is the basic work that is conducted to study volcanic reservoirs. According to the logging data of wells in the study area, combined with core observations and descriptions and thin section identification, the lithology of Carboniferous volcanic rocks in the Shixi area was identified based on the classification scheme of volcanic rock lithology (Wang et al., 2003; Zhang J et al., 2010; Xue et al., 2021). The Carboniferous volcanic rocks in the study area can be divided into volcanic lava and pyroclastic rock. Volcanic lava can be divided into basic basalt, intermediate andesite, intermediate–acidic dacite and acidic rhyolite. Pyroclastic rocks include tuff and volcanic breccia. The intermediate-acidic dacite and intermediate andesite in the study area are the most developed, accounting for 38% and 20% of the total lithology. The second most common rocks are volcanic breccia and tuff, which account for an equal proportion (15%). Basic basalt (8%) and acidic rhyolite (4%) are only minorly present in the study area (Figure 2A).
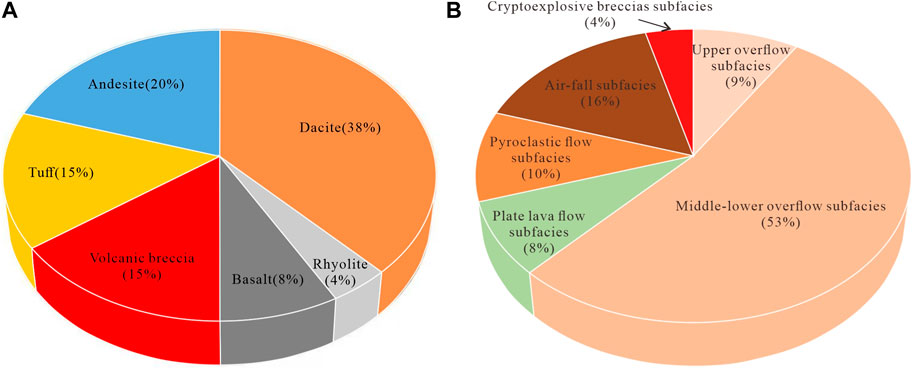
FIGURE 2. Lithologies (A) and lithofacies (B) statistics of Carboniferous volcanic rocks in the Shixi area.
According to the lithology–fabric–genetic volcanic lithofacies classification scheme (Qin et al., 2016), the volcanic lithofacies types in the study area include explosive facies, overflow facies and volcanic vent facies. The intermediate–acidic overflow facies include the upper subfacies and middle–lower subfacies. The explosive facies are mainly air-fall and pyroclastic flow subfacies. The volcanic vent facies includes only the cryptoexplosive breccia subfacies, and the basic overflow facies is plate lava flow subfacies. The study area mainly contains the middle–lower subfacies of the intermediate–acidic overflow facies (53%), followed by the air-fall subfacies of the explosive facies (16%). Volcanic vent facies are not developed (Figure 2B).
4.2 Reservoir space types
Core and thin section observations and descriptions show that the reservoir space types of the Carboniferous volcanic reservoirs in the Shixi area are divided into primary pores, secondary pores and fractures. The primary pores of the volcanic reservoirs in the study area are mainly gas cavities and apricot kernel inner pores. The gas cavities are mainly present in the upper subfacies of the intermediate and intermediate–acidic overflow facies. The gas cavities are characterized by directional elongation because they were formed when the lava gradually cooled. Microscopically, pores of different sizes and densities appear in isolation or in groups (Figures 3A, B). Most of the gas cavities were not effectively preserved, but secondary mineral filling with the participation of fluid occurred in the burial stage. Due to the influence of differential filling, some gas cavities did not completely fill the minerals and retained apricot kernel inner pores (Figure 3C). The apricot kernel inner pores are polygonal or angular. Such pores have no obvious traces of corrosion, and their contours are mostly affected by filling minerals. The apricot kernel inner pores are mostly associated with gas cavities; that is, where the primary gas cavities are present, the apricot kernel inner pores are also present.
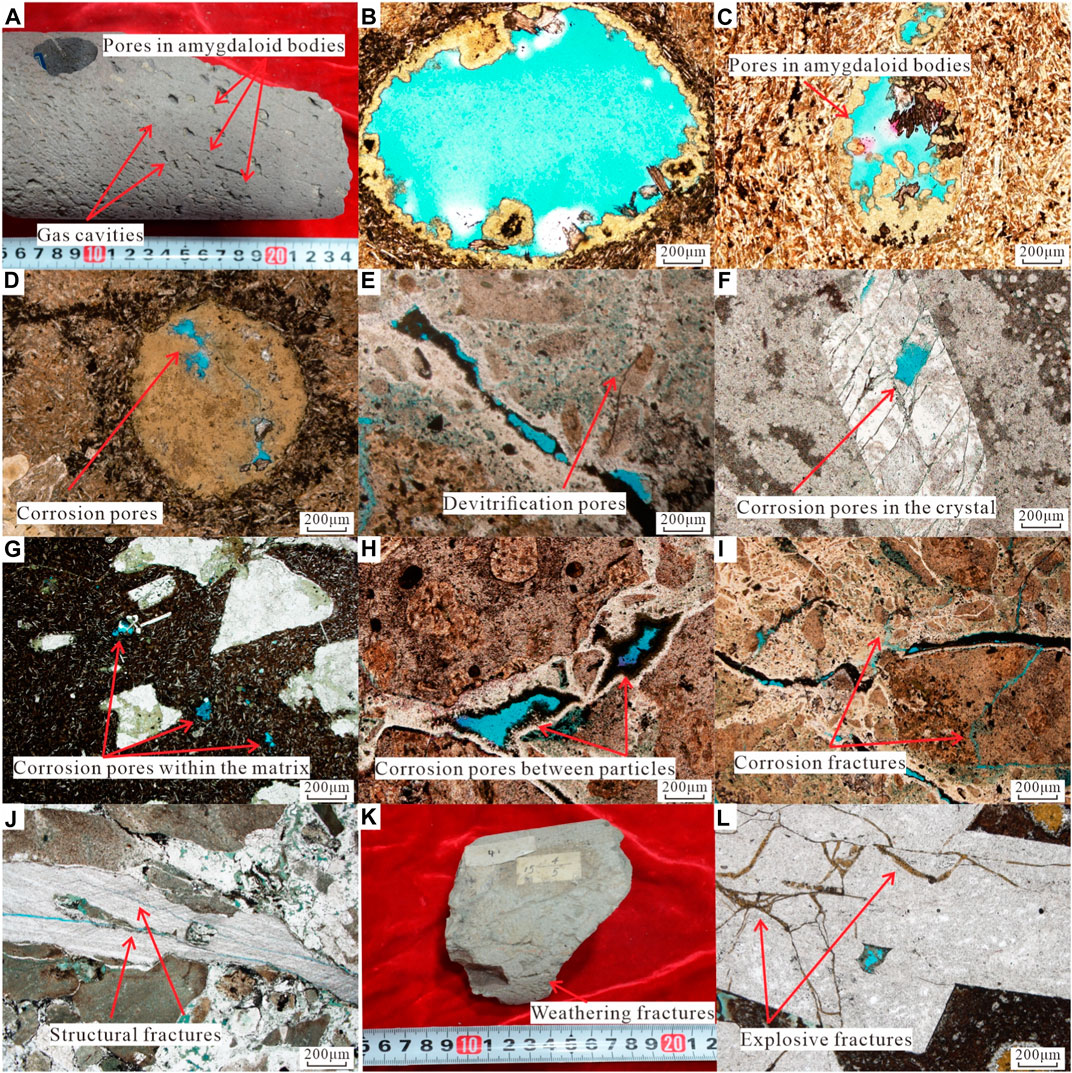
FIGURE 3. Characteristics of the volcanic reservoir space in the Shixi area. [(A). Pores in amygdaloid bodies, SX4, 4,720 m; (B) Gas cavities, SX16, 4,805 m; (C) Apricot kernel inner pores, SX16, 4,806 m; (D) Corrosion pores in amygdaloid bodies, SX16, 4,805 m; (E) Devitrification pores, SX3, 4,867.5 m; (F) Corrosion pores in the crystals, SX4, 4,606 m; (G) Corrosion pores in the matrix, SX16, 4,812 m; (H) Corrosion pores between particles, SX3, 4,867 m; (I) Corrosion fractures, SX3, 4,867.5 m; (J) Structural fractures, SX3, 4,721 m; (K) Weathering fractures, SX1, 4,295.2 m; (L) Explosive fractures, SX4, 4,822.68 m).
