- 1BGRIMM Technology Group, Beijing, China
- 2National Centre for International Research on Green Metal Mining, Beijing, China
The strength evolution law of concentrated full tailings is closely related to the storage construction method, especially the growth law of shear strength with curing age. This study aims to investigate the influence of curing materials on the shear strength of full tailings and reveal the change law of the shear strength of concentrated full tailings with the curing age. Therefore, we carried out a comparative triaxial shear test between pure full tailings and concentrated full tailings with different curing ages. The test results show that concentrated full tailings had a 70.7% higher cohesion and a 10% larger internal friction angle (IFA) than that of pure full tailings at the age of 0 days. Moreover, the concentrated full tailings cured for 3 days had a 170.2% higher cohesion and a 12.1% larger IFA than that cured for 0 days. In addition, the concentrated full tailings cured for 7 days had a 149.4% higher cohesion and a 12.1% larger IFA than that cured for 0 days. The stress-strain curve exhibits that the shear strength of full tailings is significantly influenced by the curing material and curing age. The stress-strain curve gradually moved from strain hardening to strain softening as the curing age increased, and the failure curve had two steps. This indicates that the specimen still has a certain shear capacity despite having a shear zone. The entire specimen instantly fails when the strain exceeds that capacity. The results of this study can be used as scientific bases for the design and construction of the surface storage structures of concentrated full tailings.
1 Introduction
Mine tailings stored in the tailings storage facilities (TSFs) are normally failure-prone due to liquefaction, erosion, overtopping, piping, and excess pore water pressure. Investigating of the mechanical behavior of tailings is essentially important for engineering design and safety evaluation of the TSF. For the storage and disposal of metal ore tailings, researchers are seeking more reasonable ways to mitigate the negative impacts of wet and dry discharges. The harmless ground storage of concentrated full tailings presents an effective way to improve the TSF. The first step of harmless ground storage is to overcome the problem with the high concentration of tailings. The current approach of concentrated storage increases the concentration of tailings to 60%–70% in which a deep cone thickener is used, which directly discharges the water and steams the tailings dry. The ensuing problems include the poor permeability of the discharge and the difficulty of consolidation (Yao et al., 2012; Yan et al., 2019). The concentration problem restricts the curing and storage of tailings (Indraratna, 1994) attempted to alter the mechanics of fine tailings by mixing coarse and fine tailings and improved the utilization of fine tailings.
Concentrated full tailings are a type of enhanced tailings whose mechanical properties are altered by adding curing agents to highly concentrated tailings. The preparation of concentrated full tailings is under the field of comprehensive tailings utilization. (Yin et al., 2020), (Xu et al., 2019), (Paiva et al., 2019) . explored material modification by adding waste rock, fiber, rod mill tailings, and cement to the tailings and identified the strength change pattern of tailings modified material with different mix ratios and the main factors affecting the strength. (Li et al., 2023), (Đurđevac Ignjatović et al., 2022), (Jin et al., 2022), (Hou et al., 2018), (Cui et al., 2020), (Kiventerä et al., 2019), (Wang et al., 2008; Wang et al., 2009) examined the influence law of admixtures (e.g., slag powder, quicklime, industrial gypsum, and bentonite) on tailings strength and recommended the optimal addition ratio. Temperature has a considerable effect on the strength growth of cured tailings because it influences the hydration of cement. (Wang et al., 2021). and (Li, 2019). investigated the influence law of temperature on cemented tailings strength, revealing that low temperature has an impact on the early strength of cemented tailings backfill.
In engineering practice, cyclic loading and long-term operation lead to creep deformation. (Zou et al., 2020). and (Hou et al., 2019). investigated the strength development law of cemented tailings in the presence of cyclic loading and freeze-thaw cycles to clarify the strength development law and deformation features of cemented tailings under the two actions. (Ke, 2016), (Li, 2018), and (Zhao et al., 2016). tested the creep features and long-term strength of cemented tailings, determined the long-term strength of cemented tailings, and suggested the corresponding damage model. Since age is an important impactor on strength, (Yilmaz et al., 2015), (Jiang et al., 2022), (Yilmaz et al., 2015), and (Dong, 2017). studied the effect of the curing age on the strength of cemented tailings and reported on the evolution law and mechanism of the mechanical and chemical properties of cemented tailings with age.
