- 1Department of Archaeology, Conservation and History, University of Oslo, Oslo, Norway
- 2State Key Laboratory of Loess and Quaternary Geology, Institute of Earth Environment, Chinese Academy of Sciences, Xi’an, China
- 3Coordenação de Ciências Humanas, Museu Paraense Emílio Goeldi, Belém, Brazil
- 4Soil Geography and Landscape Group, Netherlands Centre for Luminescence Dating, Environmental Sciences Group, University of Wageningen, Wageningen, Netherlands
- 5Research Center for Geochronology and Isotope Analysis, Korea Basic Science Institute, Chungbuk, Republic of Korea
- 6Department of Anthropology, University of Louisville, Louisville, KY, United States
- 7Center for Archaeology and Cultural Heritage, University of Louisville, Louisville, KY, United States
- 8Museum of Archaeology and Ethnography, University of São Paulo, São Paulo, Brazil
- 9Department of Anthropology, University of Florida, Gainesville, FL, United States
The Amazon Basin currently hosts the world’s largest pool of terrestrial biodiversity and prior to European colonization of the Americas there were large human communities living in parkland ecosystems. We examine the formation of archaeological sites in the northeast sector of the Caxiuanã National Forest (CNF) using light stable isotopes of nitrogen and carbon, total carbon and nitrogen and Optically Stimulated Luminescence to characterize long-term human landscape management practices. Previous research in the CNF has documented differences in pH, calcium, total organic carbon (TOC) and nitrogen (TN) between terra preta and terra marrom contexts as well as different forest structures based on remote sensing analysis. Therefore, we adopt a comparative approach, examining the formation processes of on-site (terra preta), near-site (terra marrom) and offsite (latosol) contexts. TOC and TN values obtained in our study augment and support previous research demonstrating significantly higher on-site values relative to near-site and off-site. However, the stable isotopes (δ13C, δ15N) assayed from terra preta, terra marrom and latosols show statistically overlapping values, indicating the persistence of closed canopy in off-site and near-site contexts and the use of this canopy in the formation of on-site soils (terra preta). Our results corroborate the hypothesis that closed canopy ecosystems and human settlements persisted in the Amazon for thousands of years and formed the foundation of the region’s rich biodiversity.
1 Introduction
The relationship between so-called terra preta do Índio (a.k.a. Amazonian Dark Earths) and ancient Amazonian cultures has been one of the lynchpin research topics of the research program of Historical Ecology for more than 30 years (Balée, 2006; Erickson, 2008; Szabó, 2015). While terra preta is specifically associated with archaeological deposits, studies have shown that the effects of soil amendments extend beyond middens into the surrounding areas (Fraser et al., 2011; Glaser and Birk, 2012; Schmidt et al., 2014; Kern et al., 2017; WinklerPrins and Levis, 2021). These contexts have been called terra marrom (a.k.a. terra mulata or Amazon Brown Earth), which are anthrosols generally devoid of artifacts surrounding terra preta sites with a gradient of melanization relative to the soils interpreted as not being directly modified by humans, called terra firme (uplands) and várzea (floodplains) (Sombroek, 1966; Denevan, 2004; Fraser et al., 2011; Alho et al., 2019). Studies have shown that terra marrom formed in areas as a response to deliberate burning and polyculture agroforestry (Costa et al., 2013; Arroyo-Kalin, 2014; Iriarte et al., 2020; Maezumi et al., 2022). Typically, soils that form outside anthropogenic areas are latosols, which are products of deep tropical weathering, iron- and aluminum oxyhydroxides-rich soils corresponding to Oxisols in the United States Department of Agriculture (USDA) classification and Ferralsols in the World Reference Base of the Food and Agriculture Association (Schaefer et al., 2008).
However, there is no concrete, uncontroversial definition of any of these soil or geographic categories—they tend to be applied interpretively in the field although broad geochemical differences among them have been characterized in relation to nutrient availability for plants (Asare, 2022). Questions remain on the degree to which terra preta formation increases net primary productivity (NPP) and sequestration of carbon and nitrogen in affected soils (Downie et al., 2011; Doughty et al., 2014; Clark et al., 2017). Soil phenotype has been shown in at least one case from the Bolivian Lowlands to be spatially non-correlated with the growth of vegetation that is economically useful for humans, which is attributed instead to natural post-abandonment disturbance processes (Paz-Rivera and Putz, 2009; see also; Piperno et al., 2019). On the other hand, a ground-truthed, remote sensing study of Caxiuanã National Forest (CNF) showed marked differences in vegetation structure as measured by a green index in anthropogenic forests correlated with anthropic soils as compared to non-managed areas (Choi et al., 2020). Similar results have also been obtained by Robinson et al. (2021). Söderström et al. (2016) use remote sensing to estimate ADE occurrence in approximately 3% of a 256 km2 study area on the Belterra Plateau, 30 km south of the city of Santarém, which correlates to McMichael et al.’s (2014) estimate for ADE across the entire Amazon Basin (see also Thayn et al., 2011). Geochemical methods using soil stable isotopes have potential to help discriminate between how soils form within the context of different human manipulated plant communities (Dawson et al., 2002; Crotty et al., 2012; Nordt and Holliday, 2020), yet few studies to date have systematically applied them to studying terra preta formation (cf., Desjardins et al., 1996; Pessenda et al., 1997; Robinson et al., 2021).
This study adds to the growing body of knowledge of anthropic soil formation in the Amazon by analyzing light stable isotopes of carbon (δ13C) and nitrogen (δ15N) and associated total concentrations of the elements as well as soil mixing from on-site (terra preta), near-site (terra marrom) and off-site (latosol) areas using optically stimulated luminescence (OSL) surrounding three archaeological sites settled over the last 2000 years in the CNF of northern Brazil (Figure 1). We tested the hypothesis that soil organic matter would possess statistically different properties from these three archaeological and edaphic contexts. Despite differences between on-site and off-site contexts in soil nutrient availability and pH, enrichment of nitrogen within terra preta relative to surrounding areas (Kern, 1996; Lemos et al., 2011; Martins da Silva et al., 2017), there are statistically overlapping δ13C and δ15N values, indicating the persistence of a closed canopy C3 forest throughout the various contexts tested and limited influence of human land management on the isotopic composition of soils from this study area. We contextualize our study within the scope of previous work done in the CNF and propose methods for developing deeper understandings of soil formation, stable isotopes and vegetation patterns in the Amazon in future research.
2 Background
2.1 Physical setting of the project area
The study area is located in CNF in Pará State, Brazil (Figure 1). Precipitation averages 2,300 mm per year with an annual mean temperature of 25.7°C (Sotta et al., 2006). Approximately 75% of the total rainfall occurs between December and June associated with the passage of the Intertropical Convergence Zone, which advects warm equatorial Atlantic moisture inland over regions including the CNF (de Souza et al., 2005; Santos et al., 2015). This portion of Brazil is naturally covered in dense stands of southern neotropical lowland rainforest growing along numerous tributaries of the Amazon River, although deforestation has been pervasive since the 1970s with on-and-off efforts to prevent clear cutting for cattle ranching (Moran, 1993; Fearnside, 2005; Fonseca et al., 2022). The CNF has protected status, which has sheltered it from deforestation. In 2020 when deforestation across the Brazilian Amazon was rampant (Silva Junior et al., 2021), the estimated total loss of CNF’s forest cover was 0.07% (2.32 km2) (Pellin et al., 2022). CNF has been a managed forest landscape for at least 3,000 years, therefore never “pristine” sensu stricto (Clement and Junqueira, 2010), but its status as a protected area has buffered it from clear cutting and significant degrees of disturbance that has beset many of the surrounding areas in recent decades.
Tropical soils of the Amazon Basin have been previously found to be naturally high in iron (Fe), manganese (Mn), aluminum (Al) oxyhydroxide minerals, while low in pH, magnesium (Mg), calcium (Ca), phosphorus (P) and total organic carbon (TOC) (Schmidt et al., 2014; Kern et al., 2017; Macedo et al., 2017; Lombardo et al., 2022). This is due to ancient, weathered iron-rich mineral bedrock substrates that occur in the eastern lowlands and are generally poor in nutrients available for plant metabolism. Within this environment, free metal cations such as Fe3+ and Al3+ react easily with phosphates, which fix P into the soil, making it insoluble and therefore unavailable for uptake by plant roots (Johnson and Loeppert, 2006). Such edaphic conditions have been hypothesized as promoting the growth of tall-canopy forests, which have low metabolic requirements compared to grass-dominated landscapes that thrive in soils with more available nutrients (Bell, 1982). On the other hand, terra preta soils are rich in TOC, P, N, Ca, Mg and potassium (K) from human excrement and faunal diagenetic derivatives as well as biochar non-or partially combusted ash (Glaser, 2007). Previous comparative studies of on-site and off-site contexts in Caxiuanã have similarly demonstrated significant differences in bulk geochemistry with terra preta sites hosting soils with higher TOC, P, C, Ca, Mg, Mn, Zn and bioavailable microminerals than surrounding areas (Kern, 1996; Lemos et al., 2011; Martins da Silva et al., 2017).
2.2 Archaeological setting of the CNF
The ceramics found in the Caxiuanã region include different styles and complexes that relate to other areas of Amazonia, signifying that this region was connected to extensive networks during pre-colonial times, although most production was cerca situ (Hofman et al., 2021). Inhabitation of the Caxiuanã region is previously known to extend >2000 years based on thermoluminescence dating of pottery (Behling and da Costa, 2000; Coirolo and D'Aquino, 2005). A sediment core from the Rio Curuá within Caxiuanã has an increase in charcoal content postdating 2,500 years BP, indicative of a human presence in the region from this time on due to the fact that concentrations are between 3-15x higher after this date than in the previous 5,300 years (Behling and da Costa, 2000).