The secondary pores are mainly corrosion pores formed by corrosion and devitrification pores formed by devitrification. Corrosion pores are the most developed pore type in the Carboniferous volcanic reservoir in the study area and are present in all kinds of volcanic rocks. They formed by a series of corrosion events in the phenocrysts, grains and matrix during diagenetic evolution. According to the different development sites, the corrosion pores can be further divided into intergranular corrosion pores, intragranular corrosion pores, matrix corrosion pores and apricot kernel corrosion pores (Figures 3D–H). The shape of the corrosion pores is irregular, and their connectivity is related to the mineral properties and corrosion strength. Corrosion pores are present in volcanic reservoirs with high soluble mineral contents and strong fluid activity in the formation. In addition, corrosion pores around gas cavities and fractures are present, indicating that gas cavities and fractures, as channels of fluid migration, promote the development of corrosion pores (Figure 3I). Devitrification pores are microscopic pores formed by devitrification of glass in volcanic rocks, resulting in volume reduction. Devitrification widely exists in tuff in the study area, so devitrification pores are the main pore type in dense tuff (Figure 3E).
The fractures in the study area are mainly structural fractures and corrosion fractures, followed by weathering fractures, blasting fractures, shrinkage fractures, etc. The fractures in the study area are relatively developed, and the pores of different scales are connected to form the main oil and gas flow channels, which greatly improves the permeability of volcanic reservoirs. The structural fractures are mostly high-angle fractures and oblique fractures, which are mostly filled with minerals. They have the characteristics of straight, long extensions and deep cutting. The phenomenon of structural fractures cutting through minerals can be observed under the microscope (Figure 3J). Affected by corrosion, corrosion fractures are also present in Carboniferous volcanic reservoirs. Most of them are secondary fractures formed on the basis of the corrosion of tectonic fractures and intergranular fractures filled with soluble minerals, which are often found in volcanic breccia. Carboniferous volcanic rocks are subjected to long-term weathering and denudation, and a large number of weathering fractures are present in the top weathering crust, and these fractures cut each other and break the rocks (Figure 3K). In addition, explosive fractures are also present in some volcanic rocks, especially in cryptoexplosive breccia, which breaks phenocrysts (Figure 3L). Diagenetic shrinkage fractures are not present in the study area, which has little effect on the physical properties of the reservoir.
4.3 Reservoir physical properties
The lithologies of the Carboniferous volcanic rocks in the Shixi area are complex and diverse, and the reservoir properties of volcanic reservoirs with different rock types are obviously different. The reservoir capacity of volcanic reservoirs determines whether oil and gas can accumulate. Therefore, it is very important to identify the reservoir properties of different types of volcanic reservoirs and clarify the types of high-quality volcanic reservoirs for the exploration of volcanic reservoirs in the study areas (Rimmer, 2004; Zhang Y. et al., 2010).
The Carboniferous volcanic reservoirs in the Shixi area are divided into four types according to the characteristics of porosity and permeability (Figure 4): Class I is a high porosity-medium permeability reservoir (φ≥12%, K≥1×10−3 um2). Class II is a high porosity-low permeability reservoir (φ≥12%, K≤1×10−3 um2). Class III is a medium porosity-low permeability reservoir (5%≤φ<12%, K≤1×10−3 um2). Class IV is a low porosity-low permeability reservoir (φ<5%, K≤1×10−3 um2). The reservoir physical property data show that the heterogeneity of the Carboniferous volcanic reservoir is strong, and the porosity values are mainly between 0.91% and 20.6%, with an average of 12.98%. The permeability values range from 0.005×10−3 um2 to 37.72×10−3 um2, with an average of 1.113×10−3 um2 (Table 1). The Carboniferous volcanic reservoir is a Class II reservoir with high porosity and low permeability and a Class III reservoir with medium porosity and low permeability. The volcanic breccia reservoir of the explosive facies is a Class I high-quality volcanic reservoir, showing high porosity–medium permeability characteristics. The cryptoexplosive breccia reservoir of the volcanic vent facies and the gas-cavity lava reservoir of the upper subfacies of the overflow facies are Class II high-quality volcanic reservoirs, showing high-porosity and low-permeability characteristics.