Structural studies are necessary for structural design and safety assessment. The tailings used in our experiment are fine-grained tailings produced from gold beneficiation, so their mechanical properties differ from those of coarse tailings. (Agapito and Bareither, 2018), (Qin et al., 2021), and (Islam, 2021). investigated the mechanical properties of fine tailings or backfill slurry and drew conclusions on the shear, seepage, and consolidation properties of fine tailings. (Suazo et al., 2016). investigated the effects of confining stress, initial static shear stress, and void ratio on the liquefaction resistance of fine-grained tailings through triaxial tests and revealed the importance of density on liquefaction susceptibility of compressible tailings and proposed a combined correction factor to account for overburden stress and density on cyclic resistance. (Roshani et al., 2017). investigated the hydro-mechanical behavior and physical properties of mature fine tailings (MFT) under atmospheric drying through a column study and found that the hydraulic-mechanical properties of the deposited tailings are closely coupled because of several mechanisms, such as evaporation, drainage, self-consolidation, suction, and crack development. Since controlling and reducing the fluid fine tailings build-up through practical methods has been an ongoing challenge, (Beier et al., 2013) explored the geotechnical aspects of meeting regulatory strength performance criteria by employing flocculation-based dewatering of fluid fine tailings. Here, artificial mine tailings/clay mixtures were used to prepare concentrated slurries, from which beds with different compositions, thicknesses, and ages were obtained through sedimentation. (Dimitrova and Yanful, 2012). added clay to mine tailings and found that the effective friction angle varied between 35.2° and 40.4° depending on the percentage of clay in the mixtures and the type of the clay additive. Shearing under partially drained conditions yielded a total friction angle of the mixtures that was always lower than the effective friction angle and varied between 15.1° and 23.3°. Since a clear understanding on the triaxial mechanical properties of cemented pasted backfill (CPB) is important to the CPB design and the stability analysis of the backfilled CPB structure, (Xiu et al., 2021; Xiu et al., 2022). carried out triaxial compression tests of the CPB samples using the geotechnical consulting and testing system (GCTS) with different curing times, drainage conditions, and curing temperatures. The results show that the shear mechanical parameters of CPB can significantly affect the vertical stress distribution inside the CPB structure, and the strength of CPB is gradually enhanced with increasing curing time.
In this study, the hydration mechanism of cementitious materials, swelling materials, and polymers is used to develop a low-cost, modified, and enhanced curing agent by exciting the alkaline activity. The proposed curing agent employs the economical and harmless concentration and storage of full tailings from metal mines. Since the surface curing environment greatly differs from the underground, a triaxial shear test under low stress is designed to identify the relationship between the shear strength and the curing age in the surface environment. So the main innovations of this study is firstly developing a new proportion of concentrated full tailings, which is supposed to resolve the disadvantage of upstream TFS, secondly carrying out a triaxial shear strength test focusing the influence of curing age to the strength of this new material, which will reveal the increasing mechanism of strength of the concentrated full tailings and provide a scientific reference for engineering practice. Through the test, the authors explored the change law of shear strength with different curing ages. This study can be used as a basis for the structural design of surface storage facilities for concentrated tailings.
2 Mix ratio
Table 1 lists the mix ratio for the triaxial shear test on concentrated full tailings. Figure 1 presents the gradation of the tailings used. First, tailings were added to the 74% tailings slurry, making the slurry thicker. Next, curing materials were added, including slag powder, quicklime, cement, latex, and fiber. Each cubic meter of the mix includes 4.97 kg of slag powder, 2.26 kg of quicklime, 0.72 kg of cement, 0.36 kg of latex, and 0.72 kg of fiber.
3 Test methods and procedure
The triaxial shear test on concentrated full tailings was performed using an SLB-1A stress-strain controlled triaxial shear instrument manufactured by Nanjing Soil Instrument Factory Co., Ltd., as shown in Figure 2. SLB-1A type stress-strain control triaxial shear permeability tester can carry out equal stress and equal strain control for triaxial test, can carry out UU, CU, CD test, equal consolidation, equal consolidation, back pressure saturation, K0 test, stress path test and permeability test. Each part of the instrument is controlled by single chip microcomputer and can work independently, it can exchange data with the computer, thus realizing centralized data acquisition and processing. The instrument belongs to multifunctional flexible control triaxial test instrument. The main technical parameters are as follows.
