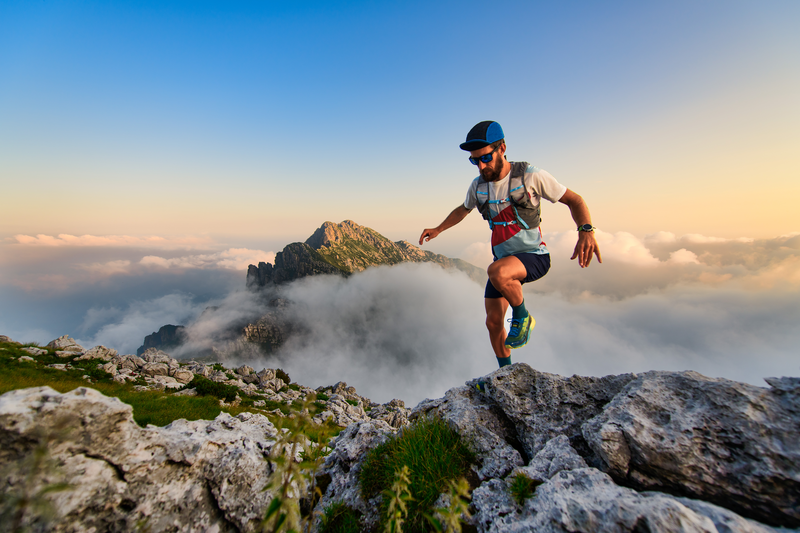
94% of researchers rate our articles as excellent or good
Learn more about the work of our research integrity team to safeguard the quality of each article we publish.
Find out more
ORIGINAL RESEARCH article
Front. Earth Sci. , 17 April 2023
Sec. Geochemistry
Volume 11 - 2023 | https://doi.org/10.3389/feart.2023.1167634
This article is part of the Research Topic Natural Hydrogen in Different Contexts: Geological, Cosmochemical and Biological View all 6 articles
A regional geochemistry field study was conducted in Albania and Kosova to spot natural H2 occurrences related to ophiolite massifs. A total of 21 sites, mainly consisting of natural springs, were studied, and nine were sampled for analyzing associated free gas and C and H isotopes of CH4 and H2 when possible. Four springs showed gas with H2 occurrence, one particularly reaching 16% of H2 in the north of Kosova in a location named Vuçe, which makes it the fifth gas seep most enriched in H2 in the Dinarides, after H2-rich gas seeps in Serbia and Bosnia and Herzegovina. This gas seep is associated with hyperalkaline water having a pH of about 10.7. This would favor the assumption that H2 is derived from the serpentinization of peridotites, a process which is likely still ongoing. H2 is associated mainly with N2 and CH4, like the other H2-rich gas springs in the Dinarides. Based on C and H isotopes, CH4 is abiotic or microbial. H isotopes suggest a formation of H2 at about a 2-km depth. Another hyperalkaline spring was found in the south of Albania, at the border of the Korça Basin, with less than 200 ppm of H2. No relation between H2 and He was identified at the scale of Albania and Kosova, nor at the scale of the whole Dinarides. This work provides a completed map of the H2 occurrences in the Dinarides and allows to highlight some hot spots for H2 exploration, mainly located inside the ophiolite massifs like in other ophiolites (such as Oman, New Caledonia, and The Philippines), and not on major faults like in the Pyrenees.
Natural H2, also known as white hydrogen in hydrogen’s type classification, is a low impact and a potential low-cost energy (Moretti, 2019; Gaucher, 2020; Lapi et al., 2022) which could be present in large quantities in the subsurface (Zgonnik, 2020). From a couple of years, this resource is being increasingly studied in some countries such as Brazil (Prinzhofer et al., 2019) and Australia (Frery et al., 2021) and more recently in Europe, in Spain, and in France (Lefeuvre et al., 2022). Analogous with the oil and gas, natural hydrogen exploration can be approached through a “system” invoking source, migration, reservoir, trap, and seal to eventually lead to an accumulation in the subsurface. Hydrogen “source rock” is a rock able, by natural physicochemical processes, to generate hydrogen gas. Natural H2 can be generated by different processes where the five major ones are 1) reduction of water via rock alteration, 2) radiolysis, 3) degassing of magmas, 4) via a reaction with surface radicals in fault environment (Zgonnik, 2020; Moretti et al., 2021), or 5) maturation of the organic matter (Horsfield et al., 2022; Mahlstedt et al., 2022). Serpentinization is the most well-known process in the literature, in particular the alteration of peridotites taking place on the mid-ocean ridge (MOR) (Charlou et al., 2002; Proskurowski et al., 2006; Andreani et al., 2014; Worman et al., 2020). However, apart from exceptions such as Iceland (Combaudon et al., 2022) or Ethiopia (Pasquet et al., 2022), MOR is particularly difficult to study and explore as it is located in deep water environment. On the contrary, the ophiolites, remains of an obducted oceanic lithosphere, are accessible targets. Their H2 potential is, thus, far easier to apprehend.
Some ophiolites have been the subject of intense studies these last years, such as those in Oman and in New Caledonia, using direct and indirect techniques to constrain the H2 origin (Neal and Stanger, 1983; Neal and Stanger, 1985; Deville and Prinzhofer, 2016; Vacquand et al., 2018). The indirect techniques consist in using proxy like the presence of hyperalkaline springs which is often related to the serpentinization:
The closure of the Tethys Ocean leads to multiple ophiolite developments in Europe, especially in its southeastern part. We studied the Dinarides–Albanides which are a 1000-km long mountain range mainly made of ophiolites and covering Croatia, Bosnia and Herzegovina, Serbia, Kosova, Albania, and extending southward with Hellenides in Greece. In Serbia, a large amount of gas seeps or free gas bubbling in natural springs have been studied, and some sites contain up to 85% of H2 (Randazzo et al., 2021). However, no systematic study has been performed so far to inventory and characterize such gases in Albania and Kosova in the frame of H2 generation. The first step of the study consisted in listing and thoroughly locating all referenced gas occurrences at surface. Then, field acquisitions were realized (free gas, dissolved gas, and water sampling) to complete the existing dataset and provide a first overview of natural hydrogen potential associated with the Dinarides ophiolites.