Evans and Meggers. (1960) identified four Amazonian ceramic horizons–Zone-Hachured, Incised Rim, Amazonian Polychrome, Incised-Punctate. The Incised Rim is associated with variably named complexes, such as incised, modelled and bi-chrome, such as the Saladoid-Barrancoid series, as well as similar developments in the central Amazon (Neves, 2022). The polychrome tradition is said to last from 1,500 to 500 BP. The most notable site are associated with this ceramic horizon is Marajó Island at the mouth of the Amazon (Schaan, 2004). The polychrome stylistic elements are currently known to date earliest in Marajó Island and expand westward and continue for over a millennium and into the historic contact period in many areas. Finally, the most recent in ceramic horizons are the Incised Punctate tradition, which includes Santarem and Konduri along the Amazon and Koriabo in the Lower Amazon and Guianas. This ceramic type extended beyond contact period (1,000 to 250 BP) (Rocha, 2020). The Incised Rim Tradition is related to the first large-scale population spread into, sedentism within and anthropization of Amazonia before 2000 cal years BP (Lima, 2008; Neves et al., 2014) (de Souza et al., 2020). Incised Rim origins are posited as the Central Amazon dating to 3,000–3500 BP until 400BP, in some cases. However, Lima (2008) suggests 2,500–2000 BP. More recent sites contain Koriabo tradition ceramics, which are associated with a cultural complex that probably originated in the Guianas approximately 750 cal years BP (Rostain, 2008). These finds connect the Guianas with the Caribbean and lower Amazon during the late pre-Columbian period (Barreto and Lima, 2021). Finally, a cemetery site excavated in the 1990s featured urns buried in an earth mound (teso) with characteristics of the Marajoara style (Lisboa et al., 2013). The presence of diverse ceramic style and complexes that relate to other areas of Amazonia, signify this region was connected to extensive networks during pre-colonial times.
A total of 32 archaeological sites in Caxiuanã have been registered as of 2023, the majority of which are located within terra preta. Sites are located in higher bluffs adjacent to large waterways. Although there are many sites in the area, only a small number have undergone extensive research. Current investigations in CNF are uncovering pottery of the Incised Rim Tradition, also known as Amazonian Barrancoid, that is related to the first large-scale population spread into, sedentism within and anthropization of Amazonia before 2000 cal years BP (Lima, 2008; Neves et al., 2014; de Souza et al., 2020). Ceramic griddles recovered in excavations demonstrate that a tuber-based diet was prevalent in CNF around 2000 cal years BP (McDaniel, 2023). More recent sites contain Koriabo tradition ceramics, which are associated with a cultural complex that probably originated in the Guianas approximately 750 cal years BP (Rostain, 2008). These finds connect the Guianas with the Caribbean and lower Amazon during the late pre-Columbian period (Barreto et al., 2021). Finally, a cemetery site excavated in the 1990s featured urns buried in an earth mound (teso) with characteristics of the Marajoara style (Lisboa et al., 2013). In general, CNF archaeological sites are found near seasonally inundated forest terrain (igapó) (Figure 1). In these igapó settings there are two important native Amazonian alimentary palms species–açai (Euterpe oleracea) and buriti (Mauritia flexuosa) as well as a host of other trees consumed by human communities (Lisboa et al., 2013).
Based on population modeling of radiocarbon ages, the wetter lower Amazon River basin is thought to have been more sparsely populated than other regions prior to the introduction of formal cultivation techniques although data points in this region of their study are comparably low (McMichael and Bush, 2019). In general, relatively sparse quantities of charcoal found in the coring sample obtained from the Curuá River, located 300 km to the west of CNF, have provided the basis for inferring low ancient population densities in places like Caxiuanã relative to other regions (Behling and da Costa, 2000). Smaller tributaries such as the Curuá are thought to host smaller settlements relative to larger waterways in the Amazon (Levis et al., 2014). However, a predictive spatial model of the occurrence of terra preta based on high-resolution (15-m) remote sensing strongly suggests undersampling of archaeological sites in Caxiuanã (Choi et al., 2020), which may be the product of the relative remoteness of the location to modern settlements and infrastructure.
Today, the CNF is managed by the Serviço Florestal Brasileiro (Brazilian Forest Service) and hosts riberinhos (riverine peoples) who practice agroforestry to manage stands of açai, Brazil nuts (Bertholletia excelsa) and other palm and fruit trees and utilize slash-and-burn agriculture to raise bitter manioc (Manihot esculenta) and other starches. According to the Unidades de Conservação there were 452 residents in 2018 within the 200 km2 reserve (https://uc.socioambiental.org/arp/640, accessed 13 February 2023). Since the study region is a sparsely populated, protected area, anthropic pressures such as deforestation, mining and cattle grazing are relatively low. One of the focal sites of this study, Ibama, is the location of a research station of the Instituto Brasileiro do Meio Ambiente e dos Recursos Naturais Renováveis and has been cleared and planted with turf. The study of anthropogenic impacts to landscape formation are therefore processually linked to both ancient and modern land use and extensive forms of modern-day land use are considered as features that potentially overprint pre-colonial features (Choi et al., 2020).
2.3 Stable isotope reconstruction in soils
The application of light stable isotopes for understanding the formation of soils can inform past land cover conditions, providing additional data to compliment other geochemical analyses. Photosynthesis is the process in plants whereby CO2, H2O and sunlight are converted into sucrose and glucose (carbohydrates). In tropical plants, photosynthesis follows one of two pathways: trees in closed canopy environments reduce and fix atmospheric CO2 to a three-carbon molecule (C3) to create carbohydrates whereas some grasses and sedges follow a more complicated (Hatch-Slack) pathway that fixes a four-carbon molecule (C4) to create the sugar. C3 plants discriminate more heavily than C4 plants against 13C (which generally occurs at a ratio of 1:99 relative to the more abundant 12C isotope) during carbon fixation. On average, C3 plants in the pre-Industrial era had a12CO2:13CO2 ratio of −26‰ relative to the Vienna Pee Dee Belemnite (VPDB) standard, whereas C4 plants had a ratio of −12‰ (Kohn, 2010). In situ decomposition of plant organic matter enriches 13C on average of ∼1‰ (Natelhoffer and Fry, 1988), so the 13C isotopic composition of soils with C3 and C4 plant cover averages −25‰ and −11‰, respectively (Ambrose and Sikes, 1991). It is estimated that approximately 60% of the grasses from Brazil follow the C4 photosynthetic pathway (Medina et al., 1999), although there is great spatial variance to their distribution. A longitudinal study of 13C from the Triunfo site in the Iténez Forest of the Bolivian Amazon shows relative isotopic enrichment of on-site (terra preta) soils compared to off-site settings, which is interpreted as a higher contribution of C4 vegetation in the formation of the soils (Robinson et al., 2021). However, isotopically depleted CO2 is susceptible to “re-fixation” by plants under closed forest canopies (Sternberg et al., 1997), providing an additional mechanistic causal link between low 13C values and denser stands of tree cover. Additionally, aquatic fauna have more strongly negative 13C values than terrestrial fauna (Villagran, 2014; Hermenegildo et al., 2017), so if the soil is amended with the decaying remains of aquatic animals or their byproducts (including feces of people eating aquatic animals), the 13C values of the soils may be lower than in soils where only terrestrial matter occurs.
The second isotope commonly studied to understand an edaphic environment is 15N. The stable isotopic composition of nitrogen (14N:15N) in the atmosphere occurs at a ratio of 273:1. During plant decomposition, the heavier 15N isotope remains in the soil preferentially to the lighter 14N isotope. Higher precipitation and temperature regimes tend to have lower 15N values than cool and arid ecosystems (Sachs, 2009). Human-amended (anthropogenic) soils tend to have higher ratios than non-amended soils (Commisso and Nelson, 2006). Within the context of the Amazon Rainforest, which has relatively stable rainfall and temperature regime, higher δ15N relative to the mean atmospheric ratio of 14N:15N can be interpreted as evidence of a stronger anthropogenic signature, although this assumption has yet to be longitudinally tested.
3 Materials and methods
This study analyzes the stable isotopic composition and formation processes of terra preta soil relative to surrounding edaphic settings by diachronically examining horizontal-scale ecologies through the soils in CNF. We contextualize datasets within the broader archaeology of the Lower Amazon River and to develop more robust frameworks of ancient indigenous regional land management practices. The specific details of the archaeological investigations are beyond the scope of the present manuscript and will be published later—here, we focus on the recovery and analyses of sediments for the purpose of reconstructing the stable isotope and formation ecology of the areas of CNF under study.
Samples for stable isotopes, radiocarbon (14C) and Optically Stimulated Luminescence (OSL) were collected from a total of 20 soil pits (Figure 2) in 2016 and 2017 following documentation of each unit’s soil profile (Supplementary Material S1). We separated our collection zones into terra preta (on-site), terra marrom (near-site) and latosol (off-site) contexts (Kern et al., 2017). Stable isotope samples were placed into 133 mL Whirlpaks using a trowel or knife, avoiding contact with human hands. All samples were emptied into aluminum trays within 12 h of collection and dried in a convection oven at 60°C for >12 h prior to repacking. Samples were checked daily to ensure that humidity did not accumulate in the sample bags and were periodically redried if there was moisture spotted in the bags. Following the conclusion of fieldwork, all samples were transported to South Korea for analysis where they were submerged in 1M HCl for >24 h on a shaker table (1000 RPM). Samples were then rinsed with distilled water, re-agitated then decanted to remove HCl. Samples were then dried in a gravity oven at 70°C overnight after which they were homogenized with a glass rod and mortar after which 10–25 mg of sediment were weighed on a mass balance and loaded into tin capsules for analysis.
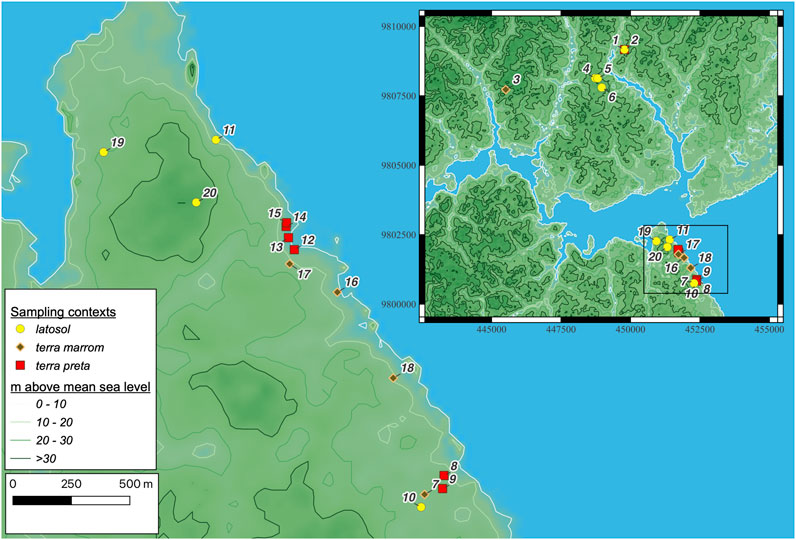
FIGURE 2. Map of the sampling locations (UTM 22S, WGS 1984). Digital elevation model rendered from MERIT Hydro (Yamazaki et al., 2019). List of collection sites correspond to data keys in Supplementary Material S1: (1) CAX1, (2) CAX2, (3) CAX3, (4) ECFP1, (5) ECFP2, (6) ECFP3, (7) FOR1, (8) FOR2/Forte site/PA-GU-07, (9) FOR2-2FOR2-2, (10) FOR3, (11) IBA1, (12) IBA2/Ibama site/PA-GU-06, (13), IBA2-2, (14) IBA2-3, (15) IBA2-4, (16) IBA3, (17) IBA4, (18) IBA5, (19) IBA6, (20) IBA7. Terra preta sites are underlined.
