- Research Institute of Petroleum Exploration and Development, PetroChina, Beijing, China
Understanding the structural style, kinematic process, and timing of superimposed structures worldwide is often difficult due to complex structure deformation process. Fortunately, the newly acquired high-quality seismic reflection data and geological observations covering the Tongnanba anticline provide an excellent chance to characterize such structures. Here, we used geological and seismic data from the Tongnanba region to evaluate the structural style and deformation sequence of Tongnanba anticline. In this regard, we propose a new model of the northeastern Sichuan Basin, which are different from the model that deep structures formed earlier than shallow structures demonstrated by previous studies, and we also discussed the implications of this new model for the deep oil and gas exploration. Compressed by Micangshan and Dabashan thrust belts and controlled by three detachment layers, the Tongnanba anticline shows a complex multi-stage, multi-directional, and multi-level superimposed structure. There were three deformation layers vertically, leading to the multi-level detachment thrust structure style. Specifically, the upper and middle deformation layers were mainly controlled by South Dabashan thrust belt in the early stage, forming long-distance detachment thrust structure extended in the NW-SE direction. A series of pop-up structures propagated toward the upper and middle detachment layers. On the other hand, the lower deformation layer was primarily controlled by the Micangshan thrust belt in the late stage, forming complex basement faults extended in the NE-SW direction, which was consistent with Trishear fault-propagation fold. Along the basement detachment developed multiple branch slopes spread from northeast to southwest. The middle and upper deformation layers was transformed by the basement faults, thus forming the complex superimposed structure of north-south zonation and east-west segmentation at present. It was such complex superimposed structure that control the process of hydrocarbon accumulation and adjustment in each deformation layer, and the deep-ultra-deep ancient oil and gas reservoirs may be worth of exploring.
Introduction
There are large number of superimposed structures develop orogenic belts and continental crustal deformation areas. Multiple phases compression causes the deformation formed in the early stage being modified by the deformation formed lately (Kusky and Loring, 2001; Saha, 2002; Dario et al., 2010; Shah et al., 2013; Deng et al., 2015; Zhang et al., 2019; Hou et al., 2020). For instance, in the core zones of the Alpi Apuane metamorphic complex in the northwest Tuscany Italy, the switch from the crustal scale contractional regime to the extensional one has been documented (Molli et al., 2022). Two phases of deformation of Paleozoic accretion of the orogenic belt are reported based on the analysis of folds on the northern margin of the North China Craton (Hou et al., 2020). Zhang (2019) report that NE-near EW trending fold superimposed NW-NNE trending fold in the Jianchi area of western Dabashan according to field investigation and identification.
However, the study of these superimposed structures pay attention to outcrop- and regional-scale, which ascertain the fold interference patterns which are influenced by two phases of orthogonal or oblique deformation. Therefore, a serious of interference patterns are proposed for the single-layer superimposed folds (Ramsay, 1962, 1967; Ghosh et al., 1992; Grasemann, 2004). Otherwise, a serious of numerical simulation and physical simulation experiments are fully used in the study of superimposed structures (Deng et al., 2016; Long et al., 2021; Ma et al., 2023).
Unlike most superimposed structures investigated currently, there are always multi-level folds superimposed vertically in front of the foreland thrust belt. And been subjected to Mesozoic-Cenozoic multi-phase, multi-directional compression, the superimposed structures are different with the conventional one. This makes us not only understand the superimposed structure in single-layer or in planar, but also comprehend it vertically.
This article chooses Tongnanba anticline as the research object to discuss the structural styles and evolution process of multi-phase, multi-directional and multi-level superimposed structure. The Tongnanba anticline is intersection zone in the fronts of the Dabashan and Micangshan fold thrust belts. Decades have been spent studying the structural style, evolutionary processes (Wu et al., 2006; Hu et al., 2012; Shi et al., 2012; Dong et al., 2013; Wen et al., 2013; Chen, 2015, Chen et al., 2016; Li et al., 2015) and oil and gas exploration (Jin et al., 2012; Li et al., 2016; Jiang et al., 2020) of the Tongnanba anticline and peripheral thrust belt. It is commonly believed that the Tongnanba anticline was compressed by Micangshan in the early stage to form the NE-SW striking deep thrust fold deformation and experienced the Dabashan over-thrusting to form the NW-SE striking compressive thrust deformation in the middle and upper parts in the late stage (Dong et al., 2006; Wu et al., 2006; Jiang et al., 2008; Li et al., 2008; Xu et al., 2009; Zhang et al., 2010; Zhang et al., 2011; Li et al., 2013; Wang et al., 2013; Chen et al., 2015; Chen et al., 2016). And a multi-detachment deformation system is formed under the control of multiple sets of detachment layers, which incorporate a classic incorporate a classic decoupled deformation model. But the regional seismic profile indicates that different tectonic deformation layers exhibit local coupling, which contradicts the notion that early deformation occurs in the lower deformation layer and late in the upper and middle deformation layers. Therefore, it is necessary to redefine the structure style and deformation sequence.
In this study, the structural style was discussed by a serious of region seismic profiles in different directions. Based on seismic profile interpretation results, a new tectonic evolution process of the study area is restored. Finally, we discussed the adjustment and modification of hydrocarbon by the new tectonic evolution process.
Geologic setting
The Tongnanba anticline, located in northeastern part of the Sichuan Basin, is a major part of Micangshan-Hannan Uplift and the Southern Dabashan arc thrust belt, with a general trend of NE to NNE (Figure 1). It experienced an orogenic collision between the Yangtze Plate and the North China Plate and uplift of the eastern margin of the Qinghai-Tibet Plateau since the late Triassic, forming a series of underground structures with northeast and northwest trends (He et al., 2011; Jia et al., 2013). From Cretaceous-Cenozoic, exhumation formed the relief at present. It composed two stratigraphic units on a Precambrian crystalline base: 1) Marine sedimentary sequences from the Sinian to Middle Triassic formed in the Marine Craton rift basin to the craton depression basin; and 2) terrestrial sedimentary sequences from the Late Triassic to the Neogene developed in the continental depression basin to foreland basin (He et al., 2011; Huang et al., 2021). The absence of an angular unconformity and the development of multiple parallel unconformities in the region are notable. Due to intense denudation, the strata exposed on the surface are mainly Jurassic and Lower Cretaceous (Figure 2).
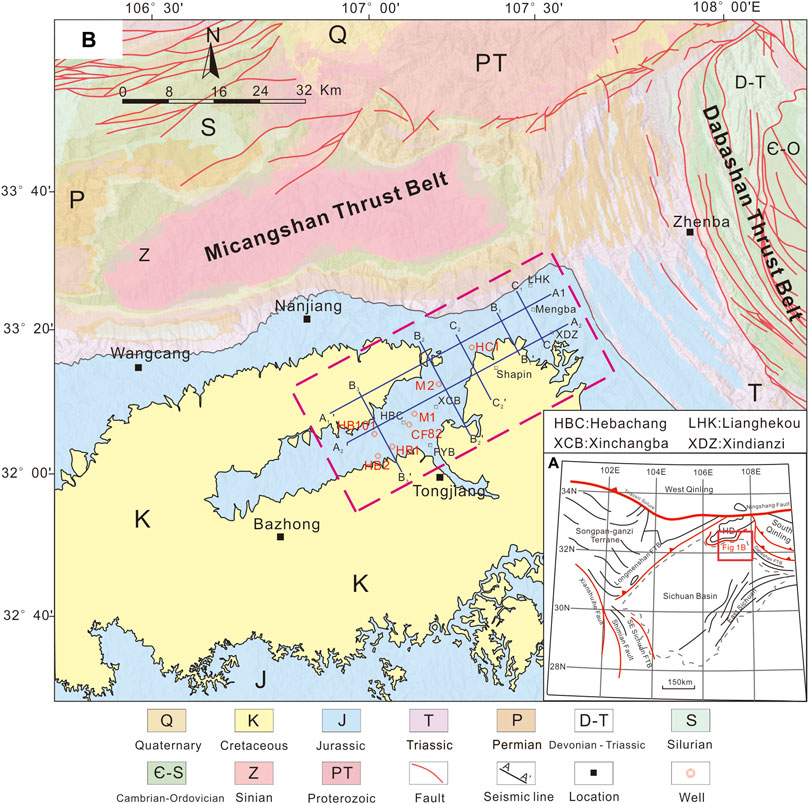
FIGURE 1. (A) A simplified structure map of the North China plate, the South China plate, the Qinling Mountains orogenic belt and the location of the study area (modified from Chen et al., 2016). HD, Hannan uplift; SD, Shennongjia uplift. (B) A simplified geologic map of Tongnanba anticline and its adjacent thrust belts.