The ophiolites from the Dinarides come from the suture of (i) one ocean or (ii) several ones, even if a consensus seems to appear in favor of the (i) model (Schmid et al., 2020). The ophiolitic oceanic crust and metamorphic soil were formed during the Middle Jurassic. This corresponds to the beginning of the closure of the Neo-Tethys Ocean initiated by the north east dipping subduction of the Adria plate under the Eurasian plate. Some rocks that are present in the ophiolite mélange trace the beginning of the obduction to the Late Jurassic. It lasted until the complete closure of the ocean forming the Sava Suture Zone which happened between the Late Cretaceous and Paleogene (Schmid et al., 2008; Maffione and van Hinsbergen, 2018; Schmid et al., 2020). During the shortening, the ophiolitic nappes have been thrusted onto about 200 km. The Tertiary slab roll back and possible break off resulted in Tertiary volcanism and intracontinental basins, mainly associated with normal faulting, filled with Miocene sediments (Schefer et al., 2011).
Studies conducted at surface in other areas suggest that hydrogen can be created by the interaction of water with mantle peridotites and migrate to the surface via major thrusts, like in the Pyrenees (Lefeuvre et al., 2022). Gas seeps or free gas bubbling in natural springs can also be found on the ophiolite massif itself, often associated with hyperalkaline waters (Vacquand et al., 2018). Some plutons can also act as a source of H2 via their alteration of radiolysis (Truche et al., 2020). In the Dinarides, the major structure of interest is the ophiolite of the West Vardar where some H2 has been measured in Bosnia and Herzegovina and Serbia with high content (Etiope et al., 2017; Randazzo et al., 2021) in free gas sampled in natural springs in ophiolite massifs. Therefore, the study will focus on the West Vardar zone in Albania and Kosova.
In Albania, the West Vardar ophiolite is represented by the Mirdita ophiolite, located in the Albanides mountain belt, a segment of the Dinarides–Albanides–Hellenides orogen (Figure 1). It is delimited on the east by the Pelagonian block and on the west by the Krasta, Cukali, and Kruja zone, considered as Adria-derived allochtons (Meshi et al., 2010; van Unen et al., 2019; Schmid et al., 2020). The Kruja zone is well-known for its geothermal potential because of deep thrust faults that allow hot fluids to reach the surface (Frasheri et al., 2009). The western massifs of the Mirdita ophiolite are mantle domes of a fossil oceanic core complex (OCC) (Nicolas et al., 2017) exhumed by a detachment rooted in the Moho transition zone under a slow-spreading MOR. The mantle is harzburgite, which is capped by mylonitic plagioclase-amphibole peridotite about 400 m thick. The eastern massifs of the Mirdita ophiolite are mainly harzburgite interpreted as derived from a supra-subduction zone [SSZ (Dilek et al., 2008)]. The thickness of the ophiolite can reach 14 km in its northeastern part, whereas on the west and southeast, it goes down to 2 km (Frasheri and Bushati, 2008). Some depressions in the ophiolite massifs have been filled with Tertiary deposits, leading to the formation of the basins of Korça and of Burrel. We can note that the ophiolite was little affected by alpine tectonics (Meshi et al., 2010).
FIGURE 1. (A) Geological map of the Dinarides modified from Schmid et al. (2020) and (B) associated cross-sections A-A′ and B-B′ crosscutting West Vardar. (C) Simplified map of the zone of interest with the major structures related to the H2 exploration: the ophiolites of West and East Vardar, the different basins, and the Kruja zone which is known as a geothermal area in Albania. The thrusts are also represented. CBDU, Cenozoic Basin of Dukagjin; CBDO, Cenozoic Basin of Kosova.
In Kosova, ophiolites outcrop on the eastern, center, southeastern, and northern part of the country, delimited by basin that can be filled by about 2 km of sediments. Apart from the southeastern ophiolite, the ophiolites are included in the West Vardar zone and are part of the Dinarides, in the continuity of Mirdita ophiolite. The central ophiolite of Kosova is represented by the massif of Golesh, bordered by Cenozoic basins (Elezaj, 2009), that suffered hydrothermal alteration (Ilich and Toshovich, 2005), leading to the precipitation of magnesite, currently exploited for their Mg content. The ophiolite in the north of Kosova is named Ibar. It was thrusted on the Jadar–Kopaonik block between Drina–Ivanjica and Serbo-Macedonian blocks (Toljić et al., 2019; Schmid et al., 2020; Spahic and Gaudenyi, 2020). It is mainly harzburgite and derived from an SSZ. Its thickness is not well constrained but based on some cross-sections, it can vary from several hundred (Miletić, 1995) of meters to ∼2 km (Schmid et al., 2020) even if gravimetric data are lacking to give a precise value. Some basins are present on the ophiolite filled with Miocene sediments or Tertiary volcanic rocks associated with some plutons that were exhumed in the Kopaonik area. This exhumation is to be related to a high erosion rate that occurred during the Neogene (Schefer et al., 2011). Gas occurrences in natural springs or in boreholes have been reported on the published exhaustive map of thermal water made by Çitaku et al. (2006). However, gas compositional or isotopic analyses were in general not performed. We can note the presence of one hyperalkaline spring in the north of Kosova (Çitaku et al., 2006).