Prepared samples were analyzed with a stable isotope ratio mass spectrometer linked to an elemental analyzer (EA-IRMS) (VisION, Isoprime Ltd., Cheadle Hulme, United Kingdom) at National Instrumentation Center for Environmental Management (NICEM), Seoul National University, South Korea. Following combustion of the samples into a gaseous state (CO2 and N2), isotope ratios were determined as differences in parts per mille (‰) from standard materials (VPDB marine limestone for 13C and atmospheric nitrogen [AIR] for 15N) as follows: δ13C or δ15N (‰) = [(Rsample - Rstandard)/Rstandard] × 103, where R is (13C/12C) or (15N/14N). NICEM reports that the laboratory errors of the mass spectrometer used in this study are <0.1‰ and <0.2‰, respectively.
The use of Bayesian bivariate ellipses to understand distributions of stable isotopes is increasingly common (e.g., Pingram et al., 2020; Rey-Iglesia et al., 2021) and utilizes known data points to project unknown distributions within the sampling context. Therefore, statistical treatment of the data include standard ellipses calculated to the 2-σ (95%) level, box and whiskers plots performed in ggplot2 in RStudio 2022.07.1 (Build 554). To put our results into a testable framework, we also conducted t-tests and ANOVA tests of the data from identified edaphic contexts as well as by depth and soil horizonation. Code and data are available at https://doi.org/10.6084/m9.figshare.22094153.
Samples for accelerator mass spectrometry (AMS) radiocarbon dating were taken from soil profiles, preferentially selecting mollusks or charcoal from archaeological features. Samples were analyzed at the Korea Institute of Geoscience and Mineral Resources following a standard acid-base-acid pretreatment for removal of contaminants. All samples are reported in years before present (BP) from AD 1950 calculated using the Libby half-life of 5,568 years and calibrated using the atmospheric correction supplied in Reimer et al. (2020) in OxCal4.4 (https://c14.arch.ox.ac.uk/oxcal.html).
Additional geochronometry of sediment deposition of various edaphic contexts was provided using OSL dating at the Korea Basic Science Institute, Ochang. In the field, light-free high-carbon steel pipes measuring 3 × 20 cm were pounded into the profile walls of test pits. For laboratory preparation of OSL samples, approximately 3–4 cm of both ends of the pipes were removed, since they have the possibility of being exposed to sunlight during sample collection. The removed parts were used to determine the dose rate (Dr), which was measured by low-level, high-resolution gamma spectrometry. Then 32–250 μm fraction sediment grains were extracted from the sampled sediments by sieving the remaining fraction in a semi-darkened room using orange-red light. The separated grains were cleaned in 10% H2O2 to remove organic material and 10% HCl to remove carbonate minerals. After chemical cleansing, grains with a specific gravity between 2.62 g cm-3 and 2.75 g cm-3 were separated using sodium polytungstate. Of the collected grains, non-quartz was dissolved, and quartz grains were etched by approximately 10 μm by subjecting the sample to hydrofluoric acid for 45 min. The quartz grains were then made into an aliquot consisting of several thousand grains by fixing them to a stainless-steel disc with silicone spray. The OSL was measured using a Risø TL/OSL-DA-20 series reader using blue LEDs applying energy up to 80 mW/cm2.
The dating process followed the Single-Aliquot Regenerative (SAR) dose protocol introduced by Murray and Wintle (2000). The SAR protocol was adopted since it provides an effective way to monitor sensitivity changes in quartz grains during the analysis (Murray and Wintle, 2003; Choi et al., 2004). In the first routine (i=0), which measures the natural OSL signal, laboratory (artificial) dose is not administered. After preheating (step 2; 240°C or 260°C for 10 s), the natural OSL signal, L0, was measured (step 3). Then, a fixed test dose, Dt was given before heating to 220°C (for 0 s), which is a process to empty the luminescence trap. After that, the test dose luminescence signal, T0, which is relevant to the natural OSL measurement, was measured (Murray and Wintle, 2003). The cycle was repeated four times with the regeneration doses being increased step by step. Per standard convention, OSL ages are reported in “years” which is the analytical date of deposition prior to the date of measurement, which, in this case was AD 2018. Thus, there is a 68-year offset between radiocarbon and OSL ages in this manuscript.
4 Results
Two archaeological sites, Forte and Ibama, were subject to screened excavations, profile documentation and radiocarbon dating and show aggraded occupation horizons (Figure 3). Radiocarbon dating of the sites in Caxiuanã indicate that the Forte site was occupied ca. 1912–1783 cal years BP (Table 1) and ceramics were from the Incised Rim/Barrancoid tradition. Aggradation of the terra preta occurred vis-à-vis the dumping of mollusk shell and other organic waste in addition to ceramics. Dating and stratigraphic analysis the test pit from the deepest to shallowest units indicates the presence of aggradational sequences as alternating packages of sediments and shells within the ca. 130-year timeframe of occupation. The site of Ibama was occupied between ca. 758 to 594 cal years BP. Ceramics recovered from the archaeological excavations are indicative of continuous settlement without a hiatus (Barreto and Lima, 2021), but alternating lenses of mollusk and organic-rich sediments are indicative of punctuated phases of aggradation of the landform. Shell temper has been found in thin section of the ceramics from both earlier (Forte) and later (Ibama) mounds illustrating the importance of mollusk shell to the production of ceramics, and shells comprised a significant portion of the matrix of the mound. A full report of the archaeological artifacts recovered from the site is in preparation and outside the scope of the present manuscript.
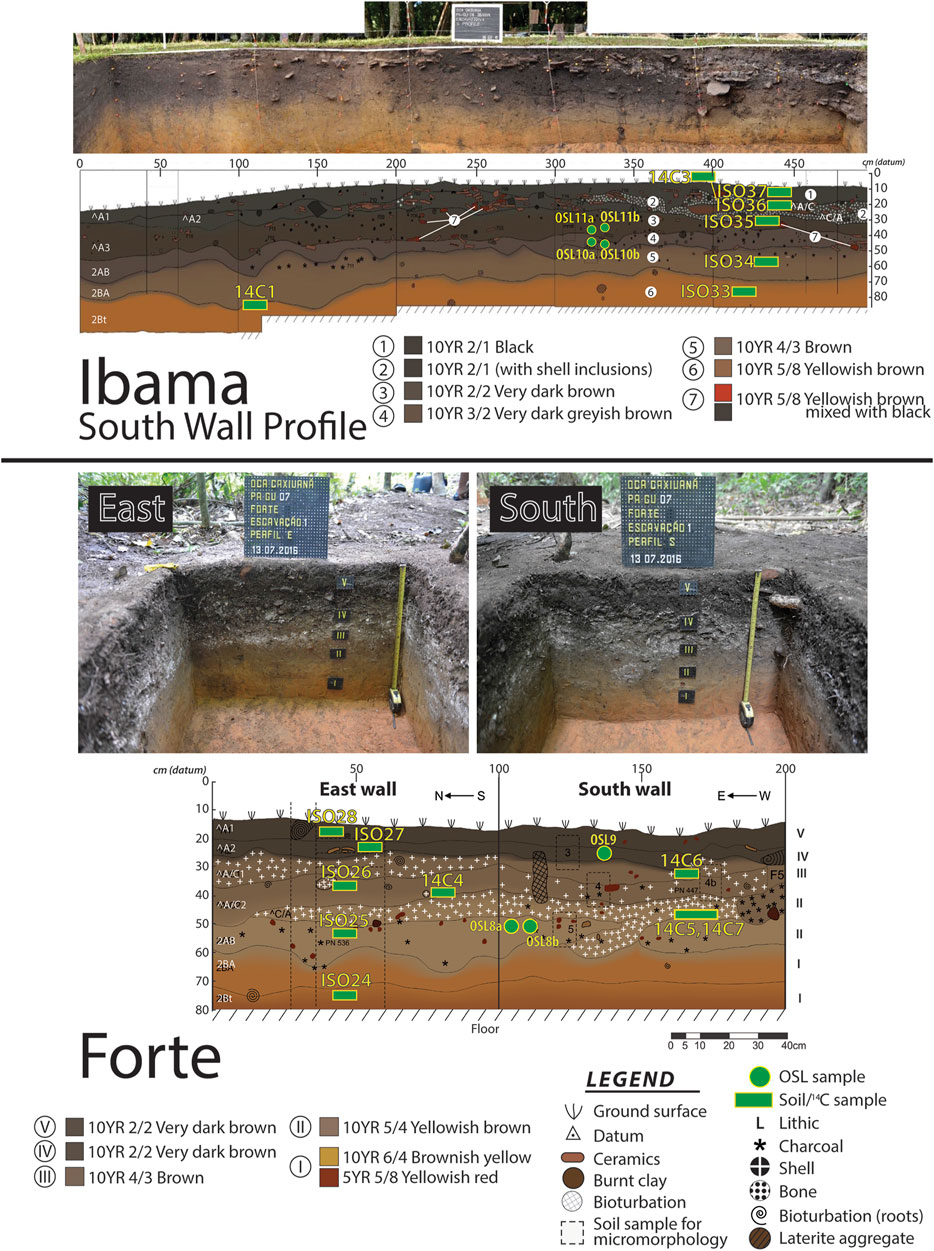
FIGURE 3. Profile photos and sketches of Ibama (top) and Forte (bottom) sites. Site elevations are relative to a datum fixed at each site. Full descriptions of the sediments are provided in Supplementary Material S1.
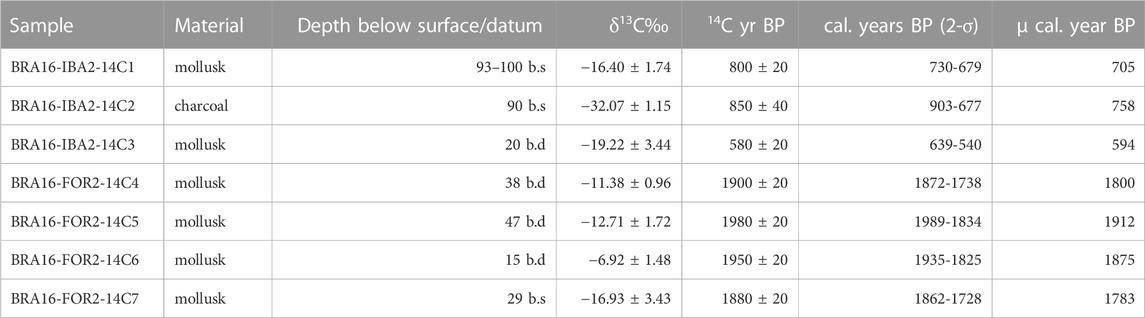
TABLE 1. Radiocarbon ages from archaeological sites sampled in the Caxiuanã National Forest (2016). Refer to Figure 2 for mapped locations of FOR2 and IBA2, and Figure 3 shows sampling points within the profiles. Radiocarbon ages calendar corrected for atmospheric production of radiocarbon using Reimer et al. (2020) in OxCal 4.4.
