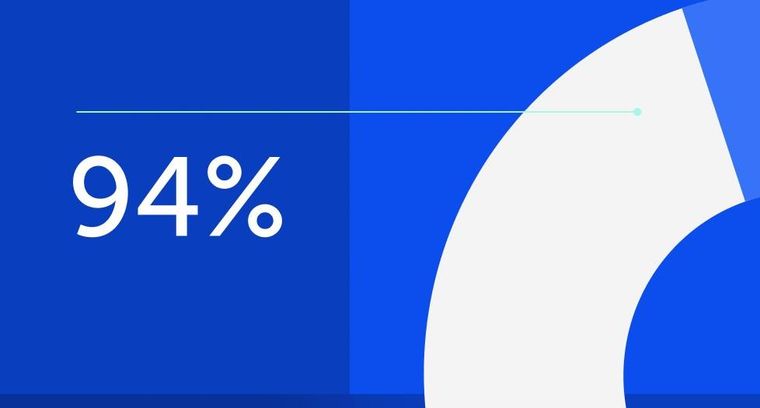
94% of researchers rate our articles as excellent or good
Learn more about the work of our research integrity team to safeguard the quality of each article we publish.
Find out more
ORIGINAL RESEARCH article
Front. Earth Sci., 09 March 2023
Sec. Economic Geology
Volume 11 - 2023 | https://doi.org/10.3389/feart.2023.1162994
This article is part of the Research TopicAccessory Mineral Geochemistry and Its Application in Mineral ExplorationView all 5 articles
The Kalmakyr deposit in Uzbekistan is one of the world’s largest gold-rich porphyry Cu deposits, and mineralization was proposed to be closely associated with the emplacement of the latest intrusive phases, that is, granodiorite porphyry. However, it is still unclear which features of these ore-bearing intrusions are crucial for the enormous metal enrichment and how they correlate with magmatic fertility. In this study, we address these questions by comparing the petrological and in situ major and trace element geochemistry of apatite for the ore-bearing and ore-barren intrusions. Magmatic apatite from the ore-bearing granodiorite porphyry has higher Eu/Eu* (0.50–0.58), Sr/Y (1.09–1.64), and La/Yb (36.19–53.42) ratios than the ore-barren quartz porphyry (0.01–0.02, 0.02–0.03, and 2.01–3.75, respectively) and monzonite (0.20–0.11, 0.36–1.12, and 19.71–56.32, respectively). Moreover, the ore-bearing granodioritic magma has higher S (average of 111 ppm) and Cl (average of 8602 ppm) contents than the magma of quartz porphyry (S contents average of 9 ppm; Cl contents average of 2652 ppm) and monzonite (S contents average of 9 ppm; Cl contents average of 8483 ppm). These features collectively suggest that the ore-bearing granodiorite porphyry has relatively high oxygen fugacity, H2O, Cl, and S contents compared to those from the ore-barren intrusions. By comparing our data with the published dataset from porphyry Cu–Mo and Mo-only deposits in the world, we suggest that the high Cl content and low F/Cl ratio in magmatic apatite might be an important indicator to prospect for gold-rich porphyry Cu deposits.
Gold-rich porphyry Cu deposits, an essential source of gold and copper in the world, are usually associated with calc-alkaline magma, accompanied by multiphase intermediate–felsic intrusions (Sillitoe, 2010; Chiaradia, 2014; Lipson, 2014). It is noteworthy that many of these deposits appear to be related to a particular intrusive phase (Cooke et al., 2005; Chiaradia et al., 2013; Chiaradia et al., 2014; Qin et al., 2022). Although the tectono-magmatic setting is thought to be a pre-requisite for the onset of economic mineralization (Cooke et al., 2005; Sillitoe, 2010), questions as to which features of the evolving magma control mineralization potential and how they correlate with diverse metal associations are still not fully understood.
The Kalmakyr porphyry Cu-Au deposit in eastern Uzbekistan (Figure 1) has the third-largest gold endowment among all porphyry Cu deposits in the world (Cooke et al., 2005; Seltmann et al., 2014; Zhao et al., 2017). It contains measured and indicated resources of 2000 Mt of ore grading 0.4% Cu and 0.6 g/t Au (Golovanov et al., 2005). Previous studies have indicated that Cu and Au mineralization at Kalmakyr is genetically related to the latest intrusive phase, i.e., the late Carboniferous granodiorite porphyry (Golovanov et al., 2005; Zhao et al., 2017). However, the physiochemical conditions, evolution processes, and magmatic fertility of different intrusive phases at the deposit remain enigmatic and require thorough exploration. Apatite is a common accessory mineral in porphyry-associated intrusions and is resistant to physical or chemical weathering and most types of hydrothermal alteration (Sha and Chappell, 1999; Pan and Fleet, 2002; Bouzari et al., 2016; Zhong et al., 2018a; Zhong et al., 2021b). Its geochemical composition can provide diagnostic information on magma composition, physiochemical condition, evolution processes, and magmatic fertility (Bouzari et al., 2016; Chelle-Michou and Chiaradia, 2017; Xu et al., 2021).
FIGURE 1. Simplified geological map of the Tien Shan orogenic belt showing the location of major copper and gold deposits (modified from (Zhao et al., 2017). The area shown in Figure 2 is marked.
This paper presents the results of in situ major and trace element geochemical analyses for apatite from the ore-barren and ore-bearing porphyry intrusions of the giant Kalmakyr porphyry Cu-Au deposit. The goal was to explore the critical features of the ore-bearing magma. These results are further integrated with available apatite geochemical data from porphyry Cu-Mo and Mo deposits to define controlling factors that resulted in diverse metal associations in porphyry deposits.
The Tien Shan orogenic belt in Uzbekistan and Kyrgyzstan comprises three suture-bounded tectonic domains, i.e., the northern, middle, and southern Tien Shan (Figure 1; Yakubchuk et al., 2002; Biske and Seltmann, 2010; Zhao et al., 2015; Zhao et al., 2022). These tectonic domains were displaced by the NW-striking Talas–Fergana strike-slip fault during the Mesozoic (Biske and Seltmann, 2010). The Kalmakyr deposit is situated within the Valerianov–Beltau–Kurama volcano-plutonic arc of the Middle Tien Shan (Figure 1; Golovanov et al., 2005). This arc is accepted to be an Andean-type arc that was formed by the northward subduction of the Turkestan Ocean during the late Paleozoic (Seltmann et al., 2011).