In this part of the West Vardar zone, gas occurrences associated with natural springs are reported. However, only rare gas compositional analyses exist, and H2 was never measured. Based on the high number of gas seeps in Albania and Kosova, we focused our effort on the regions of interest which are the Kruja zone, on the boundary between ophiolites and Cenozoic basins in Albania and Kosova, and on hyperalkaline springs. We can note that only the Duvalo, located in North Macedonia site, consisted in a “dry mofette” (i.e., crack or opening in a volcanic area emitting CO2, water vapor, and other gases), with a low flow (Markoski et al., 2020). This site was analyzed because it was located near the zone of interest but without H2 measurements.
A total of 21 sites were analyzed, and different parameters such as temperature, pH, redox potential Eh, and weather conditions were systematically recorded. Free gas was collected from nine sites (Figure 2). For the other sites, the gas flow was too modest to collect a proper gas sample for laboratory analysis. The gas, bubbling into water at spring resurgence, was first collected in an open glass bottle reversed in water with progressive water flushed by gas. A funnel was also often used to collect bubbles faster. Once enough gas was collected, the bottle was closed with a septum cap in water. A volume of 15 ml of gas was then transferred with a syringe to a 10-ml glass tube. Three to five tubes were sampled in each site to enable major gas composition and isotopic analyses afterward at the lab.
FIGURE 2. (A–J) Pictures taken in some sampled locations. Geographical coordinates in WGS84 datum are reported in Table 1.
The occurrence of H2 in the gas was analyzed on the field using Geotech BIOGAS 5000 which measures in situ the gas composition of O2, CO2, CH4, and H2. Water vapor gas filters were always used during the measurements.
The composition of collected gas was obtained using gas chromatographic (GC) measurements carried out by 45-8 Energy (Metz, France) and ISOLAB (Neerijnen, Netherlands). H2, O2, N2, CH4, CO2, and He were measured by 45-8 Energy, and H2, O2, N2, CH4, C2–C6+, CO2, H2S, and Ar were measured by ISOLAB. The gases were analyzed by 45-8 Energy on an Agilent 990 microGC. To measure He, H2, Ne, O2, and N2, the microGC was equipped with a 20-m Molsieve column, a heated injection valve, a heated backflush valve, and a TCD detector with Ar as the carrier gas. To measure CO2 and CH4, it was equipped with a 10-m PoraPLOT-Q column for a heated injection valve, a heated backflush valve, and a TCD detector with He as the carrier gas.
In ISOLAB, H2, O2+Ar, N2, and CH4 were analyzed on an Agilent 7890A GC (Agilent Technologies, Santa Clara, US) equipped with a 12-m, 0.32-mm Molsieve column, a heated injection valve, and a TCD detector. Hydrocarbons and CO2 were analyzed on an Agilent 7890B GC equipped with a 50-m, 0.32-mm Porabond-Q column, a heated injection valve, a heated backflush valve (for C6+), a TCD detector (for CO2), and a FID detector (for hydrocarbons). From the results of all three detectors, one complete composition was calculated. Detector responses were calibrated several times a day using various reference standards.
Carbon and hydrogen isotopic signatures of CH4 and hydrogen isotopic analyses of H2 were undertaken using ISOLAB (Neerijnen, Netherlands). Carbon isotopes of CH4 were analyzed with an Agilent 6890N GC (Agilent Technologies, Santa Clara, US) interfaced to a Finnigan Delta SIRMS (Finnigan is now part of Thermo Scientific, Bremen, Germany) using a Finnigan GC-C II interface. The GC was equipped with a 12-m, 0.32-mm Molsieve column (Agilent) and an injection valve. Hydrogen isotopes of CH4 and H2 were analyzed on an Agilent 7890A GC (Agilent Technologies, Santa Clara, US) interfaced to a MAT 253 IRMS (Thermo Scientific, Bremen, Germany) using a GC-Isolink interface from Thermo. The GC was equipped with a 25-m, 0.32-mm Molsieve column (Agilent) and an injection valve. The samples were calibrated regularly against a calibration standard. The results are reported with the δ13C and δD notations, using vPDB and vSMOW standards.
The different parameters such as temperature, redox potential (Eh), and pH were systematically measured at every location where gas was bubbling in water (Table 1; Figure 3). The hottest spring exhibited between 55 °C and 65 °C and was observed near and in the Kruja Zone where some deep drilling reach about 2 km (Table 1), like Bilaj and Kozan wells. Llixha and Hydraj are located next to thermal stations that use the hot water of the well of Kozan. Therefore, these two springs are connected to the Kozan well and have similar chemical parameters. Only one spring was at high temperature up to 43 °C in Peshkopi in the northeastern part of Albania. Lower temperature springs with recorded temperature between 20 °C and 35 °C were analyzed in the south of Albania (Benjes and Leskovik) and in the east of Kosova (Doberçan). The Vuçe well with a depth of 60 m also showed moderate temperature of 32 °C, whereas the Vuçe spring is at 21 °C. The remaining springs showed cold waters below 20 °C.
TABLE 1. Location of the gas seeps with the coordinates in WGS84 datum, and parameters of the water and the weather conditions associated with sampling. The intensity of bubbling is qualitatively from low (+) to high (+++). Tout is for the temperature of the air during sampling. The hour was reported because some daily variations can sometimes appear (Prinzhofer et al., 2019; Cathles and Prinzhofer, 2020).
FIGURE 3. (A) Redox potential Eh against the temperature. We observe that for temperatures above 35 °C, the Eh is below −300 mV. (B) Redox potential Eh against pH. This highlights the presence of hyperalkaline springs in Vuçe and Ujë-i-Bardhë.
The Eh of the spring waters shows very low values down to −365 mV in locations where high temperatures were measured (Figures 3A, B, 4A). For spring waters with a temperature lower than 35 °C, this relation is less clear where the Eh values were mainly between −200 and 100 mV. Some high Eh values were recorded in Doberçan (123 mV) and VRB-3 (106 mV) locations.