OSL ages of on-site contexts (IBA2, FOR2) have statistical overlap with radiocarbon ages demonstrating the efficacy of the method to reconstruct landscape sedimentation in weathered edaphic contexts such as in Caxiuanã (Table 2). Off-site samples (IBA1) and near-site (FOR1, IBA5) samples show alluvial sedimentation at ca. 15,900 years until 900 years with temporal overlap in the latter samples with site occupations at Ibama (IBA2) and Forte (FOR2). Within near- and off-site contexts, overdispersion of the samples was higher than in on-site contexts, which may be attributed to more extensive vertical bioturbation of sand grains (90–250 μm).
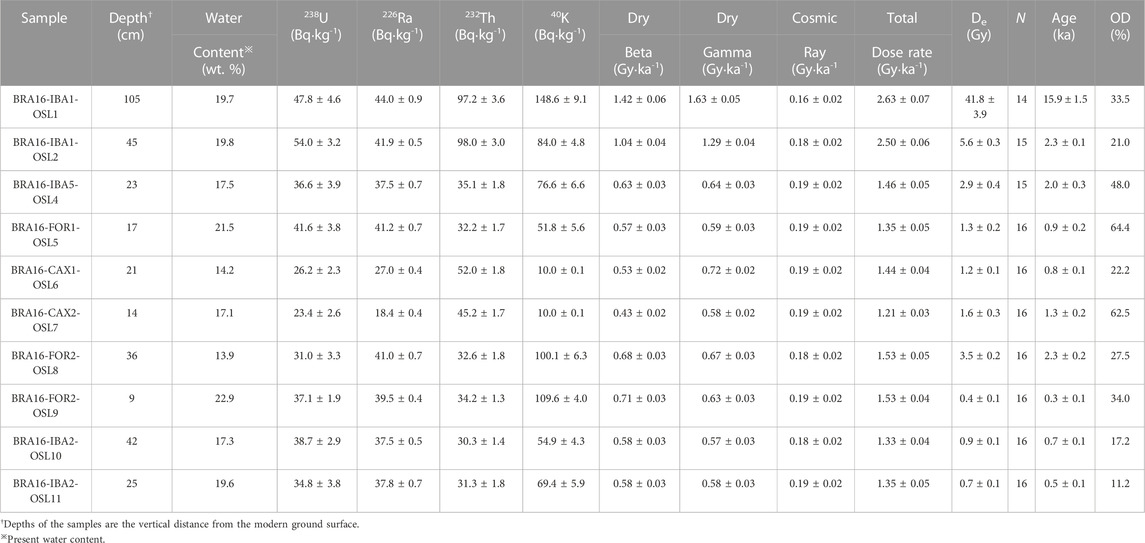
TABLE 2. Results of Optically Stimulated Luminescence dating and measures of over-dispersion (OD%) (Galbraith and Roberts, 2012) from Caxiuanã National Forest, Brazil. Refer to Figure 2 for sample locations, and Figure 3 shows sampling points within the profiles.
Bivariate plots of soil stable isotopes conducted of landform/soil classification categories (terra preta, terra marrom non-ADE anthropogenic forest and offsite latosol) show overlapping, but statistically different, isotopic distributions with enriched 13C (more C4 or open landscape conditions) and 15N levels located on terra preta relative to terra marrom and latosol (Figure 4; Supplementary Material S2). A paired t-test of 15N content showed a statistically significant difference between terra preta and terra marrom (t=3.75, p=0.0006, df=39), but there was no statistically significant difference between terra marrom and latosol (t=0.90, p=0.3766, df=31) or more broadly classified anthropic soils (terra preta + terra marrom) vs. latosol (t=0.42, p=0.6796, df=50). On the other hand, 13C between terra preta and terra marrom did show statistically significant differences (t=2.58, p=0.00138, df=39) with the former being more isotopically enriched than the latter, and terra marrom was statistically significantly more enriched relative to latosol samples (t=2.43, p=0.0212, df=31).
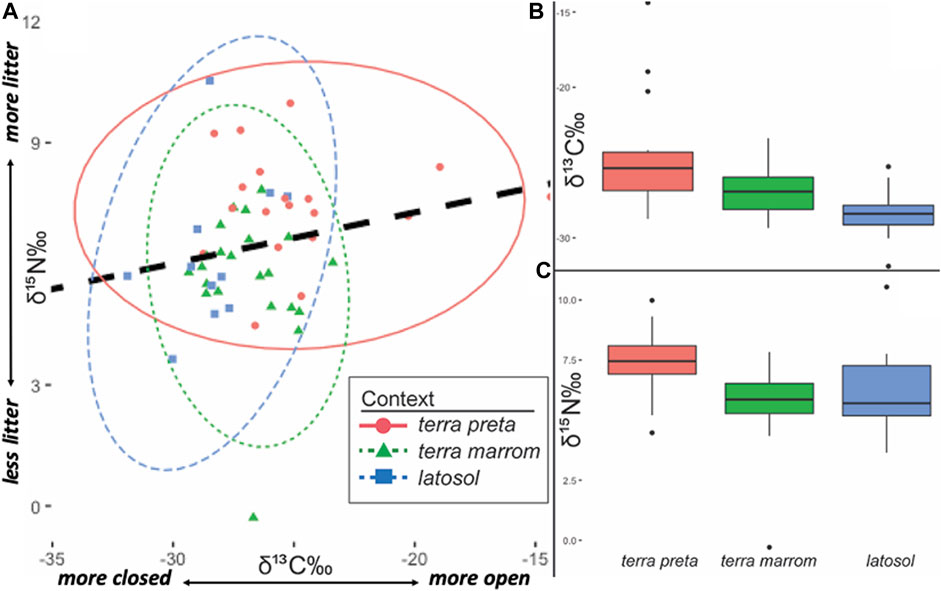
FIGURE 4. (A) Bivariate ellipses (2-σ) of edaphic contexts terra preta, terra marrom and latosols sampled from Caxiuanã National Forest, Brazil. Regression line (dashed black): r2 = +0.206 (B) 2-σ box plot of δ13C (vs. VPDB). by edaphic contexts (C) 2-σ box plot of δ15N (vs. AIR) by edaphic contexts.
To control for isotopic variability based on horizonation (Figure 5) and depth below ground surface (Figure 6), statistical tests were also performed to evaluate the effects of soil master horizon designations and relative elevation. Soil horizonation and depths are not independent measures, as soils form as depth-predicated entities. Nevertheless, to exclude the possibility that variance in isotopes identified by general edaphic contexts was controlled by one or the other factor, both were independently tested. T-tests performed between soils interpreted as anthropogenic A-horizons (^A in the USDA classification scheme, sensu Schoeneberger et al., 2012) show statistically higher 15N in anthrosol A horizons compared to non-anthrosol A horizons (t=3.50, p=0.0016, df=27). On the other hand, when taken in aggregate, non-anthropogenic A horizons did not show statistically significant differences in comparison to E, B and C horizons (t=1.26, p=0.2157, df=36), but ^A horizons were more isotopically enriched with statistically significant differences (t=3.16, p=0.0033, df=35). The content of 15N did not show statistically significant differences relative to depth below surface in t-tests of 0–10 cm vs. >40 cm below surface (t=0.26, p=0.7965, df=27) whereas 13C was significantly more depleted in upper solum samples (0–10 cm) relative to samples collected below 40 cm (t=2.66, p=0.0131, df=27).
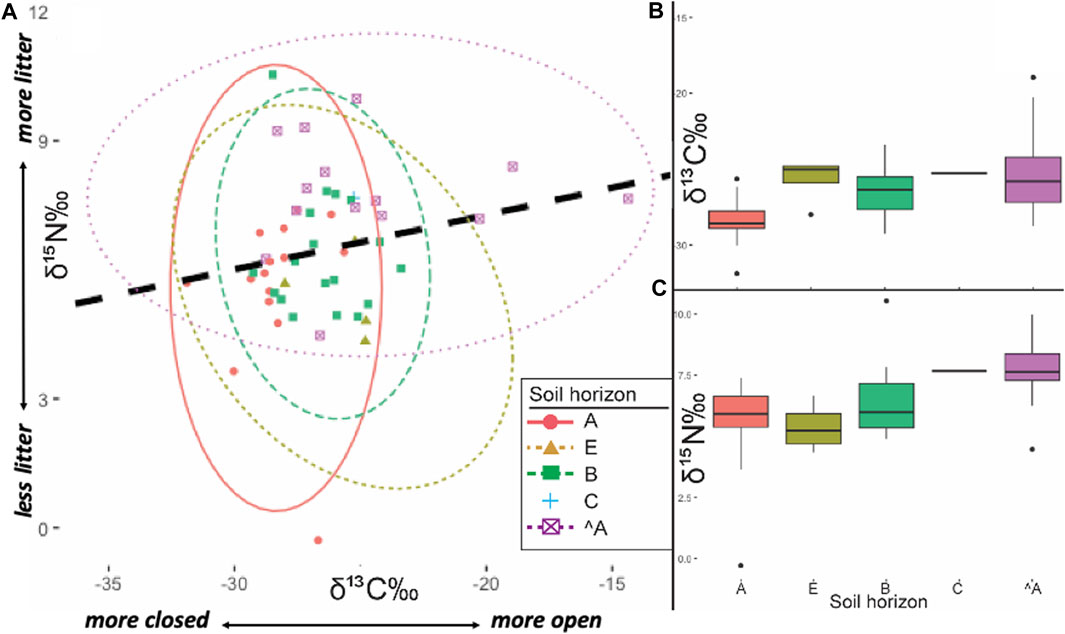
FIGURE 5. (A) Bivariate ellipses (2-σ) of soil horizon contexts (Schoeneberger et al., 2012) sampled from Caxiuanã National Forest, Brazil. Regression line (dashed black): r2 = +0.206 (B) 2-σ box plot of δ13C (vs. VPDB). by soil horizon contexts (C) 2-σ box plot of δ15N (vs. AIR). by soil horizon contexts.