4.4 Reservoir pore structure
Nitrogen adsorption experiments were carried out to qualitatively and quantitatively characterize the pore structure types and characteristics of volcanic reservoirs. According to the International Union of Pure and Applied Chemistry (IUPAC), hysteresis loops can be roughly divided into four types. Different hysteresis loop morphologies can reflect the characteristics of the pore structure (Wrihgt et al., 1987; Wignall and Twitchett, 1996). In the process of nitrogen injection and adsorption, capillary condensation occurs first in the micropores. With increasing relative pressure, capillary condensation occurs in the mesopores and macropores until all spaces are filled with adsorbed substances (Melnik et al., 2005). However, the removal of capillary condensation occurs first in the macropores in the process of desorption, followed by mesopores and micropores, which leads to the phenomenon that the adsorption curve does not coincide with the desorption curve, namely, the hysteresis phenomenon. The stronger the hysteresis phenomenon is, the more obvious the hysteresis loop is, and the more inhomogeneous the pore size is (Pearce et al., 1984; Quanei and Russell, 2003; Patricia and Nora, 2007).
There are two types of pores in the Carboniferous volcanic reservoirs in the study area: plate-shaped pores and inkpot-shaped pores. Small hysteresis loops are visible in the adsorption–desorption curves of volcanic breccia, gas-cavity lava and cryptoexplosive breccia, which are manifested as H3-type hysteresis loops. This indicates that plate-shaped pores are developed in these three types of volcanic rocks. The connectivity of these volcanic rocks is good. The desorption curves of dense lava and tuff show a sudden drop near P/Po=0.5, and the hysteresis loops do not show adsorption restriction at high relative pressure, indicating H2-type hysteresis loops. This indicates that there are inkpot-shaped pores in these two types of volcanic rocks, with large numbers of small pores and narrow throats (Figure 5).
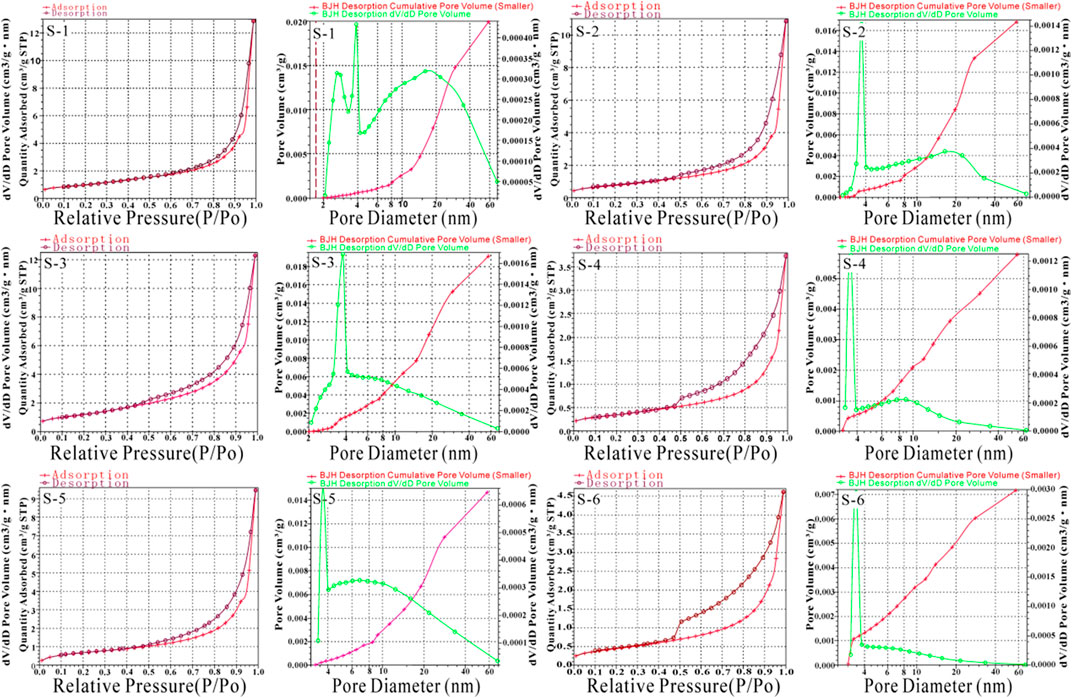
FIGURE 5. Nitrogen adsorption curves and pore size distributions of Carboniferous volcanic rocks. (S-1: Volcanic breccia, SX3, 4,720.81 m; S-2: basalt, SX4, 4,720.8 m; S-3: gas cavity andesite, SX4, 4,590 m; S-4: tuff, SX3, 4,786.59 m; S-5: cryptoexplosive breccia, SX16, 4,805.5 m; S-6: dense basalt, SX4, 4,720.2 m).
The specific surface, pore volume, and average pore size of the samples are shown in Table 2. Mesopores and macropores in volcanic breccia, gas-cavity lava and cryptoexplosive breccia are developed, which contribute greatly to the pore volume. However, dense lava and tuff mainly contain micropores and a certain number of mesopores, resulting in small pore volumes, which is consistent with the results of the porosity and permeability of volcanic rocks. The specific surface areas of lava and tuff are larger than those of volcanic breccia, gas-cavity lava and cryptoexplosive breccia because the micropores developed in lava and tuff increase the specific surface area. Therefore, the reservoir properties of volcanic breccia, gas-cavity lava and cryptoexplosive breccia are better than those of lava and tuff in terms of both the pore type and pore structure.