Porphyry Cu-Au mineralization at the Kalmakyr deposit is hosted by a late Paleozoic intrusive complex, which consists of late Devonian quartz porphyry (421 ± 4 Ma; U-Pb zircon age), middle Carboniferous monzonite (327.2 ± 5.6 Ma; U-Pb zircon age), and late Carboniferous granodiorite porphyry (313.6 ± 2.8 Ma; U-Pb zircon age; Zhao et al., 2017). The pre-mineralization quartz porphyry is exposed at the southern end of the deposit area (Figure 2). It contains phenocrysts of quartz, plagioclase, and biotite in a fine-grained quartz and feldspar groundmass (Figures 3A, D). Monzonite also undergoes pre-mineralization (Golovanov et al., 2005; Zhao et al., 2017), although it hosts most of the high-grade mineralization (Figure 2). It crops out in the central and northern sectors and gradually transitions to diorite in the western sector of the deposit area (Figure 2). Monzonite contains significant amounts of coarse-grained plagioclase and K-feldspar, with minor amounts of biotite, hornblende, and quartz (Figures 3B, E). The granodiorite porphyry represents the latest intrusive phase of the deposit and is thought to be part of a larger, deep-seated intrusion with only limited exposure at the surface (Golovanov et al., 2005). This unit comprises phenocrysts of plagioclase, quartz, K-feldspar, hornblende, and biotite within a felsic groundmass (Figures 3C, F).
FIGURE 2. (A) Simplified geological map; and (B) Cross section of the Kalmakyr giant porphyry Cu-Au deposit [modified from (Golovanov et al., 2005) and (Zhao et al., 2017)].
FIGURE 3. Photographs and photomicrographs showing the main texture and mineral assemblages of three major intrusions of the Kalmakyr deposit. (A) Quartz porphyry; (B) Monzonite; (C) Granodiorite porphyry; (D) Anhedral quartz phenocrysts of quartz porphyry under cross-polarized light; (E) Euhedral to subhedral, plagioclase, and K-feldspar, and minor biotite, hornblende, and quartz phenocrysts in monzonite under cross-polarized light; (F) Plagioclase phenocryst partially rimmed by K-feldspar in granodiorite porphyry under cross-polarized light. Mineral abbreviations: Qtz, quartz; Pl, plagioclase; Kfs, K-feldspar; Hb, hornblende; Bio, biotite.
Hydrothermal alteration and Cu-Au mineralization occur widely in the deposit area and are associated with the emplacement of the granodiorite porphyry (Golovanov et al., 2005; Zhao et al., 2017). Upward and outward from the barren core of granodiorite porphyry, hydrothermal alteration shows zonation from early K-silicate to the ore-hosting phyllic zone and later the propylitic zone (Golovanov et al., 2005). The phyllic alteration contains the quartz-sericite and quartz-sericite-chlorite-biotite zones, with extensive Cu and Au mineralization and high-grade ores spatially related to the latter zone (Golovanov et al., 2005; Turamuratov et al., 2011). Mineralization is characterized by stockwork and veinlets of chalcopyrite, pyrite, and molybdenite, as is common in many gold-rich porphyry Cu deposits (Sillitoe, 2010; Li et al., 2022; Yang et al., 2022; Tang et al., 2023).
Intrusive samples with limited alteration were collected from outcrops around the Kalmakyr open pit for apatite geochemical analyses. The granodiorite porphyry (samples KM-2, KM-3, KM-4, and KM-6) is regarded as ore-bearing, whereas quartz porphyry (sample KM-12) and monzonite (samples KM-24, KM-25, and KM-26) represent ore-barren intrusions. All samples were polished to 100-μm-thick and -thin sections for petrological and in situ geochemical analyses, respectively. Apatite grains were selected by optical and backscattered electron (BSE) microscopy. Major and trace element geochemistry of apatite grains were analyzed in situ by electron microprobe (EMP) analyses and laser ablation inductively coupled plasma mass spectrometry (LA-ICP-MS), respectively.
A JEOL-JXA8100 electron microprobe performed BSE imaging and the EMP analyses of apatite with a 15-kV accelerating voltage, 10-nA beam current, and 3-μm probe beam at the MNR Key Laboratory of Metallogeny and Mineral Assessment, Institute of Mineral Resources, Chinese Academy of Geological Sciences, Beijing. To detect the characteristic X-ray patterns, 20 s of counting time was chosen for Si, Al, Ti, Fe, Ma, Mn, K, Na, Ba, Cl, and S, 40 s for F, and 10 s for Ca and P. Trace element contents in apatite were analyzed by LA-ICP-MS at the Ore Deposit and Exploration Centre, Hefei University of Technology. The analyses consist of an Agilent 7900 ICP-MS instrument and a Cetac Analyte HE laser ablation system with helium (0.9 L min−1) as the carrier gas and argon (0.87 L min−1) as the compensation gas to enhance the sensitivity, and they mixed via a T-connector before entering the ICP. After testing the gas blank for 20 s, the laser was run for 40 s at a constant repetition rate of 7 Hz with an energy density of 4 J cm−2 and a beam aperture of 30 μm. NIST 610, NIST 612, and BCR 2G, as the external standards, were run after every 10 analyses. Measured by EMP analyses, the Ca concentrations were used as the internal standards for apatite. The detection limits were calculated for each element in each spot analysis. The analysis accuracy of most trace elements is greater than 10%. The data reduction and processing were carried out using ICPMSDataCal software (Liu et al., 2008).
Typical microscopic and BSE images of apatite from quartz porphyry, monzonite, and granodiorite porphyry of the Kalmakyr deposit are presented in Figure 4. Apatite grains from quartz porphyry are euhedral to subhedral columnar crystals of 50–200 μm in length, occurring mostly as inclusions in biotite phenocryst (Figures 4A, B). The majority of these apatite grains display a homogenous texture with a weak core-rim texture and few gaps or cracks in the BSE images (Figures 4B, C). Apatite crystals from the monzonite are euhedral to subhedral, with prismatic and granular crystals of 25–120 μm in size (Figures 4D, E). They occur as inclusions in plagioclase and hornblende, and some are also dispersed in the groundmass (Figures 4D, E). In BSE images, some apatite grains have a homogeneous texture, and some of the apatite grains have mineral or fluid inclusions (Figures 4E, F). Apatite grains from the granodiorite porphyry are euhedral to subhedral columnar and granular crystals of 20–70 μm in size, which are commonly wrapped by plagioclase and biotite (Figures 4G, H). These apatite grains show a homogeneous texture with an inclusion-free surface (Figures 4H, I).