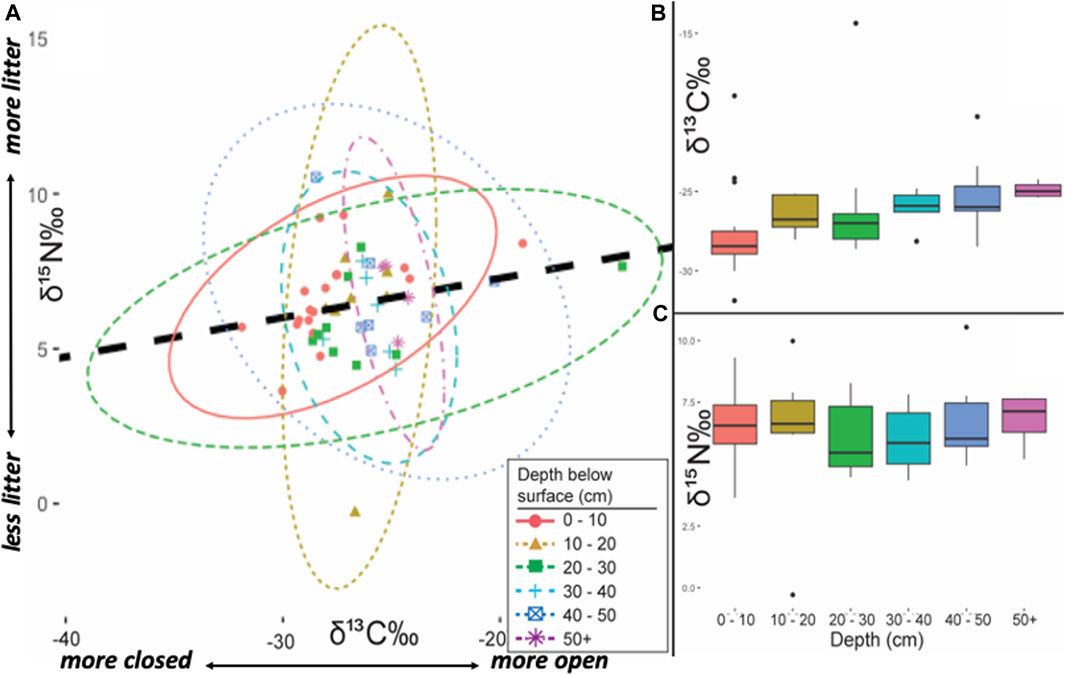
FIGURE 6. (A) Bivariate ellipses (2-σ) of depth below surface contexts sampled from Caxiuanã National Forest, Brazil. Regression line (dashed black): r2 = +0.206 (B) 2-σ box plot of δ13C (vs. VPDB). by depth below surface contexts (C) 2-σ box plot of δ15N (vs. AIR). by depth below surface contexts.
Total Organic Carbon (TOC) and Total Nitrogen (TN) were also compared across the different edaphic contexts (terra preta, terra marrom, latosol). A Shapiro-Wilks ANOVA test of normality on TOC found that the variance is non-normally distributed (W = 0.913, p-value = 0.001) as was TN (W = 0.741, p-value = <0.001). Boxplots of the data show that TOC and TN are significantly higher within terra preta settings vs. terra marrom and latosol, of which the latter two statistically overlap with one another (Figure 7).
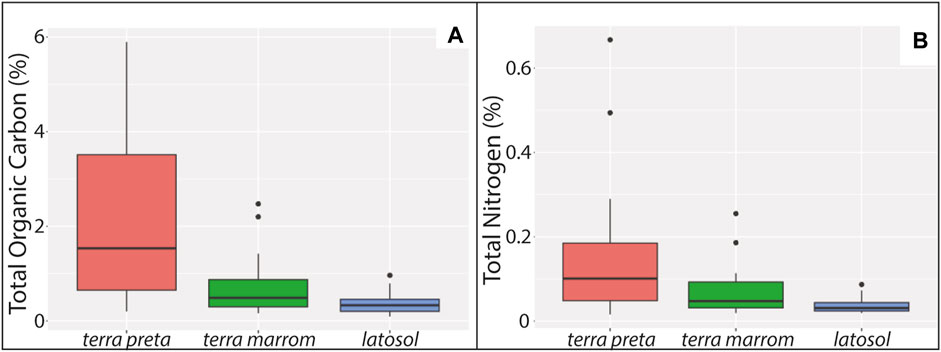
FIGURE 7. (A) Total Organic Carbon (%) of edaphic contexts sampled. (B) Total Nitrogen (%) of edaphic contexts sampled from Caxiuanã National Forest, Brazil.
5 Discussion
The CNF today represents an aggregate ecological assemblage from natural and anthropogenic forces, particularly in the regions adjacent to waterbodies. The Amazon Rainforest is earth’s largest terrestrial reservoir of biodiversity (Rodrigues et al., 2013) and within the context of the tropical setting, forests are the predominant form of land cover. Our results demonstrate the continuity of forest cover throughout the duration of sedimentation and soil formation, including the period in which humans colonized and settled the region. Beginning ca. 1900 cal years BP, terra preta was deliberately created within a shell mound matrix at Forte and later (ca. 760–590 cal years BP) at Ibama. The soils surrounding the sites (terra marrom) demonstrate a gradient effect of isotopes, TOC and TN relative to the on-site test locations. Thus, the human contribution to shaping the ecological complexion of the landscape was primarily focused on the archaeological sites themselves, but extended beyond the site areas into the surrounding forest.
We posit that the distribution of nutrients from enriched patches of land following the abandonment of terra preta sites contributed to the homogenization of carbon and nitrogen isotopes across the lowland portions of the CNF, although we note that there are some distinguishing differences. Isotopically enriched nitrogen (15N) is demonstrated to be statistically higher in terra preta than in terra marrom as well as anthropogenic A-horizons (^A) than in non-anthropogenic A-horizons. This reflects nutrient pooling and high amounts of litter on directly human amended terra preta soils compared to less managed contexts. Human manipulation of the soil conditions are interpreted here as having had the effect of concentrating isotopically heavier forms of nitrogen during respiration of NO2. Isotopically enriched carbon (13C) is more abundant overall in terra preta than surrounding soils, however we interpret this as being a function of the abundant remains of freshwater mollusks in on-site contexts, as exhibited in the δ13C values assayed during radiocarbon dating of mollusk shells (Table 1). Near-site (terra marrom) δ13C values reflect nutrient redistribution or, perhaps, occurrence of more open-canopy conditions in the past during some period of soil formation relative to off-site (latosol) contexts, which are more isotopically depleted (indicating C3 contribution to the soil and/or a canopy effect). Overdispersion of luminescence signals indicate mixing of 90–250 μm grains from their primary depositional contexts, which is an indicator of the degree of bioturbation (Kristensen et al., 2015). Grain overdispersion in tropical rainforest is correlated with more intensive forms of land management, especially anthropogenic fires, which increase the overall biodiversity of tree species present (Tovar et al., 2014). OSL data indicate significantly higher degrees of soil mixing within terra marrom and latosol contexts compared to terra preta, however the dataset is relatively small.
Total elemental concentrations provide further context for the results of the isotopic study. TOC and TN are higher with statistical significance on-site vs. near- and off-site contexts, which agrees with previous studies of these elements from Forte and Ibama (Kern, 1996; Lemos et al., 2011; Martins da Silva et al., 2017). Thus, we see that the isotopic differences between terra preta, terra marrom and latosol contexts reflect continuity of vegetal and nutrient communities in Caxiuanã, while organic nutrient pools are concentrated within terra preta, where bioturbation vis-à-vis OSL overdispersion appears to be more horizontal than vertical when compared to off-site forest contexts.
CNF remains a managed forest to this day. Although the population of the protected area is low, we argue that the persistence of human land management has created a gradient effect to the soil isotope composition of the region studied. Similar to our results, Paz-Rivera and Putz (2009) determine that there is similar a measure of nutrient homogenization on landscapes in the Bolivian Amazon with terra preta due to modern bioturbating agents and human propagation of economically beneficial tree species outside habitation areas. In addition, the relatively high rainfall and soil weathering rates have likely normalized the expression of the isotopically heavier 15N isotope in the soils relative to values expected from more temperate climates (Ambrose, 1991; Posada and Schuur, 2011). Early degradation of long-chain fatty acids (n-C22:0–30:0) relative to n-alkanes (n-C23–33) during decomposition of organic matter has been argued in a controlled study from western Europe as enriching the 13C component in the soil relative to the vegetation canopy (Hirave et al., 2020; see also; Potapov et al., 2019), which would be less likely to occur in a setting such as mound construction (terra preta) than in a managed forest. Thus, future studies of the isotope ecology in the Amazon should explore this hypothesis by undertaking compound specific isotope analyses of n-alkanes from on-site, near-site and off-site contexts to determine whether the relatively homogenous isoscape presented in this study is a function of human landscape management practices or early degradation of long-chain fatty acids (see also Thomas et al., 2021).
6 Conclusion
This landscape-scale study of 13C, 15N, TOC, TN within a portion of the CNF from archaeological sites ranging between 1912 and 1783 cal years BP and 758 to 594 cal years BP indicates that nutrient pooling of organic matter from terra preta created a gradient enrichment effect between on-site and off-site contexts. In our study terra preta is interpreted as having primarily formed from vegetal matter from the surrounding forest intercalated with mollusk shells, which resulted in the concentration of C3-derived organic matter rather than organic matter from C4 sources. Through the deliberate creation of terra preta and near-site land management practices to propagate species beneficial to agroforestry endeavors, people settling in this region were cornerstone ecosystem engineers, concentrating nutrients upon focal points (sites) adjacent to waterways. Our study supports previous research arguing terra preta formation resulted in a net enrichment of carbon and nitrogen on and near sites within forested settings and was not associated with large-scale landscape clearance, as European forms of cereal agriculture commonly are (e.g., Sombroek et al., 2003; Steiner et al., 2004; Heckenberger et al., 2008; Glaser and Birk, 2012; Piperno et al., 2015; Maezumi et al., 2018; Iriarte et al., 2020; Maezumi et al., 2022). Our results also agree, statistically, with δ13C values obtained by Robinson et al. (2021), although we interpret the cause of isotopic enrichment of 13C in the Caxiuanã terra preta setting as due to the contribution of mollusk shells and potentially inhibited degradation of long-chain fatty acids relative to surrounding off-site contexts.