5 Discussion
5.1 Factors controlling high-quality reservoirs
Whether volcanic rocks can become effective reservoirs depends on many factors (Su et al., 2011; Nazari and Riahi, 2020; Chen et al., 2021; Meng et al., 2021). The above analysis shows that the development of Carboniferous high-quality volcanic reservoirs in the Shixi area is mainly controlled by three factors: the lithology and lithofacies, weathering and corrosion, and fractures. Favourable lithology and lithofacies are the basis for high-quality reservoir development. Weathering and corrosion are the keys to high-quality reservoir development, and fractures further improve the physical properties of volcanic reservoirs.
5.1.1 Favourable lithology and lithofacies are the basis for high-quality reservoir development
Lithology and lithofacies not only determine the type and development of primary pores but also affect the corrosion and the number of fractures, thus determining the effectiveness of reservoirs (Li et al., 2010; Shan et al., 2011; Wang et al., 2020). According to the statistics of the lithology and lithofacies of the tested oil-containing sections in the Shixi area, it is found that the oil- and gas-producing sections are mainly the volcanic breccia of the pyroclastic flow subfacies and the gas-cavity lava of the upper subfacies, followed by some cryptoexplosive breccia of volcanic vent facies. The non-oil- and gas-producing sections are dominated by dense lava of the lower subfacies (Figure 6).
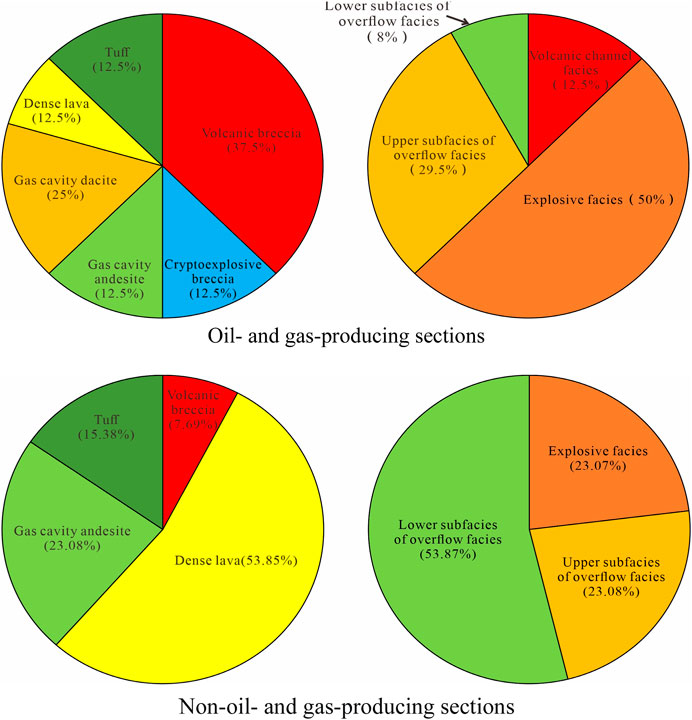
FIGURE 6. Lithologies and lithofacies of the Carboniferous tested oil-containing section in the Shixi area.
The volcanic breccia is reformed by weathering and corrosion, and some minerals and volcanic ash in the volcanic breccia have dissolved and form secondary pores (Figure 7A). In addition, the compaction resistance of breccia plays an important role in the preservation of pores, which is beneficial to the formation of high-quality reservoirs (Sruoga and Rubinstein, 2007; Yuan et al., 2015; Guo L et al., 2021). Cryptoexplosive breccia not only contains corrosion pores but also explosive fractures, which also show good physical properties (Figure 7B). The primary gas cavities of gas-cavity lava, which are important reservoir spaces of this kind of reservoir, are quite developed. Additionally, corrosion pores in the gas-cavity lava are also developed (Figure 7C). The concomitant formation of gas cavities and corrosion pores indicates that the primary pores increase the contacts between fluids and minerals. Therefore, volcanic breccia, gas-cavity lava and cryptoexplosive breccia are the lithologic bases for the development of high-quality volcanic reservoirs in the study area.
5.1.2 Weathering and corrosion are the key to the development of high-quality reservoirs
Favourable lithology and lithofacies can provide the basis for high-quality reservoir development, but high-quality reservoirs cannot form due to the lack of late corrosion. The pores and fractures formed by corrosion not only increase the reservoir space but also connect some isolated pores (He et al., 2007; Mao et al., 2015; Ma et al., 2019).
Under the influence of the late Hercynian movement, the closure of the West Junggar Ocean and Kalamari Ocean resulted in an intracontinental tectonic environment in the study area. The Carboniferous volcanic rocks were uplifted and denuded from the end of the Carboniferous to the middle of the Permian. Denudation resulted in the absence of upper Carboniferous strata and lower Permian strata, which provided a prerequisite for the development of volcanic weathering crust reservoirs on top of the Carboniferous strata (Li, 2019a; Fan et al., 2020). Vertically, the tops of volcanic rocks are strongly corroded and contain fractures. As weathering and corrosion weaken, the integrity of deep rocks improves. The development of corrosion pores and fractures is weakened significantly until no development occurs. The oil test results of Carboniferous volcanic reservoirs show that the closer to the top of the Carboniferous, the better the test production of the drilling is, indicating that weathering and corrosion control the distribution of oil and gas in the study area (Figure 8).
The weathering crust underwent hydrolysis, hydration, oxidation and other chemical processes during its formation, which both increased and decreased the contents of elements in volcanic rocks (Liu et al., 2020; Li et al., 2022). The depth of the weathering crust is dictated by the porosity, chemical index of alteration (CIA) and plagioclase index of alteration (PIA) (Figure 9). The porosity, CIA and PIA change regularly based on the distance from the top of the Carboniferous strata. The porosity, CIA and PIA decrease at approximately 200 m from the top of the Carboniferous strata, indicating that 200 m is approximately the bottom of the weathering crust.