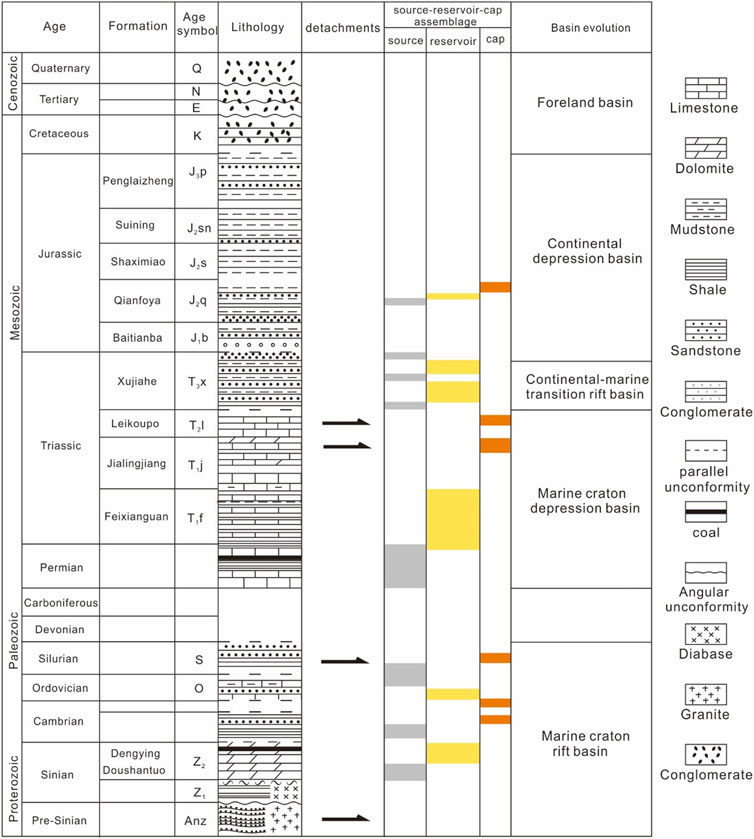
FIGURE 2. A simplified stratigraphic column of the Tongnanba anticline, indicating multistage tectonic activities (modified from Chen et al., 2016).
The Micangshan and Southern Dabashan FTB
The Micangshan FTB is connected and intersected with several structural belts, including the Sichuan Basin, the Songpan-Ganzi orogenic belt, the Longmenshan FTB and the south Dabashan FTB. It comprises Mesoproterozoic metamorphic rocks, with Paleozoic and Mesozoic sedimentary rocks surrounding it. Micangshan FTB underwent multiple phases of tectonic movement, forming a complex structural system (Wu et al., 2011; Sun et al., 2011). Two rapid uplift events occurred in Micangshan uplift, respectively during the Early Jurassic-Early Cretaceous and Late Miocene to present, which are related to the collision of the Yangzian-North China and Indo-Eurasian plate (Li et al., 2010; Zhang et al., 2022).
Located at the northern margin of the Yangtze Craton, the Southern Dabashan FTB is a curved tectonic belt in the southern Qinling orogen formed by the Late Triassic collision of the Qinling microplate and the Yangtze plate. Due to the Late Triassic collision and structural superposition during the Late Jurassic to Early Cretaceous, it experienced foreland folding and thrusting (Dong et al., 2006; Zhang et al., 2011). The Southern Dabashan FTB is mainly characterized by the multi-detachment structures. Governed by the Lower Triassic Jialingjiang Formation and Cambrian detachment layers, the multi-level and multi-stage detachment deformation are formed (Li et al., 2017; Huang et al., 2021).
Detachment layers in the Tongnanba anticline
Gypsum rock, mud, and shale demonstrate the characteristics of plastic flow upon subjection to compression deformation, which is easily transformed into a detachment layer. Other rock strata, such as limestone and dolomite, are primarily distinguished by their brittle fracture. Multiple detachment layers are recognized in the study area, including the pre-Sinian basement detachment layer, the Silurian mudstone detachment layer and the Triassic Jialingjiang-Leikoupo Formation gypsum detachment layer (Jin et al., 2006; Zheng et al., 2013). The gypsum detachment layer in the Leikoupo Formation has a chaotic seismic event feature, thickens locally under strong extrusion, according to the high-quality seismic profile (Figure 3). It is buried between 4,500 and 5,500 m deep. The Silurian detachment layer shows weak and discontinuous seismic reflection, with no obvious thickness change. Its depth is 6,000–6,500 m. The depth of the pre-Sinian basement detachment layer is currently controversial. Local detachment layers are not discussed in detail here. According to the analysis of the detachment layer in the study area presented above, three structural deformation layers (upper, middle, and lower) are formed in the upper portion of the pre-Sinian basement detachment layer, exhibiting inconsistent deformation in different deformation layers.
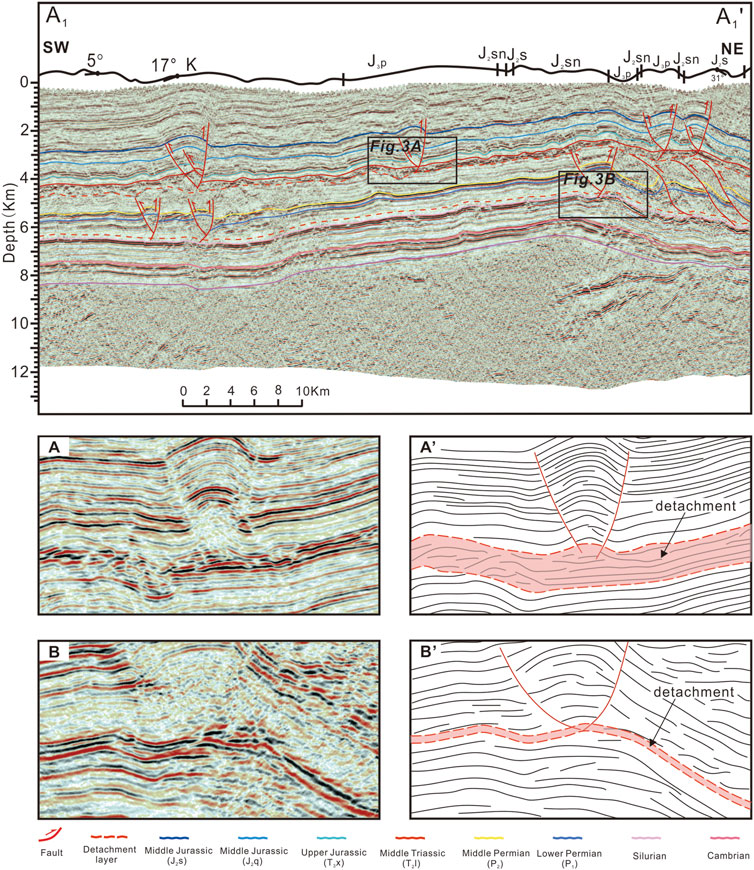
FIGURE 3. Seismic reflection characteristics of the detachment layer. (A) NE-SW regional seismic profile Hctrace710. (B) Enlarged area of seismic profile shown the Lower Triassic (Leikoupo Formation) detachment layer. (C) Line-drawing of Figure 3B. (D) Enlarged area of seismic profile shown the Lower Silurian detachment layer. (E) Line-drawing of Figure 3D.
Data and methods
Comprehensive seismic data on the Tongnanba region form the basis for studying its structural geometric features in this paper. This study employs twenty NE-SW trending and twenty-eight NW-SE trending time-migrated 2D seismic profiles. The approximate depths are converted based on an average velocity of 4,000 m per second. Although this time-depth conversion method is simple compared to the reality, the vertical stretching and compression do not affect our analysis of structure style in the study region.