FIGURE 4. (A) Temperature, (B) redox potential Eh, and (C) pH are represented on the map shown in Figure 1C.
The pH levels of bubbling waters were mainly between 6 and 8, whereas the springs from Ujë-i-Bardhë, located at the border of the Korça Basin and the ophiolite massif of Voskopoja in the south of Albania, and in Vuçe (spring and well), in the north of Kosova, have a pH at about ∼10.7. This does not seem related to temperature nor Eh (Figure 4).
The composition of major elements of the sampled gases are represented in Figures 5, 6 (Table 2), corrected from air contamination. The raw gas compositions of the gases are reported in Supplementary Table S1. We note that for the Kozan sample, H2S peak detected by 45-8 Energy’s GC could not be quantified (not calibrated for it). Therefore, for this sample, only data from ISOLAB are reported, without H2 and He quantification.
FIGURE 5. Gas compositions represented in ternary diagrams of (A) CO2–N2–CH4 and (B) H2–N2–CH4. For each diagram, the contents in H2 and CO2 are represented in red and green colors, respectively. See locations in Table 1.
FIGURE 6. Gas composition of the different gas seeps with the temperature and pH of the associated water represented on the map shown in Figure 1C. The white points represent the studied gas seeps.
TABLE 2. Gas compositions of N2, CH4, CO2, H2S, H2, and He with the associated air content used for air correction. The O2 content is not represented being at 0%. The symbol * is used on the sample ID when the analysis is an average between analyses from the GC of 45-8 Energy and ISOLAB, the symbol ** when the analysis only comes from ISOLAB and when it is only from 45-8 Energy there is not any symbol.
We can distinguish three types of gas: CH4-rich, CO2-rich, and N2-rich gas. It can be noted that Kozan and Vuçe also contain as a major gas 4.31% ± 0.01% of H2S and 16.13% ± 0.04% of H2, respectively.
H2 was also detected in the other gases with content down to several ppm except in Petroleja and Ujë-i-Bardhë that contain 195 ± 0.3 ppm and 369 ± 33.8 ppm, respectively. Kozan also contains several hundreds of ppm of H2 but as discussed in the methods, reported concentration is rather qualitative than quantitative. He was also detected as a minor gas. Its content is below 200 ppm for most of the gases, except from Torinë and Leskovik which have a He content of 1078 ± 8.3 ppm and 1676 ± 9.4 ppm. No relation is observed between H2 and He which seem to be de-correlated (Figure 7).
To constrain the biotic or abiotic origin of CH4, its C and H isotopic signatures have been measured (Table 3; Figure 8) when the gas contained enough CH4. We observe that Torinë and Petroleja contain microbial CH4, whereas CH4 from Kozan and Ujë-i-Bardhë seems thermogenic. The analyses related to Vuçe spring and Vuçe show close isotopic signatures of CH4 with δD values of −338 ± 2 and −336 ± 2 and δ13C values of −31.6 ± 0.2 and −30.6 ± 0.2, respectively. These isotopic values can be associated with abiotic CH4 related to serpentinization (Etiope, 2017) or to microbial CH4 (Xia and Gao, 2021; Xia and Gao, 2022). Finally, the measurement made in Leskovik gas shows an isotopic signature of CH4 that is out of the classical signatures reported in the literature. This would need more analyses to be confirmed.
TABLE 3. Isotopic data of CH4 and H2 and the estimated temperature of equilibration based on the equation of Horibe and Craig (1995).
FIGURE 8. δD of CH4 against δ13C of CH4. The different zones on the diagram are from Etiope (2017) apart from the range defining microbial isotopic signatures that was extended based on the study by Xia and Gao (2022).
The H isotopic signature of H2 was also measured in the gas of Vuçe spring and Vuçe well, in which H2 is abundant. The measurements revealed a δD of −728‰ ± 2‰ and −731‰ ± 2‰, respectively. Based on the equations of Horibe and Craig (1995), a temperature of about −70 °C can be estimated using the CH4–H2 couple and about −45 °C for the H2–H2O couple. The meaning of these temperatures will be explored in the discussion.
The obtained surface gas seeps dataset showed that, as anticipated, some locations exhibit a fraction of H2. We will first discuss which locations are promising for H2 exploration. The process at the origin of H2 will be then discussed, before concluding about the important locations for H2 exploration in the Dinarides.
Four locations showed the presence of H2 in the gas blend with more than 100 ppm in the area of interest: Kozan (−170 ppm before air correction), Petroleja (195 ± 0.3 ppm), Ujë-i-Bardhë (369 ± 33.8 ppm), and Vuçe (16.13% ± 0.04%). Associated with that, we can add that the gas flow is very important in Kozan, moderate in Vuçe and Ujë-i-Bardhë, and weak in Petroleja.
All these gases are associated with a variable fraction of CH4, the highest one being Petroleja and Ujë-i-Bardhë with more than 85% of CH4. Assessing the origin of CH4 is key to highlighting possible reactions between H2 and CO2 that would, therefore, suggest that more H2 has been/is being generated in the system but has been transformed into abiotic CH4. This could be the case in Vuçe where CH4 shows a potential abiotic signature and is likely to have formed via the Sabatier reaction:
However, the isotopic signature of CH4 in Vuçe can also be related to microbial origin (Xia and Gao, 2021; 2022). For Kozan and Petroleja, the C and H isotopic signatures of CH4 reveal a clear microbial origin, whereas for Ujë-i-Bardhë, it is thermogenic. The gas composition of Kozan in C1–C6+ (Supplementary Table S2) shows the presence of C2–C4 molecules which is consistent with the thermogenic origin. For Petroleja and Ujë-i-Bardhë, it cannot be ruled out that CH4 is the result of the mixing of CH4 of at least two different origins. The presence of C2 in both gases could be related to a thermogenic origin like in Kozan. Petroleja could thus be a mix between microbial and thermogenic CH4. Moreover, Ujë-i-Bardhë is a hyperalkaline spring, like Vuçe, with a pH of about 10.7. This high pH suggests that the process of serpentinization is likely to be currently ongoing (Barnes et al., 1967; Neal and Stanger, 1983; Abrajano et al., 1988; Deville and Prinzhofer, 2016), leading to the presence of OH− based on reaction [1]. Then, CH4 in Ujë-i-Bardhë could be a mix between CH4 coming from thermogenic and serpentinization processes.