1 Axial force: 0–20 kN measurement accuracy ±1% (10%-95%FS)
2 Control mode: a. Equal strain control: 0.002–4 mm/min ±10%;
b. Equal stress control: 0 kN–20 kN, control precision ±1%;
3 Sample size:
4 Confining pressure: 0–1.99MPa, control precision ±0.5%FS
5 Back pressure: 0–0.99 MPa, control precision ±0.5%FS
6 Volume change: 0–480 mL, digital display.
After mixing the full tailings slurry with a curing agent, the mixture was placed in a curing vessel with a diameter of 39.1 mm. Before testing, the excess height was removed with a geokhod knife to obtain a standard specimen with a diameter of 39.1 mm and a height of 80 mm. There were cases in which the diameter of the specimen was less than 39.1 mm because of dry shrinkage deformation. Thus, the actual diameter of the specimen was measured before the test, and the actual measured value was entered in the test system.
The test steps are as follows.
3.1 Specimen preparation
First, approximately 5 kg of full tailings is weighed, and the mass of fiber, cement, slag powder, water, and latex are weighed according to the designed mix ratio, as listed in Table 1. The above materials are evenly mixed, and specimens are prepared by the compaction method. Each specimen is divided into 5 to 8 layers with equal soil mass for compaction. After compacting a layer to the required height, that layer is polished and the next layer of the mix is added. This procedure is repeated until the last layer is compacted. Then, level the ends of the specimen in the compaction cylinder, and the specimen is taken out to weigh its mass. Standard curing (the room temperature 20°±2°, and relative humidity above 95% RH) is performed on the compacted samples to the age of 0 days, 3 days, and 7 days. Among them, the 0 days specimen is directly subjected to the triaxial shear test.
3.2 Specimen mounting
The pressure chamber base is filled with water, an impermeable plate is placed on the base, and the specimen, impermeable plate, and specimen cap are put in turn. Subsequently, the rubber membrane is applied inside the membrane tube, the tube is turned out at both ends, air is sucked through the suction hole, the membrane adheres to the inner wall of the membrane tube. The membrane that is applied outside the specimen is deflated, the two ends of the rubber membrane are turned up, and the membrane tube is taken out. Afterward, the rubber membrane is tightly tied to the pressure chamber base and specimen cap, respectively, with a rubber band. The pressure chamber cover is installed in the following steps. First, the piston is lifted to prevent colliding into the specimen, the pressure chamber cover is placed, the piston is aligned with the center of the specimen cap, and the screws are evenly tightened. Moreover, the vent is opened to fill the pressure chamber with water. The inflow rate is reduced when the pressure chamber is almost full of water. The vent is closed when water flows over the hole. The volumetric change sensor/volumetric change tube valve and pore pressure valve are closed, the surrounding pressure valves are opened, and the required confining pressure is applied. Subsequently, the lift table is raised. The piston is in contact with the specimen cap when axial force gauge has micro-readings. Finally, the readings of the axial load sensor or force gauge and axial displacement sensor or displacement gauge are set to zero.
The required confining pressure is determined by the stress state of the researched structure. In this study, the basic section of the concentrated full tailings accumulation body can be seen in Figure 3, the height of the body h=1.8 m, the unit weight of the concentrated full tailings is about 18.6 kN/m3, the maximum principal stress of this structure is not exceeding 1.8m×18.6 kN/m3=33.48kPa, so confining pressures (50, 100, 200, and 300 kPa) are adopted to simulate the stress state of the structure.
3.3 Shear test
The shear speed is set to 1.0%/min. The test machine is started to initiate the shearing process. In the beginning, measure and record the axial force and axial displacement readings once, every time the specimen produces an axial strain of 0.3%–0.4%. After the axial strain reaches 3%, extend the reading interval by measuring and recording the readings once, every time the specimen produces an axial strain of 0.7%–0.8%. The measuring/recording frequency is measured, as the readings get close to the peak values. At the end of the test, the motor is turned off, the lift table is lowered, the vent is opened to drain the water inside the pressure chamber, the pressure chamber is uncovered, the remaining water around the specimen is dried, the rubber membrane outside the chamber is removed, and the deformed shape is described.