FIGURE 4. Plane-polarized photomicrographs (A, D, G) and BSE images (B, C, E, F, H, I) illustrating petrographic features of representative apatite from the Kalmakyr porphyry deposit. (A) Euhedral to subhedral, elongated and columnar magmatic apatite occurs as inclusions in quartz porphyry’s biotite; (B) Euhedral to subhedral, homogeneous magmatic apatite occurs as inclusions in quartz porphyry’s biotite; (C) Unaltered magmatic apatite, displaying a weak core–rim texture and the homogeneous surface of quartz porphyry; (D) Euhedral to subhedral, prismatic and granular magmatic apatite occurs as inclusions in the plagioclase phenocryst of monzonite; (E) Euhedral to subhedral magmatic apatite with minor mineral inclusions of monzonite; (F) Granular magmatic apatite of monzonite; (G) Euhedral to subhedral, columnar magmatic apatite occurs as inclusions in the plagioclase phenocryst of granodiorite porphyry; (H) Euhedral to subhedral, columnar and granular magmatic apatite occurs as inclusions in the biotite phenocryst of granodiorite porphyry; and (I) Columnar magmatic apatite with a homogeneous surface of granodiorite porphyry. Mineral abbreviations: Ap, apatite; Bio, biotite; Pl, plagioclase; Mag, magnetite.
Apatite major and trace element compositions from the ore-bearing and ore-barren intrusions of the Kalmakyr deposit are summarized in Supplementary Table S1. A total of 27 apatite grains, including 7 grains for granodiorite porphyry, 6 grains for quartz porphyry, and 14 grains for monzonite, were selected for EMP analyses and LA-ICP-MS in this study.
Apatite grains from the granodiorite porphyry, quartz porphyry, and monzonite have similar CaO (53.74–55.48 wt%, 52.05–54.46 wt%, and 53.46–55.28 wt%, respectively), P2O5 (39.99–41.31 wt%, 40.55–41.56 wt%, and 39.56–41.94 wt%, respectively), Na2O (0–0.016 wt%, 0.25–0.35 wt%, and 0–0.16 wt%, respectively), and MnO contents (0.11–0.17 wt%, 0.12–0.22 wt%, and 0–0.18 wt%, respectively). The K2O contents are usually close to or below their detection limits. According to the SiO2 and MnO contents, most apatite grains are plotted in the magmatic apatite field in the SiO2 vs. MnO diagram (Chen and Zhang, 2018; Figure 5A). The negative correlation of MnO and CaO indicates the equivalent substitution of Ca2+ by Mn2+ (Pan and Fleet, 2002; Figure 5B). Ca and P show a negative correlation with Si, S, Na, La, and Ce (Figure 5C), which indicates the following substitutions: Na++S6+=Ca2++P5+, REE3++Si4+=Ca2++P5+, REE3++Na+=2Ca2+, and S6++Si4+=2 P5+ (Sha and Chappell, 1999; Mao et al., 2016). All apatite grains have higher F contents than Cl, indicating that they are fluorapatite. The negative correlation between Cl and F supports the reciprocal substitution of Cl− and F− (Pan and Fleet, 2002; Figure 5D). Apatite grains from the granodiorite porphyry have higher Cl contents (average of 0.69 wt%, n=7) and lower F contents (average of 2.15 wt%, n = 7) compared with those from quartz porphyry, i.e., Cl (average of 0.21 wt%, n = 6) and F (average of 4.08 wt%, n = 6), but are comparable to those from monzonite (average Cl content of 0.68 wt%, n = 14, and average F content of 2.96 wt%, n = 14). In addition, apatite in the granodiorite porphyry has higher SO3 concentrations (average of 0.19 wt%, n = 7) compared with those from the quartz porphyry (average of 0.02 wt%, n = 6) and monzonite (average of 0.03 wt%, n = 14).
FIGURE 5. (A) Apatite SiO2 vs. MnO plot; the fields of magmatic and hydrothermal apatite are from (Chen and Zhang, 2018); (B) Apatite MnO vs. CaO plot; (C) Apatite P+Ca vs. Si+Na+S+La+Ce plot (calculated according to Ketcham, 2015); and (D) Apatite Cl vs. F plot.
Chondrite-normalized rare earth element (REE) patterns of apatite grains from the three intrusive phases all show enrichment of light REE and depletion of heavy REE, with weak positive Ce anomalies and variably negative Eu anomalies (Figure 6). The total REE contents of apatite from granodiorite porphyry (3929–7428 ppm, average of 4926 ppm) are lower than those from quartz porphyry (9532–13260 ppm, average of 11073 ppm) and monzonite (3032–10199 ppm, average of 6546 ppm). Eu/Eu* of apatite from granodiorite porphyry (average of 0.53) is higher than that from quartz porphyry (average of 0.01) and monzonite (average of 0.14; Figure 7). Meanwhile, the apatite grains from granodiorite porphyry have Sr contents of 328.83–734.07 ppm (average of 476.07 ppm) and Y contents of 258.85–446.30 ppm (average of 345.47 ppm), with a Sr/Y ratio of 1.09–1.64. Comparatively, the apatite grains from quartz porphyry and monzonite have lower Sr contents (93.97–131.06, and 217.45–417.40 ppm, respectively), higher Y contents (4858.77–7200.35, and 342.67–705.78 ppm, respectively), and a lower Sr/Y ratio (0.01–0.03, 0.36–1.12, respectively; Figures 7, 8).
FIGURE 6. (A–C) Chondrite-normalized REE patterns of apatite from quartz porphyry, monzonite, and granodiorite porphyry of the Kalmakyr deposit. Chondrite normalization values are from Sun and McDonough (1989).
FIGURE 7. Plot of Sr vs. Eu/Eu* in the apatite of the Kalmakyr deposit. There is no positive correlation between the Sr and Eu/Eu* of apatite from the same intrusion.
FIGURE 8. Plots of Ce/Ce* vs. Eu/Eu* (A) and Sr/Y vs. La/Yb (B) in the apatite of the Kalmakyr deposit and other porphyry deposits of the world. Compiled data are from Pan et al. (2016), Qu et al. (2019), and Qu et al. (2021).