The area where serpentinization seems to occur is in the West Vardar zone which is consistent with the presence of ophiolites. However, no potential abiotic CH4 was recorded in the Kruja zone which is a tectonic zone where thrusts go down to the ophiolites. The serpentinization seems, therefore, ongoing on the massif at rather low depth (<2 km), but more data are needed to know if it is also ongoing at great depth.
For the gas seeping out of the ophiolite massif in Vuçe and Ujë-i-Bardhë, where the water is hyperalkaline with a pH at about 10.7, we can infer that H2 is likely to come from serpentinization. However, Vuçe and Ujë-i-Bardhë have to be discussed separately to better constrain how the serpentinization process might proceed.
Ujë-i-Bardhë is located at the southeastern boundary of the Korça Basin with the outcropping ophiolite, known as the massif of Voskopoja. The ophiolite is rich in lherzolite and known as intensively altered in this zone (Hoeck et al., 2014). The associated CH4 is mainly thermogenic even if it can be partially abiotic. The fluid at the origin of the alteration could be recent meteoric water or an old aquifer. The gas is a blend with thermogenic CH4 that could have been formed in the Korça Basin itself and could then have migrated via the boundary of the Korça Basin with the ophiolite. The low content of H2 in the gas below 400 ppm could be related to a limited temperature slowing down the kinetics, or the fact that the peridotites are already highly serpentinized, limiting the process of serpentinization. A part of H2 could also have been combined with CO2 to form CH4 by an abiotic or biotic process, but as the isotopic signature of CH4 is mainly thermogenic, these processes seem limited.
The gas associated with Vuçe springs is located next to a thrust delimiting the ophiolite outcrop described as rich in harzburgite and Paleozoic gneiss belonging to the Jadar–Kopaonic block which constitutes the basement of the ophiolite (Supplementary Figure S1). H2 is abundant in the gas with about −16% of H2. By the presence of CH4, whatever its origin, we can assume that more H2 has been or is currently present in depth before the recombination of H2 with CO2. Based on the hydrogen isotopes of CH4 and H2, equilibrium temperatures of CH4–H2 and H2–H2O have been estimated to be about 70 °C and 45 °C, respectively. This means that the system CH4–H2–H2O is in disequilibrium. This is typical of low-temperature serpentinization (Pester et al., 2018), where CH4 and H2 isotopic exchange is slower than that between H2O and H2. Hence, the H2O–H2 geothermometer represents a temperature of re-equilibration (Pester et al., 2018) which is lower than the CH4–H2 geothermometer. This latter geothermometer represents a temperature of equilibration between H2 and CH4 which can be associated with the alteration of H2 into CH4. This means that at 70 °C, H2 was already formed. This temperature corresponds to a minimal temperature of serpentinization. Using a mean geothermal gradient of 30 °C/km, we can estimate that H2 could have already been formed at about 2.3 km. The high pH suggests that this process is still ongoing. The thrust between the ophiolite and the gneiss is a favored path for gas.
The origin of H2 in Kozan can be related to the thermogenic process where H2 can be formed at high temperatures (Li et al., 2015; Horsfield et al., 2022). It is possible that a part of H2 led to the formation of H2S. Finally, we can propose that the H2 in Petroleja could have been formed by thermogenics and/or bacteria. More work would be necessary to better constrain the origin of H2 for Kozan and Petroleja. The origin of other sources with a low content of H2 will not be discussed.
Based on this study and several other gas geochemistry studies conducted in the Dinarides area (Minissale et al., 1989; Daskalopoulou, 2017; Etiope et al., 2017; Randazzo et al., 2021), we now are able to fill in the map of H2 occurrences in this region (Figure 9). To compare the data, we have corrected all the data in the literature from the air contamination. We observe that some hot spots for natural hydrogen appear in Bosnia and Herzegovina (8%–48% H2), southeast Serbia (32%–85% H2), and in the north of Kosova in Vuçe (16% H2). H2 is mainly associated with N2 with several percent of CH4 (Figure 10A). Regardless of the gas flow rate in the analyzed springs (which is in general modest and therefore could not be precisely measured), these locations are great to be considered in an H2 exploration. Most of these H2-rich springs are hyperalkaline and embedded in an ophiolite, therefore, inferring that H2 is likely to be derived from serpentinization. Based on equilibrium temperatures of CH4–H2 and H2–H2O (Etiope et al., 2017) and despite re-equilibration, the serpentinization seems to occur at low temperatures (<100 °C).
FIGURE 9. Compilation of the H2 content in gas seeps in the Dinarides from this study and others in the literature (Minissale et al., 1989; Daskalopoulou, 2017; Etiope et al., 2017; Randazzo et al., 2021).
FIGURE 10. Compilation of gas compositions in the Dinarides represented in (A) ternary diagram of H2–N2–CH4 in addition to gas compositions in Oman, New Caledonia, The Philippines, and Turkey (Vacquand et al., 2018) and plotted in (B) graph of H2 content against He content.
It can be noted that the H2-rich gases sampled in the Dinarides have comparable chemical compositions with the gases studied in other ophiolites such as those in Oman, New Caledonia, or The Philippines [Figure 10 (Vacquand et al., 2018)]. We can add that at the scale of the Dinarides, like for Albania and Kosova, H2 does not seem related to He (Figure 10B). Therefore, they are de-correlated and have different sources, H2 coming from serpentinization and He from crustal and/or mantle sources (Randazzo et al., 2021).