This study adopted a landscape approach to study formation processes and stable isotope ecologies from on-site (terra preta), near-site (terra marrom) and off-site (latosol) contexts. Intercomparability of sampling contexts was high because the proxy collection locations were selected based on having level ground surfaces that were free from obstructions, such as fallen trees or thick roots. Ongoing research in CNF is demonstrating that the long-term effects of historical land management processes continues to resonate in the ecosystem, especially in the types and density of vegetation biomass that occurs within managed forest regions (Choi et al., 2020). Landscape-focused archaeological research holds promise to better inform biosphere evolution and the complex relationship between human activities and soil formation processes. Particularly in the tropics, soil is a critical resource that warrants conservation because it forms over long periods of time and is the repository for the majority of tropical biomass (Geisen et al., 2019; Scow et al., 2020). As this study demonstrates, there is no intrinsic contradiction between robust conservation goals to maintain stands of tropical forest and the creation and preservation of productive soils for subsistence purposes.
Data availability statement
The datasets presented in this study can be found in online repositories. The names of the repository/repositories and accession number(s) can be found in the article/Supplementary Material. Supplementary data and code are also available at https://www.doi.org/10.6084/m9.figshare.22094153.
Author contributions
DW, HL, JC, and ABR contributed to conception and design of the study. DW organized the database. DW, JC, and J-HC prepared and analyzed data presented in the study. DW performed the statistical analysis. DW wrote the first draft of the manuscript. DW, HL, ABR, MM, and KM wrote sections of the manuscript. All authors contributed to manuscript revision, read, and approved the submitted version.
Acknowledgments
This archaeological project takes place within the institutional scope of the Museu Paraense Emílio Goeldi (Belém-Pará-Brazil), with funding from the Fundação Amazônia de Amparo à Estudos e Pesquisas (FAPESPA #3007/2014, 2014-17, granted to HL). Permission for archaeological research in Caxiuanã National Forest was granted by Instituto do Patrimônio Histórico e Artístico Nacional (IPHAN # 01492.000340/2015-01), and Instituto Chico Mendes de Conservação da Biodiversidade (ICMBio # 54169-4) to HL. This research was supported by Korea-US International Cooperation through the National Research Foundation of Korea funded by the Ministry of Science, ICT and Future Planning (NRF2015068576) (DW), the Seoul National University College of Humanities’ Research and Education (CORE) program through the NRF funded by the Korean Ministry of Education (DW), Wenner-Gren grant #9566 (DW), a CORE travel grant (JC) and National Geographic Young Explorers grant #EC-KOR-223R-18 (JC). Permission to conduct field research was provided by the Polícia Federal Brasil and the Ministéiro da Ciência, Tecnologica e Inovação under the auspices of a research visa (015474ML) granted to DW. We thank Socorro Andrade, Director of Estação Cientifica Ferreira Penna in the Caxiuanã National Forest. Richard Pace and Andrew Wyatt of Middle Tennessee State University were crucial elements of success to this project for running the 2016 MTSU Archaeological Field School in Amazon, which provided a cohort of student workers and providing crucial logistical support. Jiyoung Park and Mirae Cho (Seoul National University) prepared the isotope samples for analysis. Local crew provided us outstanding guides and boats through the rainforest, with special thanks to “Mo.” The authors also thank associate editor Nathan Stansell and two reviewers for the time they took to improve the quality of this manuscript from submission to publication.
Conflict of interest
The authors declare that the research was conducted in the absence of any commercial or financial relationships that could be construed as a potential conflict of interest.
Publisher’s note
All claims expressed in this article are solely those of the authors and do not necessarily represent those of their affiliated organizations, or those of the publisher, the editors and the reviewers. Any product that may be evaluated in this article, or claim that may be made by its manufacturer, is not guaranteed or endorsed by the publisher.
Supplementary material
The Supplementary Material for this article can be found online at: https://www.frontiersin.org/articles/10.3389/feart.2023.1172406/full#supplementary-material
References
Alho, C. F. B. V., Samuel-Rosa, A., Martins, G. C., Hiemstra, T., Kuyper, T. W., and Teixeira, W. G. (2019). Spatial variation of carbon and nutrients stocks in Amazonian Dark Earth. Geoderma 337, 322–332. doi:10.1016/j.geoderma.2018.09.040
Ambrose, S. H. (1991). Effects of diet, climate and physiology on nitrogen isotope abundances in terrestrial foodwebs. J. Archaeol. Sci. 18 (3), 293–317. doi:10.1016/0305-4403(91)90067-y
Ambrose, S. H., and Sikes, N. E. (1991). Soil carbon isotope evidence for Holocene habitat change in the Kenya Rift Valley. Science 253 (5026), 1402–1405. doi:10.1126/science.253.5026.1402
Arroyo-Kalin, M. (2014). “The variability of Amazonian Dark Earths: Comparing anthropogenic soils from three regions of the Amazonian biome,” in Antes de orellana. Actas del III encuentro de arqueología amazónica. Editor S. Rostain (Quito: Instituto Francés de Estudios Andinos), 323–330.
Asare, M. O. (2022). Anthropogenic dark Earth: Evolution, distribution, physical, and chemical properties. Eur. J. Soil Sci. 73 (5), e13308. doi:10.1111/ejss.13308
Balée, W. (2006). The research program of historical ecology. Annu. Rev. Anthropol. 35, 75–98. doi:10.1146/annurev.anthro.35.081705.123231
Barreto, C., and Lima, H. P. (2021). “Understanding the dispersion of Koriabo ceramics in the lower Amazon: What is Koriabo?,” in Koriabo: From the caribbean Sea to the Amazon River. Editors C. Barreto, S. Rostain, and H. P. Lima (Belém: Museu Paraense Emílio Goeldi), 183–202.
Barreto, C., Rostain, S., and Lima, H. P. (2021). Koriabo: From the caribbean sea to the Amazon River. Belém: Museu Paraense Emílio Goeldi.
Behling, H., and da Costa, M. L. (2000). Holocene environmental changes from the Rio Curuá record in the Caxiuanã region, eastern Amazon Basin. Quat. Res. 53 (3), 369–377. doi:10.1006/qres.1999.2117
Bell, R. H. V. (1982). “The effect of soil nutrient availability on community structure in African ecosystems,” in Ecology of tropical savannas. Editors B. J. Huntley, and B. H. Walker (Berlin: Springer-Verlag), 193–216.
Choi, J., Wright, D. K., and Lima, H. P. (2020). A new local scale prediction model of Amazonian landscape domestication sites. J. Archaeol. Sci. 123, 105240. doi:10.1016/j.jas.2020.105240
Choi, J-H., Cheong, C. S., and Chang, H. W. (2004). Principles of quartz OSL (Optically Stimulated Luminescence) dating and its geological applications (in Korean). J. Geol. Soc. Korea 40, 567–583.
Clark, D. A., Asao, S., Fisher, R., Reed, S., Reich, P. B., Ryan, M. G., et al. (2017). Reviews and syntheses: Field data to benchmark the carbon cycle models for tropical forests. Biogeosciences 14 (20), 4663–4690. doi:10.5194/bg-14-4663-2017
Clement, C. R., and Junqueira, A. B. (2010). Between a pristine myth and an impoverished future. Biotropica 42 (5), 534–536. doi:10.1111/j.1744-7429.2010.00674.x
Coirolo, A. D., and D'Aquino, G. I. D. R. (2005). Salvamento arqueológico no sítio ilha de Terra, região de Caxiuanã, melgaço, Pará. Congr. Soc. Arqueol. Bras. Soc. Arqueol. Bras. 13, 1–4.
Commisso, R. G., and Nelson, D. E. (2006). Modern plant δ15N values reflect ancient human activity. J. Archaeol. Sci. 33 (8), 1167–1176. doi:10.1016/j.jas.2005.12.005
Costa, J. A., Lima da Costa, M., and Kern, D. C. (2013). Analysis of the spatial distribution of geochemical signatures for the identification of prehistoric settlement patterns in ADE and TMA sites in the lower Amazon Basin. J. Archaeol. Sci. 40 (6), 2771–2782. doi:10.1016/j.jas.2012.12.027
Crotty, F. V., Adl, S. M., Blackshaw, R. P., and Murray, P. J. (2012). Using stable isotopes to differentiate trophic feeding channels within soil food webs. J. Eukaryot. Microbiol. 59 (6), 520–526. doi:10.1111/j.1550-7408.2011.00608.x
Dawson, T. E., Mambelli, S., Plamboeck, A. H., Templer, P. H., and Tu, K. P. (2002). Stable isotopes in plant ecology. Annu. Rev. Ecol. Syst. 33 (1), 507–559. doi:10.1146/annurev.ecolsys.33.020602.095451
de Souza, E. B., Kayano, M. T., and Ambrizzi, T. (2005). Intraseasonal and submonthly variability over the Eastern Amazon and Northeast Brazil during the autumn rainy season. Theor. Appl. Climatol. 81 (3), 177–191. doi:10.1007/s00704-004-0081-4
de Souza, J. G., Alcaina Mateos, J., and Madella, M. (2020). Archaeological expansions in tropical South America during the late Holocene: Assessing the role of demic diffusion. PLoS ONE 15 (4), e0232367. doi:10.1371/journal.pone.0232367
Denevan, W. M. (2004). “Semi-intensive pre-European cultivation and the origins of anthropogenic dark earths in Amazonia,” in Amazonian Dark earths: Explorations in space and time. Editors B. Glaser, and W. I. Woods (Berlin, Heidelberg: Springer Berlin Heidelberg), 135–143.
Desjardins, T., Filho, A. C., Mariotti, A., Girardin, C., and Chauvel, A. (1996). Changes of the forest-savanna boundary in Brazilian Amazonia during the Holocene revealed by stable isotope ratios of soil organic carbon. Oecologia 108 (4), 749–756. doi:10.1007/bf00329051
Doughty, C. E., Metcalfe, D. B., da Costa, M. C., de Oliveira, A. A., Neto, G. F., Silva, J. A., et al. (2014). The production, allocation and cycling of carbon in a forest on fertile terra preta soil in eastern Amazonia compared with a forest on adjacent infertile soil. Plant Ecol. Divers. 7 (1-2), 41–53. doi:10.1080/17550874.2013.798367
Downie, A. E., Van Zwieten, L., Smernik, R. J., Morris, S., and Munroe, P. R. (2011). Terra Preta Australis: Reassessing the carbon storage capacity of temperate soils. Agric. Ecosyst. Environ. 140 (1), 137–147. doi:10.1016/j.agee.2010.11.020
Erickson, C. L. (2008). “Amazonia: The historical ecology of a domesticated landscape,” in The handbook of South American archaeology. Editors H. Silverman, and W. H. Isbell (New York, NY: Springer), 157–183.