Magmatic apatite is generally formed in the early stages of the evolving magma, while hydrothermal apatite appears to be formed during the magmatic–hydrothermal transition or later hydrothermal stages (Bouzari et al., 2016). However, only those magmatic apatite crystals without alteration overprinting can provide original information on the parental magma (Xing et al., 2020; Pan et al., 2021; Xing et al., 2021). Most of the analyzed apatite grains from the three intrusive phases are hosted by biotite, hornblende, and plagioclase phenocrysts (Figure 4). BSE images show that the majority of apatite grains are homogeneous, and only some apatite grains from monzonite contain mineral or fluid inclusions (Figure 4E). These grains are ignored during our in situ geochemical analyses. In this study, almost all apatite grains are plotted in the magmatic apatite field in the SiO2 vs. MnO diagram (Chen and Zhang, 2018; Figure 5A). Moreover, the Cl contents of all analyzed apatite grains (<1.64 wt%) are significantly lower than those of hydrothermal apatite (usually exceeding 3.0 wt%; Palma et al., 2019). The apatite grains for a single intrusive phase show parallel REE patterns (Figure 6). It is, therefore, indicated that the analyzed apatite crystals from all three intrusive phases are magmatic apatite and can document the initial information on their parental magma. Although these apatite grains are magmatic, there are subtle differences in the apatite of the three intrusions. In petrography, apatite crystals from granodiorite porphyry are more fine-grained and homogeneous on the surface compared to those from quartz porphyry and monzonite (Figure 4).
Oxidized magma has a higher ability to convey metals and sulfur from the mantle to the shallow crust because sulfur in oxidized magma mainly exists as sulfate (SO42-) that is incompatible with chalcophile elements (Jugo, 2009; Richards, 2011; Zhong et al., 2018b; Zhong et al., 2019; Zhong et al., 2021c). The apatite Eu/Eu* value is an important indicator of magmatic oxygen fugacity since Eu3+ has a similar ionic radius with Ca2+ and is easier to substitute Ca2+ in the apatite lattice than Eu2+, which will result in high apatite Eu/Eu* values in the more oxidized magma (Sha and Chappell, 1999; Piccoli and Candela, 2002). At the Kalmakyr deposit, all the analyzed apatite grains show negative Eu/Eu* anomalies (Figure 8A). However, it should be noted that the Eu/Eu* values of apatite from the granodiorite porphyry (0.50–0.58, average of 0.53) are higher than those from the quartz porphyry (0.01–0.02, average of 0.01) and monzonite (0.20–0.11, average of 0.14) but are identical to ore-bearing intrusions from porphyry deposits elsewhere (Figure 8A). This indicates that the granodiorite magma may be more oxidized than the quartz porphyry and monzonitic magma. This inference is supported by the relatively high Ce4+/Ce3+ values (average 890) for zircon grains from the granodiorite porphyry (Zhao et al., 2017). Feldspar crystallization may also lead to negative Eu anomalies, accompanied by a significant reduction in Sr contents in the coexisting melt (Pan et al., 2021). There is no apparent positive correlation between apatite Eu/Eu* and Sr from a single intrusive phase, which excludes the previous conjecture (Figure 7). Therefore, the negative Eu anomalies are more likely to be caused by magma in an oxidized state. However, apatite grains from ore-bearing and ore-barren intrusions display similar Ce/Ce* (weakly positive; Figure 8A), indicating that apatite Ce/Ce* does not have a relationship with magmatic oxygen fugacity (Zhong et al., 2018a; Xing et al., 2021).
A high H2O content in oxidized magma is necessary for the exsolution of an aqueous volatile phase to release copper and gold (Richards, 2011; Lu et al., 2015; Zhong et al., 2021a). Magma with high H2O contents favors hornblende and garnet crystallization in early deep crust fractionation and would suppress plagioclase crystallization, resulting in elevated Sr/Y and La/Yb ratios (Richards, 2011; Nathwani et al., 2020). Many studies have shown that the characteristics of high Sr/Y and La/Yb in the hydrous magma can be recorded by the early crystallization of apatite (Nathwani et al., 2020; Xing et al., 2021). Petrographic observation indicates that apatite in all three intrusive phases occurs dominantly as an inclusion in phenocrysts such as biotite, plagioclase, and hornblende (Figure 4), suggesting that they may have been formed during the early stage of the evolving magma. The apatite in granodiorite porphyry shows relatively high Sr/Y ratios (1.09–1.64) and La/Yb ratios (36.19–53.42). This characteristic resembles ore-bearing intrusions from many other porphyry Cu-Au±(Mo) deposits (Pan et al., 2016; Qu et al., 2021) but it is obviously higher than those of quartz porphyry (Sr/Y = 0.02–0.03; La/Yb = 2.01–3.75) and other ore-barren intrusions (Qu et al., 2019; Figure 8B). This indicates that the H2O content of the granodiorite porphyry is higher than that of the ore-barren quartz porphyry. Although the apatite from the monzonite intrusion has roughly similar Sr/Y (0.36–1.12) and La/Dy (19.71–56.32) ratios to those from granodiorite porphyry (Figure 8B), the apatite from the monzonite intrusion shows relatively lower Sr/Y and La/Dy ratios. Moreover, zircon grains in granodiorite porphyry exhibit higher (10000 × Eu/Eu*)/Y ratios (5.33–24.49) and lower Dy/Yb ratios (0.12–0.21) than those from the monzonite intrusion, that is, (10000×Eu/Eu*)/Y = 1.53–7.23, and Dy/Yb = 0.19–0.44 (Zhao et al., 2017). It is, therefore, concluded that the magma of granodiorite porphyry has a higher H2O content than the magma of monzonite and quartz porphyry (Lu et al., 2016; Zhu et al., 2018).
Magmatic volatile compositions play a critical role in porphyry mineralization, largely due to the fact that they can form chemical ligands with metals (Cu and Au) that are favorable for migration and metal enrichment (Piccoli and Candela, 2002; Yardley, 2005; Jugo, 2009; Zhong et al., 2018a). According to many past studies, apatite wrapped in other igneous minerals as inclusions can preserve the initial volatile content of magma (Stock et al., 2016; Zhu et al., 2018; Li et al., 2021; Pan et al., 2021; Zhu et al., 2022). As we have previously discussed, most of the analyzed apatite grains fit this feature and could theoretically record the original volatile content of the magma. The Cl and F contents of parental magma for the three intrusive phases were calculated according to the apatite/melt partition coefficients proposed by Mathez and Webster (2005), whereas the S abundance in the parental magma was calculated according to the apatite/melt partition coefficients proposed by Parat et al. (2011). The results (Table 1) show that the Cl contents of granodioritic magma (average of 8602 ppm) are significantly higher than the magma of quartz porphyry (average of 2652 ppm) but are consistent with the monzonitic magma (average of 8483 ppm). We, therefore, infer that the Cl-rich hydrothermal fluids exsolved from the ore-bearing granodiorite porphyry may have altered the Cl content of apatite from ore-barren monzonite. The Cl-rich hydrothermal fluids not only affect the apatite Cl content but are also capable of carrying or extracting metals from the monzonite intrusion (Zajacz et al., 2012). For the F content, it is generally recognized that F is partitioned more difficultly into aqueous fluids relative to Cl (Stock et al., 2016; Li et al., 2021); thus, the F content of apatite is basically not altered by the late hydrothermal fluids. The F contents of granodioritic magma (average of 6329 ppm) are lower than those of the magmas for quartz porphyry (average of 12010 ppm) and monzonite (average of 8706 ppm), which indicate that F, unlike Cl, possibly has little influence on the extraction and transport of Cu and Au during the magmatic-hydrothermal evolution. Furthermore, the S contents of granodioritic magma (average of 111 ppm) are higher than those of magma for quartz porphyry (average of 9 ppm) and monzonite (average of 9 ppm). This result is also supported by the development of numerous anhydrite veins in the mineralized zones since anhydrite is an indicator of sulfur-rich and oxidized melts (Golovanov et al., 2005; Zhao et al., 2017; Hutchinson and Dilles, 2019; Li et al., 2021). To summarize, we conclude that the ore-bearing granodioritic magma is characterized by being Cl- and S-rich, and F-poor compared with the magma for the ore-barren quartz porphyry and monzonite.