New gas measurements associated with natural springs in Albania and Kosova were made including H2 data. Different types of springs were measured: CO2-rich, N2-rich, and CH4-rich. Four springs with H2 occurrence were analyzed. Three of them contained several hundred ppm of H2, and one, 16% of H2. This latter spring was sampled in the north of Kosova in a location named Vuçe. The main process at the origin of H2 is likely to be serpentinization, occurring at about a 2-km depth based on H isotopic signatures and assuming normal geothermal gradient. No relation was found with He. With this new discovery, five seeps with more than 10% of H2 are now referenced in Bosnia and Herzegovina, Serbia, and Kosova.
This study completes data at the scale of the Dinarides and shows that H2 gas seeps are mainly located inside ophiolite massifs and not on major faults in the Kruja zone where the gas could have migrated like it is suggested in the Pyrenees (Lefeuvre et al., 2022). More work is now needed to better understand how the H2-rich gas migrates to the surface and locate the potential gas traps in order to look for a reservoir of natural H2.
The original contributions presented in the study are included in the article/Supplementary Material; further inquiries can be directed to the corresponding author.
DL wrote the paper with the contribution of all co-authors. The fieldtrip was made by DL and MB-M who acquired field data, guided by AM in Albania and by IF in Kosova. The analyses on the GC from 45-8 Energy were conducted by MB-M and TG. BH and NP led the direction of the project.
This study was funded by the program France Relance and 45-8 Energy.
The authors thank the program France Relance for funding the project that was a collaboration between the University of Pau (UPPA) and 45-8 Energy. The authors also thank all the persons who allowed us to have access to sampling and data like Fidaim Sahiti and Ivan Kovacevic and Bart van der Haven for the discussion about the data. The authors finally thank Isabelle Moretti for the useful discussions about the project and all the reviewers for their useful comments.
MB-M, TG, BH, and NP were employed by the company 45-8 Energy.
The remaining authors declare that the research was conducted in the absence of any commercial or financial relationships that could be construed as a potential conflict of interest.
All claims expressed in this article are solely those of the authors and do not necessarily represent those of their affiliated organizations, or those of the publisher, the editors, and the reviewers. Any product that may be evaluated in this article, or claim that may be made by its manufacturer, is not guaranteed or endorsed by the publisher.
The Supplementary Material for this article can be found online at: https://www.frontiersin.org/articles/10.3389/feart.2023.1167634/full#supplementary-material
Abrajano, T. A., Sturchio, N. C., Bohlke, J. K., Lyon, G. L., Poreda, R. J., and Stevens, C. M. (1988). Methane-hydrogen gas seeps, Zambales Ophiolite, Philippines: Deep or shallow origin? Chem. Geol. 71, 211–222. doi:10.1016/0009-2541(88)90116-7
Andreani, M., Escartin, J., Delacour, A., Ildefonse, B., Godard, M., Dyment, J., et al. (2014). Tectonic structure, lithology, and hydrothermal signature of the Rainbow massif (Mid-Atlantic Ridge 36°14′N). Geochem. Geophys. Geosyst. 15, 3543–3571. doi:10.1002/2014GC005269
Barnes, I., Lamarche, V. C., and Himmelberg, G. (1967). Geochemical evidence of present-day serpentinization. Science 156, 830–832. doi:10.1126/science.156.3776.830
Cathles, L., and Prinzhofer, A. (2020). What pulsating H2 emissions suggest about the H2 resource in the sao francisco Basin of Brazil. Geosciences 10, 149. doi:10.3390/geosciences10040149
Charlou, J. L., Donval, J. P., Fouquet, Y., Jean-Baptiste, P., and Holm, N. (2002). Geochemistry of high H2 and CH4 vent fluids issuing from ultramafic rocks at the Rainbow hydrothermal field (36°14′N, MAR). Chem. Geol. 191, 345–359. doi:10.1016/S0009-2541(02)00134-1
Çitaku, L., Knobloch, A., Legler, C., and Dickmayer, K. (2006). Map of mineral and thermal water in Kosovo.
Combaudon, V., Moretti, I., Kleine, B. I., and Stefánsson, A. (2022). Hydrogen emissions from hydrothermal fields in Iceland and comparison with the Mid-Atlantic Ridge. Int. J. Hydrogen Energy 47, 10217–10227. doi:10.1016/j.ijhydene.2022.01.101
Deville, E., and Prinzhofer, A. (2016). The origin of N2-H2-CH4-rich natural gas seepages in ophiolitic context: A major and noble gases study of fluid seepages in New Caledonia. Chem. Geol. 440, 139–147. doi:10.1016/j.chemgeo.2016.06.011
Dilek, Y., Furnes, H., and Shallo, M. (2008). Geochemistry of the Jurassic Mirdita Ophiolite (Albania) and the MORB to SSZ evolution of a marginal basin oceanic crust. Lithos 100, 174–209. doi:10.1016/j.lithos.2007.06.026
Elezaj, Z. (2009). Cenozoic molassse basins in Kosovo and their geodynamic evolution. Geosphere 8, 1. doi:10.1130/GES00006.1
Etiope, G. (2017). Abiotic methane in continental serpentinization sites: An overview. Procedia Earth Planet. Sci. 17, 9–12. doi:10.1016/j.proeps.2016.12.006
Etiope, G., Samardžić, N., Grassa, F., Hrvatović, H., Miošić, N., and Skopljak, F. (2017). Methane and hydrogen in hyperalkaline groundwaters of the serpentinized Dinaride ophiolite belt, Bosnia and Herzegovina. Appl. Geochem. 84, 286–296. doi:10.1016/j.apgeochem.2017.07.006
Frasheri, A., and Bushati, S. (2008). “Albanides, a part of the alpine mediterranean folded belt, in the ligt of the geophysical studies,” in 70th EAGE conference 48.