We primarily employed fault-related fold, balanced section restoration technique, and forward modeling analysis to characterize the Tongnanba anticline’s superimposed deformation pattern, evolution process, and spatial structure characteristics. To constrain the underground structural model while interpreting seismic reflection profiles, we projected 1:200,000 regional geological mapping results onto seismic profiles. Two NE-SW 2D seismic profiles and five NW-SE 2D seismic profiles are chosen as examples for this explanation. Otherwise, the Trace710 seismic profile was constructed to investigate the evolution process and variations in shortening. Its tectonic strike is perpendicular to Micangshan FTB and parallel to Dabashan FTB, containing the maximum structural features. Finally, the relationship between regional geological structure, evolution processes, and petroleum is discussed. We divided the characteristics of source-reservoir-cap assemblages into those of the upper, middle, and lower tectonic deformation layers by investigating the characteristics of source-reservoir-cap assemblages of different tectonic deformation layers.
Structural geometry of the Tongnanba anticline
The regional seismic profiles’ structural analysis clarifies the structural characteristics of the piedmont area of South Dabashan and Micangshan FTB, respectively. In this paper, use two NE-SW and five NW-SE 2D seismic profiles are interpreted explain the typical tectonic features of the southern Dabashan and Micangshan front regions, with the location of the seismic profile is shown in Figure 1. The interpreted results explains that the Tongnanba anticline belt is characterized by the NW-trend long-distance detachment thrust deformation of the upper and middle deformation layers, and NE-SW trending deep basement fold structural deformation of the lower structural deformation layer, which is evidence of the prominent vertically stratified multi-detachment thrust deformation pattern of the Tongnanba anticline (Figure 4).
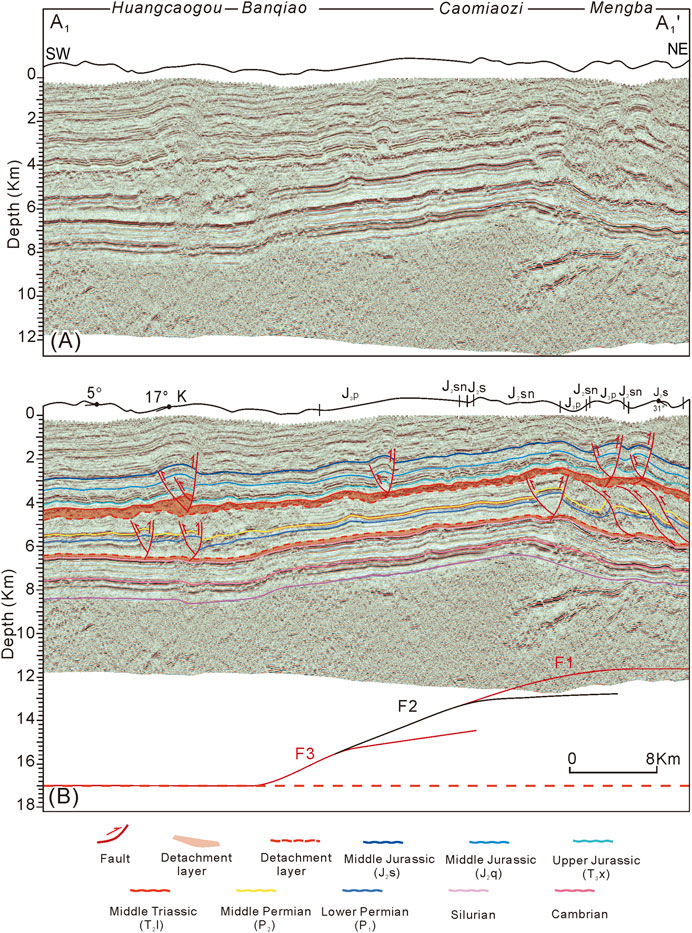
FIGURE 4. NE-SW seismic profile (A1-A1’) in the Tongnanba anticline shown the structure of Dabashan thrust system. (A, B) Seismic profile and its interpretation result for (A1-A1’). The basement faults are distributed in SE-directing, which are flat in the NE-SW striking section.
Structural analysis of NE-SW directed seismic profiles
Three deformation layers are displayed from bottom to top in the NE-SW seismic profiles: 1) The chaotic reflection with depths greater than 8 km, representing the Precambrian crystalline basement; 2) the strong continuous reflection with depths approximately 8–4 km, representing the Cambrian-Permian; and 3) the shallow, weak continuous reflection with depths approximately 4 km, representing the Triassic-Cretaceous (Figures 4A, 5A).
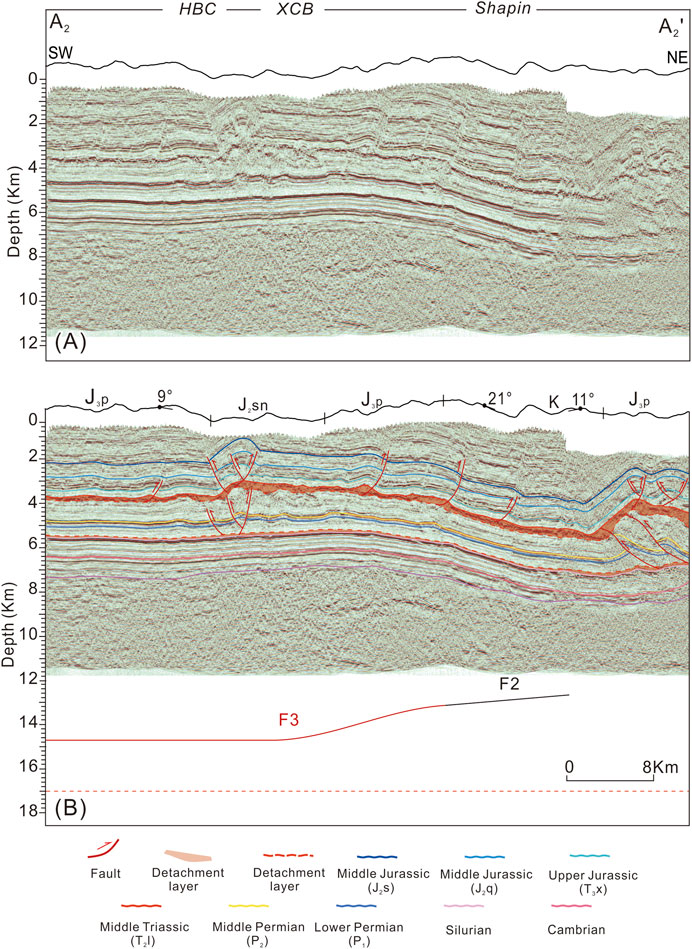
FIGURE 5. NE-SW seismic profile (A2-A2’) in the Tongnanba anticline shown the structure of Dabashan thrust system. (A, B) Seismic profile and its interpretation result for (A2-A2’). The basement fault is distributed in SE-directing, which are flat in the NE-SW striking section.
NE-SW directed seismic profiles reflect the detachment thrust deformation in the upper and middle deformation layers. On the whole, the deformation distribution is wide, but the fault displacement is small. Because of differences in the shorting strength, different styles of structures are exposed from South Dabashan to the Sichuan Basin. In the upper deformation layer, it is revealed that multiple small folds and pop-up structures developed along the upper detachment layer on both limbs, showing the unequal distance distribution of structures. There are large-scale detachment structures near the Huangcaogou and HBC area, forming the subsidiary anticline with large amplitude (Figures 4B, 5B). Also, it can be seen that multiple NE-dipping thrust faults distributed in the Shapin area and numbers of pop-up structures near the South Dabashan. Closed to the Southern Dabashan FTB, the structural deformation becomes complicated and the upper detachment layer gradually uplift. In addition, salt accumulation occurs in the upper detachment layer, resulting in the thickening of the detachment layer in the local place (Figures 4A, B).
Because of the strong thrusting, different types of structures are developed in the middle deformation layers. Like the upper deformation layers, thin-skinned thrust fold structures distributed on the two limbs of the anticline, and the position of deformation is the same as the upper deformation layers locally. Deformation close to the South Dabashan is characterized by an imbricate thrust structure, which involved the Sinian, Lower Paleozoic, and Permian and a small portion of Triassic units. Continuous imbricate structure resulted in obvious thickening of strata (Figures 4B, 5B). There are also several thrust fault and back thrust fault appear near the region of Hebachang, with the strata in the upper deformation uplifting locally.
The deformation in the lower structure deformation layer is controlled by Micangshan FTB, which form the SE-directing thrust faults. Therefore, in the NE-SW seismic profiles, these SE-directing faults are showed as several branch fault flats superimposed one by one. And there are different fault flats in different seismic profiles.