TABLE 1. Estimated S, Cl, and F contents in the porphyry-associated magma of the Kalmakyr deposit using apatite geochemical compositions.
The porphyry deposit contains significant resources of Cu, Mo, and Au, forming different subgroups with diverse metal associations (e.g., Cu–Au, Cu–Mo, and Mo; Sillitoe, 2010). Magmatic volatile (F, Cl, and S) contents have been proposed to be an important controlling factor for their difference (Zajacz et al., 2012); however, which magmatic features are most critical remains unclear. In this study, we attempted to address this question by comparing the volatile contents of apatite from the ore-bearing granodiorite porphyry at Kalmakyr with available data from porphyry Cu–Au, Cu–Mo, and Mo deposits around the world. As shown in Figures 9A, B, the apatite SO3 and F contents for ore-bearing intrusions in porphyry Cu–Au, Cu–Mo, and Mo-only deposits are essentially similar, suggesting that these contents in magma may not be key factors controlling metal differences. In contrast, the Cl content and F/Cl ratio in apatite vary significantly (Figure 9C), i.e., gold-rich porphyry deposits contain the highest Cl content (0.4–2.0 wt%) with the lowest F/Cl ratio (0.8–8.0), Cu–Mo porphyry deposits have a moderate Cl content (0.1–0.6 wt%) and F/Cl ratio (5.0–30), and Mo-only porphyry deposits show the lowest Cl content (0.01–0.12 wt%) with the highest F/Cl ratio (25-360). This variation might be related to the higher solubility of Au relative to Cu and Mo in Cl-rich melts, resulting in elevated Au enrichment in evolving magma (Zajacz et al., 2012). It is, therefore, suggested that the Cl content in evolving magma might be one of the crucial factors controlling the diverse metal associations in porphyry deposits, and a high apatite Cl content (0.4–2.0 wt%) and low F/Cl ratio (0.8–8.0) in ore-bearing intrusions could be used as an important exploration indicator for gold-rich porphyry Cu deposits.
FIGURE 9. Plots of SO3 vs. Cl (A), F vs. Cl (B), and F/Cl vs. Cl (C) in apatite of the Kalmakyr deposit and other porphyry deposits of the world. Data for porphyry Cu-Au deposits are from Richards et al. (2017) and Zhu et al. (2018); data for porphyry Cu-Mo deposits are from Xu et al. (2021); and data for porphyry Mo deposits are from Xing et al. (2020) and Xing et al. (2021).
1) Apatite in multiphase intrusions occurs as inclusions within early phenocrysts, with a homogeneous surface and low Cl and moderate SiO2 contents, indicating a magmatic origin.
2) The ore-bearing granodiorite porphyry at Kalmakyr is characterized by high oxygen fugacity and high H2O, Cl, and S contents compared to the ore-barren intrusions.
3) A high Cl content (0.4–2.0 wt%) and low F/Cl ratio (0.8–8.0) in magmatic apatite can be used as an important indicator to prospect gold-rich porphyry Cu deposits.
The original contributions presented in the study are included in the article/Supplementary Material, further inquiries can be directed to the corresponding author.
YL: investigation, analysis of the data, methodology, and writing—original draft. XZ: investigation, conceptualization, sample collection, designing the experiment, supervision, and writing—review and editing. CX: investigation, supervision, and writing—review and editing. BN: investigation and writing—review and editing. JC: conducting the experiment.
The authors declare that this study received funding from Zijin Mining Group Co., Ltd. The funder was not involved in the study design, collection, analysis, interpretation of data, the writing of this article, or the decision to submit it for publication.
This study was sponsored by the National Natural Science Foundation of China (U1303292, 42202100), the Open Research projects from the State Key Laboratory of Geological Processes and Mineral Resources (GPMR202117) of Beijing, China, and the MNR Key Laboratory of Metallogeny and Mineral Assessment (ZS2204) of Beijing, China, and Zijin Mining Group Co., Ltd.
CX acknowledges funding under Department of Human Resources and Social Security of Xinjiang Uygur Autonomous Region Introduced Project “Tianchi talent”.
The authors are grateful to Fangyue Wang and Xiaohong Mao for their help during the analysis. This paper benefits from thoughtful reviews by reviewers and editorial handling by Shihua Zhong.
The authors declare that the research was conducted in the absence of any commercial or financial relationships that could be construed as a potential conflict of interest.
All claims expressed in this article are solely those of the authors and do not necessarily represent those of their affiliated organizations, or those of the publisher, the editors, and the reviewers. Any product that may be evaluated in this article, or claim that may be made by its manufacturer, is not guaranteed or endorsed by the publisher.
The Supplementary Material for this article can be found online at: https://www.frontiersin.org/articles/10.3389/feart.2023.1162994/full#supplementary-material
Supplementary Table S1 | Apatite major and trace element compositions with calculated apatite ΣREE, Eu/Eu*, Ce/Ce*, and atoms per formula unit (apfu) values of the Kalmakyr porphyry Cu–Au deposit, Uzbekistan.
Supplementary Table S2 | Compiled apatite Eu/Eu*, Ce/Ce*, La/Yb, and Sr/Y ratios for ore-bearing and ore-barren intrusions of porphyry deposits.
Supplementary Table S3 | Compiled apatite SO3, Cl, F, and F/Cl ratios of porphyry deposits.