Frasheri, A., Bushati, S., and Bare, V. (2009). Geophysical outlook on structure of the Albanides. Journal of the Balkan geophysical society 22, 9–30.
Frery, E., Langhi, L., Maison, M., and Moretti, I. (2021). Natural hydrogen seeps identified in the North perth basin, western Australia. Int. J. Hydrogen Energy 46, 31158–31173. doi:10.1016/j.ijhydene.2021.07.023
Gaucher, E. C. (2020). New perspectives in the industrial exploration for native hydrogen. Elements 16, 8–9. doi:10.2138/gselements.16.1.8
Hoeck, V., Ionescu, C., and Onuzi, K. (2014). Southern Albanian ophiolites. Bul. i Skhencave Gjeol. 6, 48.
Horibe, Y., and Craig, H. (1995). D/H fractionation in the system methane-hydrogen-water. Geochimica Cosmochimica Acta 9, 5209. doi:10.1016/0016-7037(95)00391-6
Horsfield, B., Mahlstedt, N., Weniger, P., Misch, D., Vranjes-Wessely, S., Han, S., et al. (2022). Molecular hydrogen from organic sources in the deep Songliao Basin, P.R. China. Int. J. Hydrogen Energy 47, 16750–16774. doi:10.1016/j.ijhydene.2022.02.208
Ilich, M., and Toshovich, R. (2005). Geology and origin of the Golesh vein magnesite depost: A brief survey. Geologica Carpatica 7.
Lapi, T., Chatzimpiros, P., Raineau, L., and Prinzhofer, A. (2022). System approach to natural versus manufactured hydrogen: An interdisciplinary perspective on a new primary energy source. Int. J. Hydrogen Energy 47, 21701–21712. doi:10.1016/j.ijhydene.2022.05.039
Lefeuvre, N., Truche, L., Donzé, F.-V., Gal, F., Tremosa, J., Fakoury, R.-A., et al. (2022). Natural hydrogen migration along thrust faults in foothill basins: The North Pyrenean Frontal Thrust case study. Appl. Geochem. 105396, 105396. doi:10.1016/j.apgeochem.2022.105396
Li, X., Krooss, B. M., Weniger, P., and Littke, R. (2015). Liberation of molecular hydrogen (H2) and methane (CH4) during non-isothermal pyrolysis of shales and coals: Systematics and quantification. Int. J. Coal Geol. 137, 152–164. doi:10.1016/j.coal.2014.11.011
Maffione, M., and van Hinsbergen, D. J. J. (2018). Reconstructing Plate boundaries in the jurassic neo-tethys from the East and West Vardar ophiolites (Greece and Serbia). Tectonics 37, 858–887. doi:10.1002/2017TC004790
Mahlstedt, N., Horsfield, B., Weniger, P., Misch, D., Shi, X., Noah, M., et al. (2022). Molecular hydrogen from organic sources in geological systems. J. Nat. Gas Sci. Eng. 105, 104704. doi:10.1016/j.jngse.2022.104704
Markoski, B., Jovanovski, M., and Peshevski, I. (2020). Duvalo: A geological phenomenon near orhid. AGB 6, 43–56. doi:10.18509/AGB.2020.05
Meshi, A., Boudier, F., Nicolas, A., and Milushi, I. (2010). Structure and tectonics of lower crustal and upper mantle rocks in the Jurassic Mirdita ophiolites, Albania. Int. Geol. Rev. 52, 117–141. doi:10.1080/00206810902823982
Miletić, I. (1995). “The structure of the lead and zinc deposit “Crnac.”,” in Geology and Metallogeny of the Kopaonik Mt. Symposium, 299–304.
Minissale, A., Duchi, V., Kolios, N., and Totaro, G. (1989). Geochemical characteristics of Greek thermal springs. J. Volcanol. Geotherm. Res. 39, 1–16. doi:10.1016/0377-0273(89)90017-6
Moretti, i. (2019). H2: Energy vector or source? L’Actualité chimique. Available at: https://hal-univ-pau.archives-ouvertes.fr/hal-02187452 (Accessed June 2, 2022).