Figure 4 presents the curing situation of the concentrated full tailings specimens. Figure 5 shows the specimen mounting of the triaxial shear test.
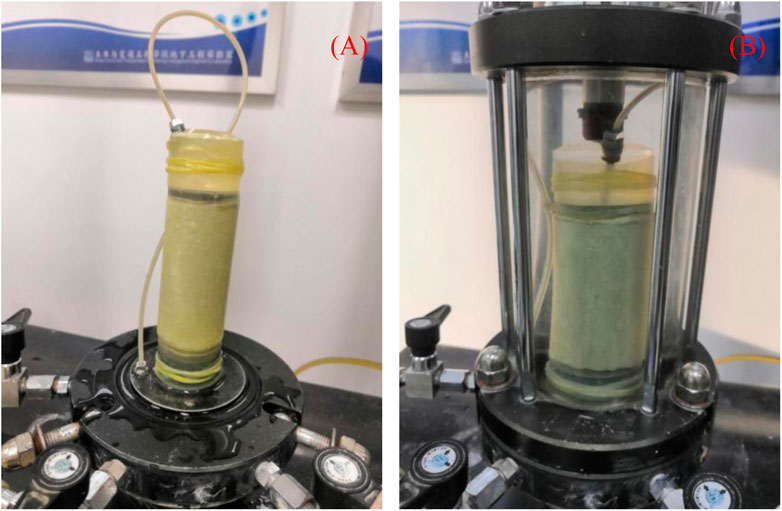
FIGURE 5. Specimen mounting of triaxial shear test. (A) Specimen loaded into rubber membrane and (B) specimen loaded into pressure chamber cover and filled with water.
4 Results and discussions
4.1 Stress-strain results
The relationship curves between the main stress difference (σ1−σ3) and axial deformation were plotted according to the data collected during the test, with the peak of (σ1−σ3) as the failure point. The stress-strain curves of pure tailings, 0 days concentrated full tailings, 3 days concentrated full tailings, and 7 days concentrated full tailings are displayed in Figure 6. Based on the test results, the stress-strain relationship of the concentrated full tailings exhibited a certain trend of strain softening compared with that of the pure tailings. For specimens of the same curing age, the strain softening increased with the confining pressure. The peak stress increased with the growing confining pressure. After the stress reached the peak, the stress difference (σ1−σ3) greatly decreased.
The stress-axial strain curve of different curing time under the same circumferential pressure are displayed in Figure 7. It can be seen that for the pure tailings, the type of stress-strain under different confining pressure is mainly strain hardening, when cementitious materials added, the stress-strain curves change into strain-softening type, even in a low confining pressure. With the increase of confining pressure, the strain-softening tendency of concentrated full tailings of different ages decreases on the whole.
Figure 8 shows the comparison of the triaxial test results of loose and concentrated tailings. By comparing the results of pure tailings with those of full tailings with different curing ages, it shows that the stress-strain curve of the pure tailings, which has no curing agent, was strain hardened. The strain hardening was accompanied by volume contraction (shear expansion) and a reduction in pore ratio. The stress-strain curve of the 0 days concentrated full tailings fell between strain hardened and strain softened, while that of the 3 days and 7 days concentrated full tailings have significant strain-softening features, which is accompanied by shear expansion and an increase in the pore ratio. Strain softening comes along with strain localization and the formation of the shear slip zone, as shown in Figure 9. The shear zone has a large local pore ratio, which suppresses the shear strength of the concentrated full tailings (Libos and Cui, 2020; Yu et al., 2022). The stress-strain curve of some specimens had two strain-softening processes, as shown in Figure 10. After the first strain softening, the deformation greatly increased. This is followed by the second strain softening, where the stress-strain curve stepped down again. Then, the deformation worsened until specimen failure occurred.