Structural analysis of NW-SE directed seismic profiles
NW-SE seismic profiles reflect the deformation formed by Micangshan FTB, with the basement anticline of the Tongnanba area (Figures 6, 7). There are also three parts to the reflections, but unlike the NE-SW profile, there is no discernible dislocation of the reflection axis in the shallow layers. On the contrary, obvious reflection axis deformation occurs in the middle and deep layers, which reveals the basement fold structure and its control over the upper strata. The seismic profile also reveals that the shallow, middle, and deep structure deformation layers exhibit partially synchronous deformation in the NW-SE direction, indicating that the deep structure deformation time is later than that of the middle and shallow long-distance detachment thrust structural layers.
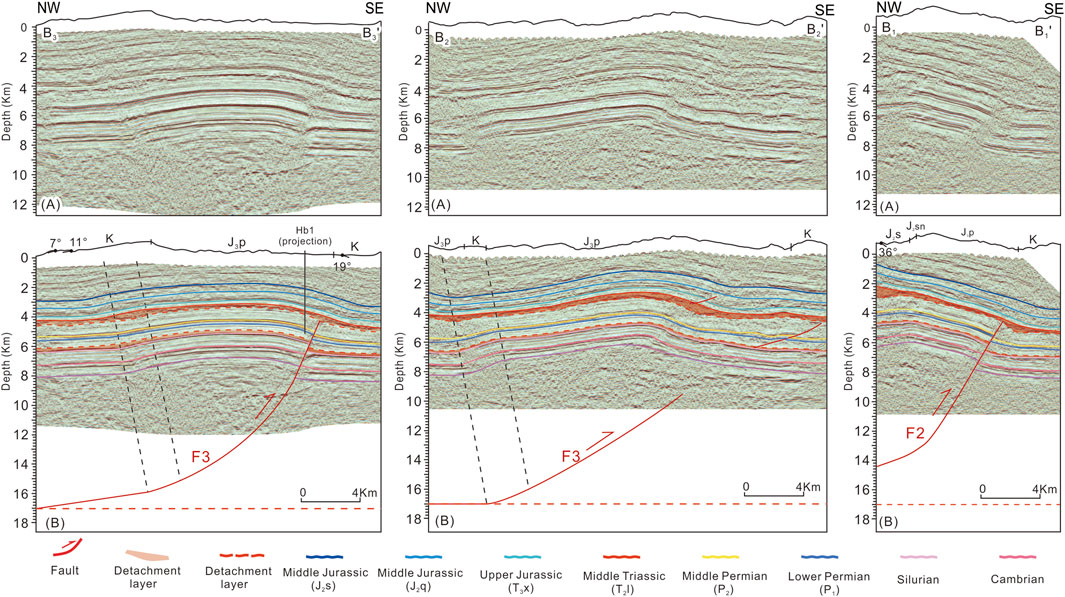
FIGURE 6. NW-SE seismic profile in the Tongnanba anticline shown the structure of Micangshan thrust system. From NW to SE showed the Seismic profile and interpretation result for B1-B1’, B2-B2’, and B3-B3’.
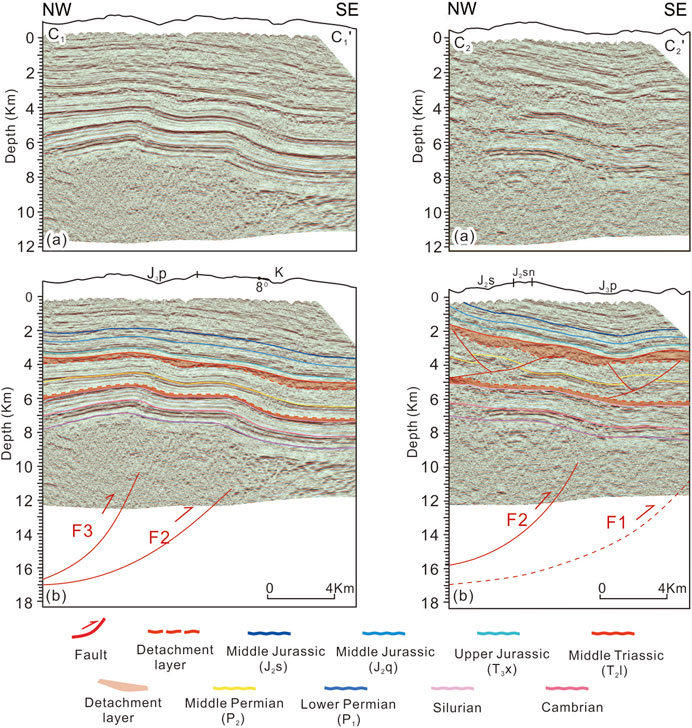
FIGURE 7. NW-SE seismic profile in the northeastern Tongnanba anticline shown the structure of Micangshan thrust system. From NW to SE showed the Seismic profile and interpretation result for C1-C1’ and C2-C2’.
Generally, from NW to SE, the Tongnanba anticline is a broad anticline whose shape changes from monocline to asymmetric anticline from northeast to southwest, and it can be seen that there are two types of basement anticlines arise in the study area (Figures 6, 7). One is single basement anticline controlled by one basement fault (Figure 6). According to 2D move-forward modeling, it is inferred in this paper that the pre-Sinian basement detachment layer has a significant burial depth about 17 km and maybe a weak layer in the middle-lower crust. From SW to NE, the anticline transited from broad gentle anticline to monocline. The basement fault disappeared downward into the lower detachment, and terminated in the upper detachment layer locally, showing that the middle structure deformation layer is severed by basement faults (Figure 6 B1-B1’, B3-B3’). It is worth noting that the fault displacement decreases gradually from Sinian to the upper detachment layer. In specific profiles, the basement fault does not cut through the upper strata, and SE-dipping faults appeared in the middle and upper deformation layers, representing the NW-strike faults from Dabashan FTB which cause the stratum to thicken. Under the adjustment of the upper detachment layer, the upper deformation layer has no noticeable thrust faults, and the strata in the upper deformation layer is relatively continuous and complete. Moreover, due to compression, the upper detachment layer thickens close to the Micangshan.
Another one is double basement anticline controlled by two basement faults (Figure 7). Like the anticline which is controlled by one basement fault, the upper detachment layer thickens because of compression from Micangshan FTB. And near south Dabashan, a series of NW-strike thrust faults cause the middle deformation layer to thicken (Figure 7 C1-C1’b). However, the difference is that the lower and middle deformation layer are double anticlines, while the upper tectonic deformation is simple anticlines, which illustrate that in the vertical it is decoupled locally. According to the planar distribution of the NW-SE seismic profiles, it can be seen that the single basement anticline and the complex basement anticline appear alternately, which represented the transitional location of two basement faults.
According to the seismic profile interpretation and balanced cross-section restoration, it is confirmed that the basement faults in the lower structure deformation layer conforms to the Trishear fault-propagation fold model (Suppe J and Medwedeff D A, 1984; Erslev, 1991; Allinendinger R W, 1998). It is known that the Trishear fault-propagation fold model has the characteristics that the shape of the anticline is asymmetric, whose front wing is narrow and the rear wing is wide. Besides, the fold becomes more and more tightly closed with the increase of depth and the deformation cannot be transmitted forward. The fault displacement gradually increases from bottom to top. In this paper, the NW-SE seismic profiles have the same feature. Firstly, the seismic profile shows asymmetric anticline in Figures 6, 7, with the front limb being steeper than the back limb. The dip angle of the front limb decreasing gradually from bottom to top (Figure 7). Otherwise, the fault displacement gradually decreases from Sinian to the lower Triassic. Furthermore, there is no discernible deformation propagated towards the basin, indicating that the basement deformation the basement deformation from Micangshan FTB is no longer transmitting forward. All the above proved that the basement faults are consistent with the Trishear fault-propagation fold model.
Characteristics of fault distribution
To comprehend the fault distribution and geometric style of the Tongnanba anticline systematically and comprehensively, we analyze the planar fault distribution characteristics of the different structure deformation layers using regional seismic profiles (Figure 8).
In the upper structure deformation layer, under the compression of South Dabashan, it forms a series of NW-SE and NS striking pop-up structures which were primarily formed in Fuyangba and Xindianzi area. Near the Hebachang area develop three groups of NW-trending thrust and back-thrust faults, and faults with slight displacement dominate the Fuyangba. On the southern side of the Well M-2 forms two near-NS faults. Multiple large-displacement faults distribute near Xindianzi and Lianghekou area, illustrating the intense compression in the vicinity of the Southern Dabashan (Figure 8A).