Biske, Y. S., and Seltmann, R. (2010). Paleozoic tian-Shan as a transitional region between the rheic and urals-turkestan oceans. Gondwana Res. 17 (2-3), 602–613. doi:10.1016/j.gr.2009.11.014
Bouzari, F., Hart, C. J., Bissig, T., and Barker, S. (2016). Hydrothermal alteration revealed by apatite luminescence and chemistry: A potential indicator mineral for exploring covered porphyry copper deposits. Econ. Geol. 111 (6), 1397–1410. doi:10.2113/econgeo.111.6.1397
Chelle-Michou, C., and Chiaradia, M. (2017). Amphibole and apatite insights into the evolution and mass balance of Cl and S in magmas associated with porphyry copper deposits. Contributions Mineralogy Petrology 172 (11-12), 105. doi:10.1007/s00410-017-1417-2
Chen, L., and Zhang, Y. (2018). In situ major-trace-elements and Sr-Nd isotopic compositions of apatite from the Luming porphyry Mo deposit, NE China: Constraints on the petrogenetic-metallogenic features. Ore Geol. Rev. 94, 93–103. doi:10.1016/j.oregeorev.2018.01.026
Chiaradia, M. (2014). Copper enrichment in arc magmas controlled by overriding plate thickness. Nat. Geosci. 7 (1), 43–46. doi:10.1038/ngeo2028
Chiaradia, M., Schaltegger, U., and Spikings, R. (2014). Time scales of mineral systems—Advances in understanding over the past decade. Soc. Econ. Geol. -Special Publ. 18, 37–58. doi:10.5382/SP.18.03
Chiaradia, M., Schaltegger, U., Spikings, R., Wotzlaw, J. F., and Ovtcharova, M. (2013). How accurately can we date the duration of magmatic-hydrothermal events in porphyry systems? —an invited paper. Econ. Geol. 108 (4), 565–584. doi:10.2113/econgeo.108.4.565
Cooke, D. R., Hollings, P., and Walshe, J. L. (2005). Giant porphyry deposits: Characteristics, distribution, and tectonic controls. Econ. Geol. 100 (5), 801–818. doi:10.2113/gsecongeo.100.5.801
Golovanov, I. M., Seltmann, R., Kremenetsky, A. A., and Porter, T. (2005). “The almalyk (Kalmakyr–Dalnee) and saukbulak Cu–Au porphyra systems, Uzbekistan,” in Super porphyry copper and gold deposits: A global perspective, 2. Editor T. M. Porter (Adelaide: PGC Publishing), The porphyry Cu–Au/Mo deposits of Central Euroasia, 2, 513–523.
Hutchinson, M. C., and Dilles, J. H. (2019). Evidence for magmatic anhydrite in porphyry copper intrusions. Econ. Geol. 114 (1), 143–152. doi:10.5382/econgeo.2019.4624
Jugo, P. J. (2009). Sulfur content at sulfide saturation in oxidized magmas, Geology 37(5), 415–418. doi:10.1130/g25527a.1
Ketcham, R. A. (2015). Technical Note: Calculation of stoichiometry from EMP data for apatite and other phases with mixing on monovalent anion sites. Am. Mineralogist 100 (7), 1620–1623. doi:10.2138/am-2015-5171
Li, J. X., Li, G. M., Evans, N. J., Zhao, J. X., Qin, K. Z., and Xie, J. (2021). Primary fluid exsolution in porphyry copper systems: Evidence from magmatic apatite and anhydrite inclusions in zircon. Miner. Deposita 56, 407–415. doi:10.1007/s00126-020-01013-4
Li, W. C., Zhang, X. F., Yu, H. J., Tao, D., and Liu, X. L. (2022). Geology and mineralization of the pulang supergiant porphyry copper deposit (5.11 Mt) in shangri-la, yunnan province, China: A review. China Geol. 5 (4), 662–695.
Lipson, R. (2014). The promise and perils of porphyry deposits in the future of gold production. Seg. Discov. 98, 1–21. doi:10.5382/segnews.2014-98.fea
Liu, Y., Hu, Z., Gao, S., Günther, D., Xu, J., Gao, C., et al. (2008). In situ analysis of major and trace elements of anhydrous minerals by LA-ICP-MS without applying an internal standard. Chem. Geol. 257 (1-2), 34–43. doi:10.1016/j.chemgeo.2008.08.004
Lu, Y. J., Loucks, R. R., Fiorentini, M. L., Yang, Z. M., and Hou, Z. Q. (2015). Fluid flux melting generated postcollisional high Sr/Y copper ore–forming water-rich magmas in Tibet.Geology, , 43(7), 583–586. doi:10.1130/g36734.1
Lu, Y. J., Loucks, R. R., Fiorentini, M., McCuaig, T. C., Evans, N. J., Yang, Z. M., et al. (2016). Zircon compositions as a pathfinder for porphyry Cu±Mo±Au deposits. Soc. Econ. Geol. -Special Publ. 19, 1–57. doi:10.5382/SP.19.13
Mao, M., Rukhlov, A. S., Rowins, S. M., Spence, J., and Coogan, L. A. (2016). Apatite trace element compositions: A robust new tool for mineral exploration. Econ. Geol. 111 (5), 1187–1222. doi:10.2113/econgeo.111.5.1187
Mathez, E. A., and Webster, J. D. (2005). Partitioning behavior of chlorine and fluorine in the system apatite-silicate melt-fluid. Geochimica Cosmochimica Acta 69 (5), 1275–1286. doi:10.1016/j.gca.2004.08.035
Nathwani, C. L., Loader, M. A., Wilkinson, J. J., Buret, Y., Sievwright, R. H., and Hollings, P. (2020). Multi-stage arc magma evolution recorded by apatite in volcanic rocks.Geology,. Geology 48 (4), 323–327. doi:10.1130/g46998.1