Evans, C., and Meggers, B. J. (1960). Archaeological investigations in British guiana. Washington, DC: Smithsonian Institute.
Fearnside, P. M. (2005). Deforestation in Brazilian Amazonia: History, rates, and consequences. Conserv. Biol. 19 (3), 680–688. doi:10.1111/j.1523-1739.2005.00697.x
Fonseca, I. F. D., Lindoso, D. P., and Bursztyn, M. (2022). (Falta de) controle do desmatamento na Amazônia brasileira: Do fortalecimento ao desmantelamento da autoridade governamental (1999-2020). Sustain. Debate 13 (2), 12–31. doi:10.18472/sustdeb.v13n2.2022.44532
Fraser, J., Teixeira, W., Falcão, N., Woods, W., Lehmann, J., and Junqueira, A. B. (2011). Anthropogenic soils in the Central Amazon: From categories to a continuum. Area 43 (3), 264–273. doi:10.1111/j.1475-4762.2011.00999.x
Galbraith, R. F., and Roberts, R. G. (2012). Statistical aspects of equivalent dose and error calculation and display in OSL dating: An overview and some recommendations. Quat. Geochronol. 11, 1–27. doi:10.1016/j.quageo.2012.04.020
Geisen, S., Wall, D. H., and van der Putten, W. H. (2019). Challenges and opportunities for soil biodiversity in the Anthropocene. Curr. Biol. 29 (19), R1036–R1044. doi:10.1016/j.cub.2019.08.007
Glaser, B., and Birk, J. J. (2012). State of the scientific knowledge on properties and Genesis of anthropogenic dark earths in central Amazonia (terra preta de Índio). Geochimica Cosmochimica Acta 82, 39–51. doi:10.1016/j.gca.2010.11.029
Glaser, B. (2007). Prehistorically modified soils of central Amazonia: A model for sustainable agriculture in the twenty-first century. Philosophical Trans. R. Soc. B Biol. Sci. 362 (1478), 187–196. doi:10.1098/rstb.2006.1978
Heckenberger, M. J., Russell, J. C., Fausto, C., Toney, J. R., Schmidt, M. J., Pereira, E., et al. (2008). Pre-Columbian urbanism, anthropogenic landscapes, and the future of the Amazon. Science 321 (5893), 1214–1217. doi:10.1126/science.1159769
Hermenegildo, T., O'Connell, T. C., Guapindaia, V. L. C., and Neves, E. G. (2017). New evidence for subsistence strategies of late pre-colonial societies of the mouth of the Amazon based on carbon and nitrogen isotopic data. Quat. Int. 448, 139–149. doi:10.1016/j.quaint.2017.03.003
Hirave, P., Wiesenberg, G. L. B., Birkholz, A., and Alewell, C. (2020). Understanding the effects of early degradation on isotopic tracers: Implications for sediment source attribution using compound-specific isotope analysis (CSIA). Biogeosciences 17 (8), 2169–2180. doi:10.5194/bg-17-2169-2020
Hofman, C., Rostain, S., and Barreto, C. (2021). “Koriabo: The mysterious amazonian/caribbean culture,” in Koriabo: From the caribbean Sea to the Amazon River. Editor C. Barreto (Belém: Museu Paraense Emílio Goeldi), 13–30.
Iriarte, J., Elliott, S., Maezumi, S. Y., Alves, D., Gonda, R., Robinson, M., et al. (2020). The origins of Amazonian landscapes: Plant cultivation, domestication and the spread of food production in tropical South America. Quat. Sci. Rev. 248, 106582. doi:10.1016/j.quascirev.2020.106582
Johnson, S. E., and Loeppert, R. H. (2006). Role of organic acids in phosphate mobilization from iron oxide. Soil Sci. Soc. Am. J. 70 (1), 222–234. doi:10.2136/sssaj2005.0012
Kern, D. C. (1996). Geoquímica e pedogeoquímica em sítios arqueológicos com terra preta na floresta nacional de Caxiuanã (Portel-PA). Belém: Universidade Federal do Pará.
Kern, D. C., Lima, H. P., da Costa, J. A., de Lima, H. V., Browne Ribeiro, A., Moraes, B. M., et al. (2017). Terras pretas: Approaches to formation processes in a new paradigm. Geoarchaeology 32 (6), 694–706. doi:10.1002/gea.21647
Kohn, M. J. (2010). Carbon isotope compositions of terrestrial C3 plants as indicators of (paleo)ecology and (paleo)climate. Proc. Natl. Acad. Sci. U. S. A. 107 (46), 19691–19695. doi:10.1073/pnas.1004933107
Kristensen, J. A., Thomsen, K. J., Murray, A. S., Buylaert, J. P., Jain, M., and Breuning-Madsen, H. (2015). Quantification of termite bioturbation in a savannah ecosystem: Application of OSL dating. Quat. Geochronol. 30, 334–341. doi:10.1016/j.quageo.2015.02.026
Lemos, V. P., Meireles, A. RdO., Fernandes, KdG., Moraes, M. C. d., Costa, M. L. d., Silva, A. K. T., et al. (2011). Nutrients in amazonian black earth from Caxiuanã region. J. Braz. Chem. Soc. 22 (4), 772–779. doi:10.1590/S0103-50532011000400022
Levis, C., de Souza Silva, M., and Almeida e Silva, M. (2014). “What do we know about the distribution of Amazonian Dark Earth along tributary rivers in Central Amazonia?,” in Antas de Orellana - actas del 3er Encuentro Internacional de Arqueología Amazónica. Editor S. Rostain (Quito: Instituto Francés de Estudios Andinos), 305–311.
Lima, H. P. (2008). História das caretas: A tradição borda incisa na Amazônia central universidade de São paulo. São Paulo: Universidade de São Paulo.
Lisboa, P. L. B., Bezerra, MdG. F., and Cardoso, A. LdR. (2013). Caxiuanã: História natural e ecologia de uma floresta nacional da Amazônia. Belem, Brazil: Museu Paraense Emílio Goeldi.
Lombardo, U., Arroyo-Kalin, M., Schmidt, M., Huisman, H., Lima, H. P., de Paula Moraes, C., et al. (2022). Evidence confirms an anthropic origin of amazonian dark earths. Nat. Commun. 13 (1), 3444. doi:10.1038/s41467-022-31064-2
Macedo, R. S., Teixeira, W. G., Corrêa, M. M., Martins, G. C., and Vidal-Torrado, P. (2017). Pedogenetic processes in anthrosols with pretic horizon (amazonian dark earth) in central Amazon, Brazil. PLoS ONE 12 (5), e0178038. doi:10.1371/journal.pone.0178038
Maezumi, S. Y., Elliott, S., Robinson, M., Betancourt, C. J., Gregorio de Souza, J., Alves, D., et al. (2022). Legacies of Indigenous land use and cultural burning in the Bolivian Amazon rainforest ecotone. Philosophical Trans. R. Soc. B Biol. Sci. 377 (1849), 20200499. doi:10.1098/rstb.2020.0499
Maezumi, S. Y., Robinson, M., de Souza, J., Urrego, D. H., Schaan, D., Alves, D., et al. (2018). New insights from pre-Columbian land use and fire management in Amazonian Dark Earth forests. Front. Ecol. Evol. 6. doi:10.3389/fevo.2018.00111
Martins da Silva, D., Quaresma Martins, R., and Lima, H. P. (2017). Estudo de caracterização das terras pretas arqueológicas da região de Caxiuanã no âmbito do projeto: Ocupação Humana no Delta Amazônico. 57° Congresso Brasileiro de Química. Rio de Janeiro: Associação Brasileiro de Química.
McDaniel, K. (2023). Gastronauts of the lower Amazon: Shell, ceramic and landscape across two millennia in Caxiuanã. PhD Thesis. Gainesville, Florida: University of Florida.
McMichael, C. N. H., and Bush, M. B. (2019). Spatiotemporal patterns of pre-Columbian people in Amazonia. Quat. Res. 92 (1), 53–69. doi:10.1017/qua.2018.152
McMichael, C. N. H., Palace, M. W., Bush, M. B., Braswell, B., Hagen, S., Neves, E. G., et al. (2014) Predicting pre-Columbian anthropogenic soils in Amazonia. Proc. R. Soc. B Biol. Sci. 281, 20132475. doi:10.1098/rspb.2013.2475
Medina, E., Martinelli, L. A., Barbosa, E., and Victoria, R. L. (1999). Natural abundance of 13C in tropical grasses from the INPA, Instituto Nacional de Pesquisas da Amazônia, herbarium. Braz. J. Bot. 22, 44–51. doi:10.1590/s0100-84041999000100007
Moran, E. F. (1993). Deforestation and land use in the Brazilian Amazon. Hum. Ecol. 21 (1), 1–21. doi:10.1007/bf00890069
Murray, A. S., and Wintle, A. G. (2000). Luminescence dating of quartz using an improved single-aliquot regenerative-dose protocol. Radiat. Meas. 32 (1), 57–73. doi:10.1016/s1350-4487(99)00253-x
Murray, A. S., and Wintle, A. G. (2003). The single aliquot regenerative dose protocol: Potential for improvements in reliability. Radiat. Meas. 37 (4-5), 377–381. doi:10.1016/s1350-4487(03)00053-2
Natelhoffer, K. J., and Fry, B. (1988). Controls on natural nitrogen-15 and carbon-13 abundances in forest soil organic matter. Soil Sci. Soc. Am. J. 52 (6), 1633–1640. doi:10.2136/sssaj1988.03615995005200060024x
Neves, E. (2022). Sob os tempos do equinócio: Oito mil anos de história na Amazônia central. Sao Paulo: Ubu Editoria.
Neves, E. G., Guapindaia, V., and Lima, H. P. (2014). “A tradição Pocó-Açutuba e os primeiros sinais visíveis de modificações de paisagens na calha do Amazonas,” in Amazonía: Memoras de las Conferencias Magistrales del 3er Encuentro Internacional de Arqueología Amazónica. Editor S. Rostain (Quito: Ekseption Publicidad), 137–158.
Nordt, L. C., and Holliday, V. T. (2020). “Stable carbon isotopes in soils,” in Encyclopedia of geoarchaeology. Editors A. S. Gilbert, P. Goldberg, and R. D. Mandel (Cham: Springer International Publishing), 1–6.