The middle structure deformation layer, also under the compression from South Dabashan, develops a series of NW and near-EW trending fault groups. Compared to the upper structure deformation layer, no faults have formed near the well HB-101 and HB-2, indicating that the compression from South Dabashan has yet to be transmitted here. In the Hebachang region, there are three groups of NW-trending thrust and back-thrust faults whose positions corresponded to those of the upper structure deformation layer. Fuyangba area is devoid of small faults, whereas in the Xindianzi and Lianghekou area exhibit near-EW imbricate structures which structural strike consistent with the western section of the Dabashan FTB (Figure 8B).
Compared to the upper and middle structure deformation layers, the lower structure deformation layer has the opposite fault distribution direction. A group of NE-trending thrust faults form a pattern of lateral spread. The F1 fault has the smallest distribution range, which terminates north of Lianghekou. Fault F2 extends northeast from HC-1, while Fault F3 extends northeast to the Shaping region. These faults are stacked on top of one another in a piggyback fashion (Figure 8C), which controlled the structure of the lower structural deformation layer.
Under the compression along the South Dabashan and Micangshan, the planar distribution of faults reveals that faults of different stages have diverse distribution characteristics. The faults in the upper and middle structural deformation layers parallel or obliquely intersect the structural trend of South Dabashan, resulting in groups of thrust and back-thrust faults of varying scales. The NE-SW striking faults in the lower structural deformation layer are nearly parallel or oblique to the trend of the Micangshan thrust belt. These faults traversed the entire Tongnanba anticline, forming a structural combination of NW and NE striking faults stacked vertically.
Restoration of tectonic evolution
The complex deformation of the Tongnanba anticline can be attributed to the multistage regional tectonic activities. This article, the NW-SE seismic profile is restored under the analysis of structure deformation in the Tongnanba anticline for clarifying the structure deformation process of different stages in the Tongnanba region. Different stages of the compression generated by the Micangshan and South Dabashan FTB were transmitted to the Tongnanba region, resulting in the multi-detachment thrust structure (Figure 9).
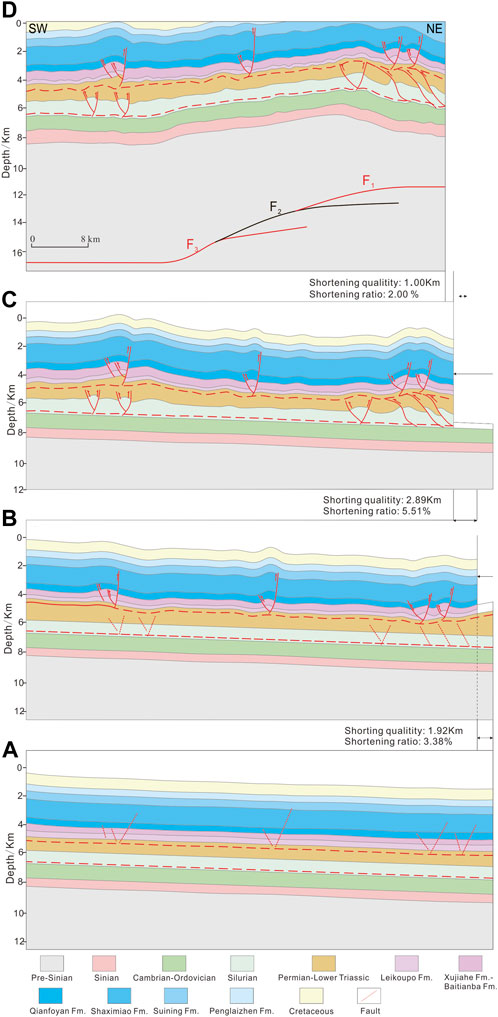
FIGURE 9. Multi-stage deformation of the Tongnanba anticline (see Figure 1A–A’ for the profile).
This article suggested that the upper and middle structure deformation layers formed earlier than the lower structure deformation layer, which means that the deformation from South Dabashan FTB is earlier than Micangshan FTB. Firstly, it is evident that three structure deformation layers are coupled locally in the NE-SW and NW-SE seismic profiles, which have consistent axes in some seismic profiles. Otherwise, Sandbox simulation experiment indicates that the structure trace of shallow deformation if the paleo-uplift was formed first (Marshak, 2004; Long et al., 2021). And we believe that the middle and shallow structures could not spread forward across the paleo-uplift. In addition, there is no significant regional unconformity in the stratigraphic sequences, which may imply that the development of the anticline is in the late stage. Therefore, the deformation from south Dabashan FTB is early than Micangshan FTB.
In the early stage, a series of thrust and back-thrust faults were formed during the early stage that South Dabashan produced NW-SE compression (Figure 9B). The deformation traveled long distances along the upper detachment layer. Several imbricate thrust structures develop near the front of South Dabashan during the middle stage (Figure 9C). And there are a series of thrust and back-thrust faults occur far from South Dabashan. The upper structure deformation layer is further folded due to the substantial folding deformation of the middle structure deformation layer. In the late stage, under the compression of Micangshan FTB, three forward thrust fault were formed which were characterized by laterally-arranged piggyback thrust structures. In the meantime, these faults modified the deformation which is generated earlier (Figure 9D).
According to the above analysis, the Tongnanba anticline is subjected to two long-distance detachment thrust formations above the Silurian mudstone detachment layer, producing near-EW and NW-SE striking faults in the shallow and middle structure deformation layers. Subsequently, basement structure produced by Micangshan FTB modified the upper deformation. These tectonic processes combine to form the Tongnanba anticline’s multi-level superimposed structure.
Discussion
A new model explains the superimposed structure
The lateral extension-superposition model of the deep basement detachment thrust structure
We use 2D MOVE for the forward modeling of basement faults to verify the validity of the model of the basement fault structure. In the model, the dip angle of the faults is 20.5°, the trishear angle is 80°, and the fault propagation slip ratio is 1 (Figure 10).
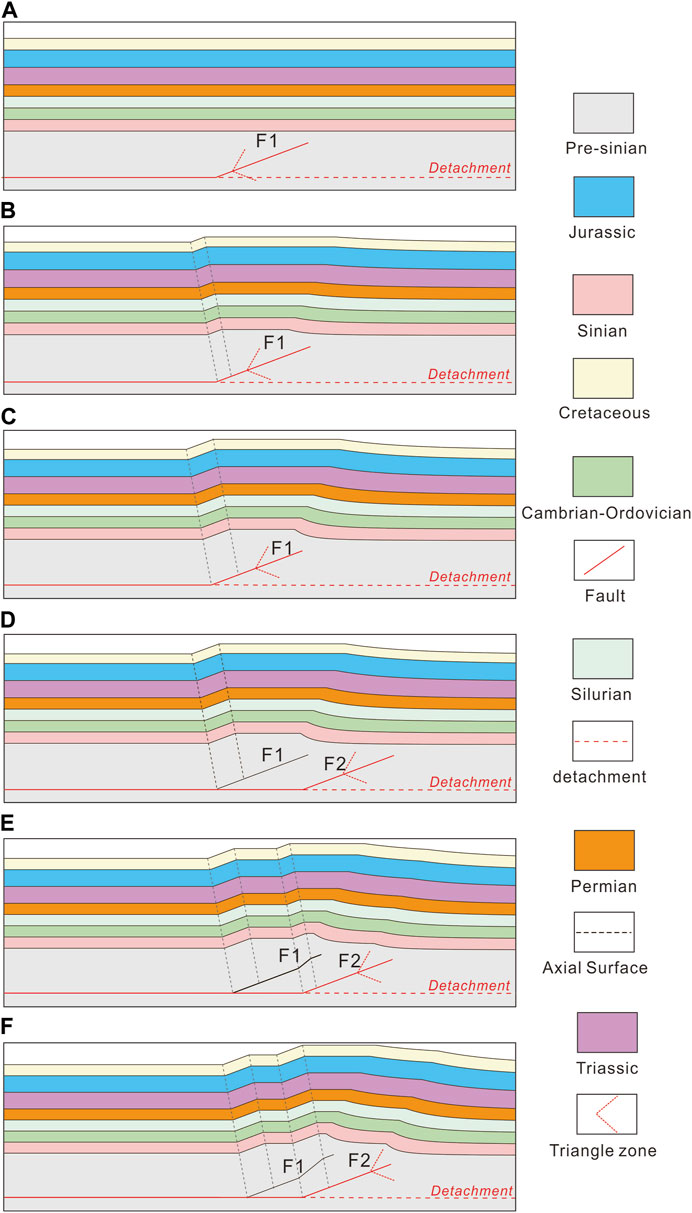
FIGURE 10. Sequential model of the growth Tongnanba anticline controlled by lower detachment. F1: dip angle is 20.5°, trishear angle is 80°, propagation/slip ratio is 1; F2: dip angle is 20.5°, trishear angle is 80°, propagation/slip ratio is 1.