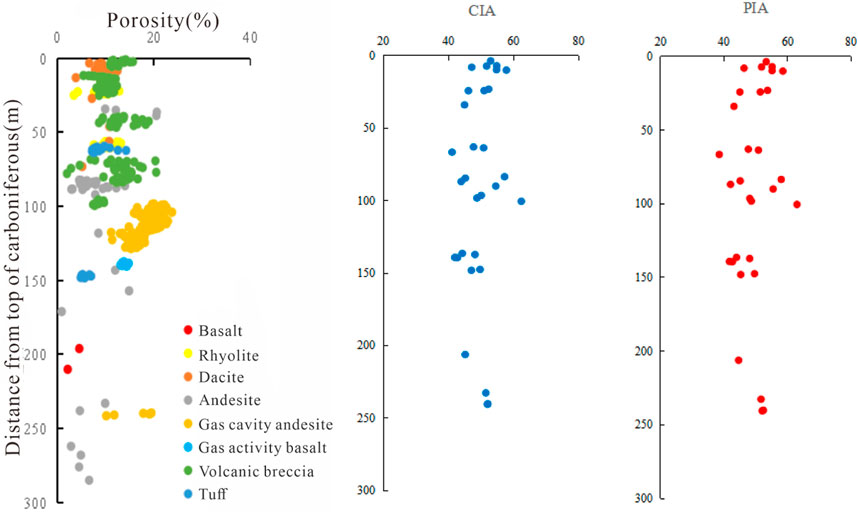
FIGURE 9. Relationships between the porosity, CIA and PIA and the distance from the top of the Carboniferous strata.
5.1.3 Fractures further improve the reservoir physical properties of volcanic reservoirs
Fractures are very important for hydrocarbon accumulation in tight volcanic reservoirs. Fractures not only improve the physical properties of volcanic reservoirs but also serve as the main migration channels for formation fluid, which promotes the development of corrosion pores and fractures. The fractures in the study area are mainly structural fractures and corrosion fractures, of which structural fractures have a great impact on the physical properties of the reservoir (Li et al., 2017; Gao et al., 2021).
Three stages of fractures are present in the study area: the near SN- and NE-trending shear fractures that formed in the middle and late Hercynian, the NW-trending shear fractures that formed in the Indosinian, and the NE- and EW-trending fractures that formed in the Yanshan–Himalayan (Li, 2019b). The closer to the fault zone, the more fractures that develop. Surface water or formation fluid migrates along the faults and fractures, increasing the contact with minerals and effectively improving the corrosion efficiency. Moreover, the faults cause the strata to be uplifted and weathered, which controls the thickness and distribution of the weathering crust reservoir. Figure 10 shows that the unfilled and half-filled fractures in the study area account for approximately 28% and 46% of the total number of fractures, respectively. These unfilled and half-filled fractures play an important role in the enrichment of oil and gas. The porosity and permeability of volcanic reservoirs in the study area are negatively correlated with the distance from the faults, indicating that the closer the volcanic rock is to the fault zone, the higher the porosity and permeability of the reservoir are, and the more favourable the area is for the development of high-quality reservoirs.
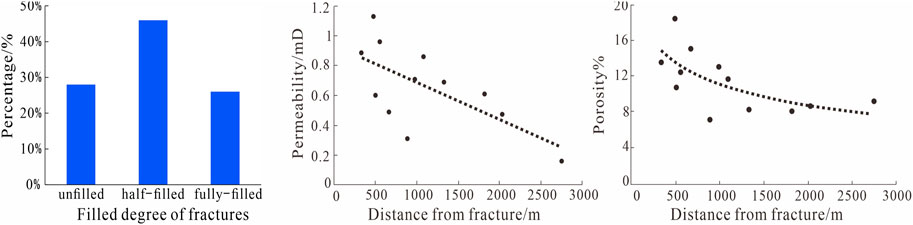
FIGURE 10. Fracture-filling characteristics and relationships between the porosity, permeability and distance from faults.
5.2 Modes of high-quality volcanic reservoir development
On the basis of identifying the main factors controlling high-quality volcanic reservoirs in the study area, a model for the development of high-quality volcanic reservoirs in the Carboniferous strata in the Shixi area is established (Figure 11). According to the different formation mechanisms, the reservoirs can be divided into weathering crust reservoirs and inner reservoirs. The reservoir space in the weathering crust reservoir is mainly composed of the secondary pores formed by weathering and corrosion. Due to the distance from the top of the Carboniferous strata, the weathering of the inner reservoirs is weak, and the reservoir space is composed of primary gas cavities and fractures.
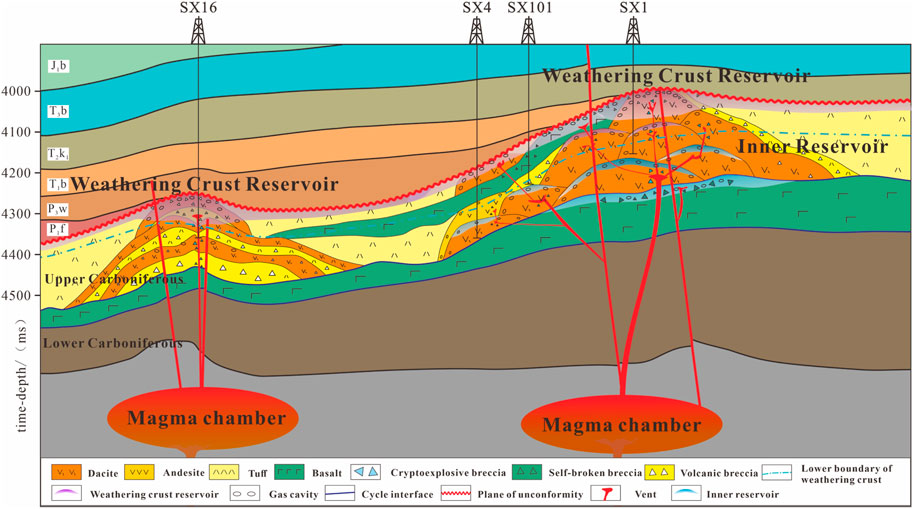
FIGURE 11. Model for the development of Carboniferous high-quality volcanic reservoirs in the study area.