Moretti, I., Brouilly, E., Loiseau, K., Prinzhofer, A., and Deville, E. (2021). Hydrogen emanations in intracratonic areas: New guide lines for early exploration basin screening. Geosciences 11, 145. doi:10.3390/geosciences11030145
Neal, C., and Stanger, G. (1983). Hydrogen generation from mantle source rocks in Oman. Earth Planet. Sci. Lett. 66, 315–320. doi:10.1016/0012-821X(83)90144-9
Neal, C., and Stanger, G. (1985). “Past and present serpentinisation of ultramafic rocks; an example from the semail ophiolite nappe of northern Oman,” in The Chemistry of weathering nato ASI series. Editor J. I. Drever (Dordrecht: Springer Netherlands), 249–275. doi:10.1007/978-94-009-5333-8_15
Nicolas, A., Meshi, A., Boudier, F., Jousselin, D., and Muceku, B. (2017). Mylonites in ophiolite of Mirdita (Albania): Oceanic detachment shear zone. Geosphere 13, 136–154. doi:10.1130/GES01383.1
Pasquet, G., Houssein Hassan, R., Sissmann, O., Varet, J., and Moretti, I. (2022). An attempt to study natural H2 resources across an oceanic ridge penetrating a continent: The asal–ghoubbet rift (republic of Djibouti). Geosciences 12, 16. doi:10.3390/geosciences12010016
Pester, N. J., Conrad, M. E., Knauss, K. G., and DePaolo, D. J. (2018). Kinetics of D/H isotope fractionation between molecular hydrogen and water. Geochimica Cosmochimica Acta 242, 191–212. doi:10.1016/j.gca.2018.09.015
Prinzhofer, A., Moretti, I., Françolin, J., Pacheco, C., D’Agostino, A., Werly, J., et al. (2019). Natural hydrogen continuous emission from sedimentary basins: The example of a Brazilian H2-emitting structure. Int. J. Hydrogen Energy 44, 5676–5685. doi:10.1016/j.ijhydene.2019.01.119
Proskurowski, G., Lilley, M. D., Kelley, D. S., and Olson, E. J. (2006). Low temperature volatile production at the Lost City Hydrothermal Field, evidence from a hydrogen stable isotope geothermometer. Chem. Geol. 229, 331–343. doi:10.1016/j.chemgeo.2005.11.005
Randazzo, P., Caracausi, A., Aiuppa, A., Cardellini, C., Chiodini, G., D’Alessandro, W., et al. (2021). Active degassing of deeply sourced fluids in central Europe: New evidences from a geochemical study in Serbia. Geochem Geophys Geosyst 22. doi:10.1029/2021GC010017
Schefer, S., Cvetković, V., Fügenschuh, B., Kounov, A., Ovtcharova, M., Schaltegger, U., et al. (2011). Cenozoic granitoids in the Dinarides of southern Serbia: Age of intrusion, isotope geochemistry, exhumation history and significance for the geodynamic evolution of the balkan peninsula. Int. J. Earth Sci. Geol. Rundsch) 100, 1181–1206. doi:10.1007/s00531-010-0599-x
Schmid, S. M., Bernoulli, D., Fügenschuh, B., Matenco, L., Schefer, S., Schuster, R., et al. (2008). The alpine-carpathian-dinaridic orogenic system: Correlation and evolution of tectonic units. Swiss J. Geosci. 101, 139–183. doi:10.1007/s00015-008-1247-3
Schmid, S. M., Fügenschuh, B., Kounov, A., Maţenco, L., Nievergelt, P., Oberhänsli, R., et al. (2020). Tectonic units of the alpine collision zone between eastern alps and Western Turkey. Gondwana Res. 78, 308–374. doi:10.1016/j.gr.2019.07.005
Spahic, D., and Gaudenyi, T. (2020). Reconsidering paleozoic differences between the Jadar block and the Drina-Ivanjica unit. Geol Balk Poluos 81, 1–9. doi:10.2298/GABP191014002S
Toljić, M., Stojadinović, U., and Krstekanić, N. (2019). “Vardar zone: New insights into the tectono-depositional subdivisions,” in Congress of geologists of Bosnia and Herzegovina 16.
Truche, L., McCollom, T. M., and Martinez, I. (2020). Hydrogen and abiotic hydrocarbons: Molecules that change the world. Elements 16, 13–18. doi:10.2138/gselements.16.1.13
Vacquand, C., Deville, E., Beaumont, V., Guyot, F., Sissmann, O., Pillot, D., et al. (2018). Reduced gas seepages in ophiolitic complexes: Evidences for multiple origins of the H2-CH4-N2 gas mixtures. Geochimica Cosmochimica Acta 223, 437–461. doi:10.1016/j.gca.2017.12.018
van Unen, M., Matenco, L., Nader, F. H., Darnault, R., Mandic, O., and Demir, V. (2019). Kinematics of foreland-vergent crustal accretion: Inferences from the Dinarides evolution. Tectonics 38, 49–76. doi:10.1029/2018TC005066
Worman, S. L., Pratson, L. F., Karson, J. A., and Schlesinger, W. H. (2020). Abiotic hydrogen (H2) sources and sinks near the Mid-Ocean Ridge (MOR) with implications for the subseafloor biosphere. PNAS 117, 13283–13293. doi:10.1073/pnas.2002619117
Xia, X., and Gao, Y. (2021). Methane from microbial hydrogenolysis of sediment organic matter before the Great Oxidation Event. Nat. Commun. 12, 5032. doi:10.1038/s41467-021-25336-6
Xia, X., and Gao, Y. (2022). Validity of geochemical signatures of abiotic hydrocarbon gases on Earth. JGS 179, jgs2021–077. doi:10.1144/jgs2021-077
Keywords: H2, Albania, Kosova, Dinarides, ophiolite, serpentinization
Citation: Lévy D, Boka-Mene M, Meshi A, Fejza I, Guermont T, Hauville B and Pelissier N (2023) Looking for natural hydrogen in Albania and Kosova. Front. Earth Sci. 11:1167634. doi: 10.3389/feart.2023.1167634
Received: 16 February 2023; Accepted: 27 March 2023;
Published: 17 April 2023.
Edited by:
Mahmoud Leila, Mansoura University, EgyptReviewed by:
Laurent Truche, Université Grenoble Alpes, FranceCopyright © 2023 Lévy, Boka-Mene, Meshi, Fejza, Guermont, Hauville and Pelissier. This is an open-access article distributed under the terms of the Creative Commons Attribution License (CC BY). The use, distribution or reproduction in other forums is permitted, provided the original author(s) and the copyright owner(s) are credited and that the original publication in this journal is cited, in accordance with accepted academic practice. No use, distribution or reproduction is permitted which does not comply with these terms.
*Correspondence: Dan Lévy, ZGxldnlAaXBncC5mcg==
†Present address: Dan Lévy, IPGP, Institut Physique du Globe de Paris, Paris, France
Disclaimer: All claims expressed in this article are solely those of the authors and do not necessarily represent those of their affiliated organizations, or those of the publisher, the editors and the reviewers. Any product that may be evaluated in this article or claim that may be made by its manufacturer is not guaranteed or endorsed by the publisher.
Research integrity at Frontiers
Learn more about the work of our research integrity team to safeguard the quality of each article we publish.