As depicted in Figure 10, the fault F1 developed in the basement, with its lower endpoint in the pre-Sinian detachment layer and its upper endpoint in the basement (Figure 10A). When the displacement is transmitted along F1, the rear limb bends and slides along the fault, forming a broad anticline. At this time, the displacement of the fault is the distance between the inactive axial plane and the active axial plane projected onto Fault F1 (Figure 10B). The anticline core contracted when the displacement was increased, and the high point of the anticline roes at the same time (Figure 10C). This model resembles the single anticline in the Tongnanba region.
When the fault F2 is created along the basement detachment layer in the front limb direction of a previously formed anticline, it manifested two forward thrust faults, with fault F2 controlling the deformation and generating new displacement along the fault F2. And the fault F1 deformed passively in response to the fault F2 (Figure 10D). The anticline formed previously was altered and reflected the double anticline with one anticline high point (Figure 10E). With the displacement increasing, the folds become more tightly closed. This model is consistent with the double anticline of the Tongnanba anticline, which is controlled by two branch faults.
Based on the analysis and forward modeling, we established the basement fault spatial distribution of Tongnanba anticline (Figure 11). It is clear that three faults, developed from NE to SW, result in the broad anticline. While two faults which is stacking between the fault slopes displays a double anticline. Under the influence of basement faults, it is showing that the Tongnanba anticline has a complex geological structure, which is segmented from NE to SW.
Implications for oil and gas explorations
Complex segmentation is developed in the Tongnanba anticline due to the multi-detachment superimposed structure. The structural segmentation and tectonic evolution are closely related to hydrocarbon accumulation. Consequently, it is necessary to analyses the hydrocarbon accumulation in the vertical and horizontal direction (Figure 12).
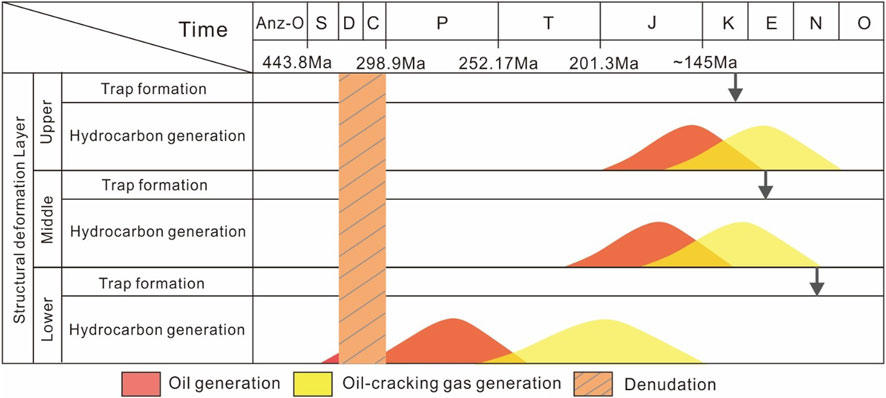
FIGURE 12. The relationship between hydrocarbon generation and structural trap formation of multilayer source rocks in Tongnanba Anticline.
In the upper structure deformation layer, the shallow Triassic Xujiahe Formation, such as the continental 2nd and 4th Members, are the focus of shallow exploration. The source rocks are the 3rd and 5th Members of the Xujiahe Formation, and the regional caprock is the upper mudstone. Consequently, the advantageous source-reservoir-cap combination is formed (Du et al., 2019; Jiang et al., 2020). In the Late Jurassic, the source rock reached the peak of hydrocarbon generation (Wang et al., 2014) (Figure 12). During the Late Cretaceous, intense compression is produced from South Dabashan, resulting in crude oil and natural gas generation. Thus, structural hydrocarbon reservoir is formed, in which the shallow structural traps is corresponded with the oil and gas generation of the source rocks. Otherwise, faults in the upper structure deformation layer enhance the physical properties of the reservoir and connect the source rocks and reservoir effectively. Therefore, the reconstruction of shallow layers from late basement faults may alter or destroy previously formed oil and gas reservoirs.
In the middle structure deformation layer, it develops the gas reservoirs of the granular beach facies in the 3rd Member of the Lower Triassic Feixianguan Formation and the reef-beach facies of the Upper Permian Changxing Formation. Permian Wujiaping Formation and Dalong Formation are primarily source rocks. In addition, older source rocks like Silurian may also contribute to the middle trap. The Jialingjiang Formation’s gypsum rocks serve as a high-quality regional caprock (Lan et al., 2019; Wang and Ji, 2020). During the Early to Middle Jurassic, oil production peaked in the Permian source rocks. The paleo-lithologic reservoirs may be taken shape in the Feixianguan Formation and Changxing Formation On the premise that no paleo-structures existed at the time. In the Late Jurassic, the crude oil cracked to produced gas, leading to paleo-gas reservoir (Zhang et al., 2013; Liu et al., 2019). The structural trap of the middle structure deformation layer was formed after the Late Cretaceous because of Dabashan compression, which corresponded to the stage of thermal cracking and gas generation in crude oil. There is some possibility that structural-lithologic or structural hydrocarbon reservoirs are existed. Besides, these hydrocarbon reservoirs are difficult to be modified by the basement faults in the late stage. So special consideration must be given to these reservoirs during exploring the middle layers.
Dengying Formation in the Sinian is the exploration focus in the lower structure deformation layer, which consists primarily of alga-rich microbial dolomite. The source rocks consist of mudstone and shale from the Qiongzhusi Formation and Doushantuo Formation, with Silurian mudstone serving as the regional caprock (Jia et al., 2015; Lan et al., 2019; Guo et al., 2020; Guo et al., 2020). During the Late Silurian, with the burial depths increasing, the source rocks of the Qiongzhusi Formation and Sinian began producing oil, but the paleo-reservoir was initially formed during the Middle Permian (Liu et al., 2021). It may migrate laterally into the platform margin at the higher elevation to form lithologic reservoirs under the condition of no significant paleo-structures. In the Late Triassic, the crude oil in the Sinian paleo-reservoirs cracked into gas and paleo-gas reservoirs were formed because of the increased formation temperature (Liu et al., 2021) (Figure 12). The crude oil completed the oil-cracking process before the Jurassic and the basement deformation occurred after oil-cracking process, which means that no structural traps is matched with the hydrocarbon. Therefore, early-formed paleolithic reservoirs in deep layers may be the key to oil and gas exploration.
Conclusion
(1) Due to the multi-stage uplift tectonic events of Micangshan and South Dabashan, the Tongnanba anticline is primarily controlled by three sets of detachment layers: the pre-Sinnian basement, Silurian mudstone, and Lower Triassic gypsum salt rock to form a complex multistage, multi-directional and multi-level superimposed structure. As a result, the Tongnanba anticline is vertically stratified and differentiated.
(2) The NW-SE striking long-distance detachment thrust structures develop in the upper and middle structural deformation layers of the Tongnanba anticline because of the early stage thrusting of South Dabashan, which forms a series of thrust and imbricate thrust structures. In the late stage, the Micangshan uplift produces three basement faults in the lower structure deformation layer that are laterally arranged in a piggyback fashion. The early-formed deformation is reconstructed by basement faults. Consequently, the Tongnanba anticline is segmented and planarly differentiated.
(3) The Tongnanba anticline has hydrocarbon accumulation that is vertically stratified and laterally segmented. The formation of shallow gas reservoirs corresponds well with the emergence of structural traps. Nevertheless, the formation of deep oil and gas reservoirs temporally mismatches the formation of structural traps, making a complex process of reservoir formation. The exploration may be given to pay attention to early paleo-oil and gas reservoirs.
Data availability statement
The original contributions presented in the study are included in the article/Supplementary Material, further inquiries can be directed to the corresponding authors.
Author contributions
CZ and ZY come up with this research topic, completed the seismic interpretations and then wrote the manuscript. ZY put forward a lot of valuable comments for the figures and the manuscript. RR, WL, SN, and YG provided important advice. All authors contributed to the article and approved the submitted version.