Palma, G., Barra, F., Reich, M., Valencia, V., Simon, A. C., Vervoort, J., et al. (2019). Halogens, trace element concentrations, and Sr-Nd isotopes in apatite from iron oxide-apatite (IOA) deposits in the Chilean iron belt: Evidence for magmatic and hydrothermal stages of mineralization. Geochimica Cosmochimica Acta 246, 515–540. doi:10.1016/j.gca.2018.12.019
Pan, L. C., Hu, R. Z., Oyebamiji, A., Wu, H. Y., Li, J. W., and Li, J. X. (2021). Contrasting magma compositions between Cu and Au mineralized granodiorite intrusions in the Tongling ore district in South China using apatite chemical composition and Sr-Nd isotopes. Am. Mineralogist J. Earth Planet. Mater. 106 (12), 1873–1889. doi:10.2138/am-2021-7497
Pan, L. C., Hu, R. Z., Wang, X. S., Bi, X. W., Zhu, J. J., and Li, C. (2016). Apatite trace element and halogen compositions as petrogenetic-metallogenic indicators: Examples from four granite plutons in the Sanjiang region, SW China.Lithos. Lithos 254, 118–130. doi:10.1016/j.lithos.2016.03.010
Pan, Y., and Fleet, M. E. (2002). Compositions of the apatite-group minerals: Substitution mechanisms and controlling factors. Rev. mineralogy Geochem. 48 (1), 13–49. doi:10.2138/rmg.2002.48.2
Parat, F., Holtz, F., and Klügel, A. (2011). S-Rich apatite-hosted glass inclusions in xenoliths from La Palma: Constraints on the volatile partitioning in evolved alkaline magmas. Contributions Mineralogy Petrology 162, 463–478. doi:10.1007/s00410-011-0606-7
Piccoli, P. M., and Candela, P. A. (2002). Apatite in igneous systems. Rev. Mineralogy Geochem. 48 (1), 255–292. doi:10.2138/rmg.2002.48.6
Qin, J., Huang, F., Zhong, S., Wang, D., and Seltmann, R. (2022). Unraveling evolution histories of large hydrothermal systems via garnet U-Pb dating, sulfide trace element and isotopic analyses: A case study of shuikoushan polymetallic ore field, south China. South China. Ore Geol. Rev. 149, 105063. doi:10.1016/j.oregeorev.2022.105063
Qu, P., Li, N. B., Niu, H. C., Shan, Q., Weng, Q., and Zhao, X. C. (2021). Difference in the nature of ore-forming magma between the Mesozoic porphyry Cu-Mo and Mo deposits in NE China: Records from apatite and zircon geochemistry. Ore Geol. Rev. 135, 104218. doi:10.1016/j.oregeorev.2021.104218
Qu, P., Li, N. B., Niu, H. C., Yang, W. B., Shan, Q., and Zhang, Z. Y. (2019). Zircon and apatite as tools to monitor the evolution of fractionated I-type granites from the central Great Xing’an Range, NE China.Lithos. Lithos 348, 105207. doi:10.1016/j.lithos.2019.105207
Richards, J. P. (2011). High Sr/Y arc magmas and porphyry Cu±Mo±Au deposits: Just add water. Econ. Geol. 106 (7), 1075–1081. doi:10.2113/econgeo.106.7.1075
Richards, J. P., López, G. P., Zhu, J. J., Creaser, R. A., Locock, A. J., and Mumin, A. H. (2017). Contrasting tectonic settings and sulfur contents of magmas associated with Cretaceous porphyry Cu±Mo±Au and intrusion-related iron oxide Cu-Au deposits in northern Chile. Econ. Geol. 112 (2), 295–318. doi:10.2113/econgeo.112.2.295
Seltmann, R., Konopelko, D., Biske, G., Divaev, F., and Sergeev, S. (2011). Hercynian post-collisional magmatism in the context of Paleozoic magmatic evolution of the Tien Shan orogenic belt. J. Asian Earth Sci. 42 (5), 821–838. doi:10.1016/j.jseaes.2010.08.016
Seltmann, R., Porter, T. M., and Pirajno, F. (2014). Geodynamics and metallogeny of the central eurasian porphyry and related epithermal mineral systems: A review. J. Asian Earth Sci. 79, 810–841. doi:10.1016/j.jseaes.2013.03.030
Sha, L. K., and Chappell, B. W. (1999). Apatite chemical composition, determined by electron microprobe and laser-ablation inductively coupled plasma mass spectrometry, as a probe into granite petrogenesis. Geochimica Cosmochimica Acta 63 (22), 3861–3881. doi:10.1016/s0016-7037(99)00210-0
Sillitoe, R. H. (2010). Porphyry copper systems. Econ. Geol. 105 (1), 3–41. doi:10.2113/gsecongeo.105.1.3
Stock, M. J., Humphreys, M. C., Smith, V. C., Isaia, R., and Pyle, D. M. (2016). Late-stage volatile saturation as a potential trigger for explosive volcanic eruptions. Nat. Geosci. 9 (3), 249–254. doi:10.1038/ngeo2639
Sun, S. S., and McDonough, W. F. (1989). Chemical and isotopic systematics of oceanic basalts: Implications for mantle composition and processes. Geol. Soc. Lond. Spec. Publ. 42 (1), 313–345. doi:10.1144/gsl.sp.1989.042.01.19
Tang, H., Deng, Z., Zhong, S., Meng, G., Wang, Z., Yuan, L., et al. (2023). Geochemistry and Sr–Nd–Hf–O isotopes of ore-bearing plutons from the Yundukala Au–Cu–Co deposit, East Junggar, Xinjiang, Northwest China: Implications for petrogenesis and tectonic setting. Ore Geol. Rev. 153, 105274. doi:10.1016/j.oregeorev.2022.105274
Turamuratov, I. B., Isokov, M. U., Hodjaev, N. T., Abduazimova, Z. M., Zimalina, V. Y., Tsoy, V. D., et al. (2011). Atlas of ore deposits models of Uzbekistan. State Comm. Repub. Uzbekistan Geol. Mineral Resour. Sci. Res. Inst. Mineral Resour. Tashkent, 1–100.