Vertically, the weathering crust reservoir at the top of the Carboniferous strata underwent long-term weathering and corrosion, resulting in a large number of secondary corrosion pores. The weathering crust reservoir also developed a large number of structural fractures and weathering fractures, which not only improved the reservoir properties but also further promoted the corrosion of minerals. The inner reservoir is far from the top of the Carboniferous strata. The corrosion in the inner reservoir was relatively weak, and secondary corrosion pores were not developed, which improved the physical properties through gas cavities and structural fractures. Horizontally, favourable lithology and lithofacies, ancient landforms, and fractures are the most important controlling factors. The volcanic breccia of the pyroclastic flow subfacies of the explosive facies, the gas-cavity lava of the upper subfacies of the overflow facies and the cryptoexplosive breccia of the volcanic vent facies are more likely to contain high-quality reservoirs. Volcanic rocks located in uplifts and slopes are more likely to form high-quality weathering crust reservoirs. Volcanic rocks located in sags can also form high-quality inner reservoirs via primary gas cavities and fractures.
6 Conclusion
The Carboniferous strata in the Shixi area of the Junggar Basin mainly contains intermediate–acidic dacite, andesite and some volcanic breccia and tuff. The volcanic lithofacies are mainly the middle–lower subfacies of the intermediate–acidic overflow facies, followed by the air-fall subfacies and pyroclastic flow subfacies of the explosive facies. In terms of reservoir characteristics, the secondary corrosion pores in the volcanic rocks are the most developed, followed by the primary gas cavities in the upper subfacies of the overflow facies. In addition, a certain number of fractures are also present in volcanic rocks. Primary pores and fractures promote corrosion, and the association of corrosion pores with primary pores and fractures is common. Volcanic breccia, gas-cavity lava, and cryptoexplosive breccia are three types of high-quality volcanic reservoirs with good physical properties and pore structures in the Shixi area. Favourable lithology and lithofacies, weathering and corrosion, and fractures are the three main factors controlling the development of high-quality volcanic reservoirs. Volcanic breccia, gas-cavity lava, cryptoexplosive breccia and other favourable lithologies underwent weathering, corrosion and fracture transformation to form weathering crust reservoirs, and gas-cavity lava can contain inner reservoirs in the deep Carboniferous strata via primary gas cavities.
Data availability statement
The original contributions presented in the study are included in the article/Supplementary Material, further inquiries can be directed to the corresponding author.
Author contributions
AY and JZ is responsible for the idea, writing and revision of this paper. BB, HL, JW, and XW are responsible for the data analysis and drawing. YZ is responsible for provided research materials. LL and DS is responsible for reviewing and editing. All authors contributed to the article and approved the submitted version.
Funding
This paper is supported by the 14th Five-year Major Project of CNPC “Continental Deep and Ultra-Deep Oil and Gas Enrichment Law and Exploration Evaluation Research” (2021DJ0206) and China Petroleum Prospective Basic Technology Research Project “Marine Devonian-Carboniferous Oil and Gas Geology Research in Northern Xinjiang” (2022DJ0507).
Acknowledgments
The authors would like to thank the staff of the laboratories that performed the tests and analyses. We are also grateful to the editors and reviewers, whose comments improved the quality of this manuscript.
Conflict of interest
Authors AY, BB, HL, JW, XW, YZ, LL, and DS were employed by Xinjiang Oilfield Company.
The remaining authors declare that the research was conducted in the absence of any commercial or financial relationships that could be construed as a potential conflict of interest.
Publisher’s note
All claims expressed in this article are solely those of the authors and do not necessarily represent those of their affiliated organizations, or those of the publisher, the editors and the reviewers. Any product that may be evaluated in this article, or claim that may be made by its manufacturer, is not guaranteed or endorsed by the publisher.
References
Chen, L., Yang, Y., Wang, F., Lu, H., Zhang, Y., Wang, X., et al. (2020). Exploration history and enlightenment of Junggar Basin. Xinjiang Pet. Geol. 41, 505–518. doi:10.7657/XJPG20200501
Chen, G., Li, T., Yang, L., Zhang, G., Li, J., and Dong, H. (2021). Mechanical properties and failure mechanism of combined bodies with different coal-rock ratios and combinations. J. Min. Strata Control Eng. 3 (2), 023522. doi:10.13532/j.jmsce.cn10-1638/td.20210108.001
Fan, C., Li, H., Qin, Q., Shang, L., Yuan, Y., and Li, Z. (2020). formation mechanisms and distribution of weathered volcanic reservoirs: A case study of the carboniferous volcanic rocks in northwest Junggar Basin, China. Energy Sci. Eng. 8 (8), 2841–2858. doi:10.1002/ese3.702
Feng, Z., Huo, Q., Wang, X., Zeng, H., and Fu, L. (2015). Organic geochemical characteristics and paleosedimentary environment of source rocks in the first member of Qingshankou Formation. Petroleum Geol. Oilfield Dev. Daqing 34, 1–7. doi:10.3969/J.ISSN.1000-3754.2015.04.001
Gao, Y., Li, S., Ba, Z., Mirenisha, T., Zhou, H., Xu, C., et al. (2021). Oral administration of Bacillus subtilis subunit vaccine significantly enhances the immune protection of grass carp against GCRV-II infection. Petroleum Geol. Oilfield Dev. Daqing 40, 30–41. doi:10.3390/v14010030
Guo, H., Ji, M., Sun, Z., and Zhou, Z. (2021). Energy evolution characteristics of red sandstone under cyclic load. J. Min. Strata Control Eng. 3 (4), 043019. doi:10.13532/j.jmsce.cn10-1638/td.20211008.001
Guo, L., Zhou, D., Zhang, D., and Zhou, B. (2021). Deformation and failure of surrounding rock of a roadway subjected to mining-induced stresses. J. Min. Strata Control Eng. 3 (2), 023038. doi:10.13532/j.jmsce.cn10-1638/td.20200727.001