Paz-Rivera, C., and Putz, F. E. (2009). Anthropogenic soils and tree distributions in a lowland forest in Bolivia. Biotropica 41 (6), 665–675. doi:10.1111/j.1744-7429.2009.00521.x
Pellin, A., Dias, L., Soares, N., and Prado, F. (2022). Management effectiveness and deforestation in protected areas of the Brazilian Amazon. Parks 28 (2), 45–54. doi:10.2305/iucn.ch.2022.parks-28-2ap.en
Pessenda, L. C. R., Gouveia, S. E. M., Aravena, R., Gomes, B. M., Boulet, R., and Ribeiro, A. S. (1997). 14C dating and stable carbon isotopes of Soil Organic Matter in forest–savanna boundary areas in the southern Brazilian Amazon region. Radiocarbon 40 (2), 1013–1022. doi:10.1017/s0033822200018981
Pingram, M. A., Collier, K. J., Özkundakci, D., and Garrett-Walker, J. (2020). Food web characteristics of fish communities across degraded lakes provide insights for management in multi-stressor environments. Aquat. Ecol. 54 (1), 401–419. doi:10.1007/s10452-020-09750-4
Piperno, D. R., McMichael, C., and Bush, M. B. (2015). Amazonia and the Anthropocene: What was the spatial extent and intensity of human landscape modification in the Amazon Basin at the end of prehistory? Holocene 25 (10), 1588–1597. doi:10.1177/0959683615588374
Piperno, D. R., McMichael, C. N. H., and Bush, M. B. (2019). Finding forest management in prehistoric Amazonia. Anthropocene 26, 100211. doi:10.1016/j.ancene.2019.100211
Posada, J. M., and Schuur, E. A. G. (2011). Relationships among precipitation regime, nutrient availability, and carbon turnover in tropical rain forests. Oecologia 165 (3), 783–795. doi:10.1007/s00442-010-1881-0
Potapov, A. M., Tiunov, A. V., and Scheu, S. (2019). Uncovering trophic positions and food resources of soil animals using bulk natural stable isotope composition. Biol. Rev. 94 (1), 37–59. doi:10.1111/brv.12434
Reimer, P. J., Austin, W. E. N., Bard, E., Bayliss, A., Blackwell, P. G., Bronk Ramsey, C., et al. (2020). The IntCal20 northern hemisphere radiocarbon age calibration curve (0–55 cal kBP). Radiocarbon 62 (4), 725–757. doi:10.1017/rdc.2020.41
Rey-Iglesia, A., Lister, A. M., Stuart, A. J., Bocherens, H., Szpak, P., Willerslev, E., et al. (2021). Late Pleistocene paleoecology and phylogeography of woolly rhinoceroses. Quat. Sci. Rev. 263, 106993. doi:10.1016/j.quascirev.2021.106993
Robinson, M., Jaimes-Betancourt, C., Elliott, S., Maezumi, S. Y., Hilbert, L., Alves, D., et al. (2021). Anthropogenic soil and settlement organisation in the Bolivian Amazon. Geoarchaeology 36 (3), 388–403. doi:10.1002/gea.21839
Rocha, B. (2020). “The incised punctate tradition: Evidence of a ‘lingua franca’ in operation? A view from one of its peripheries,” in Koriabo: From the caribbean Sea to the Amazon River. Belém. Editors C. Barreto, H. P. Lima, and S. Rostain (Belém, Brazil: Museu Paraense Emílio Goeldi), 267–287.
Rodrigues, J. L. M., Pellizari, V. H., Mueller, R., Baek, K., Jesus, E. d. C., Paula, F. S., et al. (2013). Conversion of the Amazon rainforest to agriculture results in biotic homogenization of soil bacterial communities. Proc. Natl. Acad. Sci. 110 (3), 988–993. doi:10.1073/pnas.1220608110
Rostain, S. (2008). “The archaeology of the Guianas: An overview,” in The handbook of South American archaeology. Editors H. Silverman, and W. H. Isbell (New York: Springer), 279–302.
Sachs, J. P. (2009). “Nitrogen isotopes,” in Encyclopedia of paleoclimatology and ancient environments. Editor V. Gornitz (Dordrecht: Springer Netherlands), 612–614.
Santos, E. B., Lucio, P. S., and Silva, C. M. S. E. (2015). Precipitation regionalization of the Brazilian Amazon. Atmos. Sci. Lett. 16 (3), 185–192. doi:10.1002/asl2.535
Schaan, D. P. (2004). The camutins chiefdom: Rise and development of social complexity on Marajó Island, Brazilian Amazon. PhD Thesis. Pittsburgh, Pennsylvania: University of Pittsburgh.
Schaefer, C. E. G. R., Fabris, J. D., and Ker, J. C. (2008). Minerals in the clay fraction of Brazilian latosols (Oxisols): A review. Clay Miner. 43 (1), 137–154. doi:10.1180/claymin.2008.043.1.11
Schmidt, M. J., Rapp Py-Daniel, A., de Paula Moraes, C., Valle, R. B., Caromano, C. F., Texeira, W. G., et al. (2014). Dark earths and the human built landscape in Amazonia: A widespread pattern of anthrosol formation. J. Archaeol. Sci. 42, 152–165. doi:10.1016/j.jas.2013.11.002
Schoeneberger, P. J., Wysocki, D. A., and Benham, E. C. (2012). “Field Book for Describing and sampling soils, version 3.0,” in Natural resources conservation Service (Lincoln, Nebraska: National Soil Survey Center).
Scow, K., Bardgett, R. D., and Pennock, D. (2020). State of knowledge of soil biodiversity: Status, challenges and potentialities. UN: Food and Agriculture Organization of the United Nations FAO.
Silva Junior, C. H. L., Pessôa, A. C. M., Carvalho, N. S., Reis, J. B. C., Anderson, L. O., and Aragão, L. E. O. C. (2021). The Brazilian Amazon deforestation rate in 2020 is the greatest of the decade. Nat. Ecol. Evol. 5 (2), 144–145. doi:10.1038/s41559-020-01368-x
Söderström, M., Eriksson, J., Isendahl, C., Schaan, D. P., Stenborg, P., Rebellato, L., et al. (2016). Sensor mapping of amazonian dark earths in deforested croplands. Geoderma 281, 58–68. doi:10.1016/j.geoderma.2016.06.024
Sombroek, W., Ruivo, M. D. L., and Fearnside, P. M. (2003). “Amazonian Dark Earths as carbon stores and sinks,” in Amazonian Dark earths: Origins, properties, management. Editors J. Lehmann, D. C. Kern, and B. Glaser (New York: Kluwer Academic Publishers), 125–139.
Sombroek, W. G. (1966). Amazonan soils. A reconnaissance of the soils of the Brazilian Amazon region. Wageningen: Centre for Agricultural Publication and Documentation.
Sotta, E. D., Veldkamp, E., Guimarães, B. R., Paixão, R., Ruivo, M., and Almeida, S. (2006). Landscape and climatic controls on spatial and temporal variation in soil CO2 efflux in an eastern Amazonian rainforest, Caxiuanã, Brazil. For. Ecol. Manag. 237 (1), 57–64. doi:10.1016/j.foreco.2006.09.027
Steiner, C., Teixeira, W. G., and Zech, W. (2004). “Slash and char: An alternative to slash and burn practiced in the Amazon Basin,” in Amazonian Dark earths: Explorations in space and time. Editors B. Glaser, and W. I. Woods (Berlin, Heidelberg: Springer Berlin Heidelberg), 183–193.
Sternberg, LdS. L., Moreira, M. Z., Martinelli, L. A., Victoria, R. L., Barbosa, E. M., Bonates, L. C., et al. (1997). Carbon dioxide recycling in two Amazonian tropical forests. Agric. For. Meteorology 88 (1), 259–268. doi:10.1016/s0168-1923(97)00038-5
Szabó, P. (2015). Historical ecology: Past, present and future. Biol. Rev. 90 (4), 997–1014. doi:10.1111/brv.12141
Thayn, J. B., Price, K. P., and Woods, W. I. (2011). Locating Amazonian Dark Earths (ADE) using vegetation vigour as a surrogate for soil type. Int. J. Remote Sens. 32 (21), 6713–6729. doi:10.1080/01431161.2010.512941
Thomas, C. L., Jansen, B., van Loon, E. E., and Wiesenberg, G. L. B. (2021). Transformation of <i&gt;n&lt;/i&gt;-alkanes from plant to soil: A review. SOIL 7 (2), 785–809. doi:10.5194/soil-7-785-2021
Tovar, C., Breman, E., Brncic, T., Harris, D. J., Bailey, R., and Willis, K. J. (2014). Influence of 1100 years of burning on the central African rainforest. Ecography 37 (11), 1139–1148. doi:10.1111/ecog.00697
Villagran, X. S. (2014). A redefinition of waste: Deconstructing shell and fish mound formation among coastal groups of southern Brazil. J. Anthropol. Archaeol. 36, 211–227. doi:10.1016/j.jaa.2014.10.002
WinklerPrins, A. M. G. A., and Levis, C. (2021). Reframing pre-European Amazonia through an anthropocene lens. Ann. Am. Assoc. Geogr. 111 (3), 858–868. doi:10.1080/24694452.2020.1843996
Keywords: Amazonian Dark Earth, landscape archaeology, latosol, soil formation, stable C and N isotopes, terra marrom, terra preta do Índio
Citation: Wright DK, Lima HP, Choi J, Choi J-H, Browne Ribeiro AT, Mariano MCP and McDaniel K (2023) Stable isotope ecology of terra preta in Caxiuanã National Forest, Brazil. Front. Earth Sci. 11:1172406. doi: 10.3389/feart.2023.1172406
Received: 23 February 2023; Accepted: 05 July 2023;
Published: 19 July 2023.
Edited by:
Nathan D. Stansell, Northern Illinois University, United StatesReviewed by:
Jose Iriarte, University of Exeter, United KingdomMarcondes Lima Da Costa, Federal University of Pará, Brazil
Copyright © 2023 Wright, Lima, Choi, Choi, Browne Ribeiro, Mariano and McDaniel. This is an open-access article distributed under the terms of the Creative Commons Attribution License (CC BY). The use, distribution or reproduction in other forums is permitted, provided the original author(s) and the copyright owner(s) are credited and that the original publication in this journal is cited, in accordance with accepted academic practice. No use, distribution or reproduction is permitted which does not comply with these terms.
*Correspondence: David K. Wright, ZC5rLndyaWdodEBpYWtoLnVpby5ubw==
†ORCID: David K. Wright, orcid.org/0000-0003-1704-0423; Jungyu Choi, orcid.org/0000-0001-5015-7194; Jeong-Heon Choi, orcid.org/0000-0003-2423-9959; Mayara C. P. Mariano, orcid.org/0000-0003-4156-1894; Kevin McDaniel, orcid.org/0000-0002-0137-9484