Funding
The authors declare that this study received funding from PetroChina Science and Technology Project (Grants No. 2021DJ0301, 2023ZZ0202). The funder was not involved in the study design, collection, analysis, interpretation of data, the writing of this article, or the decision to submit it for publication. This research was financially supported by the National Natural Science Foundation of China (Grant No. U22B6002).
Acknowledgments
We express our gratitude to Denegfa He, Guanshen Liu, Fanglei Tian and others for technical support.
Conflict of interest
Authors YZ, ZC, YZ, NS, LW, RR, and GY were employed by PetroChina.
Publisher’s note
All claims expressed in this article are solely those of the authors and do not necessarily represent those of their affiliated organizations, or those of the publisher, the editors and the reviewers. Any product that may be evaluated in this article, or claim that may be made by its manufacturer, is not guaranteed or endorsed by the publisher.
References
Allinendinger, R. W. (1998). Inverse and forward numerical modeling of trishear fault-propagation folds. Tectonics 17 (4), 640–656. doi:10.1029/98tc01907
Chen, L., He, D., Wen, Z., Mei, Q., and Li, Y. (2016). The geometry, kinematics of Tongnanba anticline and its structural model. Chin. J. Geol. 51 (2), 384–401. doi:10.12017/dzkx.2016.004
Chen, L. (2015). Structural geometric, kinematic features and three-dimensional restoration of Tongnanba anticline. (Beijing): China University of Geosciences.
Dario, C., Claudio, M., and Mossimo, Z. (2010). Contractional deformation and Neogene tectonic evolution of the pergola-melandro valley area (southern apennines). GeoActa (Bologna) 9, 1e19.
Deng, H., and Koyi, H. A. (2015). Mega arrowhead interference patterns in the central part of the Yanshan orogenic belt, North China. J. Struct. Geol. 80, 25–37. doi:10.1016/j.jsg.2015.06.004
Deng, H., Koyi, H. A., and Nilfouroushan, F. (2016). Superimposed folding and thrusting by two phases of mutually orthogonal or oblique shortening in analogue models[J]. J. Struct. Geol. 83, 28–45. doi:10.1016/j.jsg.2015.08.005
Dong, S., Gao, R., Yin, A., Guo, T., Zhang, Y., Hu, J., et al. (2013). What drove continued continent-continent convergence after ocean closure? Insights from high-resolution seismic-reflection profiling across the daba Shan in central China. Geology 41 (6), 671–674. doi:10.1130/g34161.1
Dong, S., Hu, J., Shi, W., Zhang, Z., and Liu, G. (2006). Jurassic superposed folding and jurassic foreland in the daba mountain, central China. Acta Geosci. Sin. 27 (05), 403–410.
Du, H., Wang, W., Shi, Z., Tan, J., Cao, H., and Yin, X. (2019). Geochemical characteristics and source of natural gas of the upper triassic xuiiahe Formation in malubei area, northeastern Sichuan Basin. Oil Gas Geol. 40 (01), 34–40. doi:10.11743/ogg20190104
Erslev, E. A. (1991). Trishear fault-propagation folding. Geology 19 (6), 617–620. doi:10.1130/0091-7613(1991)019<0617:tfpf>2.3.co;2
Ghosh, S. K., Mandal, N., Khan, D., and Deb, S. (1992). Modes of superposed buckling in single layers controlled by initial tightness of early folds. J. Struct. Geol. 14 (4), 381–394. doi:10.1016/0191-8141(92)90100-b
Grasemann, B., Wiesmayr, G., Draganits, E., and Fusseis, F. (2004). Classification of refold structures, 119–125.
Guo, T., He, X., Zeng, P., Gao, Y., Zhang, P., and He, G. (2020a). Geological characteristics and beneficial development scheme of shale gas reservoirs in complex tectonic regions: A case study of Wufeng-longmaxi formations in Sichuan Basin and its periphery. Acta Pet. Sin. 41 (12), 1490–1500. doi:10.7623/syxb202012004
Guo, X., Hu, D., Huang, R., Duan, J., Jiang, Z., and Zhu, X. (2020b). Feature of paleo-oil pools in the Sinian dengying formation, northeastern Sichuan Basin, and its significance to exploration. Oil Gas Geol. 41 (04), 673–683. doi:10.11743/ogg20200402
He, D., Li, D., Zhang, G., Zhao, L., Fan, C., Lu, R., et al. (2011). Formation and evolution of multi-cycle superposed Sichuan Basin, China. Chin. J. Geol. 46 (03), 589–606.
Hou, L., Zhang, C., Lin, Y., Li, C., Huang, Y., and Dong, T. (2020). From oblique arc-continent collision to orthogonal plate subduction in the southeastern central Asia Orogenic Belt during Paleozoic: Evidence from superimposed folds at the northern margin of the north China Craton. J. Asian Earth Sci. 200, 104499–109120. doi:10.1016/j.jseaes.2020.104499
Hu, J., Chen, H., Qu, H., Wu, G., Yang, J., and Zhang, Z. (2012). Mesozoic deformations of the Dabashan in the southern Qinling orogen, central China. J. Asian Earth Sci. 47, 171–184. doi:10.1016/j.jseaes.2011.12.015
Huang, H., Mei, Q., He, D., Lu, R., and Li, Y. (2021). Multi-level detachment deformation of the west segment of the South Dabashan fold-and-thrust belt, South China: Insights from seismic-reflection profiling. Front. Earth Sci. 24 (09), 1–17. doi:10.3389/feart.2021.633816
Jia, C., Li, B., Lei, Y., and Chen, Z. (2013). The structure of Circum-Tibetan Plateau Basin-Range System and the large gas provinces. Sci. China Earth Sci. 56, 1853–1863. doi:10.1007/s11430-013-4649-7
Jia, C., and Pang, X. (2015). Research process and main development directions of deep hydrocarbon geological theories. Acta Pet. Sin. 36 (12), 1457–1469. doi:10.7623/syxb201512001
Jiang, F., Su, P., and Qin, Q. (2008). Discussion of main tectogenetic models in Tongnanba area. Fault-Block Oil Gas Field 15 (03), 14–17.
Jiang, Y., Li, M., Wang, L., Zeng, T., Liu, J., and Li, J. (2020). Controlling effect of faults on tight sandstone natural gas accumulation and enrichment in Tongnanba Area, Northeast Sichuan. J. China Univ. Petroleum Ed. Nat. Sci. 44 (05), 19–31. doi:10.3969/j.issn.1673-5005.2020.05.003
Jin, Z., Wan, G., Cui, Z., Wang, J., Yang, X., and Bai, W. (2012). Key tectonic change epoch and hydrocarbon accumulation periods of continental clastic reservoir in Sichuan Basin. Fault-Block Oil Gas Field 19 (03), 273–277.
Jin, Z., Long, S., Zhou, Y., Wo, Y., Xiao, K., Yang, Z., et al. (2006). A study on the distribution of saline-deposit in southern China. Oil Gas Geol. 27 (05), 571–583+593.
Kusky, T. M., and Loring, D. P. (2001). Structural and U/Pb chronology of superimposed folds, adirondack Mountains: Implications for the tectonic evolution of the grenville province. J. Geodyn. 32 (3), 395–418. doi:10.1016/s0264-3707(01)00038-2
Lan, C., Xu, Z., Ma, X., Hu, C., Chen, H., and Zou, H. (2019). Development and distribution of mound-shoal complex in the Sinian dengying formation, Sichuan Basin and its control on reservoirs. Acta Pet. Sin. 40 (09), 1069–1084.
Li, H. (2016). Accumulation process and pattern of oolitic shoal gas pools in the platform: A case from member 3 of lower triassic Feixianguan Formation in the heba area, northeastern Sichuan Basin. Petroleum Explor. Dev. 43 (05), 787–797. doi:10.1016/s1876-3804(16)30094-5
Li, J., Dong, S., Yin, A., Zhang, Y., and Shi, W. (2015). Mesozoic tectonic evolution of the Daba Shan Thrust Belt in the southern Qinling orogen, central China: Constraints from surface geology and reflection seismology. Tectonics 34 (8), 1545–1575. doi:10.1002/2014tc003813
Li, J., Zhang, Y., Dong, S., Shi, W., and Li, H. (2010). Apatite fission track thermochronologic constraint on Late Mesozoic uplifting of the Fenghuangshan basement uplift. Chin. J. Geol. 45 (4), 969–986.