He, X., Liu, Y., Xu, X., Liu, B., and Zhang, S. (2007). Controlling factors of Carboniferous volcanic reservoirs and favorable reservoir prediction in Xi Quan area, Junggar Basin. Lithol. Reserv. 29, 42–51. doi:10.3969/j.issn.1673-8926.2017.03.006
Hirschmann, M. M., and Cottrell, E. (2017). Effect of pressure on Fe3+/Sigma Fe ratio in a mafic magma and consequences for magma ocean redox gradients. Geochimica Cosmochimica Acta J. Geochem. Soc. Meteorit. Soc. 204, 83–103. doi:10.1016/j.gca.2017.01.023
Lan, S., Song, D., Li, Z., and Liu, Y. (2021). Experimental study on acoustic emission characteristics of fault slip process based on damage factor. J. Min. Strata Control Eng. 3 (3), 033024. doi:10.13532/j.jmsce.cn10-1638/td.20210510.002
Li, W., He, S., Tan, K., Zhang, F., and Zhao, Y. (2010). New perspective for phage display as an efficient and versatile technology of functional proteomics. Nat. Gas. Geosci. 21, 909–919. doi:10.1007/s00253-009-2277-0
Li, H., Qin, Q., Li, Z., Fan, C., Zhong, K., Li, Z., et al. (2017). Characteristics and distribution of cracks in Carboniferous buried volcanic reservoirs of the Shixi oilfield. Geol. Explor. 53 (6), 1219–1228. doi:10.13712/j.cnki.dzykt.2017.06.018
Li, A., Su, S., Li, X., Zhang, J., Jing, C., and Zhao, Z. (2022). Effects of organic matter graphitization on shale gas accumulation in the lower paleozoic longmaxi formation from the changning area, southern sichuan basin. Lithosphere 12, 7102509. doi:10.2113/2022/7102509
Li, H., Li, J., Xu, X. Y., Yang, G. X., Wang, Z. P., Xu, Q., et al. (2021). Petrogenesis and tectonic implications of alkali basalts in kalamaili area, east junggar, Xinjiang (NW China): Constraints from petrology, geochronology and geochemistry. Acta Geol. Sin. 95 (11), 3282–3300. doi:10.19762/j.cnki.dizhixuebao.2020281
Li, S. B., Guo, X. G., Zheng, M. L., Wang, Z. S., and Liu, X. L. (2021). Lithology identification of Carboniferous volcanic rocks in Xiquan area, eastern Junggar Basin. Lithol. Reserv. 33 (1), 258–266. doi:10.12108/yxyqc.20210124
Li, Z. (2019a). Study on reservoir characteristics of Carboniferous volcanic weathered crust reservoir in Shixi oilfield, Junggar abdomen. Chengdu: Southwest Petroleum University. doi:10.27420/d.cnki.gxsyc.2019.000185
Li, Y. X. (2019b). Fracture characteristics and prediction of carboniferous volcanic rocks in Shixi Oilfield, Junggar Basin. Chengdu: Southwest Petroleum University. doi:10.27420/d.cnki.gxsyc.2019.000738
Liu, X., Wang, Q., Dai, L., Liu, S., and Hao, Y. (2020). Reservoir characteristics and formation mechanisms of lacustrine Carbonate and volcanics mixing sediments, Laizhouwan Sag. Earth Sci. 45, 3579–3588. doi:10.3799/dqkx.2020.119
Lollar, B. S., Onstott, T. C., Lamcrampe-Couloume, G., and Ballentine, C. J. (2014). The contribution of the Precambrian continental lithosphere to global H2 production. Nature 516 (7531), 379–382. doi:10.1038/nature14017
Ma, S., Chen, C., Luo, J., Wei, L., Liu, Y., Dai, J., et al. (2019). Research of major controlling factors on favorable reservoir of the Carboniferous volcanic rocks in Xi Quan area, Junggar Basin. Geol. J. China Univ. 25, 197–205. doi:10.16108/j.issn1006-7493.2018087
Mao, Z., Zhu, R., Luo, J., Wang, J., Du, Z., Su, L., et al. (2015). Reservoir characteristics, formation mechanisms and petroleum exploration potential of volcanic rocks in China. Petroleum Sci. 12, 54–66. doi:10.1007/S12182-014-0013-6
Melnik, O. E., Barmin, A. A., and Sparks, R. S. J. (2005). Dynamics of magma flow inside volcanic conduits with bubble overpressure buildup and gas loss through permeable magma. J. Volcanol. Geotherm. Res. 143, 53–68. doi:10.1016/j.jvolgeores.2004.09.010
Meng, Q., Jin, Z., Sun, D., Liu, Q., Zhu, D., Liu, J., et al. (2021). Geological background and exploration prospects for the occurrence of high-content hydrogen. Pet. Geol. Exp. 43 (02), 208–216. doi:10.11781/sysydz202102208
Nazari, S. M., and Riahi, M. A. (2020). Geomechanical unit modeling using seismic and well log data in one of the southwestern Iranian oilfields. Pet. Explor. Prod. Technol. 10 (7), 2805–2813. doi:10.1007/s13202-020-00962-w
Patricia, S., and Nora, R. (2007). Processes controlling porosity and permeability in volcanic reservoirs from the Austral and Neuquen basins, Argentina. AAPG Bull. 9, 115–129. doi:10.1306/08290605173
Pearce, J. A., Harris, N. B. W., and Tindle, A. G. (1984). Trace element discrimination diagrams for the tectonic interpretation of granitic rocks. Petro 25, 956–983. doi:10.1093/petrology/25.4.956
Qin, X., Shi, Y., Wu, W., Kou, Y., Wang, L., Li, X., et al. (2012). Controlling factors of carboniferous volcanic reservoirs in Junggar Basin. Acta Sci. Nat. Univ. Pekin. 48, 54–60. doi:10.13209/j.0479-8023.2012.009
Qin, Z., Wei, P., and Zhang, S. (2016). The lithofacies of Carboniferous volcanic rocks in Dixi-Wucaiwan area. J. Southwest Petroleum Univ. Sci. Technol. Ed. 38, 9–21. doi:10.11885/j.issn.16745086.2014.12.07.04
Qu, J., Gao, C., Cha, M., and Zhang, Y. (2014). Eye and vision (E & V): The critical link between eye and vision. J. China Univ. Petroleum Ed. Nat. Sci. 38, 1–8. doi:10.1186/s40662-014-0001-3
Quanei, S. L., and Russell, K. (2003). Rock strength as a metric of welding intensity in pyroclastic deposits. Eur. J. Mineralogy 15, 855–864. doi:10.1127/0935-1221/2003/0015-0855
Rimmer, S. M. (2004). Geochemical paleoredox indicators in devonian-mississippian black shales, central appalachian basin (USA). Chem. Geol. 206, 373–391. doi:10.1016/j.chemgeo.2003.12.029
Shan, X. L., Chen, Y. P., Tang, L. M., and Yi, J. (2011). Comprehensive evaluation method for volcanic rock reservoirs and its application: Taking songnan gas field for example. J. Shandong Univ. Sci. Technol. 30 (3), 1–6. doi:10.16452/j.cnki.sdkjzk.2011.03.001
Sruoga, P., and Rubinstein, N. (2007). Processes controlling porosity and permeability in volcanic reservoirs from the Austral and Neuquen basins, Argentina. AAPG Bull. 91, 115–129. doi:10.1306/08290605173
Su, P., Qin, Q., Yuan, Y., and Jiang, F. (2011). Characteristics of volcanic reservoir fractures in upper wall of Hong Che fault belt. Xinjiang Pet. Geol. 32, 457–460. CNKI:SUN:XJSD.0.2011-05-005.