Xing, K., Shu, Q., and Lentz, D. R. (2021). Constraints on the formation of the giant Daheishan porphyry Mo deposit (NE China) from whole-rock and accessory mineral geochemistry. J. Petrology 62 (4), egab018. doi:10.1093/petrology/egab018
Xing, K., Shu, Q., Lentz, D. R., and Wang, F. (2020). Zircon and apatite geochemical constraints on the formation of the Huojihe porphyry Mo deposit in the Lesser Xing’an Range, NE China. Am. Mineralogist J. Earth Planet. Mater. 105 (3), 382–396. doi:10.2138/am-2020-7226
Xu, B., Hou, Z. Q., Griffin, W. L., Lu, Y., Belousova, E., Xu, J. F., et al. (2021). Recycled volatiles determine fertility of porphyry deposits in collisional settings. Am. Mineralogist J. Earth Planet. Mater. 106 (4), 656–661. doi:10.2138/am-2021-7714
Yakubchuk, A., Cole, A., Seltmann, R., and Shatov, V. (2002). Tectonic setting, characteristics, and regional exploration criteria for gold mineralization in the altaid orogenic collage: The tien Shan province as a key example. Soc. Econ. Geol. -Special Publ. 9, 177–202. doi:10.5382/SP.09.09
Yang, H. H., Wang, Q., Li, Y. B., Lin, B., Song, Y., Wang, Y. Y., et al. (2022). Geology and mineralization of the tiegelongnan supergiant porphyry-epithermal Cu (Au, Ag) deposit (10 Mt) in Western tibet, China: A review. China Geol. 5 (1), 136–159. doi:10.1016/S2096-5192(22)00091-X
Yardley, B. W. D. (2005). 100th anniversary special paper: Metal concentrations in crustal fluids and their relationship to ore formation. Econ. Geol. 100, 613–632. doi:10.2113/gsecongeo.100.4.613
Zajacz, Z., Candela, P. A., Piccoli, P. M., Wälle, M., and Sanchez-Valle, C. (2012). Gold and copper in volatile saturated mafic to intermediate magmas: Solubilities, partitioning, and implications for ore deposit formation. Geochimica Cosmochimica Acta 91, 140–159. doi:10.1016/j.gca.2012.05.033
Zhao, X. B., Xue, C. J., Chi, G. X., Mo, X. X., Nurtaev, B., and Zhang, G. Z. (2017). Zircon and molybdenite geochronology and geochemistry of the Kalmakyr porphyry Cu–Au deposit, Almalyk district, Uzbekistan: Implications for mineralization processes.Ore Geology Reviews. Ore Geol. Rev. 86, 807–824. doi:10.1016/j.oregeorev.2017.04.008
Zhao, X. B., Xue, C. J., Chi, G. X., Pak, N., and Zu, B. (2015). Re–Os pyrite and U–Pb zircon geochronology from the Taldybulak Levoberezhny gold deposit: Insight for Cambrian metallogeny of the Kyrgyz northern Tien Shan. Ore Geol. Rev. 67, 78–89. doi:10.1016/j.oregeorev.2014.12.002
Zhao, X. B., Xue, C. J., Zu, B., Seltmann, R., Chi, G. X., Dolgopolova, A., et al. (2022). Geology and genesis of the unkurtash intrusion-related gold deposit, tien Shan, Kyrgyzstan. Econ. Geol. 117, 1073–1103. doi:10.5382/econgeo.4918
Zhong, S., Feng, C., Seltmann, R., Li, D., and Dai, Z. (2018a). Geochemical contrasts between late triassic ore-bearing and barren intrusions in the weibao Cu–Pb–Zn deposit, east kunlun mountains, NW China: Constraints from accessory minerals (zircon and apatite).Mineralium deposita. Min. Depos. 53, 855–870. doi:10.1007/s00126-017-0787-8
Zhong, S., Feng, C., Seltmann, R., Li, D., and Qu, H. (2018b). Can magmatic zircon be distinguished from hydrothermal zircon by trace element composition? The effect of mineral inclusions on zircon trace element composition. Lithos 314-315, 646–657. doi:10.1016/j.lithos.2018.06.029
Zhong, S., Li, S., Feng, C., Gao, Y., Qu, H., Seltmann, R., et al. (2021a). Geochronology and geochemistry of mineralized and barren intrusive rocks in the yemaquan polymetallic skarn deposit, northern qinghai-tibet plateau: A zircon perspective. Ore Geol. Rev. 139, 104560. doi:10.1016/j.oregeorev.2021.104560
Zhong, S., Li, S., Feng, C., Liu, Y., Santosh, M., He, S., et al. (2021b). Porphyry copper and skarn fertility of the northern Qinghai-Tibet Plateau collisional granitoids. Earth-Science Rev. 214, 103524. doi:10.1016/j.earscirev.2021.103524
Zhong, S., Li, S., Seltmann, R., Lai, Z., and Zhou, J. (2021c). The influence of fractionation of REE-enriched minerals on the zircon partition coefficients. Geosci. Front. 12, 101094. doi:10.1016/j.gsf.2020.10.002
Zhong, S., Seltmann, R., Qu, H., and Song, Y. (2019). Characterization of the zircon Ce anomaly for estimation of oxidation state of magmas: A revised Ce/Ce* method. Mineralogy Petrology 113, 755–763. doi:10.1007/s00710-019-00682-y
Zhu, J. J., Hu, R., Bi, X. W., Hollings, P., Zhong, H., Gao, J. F., et al. (2022). Porphyry Cu fertility of eastern Paleo-Tethyan arc magmas: Evidence from zircon and apatite compositions. Lithos 424, 106775. doi:10.1016/j.lithos.2022.106775
Zhu, J. J., Richards, J. P., Rees, C., Creaser, R., DuFrane, S. A., Locock, A., et al. (2018). Elevated magmatic sulfur and chlorine contents in ore-forming magmas at the Red Chris porphyry Cu-Au deposit, northern British Columbia, Canada. Econ. Geol. 113 (5), 1047–1075. doi:10.5382/econgeo.2018.4581
Keywords: apatite geochemistry, oxygen fugacity, magmatic volatile contents, Kalmakyr porphyry Cu-Au deposit, Tien Shan
Citation: Liu Y, Zhao X, Xue C, Nurtaev B and Chen J (2023) Contrasting apatite geochemistry between ore-bearing and ore-barren intrusions of the giant Kalmakyr gold-rich porphyry Cu deposit, Tien Shan, Uzbekistan. Front. Earth Sci. 11:1162994. doi: 10.3389/feart.2023.1162994
Received: 10 February 2023; Accepted: 21 February 2023;
Published: 09 March 2023.
Edited by:
Shihua Zhong, Ocean University of China, ChinaReviewed by:
Pf Zuo, Henan Polytechnic University, ChinaCopyright © 2023 Liu, Zhao, Xue, Nurtaev and Chen. This is an open-access article distributed under the terms of the Creative Commons Attribution License (CC BY). The use, distribution or reproduction in other forums is permitted, provided the original author(s) and the copyright owner(s) are credited and that the original publication in this journal is cited, in accordance with accepted academic practice. No use, distribution or reproduction is permitted which does not comply with these terms.
*Correspondence: Xiaobo Zhao, eGlhb2Jvemhhb0BjdWdiLmVkdS5jbg==
Disclaimer: All claims expressed in this article are solely those of the authors and do not necessarily represent those of their affiliated organizations, or those of the publisher, the editors and the reviewers. Any product that may be evaluated in this article or claim that may be made by its manufacturer is not guaranteed or endorsed by the publisher.
Research integrity at Frontiers
Learn more about the work of our research integrity team to safeguard the quality of each article we publish.