Li, J., Zhang, Y., Dong, S., and Shi, W. (2013). Structural and geochronological constraints on the mesozoic tectonic evolution of the North dabashan zone, south qinling, central China. J. Asian Earth Sci. 64, 99–114. doi:10.1016/j.jseaes.2012.12.001
Li, W., Liu, S., Wang, Y., Qian, T., and Gao, T. (2017). Duplex thrusting in the South Dabashan arcuate belt, central China. J. Struct. Geol. 103, 120–136. doi:10.1016/j.jsg.2017.09.007
Li, Y., Qu, S., Liu, S., and Zhang, H. (2008). Structural characters and mechanism in Micang Shan and southern Daba Shan Mountains front. Geotect. Metallogenia 32 (03), 285–292.
Liu, S., Li, Z., Deng, B., Zhong, Y., Wen, L., Sun, W., et al. (2021). Occurrence morphology of bitumen in Dengying Formation deep and ultra-deep carbonate reservoirs of the Sichuan Basin and its indicating significance to oil and gas reservoirs. Nat. Gas. Ind. 41 (08), 102–112.
Liu, Z., Luo, K., Tang, Y., Yang, F., Mei, L., and Shen, C. (2019). Critical tectonic periods and the response of gas accumulation in non-marine tight sandstone reservoir in Yuanba-Tongnanba area, Sichuan Basin. Earth Sci. 44 (03), 756–772. doi:10.3799/dqkx.2019.032
Long, Y., Chen, H., Cheng, X., Deng, H., and Lin, X. (2021). Influence of paleo-uplift on structural deformation of salt-bearing fold-and-thrust belt: Insights from physical modeling. J. Struct. Geol. 153 (153-Dec), 104445. doi:10.1016/j.jsg.2021.104445
Ma, D., Wang, H., Zhang, X., He, D., Fan, R., Wang, Y., et al. (2023). Analogue modeling of structural deformation of Tongnanba Anticline in mesozoic and cenozoic, NE Sichuan Basin. Earth Sci. 48 (4), 1307–1320. doi:10.3799/dqkx.2022.423
Marshak, S. (2005). Salients, recesses, arcs, oroclines, and syntaxes-A review of ideas concerning the formation of map-view curves in fold-thrust belts. AAPG Mem. 82 (82), 131–156.
Molli, G., Koehn, D., Allacorta, L., Danese, L., Zampelli, S., and Zazzeri, M. (2022). Superimposed structures, incremental strain and deformation path from field data to modelling: A case study from the Alpi Apuane metamorphic core complex (NW Tuscany, Italy). J. Struct. Geol. 161, 104676. doi:10.1016/j.jsg.2022.104676
Ramsay, J. G. (1962). Interference patterns produced by the superposition of folds of similar type. J. Geol. 60, 466–481. doi:10.1086/626837
Saha, D. (2002). Multi-stage deformation in the Nallamalai fold belt, Cuddapah Basin, South India - implications for mesoproterozoic tectonisrn along Southeastern margin of India. Gondwana Reseach 5 (3), 701e719.
Shah, Z. S., Sayab, M., Aerden, D., and Iqbal, Q. (2013). Formation mechanism and tectonic significance of millipede microstructures in the NW Himalaya. J. Asian Earth Sci. 59, 3e13–13. doi:10.1016/j.jseaes.2012.05.001
Shi, W., Zhang, Y., Dong, S., Hu, J., Wiesinger, M., Ratschbacher, L., et al. (2012). Intra-continental dabashan orocline, southwestern qinling, central China. J. Asian Earth Sci. 46, 20–38. doi:10.1016/j.jseaes.2011.10.005
Sun, D. (2011). The structural character and meso-cenozoic evolution of micang mountain structural Zone, Northern Sichuan Basin. China: Chengdu University of Technology.
Suppe, J., and Medwedeff, D. A. (1984). Fault-Propagation folding geological society of America abstracts with programs, 670.
Wang, R., Zhang, Y., and Xie, G. (2013). Physical modeling of fold-and-thrust belt evolution and triangle zone development: Dabashan foreland belt (Northeast Sichuan basin, China) as an example. Acta Geol. Sinica-English Ed. 87 (01), 59–72. doi:10.1111/1755-6724.12030
Wang, W., Zhang, X., Liu, R., Luo, H., and Wang, G. (2014). Origin of natural gas in terrestrial reservoirs in Yuanba and Heba regions in northeastern Sichuan Basin, China. Acta Pet. Sin. 35 (01), 26–36.
Wang, W., and Chunhui, J. (2020). Genesis and exploration direction of high-efficiency gas reservoir in the third member of Feixianguan Formation in Tongnanba area. Petroleum Geol. Recovery Effic. 27 (03), 39–47.
Wen, Z., He, D., Fan, C., and Meng, Z. (2013). Geomentry and kinematics model of Donghe geological cross section of Micangshan Mountain and constraint to the intracontinental subduction in the northern Yangtze. Chin. J. Geol. 48 (01), 93–108.
Wu, L., Qian, J., Xiao, A., Shen, Z., Wang, L., Wei, G., et al. (2011). Structural analysis of the Micang Shan basement-involved thrust belt in the Western edge of the Yangtze Block. Acta Petrol. Sin. 94 (02), 426–438.
Wu, S., Ma, Y., Jin, Z., Tang, L., and Li, R. (2006). Tectonic evolution and oil and gas accumulation in the East Micangshan foreland basin, Sichuan. Petroleum Explor. Dev. 33 (01), 14–16.
Xu, H., Liu, S., Qu, G., Li, Y., Sun, G., and Liu, K. (2009). Structural characteristics and formation mechanism in the micangshan foreland, South China. Acta Geol. Sinica-English Ed. 83 (01), 81–91. doi:10.1111/j.1755-6724.2009.00010.x
Zhang, J., Chang, J., Li, W., Fu, X., Yang, L., and He, Y. (2022). Tectono-thermal evolution of the micangshan uplift in thenorthern Sichuan Basin. Sediment. Geol. Tethyan Geol.
Zhang, Q., Chen, Z., Zhao, Y., Zhang, W., and Luo, W. (2013). Relationship between tectonic evolution and hydrocarbon accumulation in the Changxing and Feixianguan Fms in the frontal concealed structural zone of Micangshan Mountain, northeastern Sichuan Basin. Nat. Gas. Ind. 33 (05), 24–29. doi:10.3787/J.ISSN.1000-0976.2013.05.004
Zhang, Y., Dong, S., Li, J., and Shi, W. (2011). Mesozoic multi-directional compressional tectonics and formation-reformation of Sichuan basin. Geol. China 38 (02), 233–250.
Zhang, Y., Shi, W., Li, J., Wang, R., Li, H., Dong, S., et al. (2010). Formation mechanism of the Dabashan foreland arc-shaped structural belt. Acta Geol. Sin. 84 (09), 1300–1315.
Zhang, Z. (2019). Superposed buckle folding at the upper structural levels in Western dabashan: Example from the Jianchi area in zhenba county. Earth Sci. Front. 26 (2), 001–015. doi:10.13745/j.esf.sf.2019.3.10
Keywords: superimposed structure, multi-detachments, Micangshan, Dabashan, Sichuan basin
Citation: Zhai Y, Chen Z, Zhang Y, Su N, Wang L, Ren R and Yang G (2023) A new superimposed model of the Tongnanba anticline in northeastern Sichuan and its exploration implications. Front. Earth Sci. 11:1162586. doi: 10.3389/feart.2023.1162586
Received: 09 February 2023; Accepted: 26 June 2023;
Published: 17 July 2023.
Edited by:
Wenlong Ding, China University of Geosciences, ChinaReviewed by:
Gang Rao, Southwest Petroleum University, ChinaYangwen Pei, China University of Petroleum, Huadong, China
Copyright © 2023 Zhai, Chen, Zhang, Su, Wang, Ren and Yang. This is an open-access article distributed under the terms of the Creative Commons Attribution License (CC BY). The use, distribution or reproduction in other forums is permitted, provided the original author(s) and the copyright owner(s) are credited and that the original publication in this journal is cited, in accordance with accepted academic practice. No use, distribution or reproduction is permitted which does not comply with these terms.
*Correspondence: Zhuxin Chen, Y2hlbnpodXhpbkBwZXRyb2NoaW5hLmNvbS5jbg==