Sun, J., Xue, J., Hou, G., Wu, A., Song, M., and Zhu, F. (2019). Sedimentary characteristics and models of sandy clastic flow in lacustrine depression: A case study of jurassic Sangonghe Formation in bun-1 jing Xi depression, Junggar Basin. J. China Univ. Min. 13, 858–869. doi:10.13247/j.cnki.jcumt.000973
Wang, J., and Wang, X. (2021). Seepage characteristic and fracture development of protected seam caused by mining protecting strata. J. Min. Strata Control Eng. 3 (3), 033511. doi:10.13532/j.jmsce.cn10-1638/td.20201215.001
Wang, P., Chi, Y., and Liu, W. (2003). Volcanic facies in Songliao Basin: Class type, characteristics and reservoir significance. J. Jilin Univ. (Earth Sci. Ed. 33, 449–456. doi:10.13278/j.cnki.jjuese.2003.04.011
Wang, W., Gao, B., Wei, P., Pan, J., Li, F., and Yi, Z. (2012). Research on volcanic reservoirs characteristics and oil and gas accumulation model. Prog. Geophys. 27, 2478–2491. doi:10.6038/j.issn.1004-2903.2012.06.023
Wang, L., Xu, Y., Zhang, Y., Liu, Z., and Shang, F. (2020). Predominant factors and development mode of carboniferous reservoirs in chepaizi uplift, Junggar Basin. J. Northeast Petroleum Univ. 44, 79–90. doi:10.3969/j.issn.2095-4107.2020.02.008
Wang, H., Lin, C., Jiang, F., Wang, J., Zhao, Y. H., and Xing, B. (2021). Risk factors for early dislocation of the hip after periacetabular tumour resection and endoprosthetic reconstruction of the hemipelvis, Songliao Basin. Glob. Geol. 40 (2), 382–390. doi:10.3969/j.issn.1004-5589.2021.02.015
Wignall, P. B., and Twitchett, R. J. (1996). Oceanic anoxia and the end Permian mass extinction. Science 272, 1155–1158. doi:10.1126/science.272.5265.1155
Wrihgt, J., Schrader, H., and Holser, W. T. (1987). Paleoredox variations in ancient oceans recorded by rare Earth elements in fossil apatite. Geochimica Cosmochimica Acta 51, 631–644. doi:10.1016/0016-7037(87)90075-5
Xue, F., Liu, X., and Wang, T. (2021). Research on anchoring effect of jointed rock mass based on 3D printing and digital speckle technology. J. Min. Strata Control Eng. 3 (2), 023013. doi:10.13532/j.jmsce.cn10-1638/td.20201020.001
Yuan, X., Li, W., Dong, H., Wang, T., Zhang, B., and Li, S. (2015). Characteristics and controlling factors of Permian volcanic reservoirs in Kebai area. Fault-Block Oil Gas Field 22, 445–449. doi:10.6056/dkyqt201504008
Zhang, F., Hou, Y., Zhu, M., Cui, W., Hu, T., Chen, G., et al. (2016). Current status and development trend of volcanic reservoir logging evaluation technology. Prog. Geophys. 31, 1732–1751. doi:10.6038/pg20160443
Zhang, J., Wang, Z., and Zhao, J. (2010). Study on igneous reservoir characteristics and oil and gas accumulation law in Shixi oilfield, Junggar Basin. Gas Explor. Dev. 33, 1–6. doi:10.12017/dzkx.2022.041
Keywords: Junggar Basin, Carboniferous, volcanic reservoirs, main controlling factors, development modes
Citation: Yiming A, Bian B, Liu H, Wang J, Wang X, Zhu J, Zhu Y, Liu L and Su D (2023) Development characteristics and main controlling factors of Carboniferous volcanic reservoirs in the Shixi area, Junggar Basin. Front. Earth Sci. 11:1185213. doi: 10.3389/feart.2023.1185213
Received: 13 March 2023; Accepted: 15 May 2023;
Published: 02 June 2023.
Edited by:
Shuai Yin, Xi’an Shiyou University, ChinaReviewed by:
Saipeng Huang, University of Barcelona, SpainJianhua He, Chengdu University of Technology, China
Copyright © 2023 Yiming, Bian, Liu, Wang, Wang, Zhu, Zhu, Liu and Su. This is an open-access article distributed under the terms of the Creative Commons Attribution License (CC BY). The use, distribution or reproduction in other forums is permitted, provided the original author(s) and the copyright owner(s) are credited and that the original publication in this journal is cited, in accordance with accepted academic practice. No use, distribution or reproduction is permitted which does not comply with these terms.
*Correspondence: Junyong Zhu, emh1ankyMkBtYWlscy5qbHUuZWR1LmNu