4.2 Shear failure patterns of specimens
Figure 11 shows the shear failure images of the pure tailings, as well as the 0 days, 3 days, and 7 days concentrated full tailings. The shear failure of the pure tailings was approximately soft plastic deformation without an apparent shear zone. The test on this specimen was terminated when the strain reached the limit. For the concentrated full tailings cured at different ages, the failure was accompanied by the formation of the shear zone. The shear strength greatly decreased, and the specimen failed when the zone penetrated the specimen. The shear zone of the failed 3 days specimen was more apparent than that of the failed 0 days specimen. For the 7 days concentrated full tailings, the failure pattern can be summarized as follows: local weak zones were deformed under compression. Then, the zones were further crushed, resulting in a larger deformation area. The test was terminated when the strain reached the limit. Dual strain softening processes occurred. At the end of the test, no through-shear surface was formed, and part of the specimen remained intact.
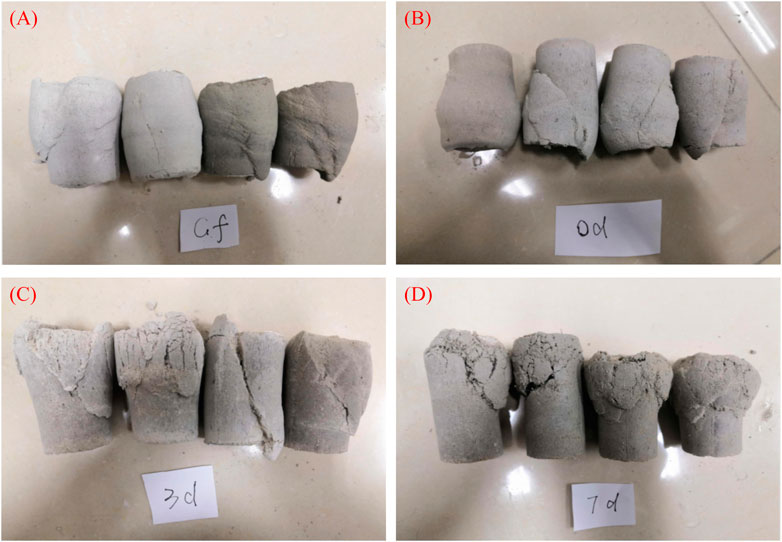
FIGURE 11. Damage patterns of specimens at different curing ages. (A) Pure tailings, (B) 0 days specimen, (C) 3 days specimen, and (D) 7 days specimen.
4.3 Shear strength c and φ
Since the stress-strain curve of concentrated full tailings under low confining pressure is a strain softening type, the peak point of each curve is taken as the failure point. Therefore, the peak value of (σ1−σ3) is taken as the diameter of the Mohr stress circle, and the value of (σ1+σ3)/2 is taken as the centre of the circle to draw the stress circles under different confining pressures. The common tangent of the stress circles is taken as the tanφ value, and the intercept between the common tangent and the σ-axis is taken as the c value. That is, the damage envelope is approximated into a straight line, which can be expressed as follows:
Figures 12–15 show the shear strengths of pure tailings and concentrated full tailings at different ages. It can be seen from the figures that the strength envelope of mohr-stress circle under different confining pressures is basically liner, indicating that under low confining pressures, the shear strength of different curing-age concentrated full tailings presents a linear increase trend with the increase of vertical stress.
Based on the test results, the concentrated full tailings, which were added cementitious material, have an overall greater shear strength than that of the pure tailings. The test results are summarized in Table 2 and Figure 16, it can be derived that, with the extension of the curing age, the cohesion increased first and then decreased. In addition, the internal friction angle (IFA) grew first and then stabilized. Concentrated full tailings exhibited a 70.7% higher cohesion and a 10% larger IFA than that of pure full tailings after being cured for 0 days. In comparison to those cured for 0 days, the concentrated full tailings cured for 3 days and 7 days exhibited 170.2% and 149.4% higher cohesion, respectively, and a 12.1% large IFA in both cases. This means, as the curing age lengthens, the degree of hydration grows, and the cohesion increases. When the age reaches a certain value, the shrinkage deformation creates micro-cracks inside the specimen (Yu et al., 2022). This is the reason for the reduction of cohesion.
The quantitative comparison results are calculated by the following equation:
Where,
5 Conclusion
This study carried out a triaxial shear test on pure tailings and concentrated full tailings cured at different ages to investigate how the curing agent affects the shear strength of tailings and how the curing age influences the shear strength. The main conclusions are as follows.
(1) Concentrated full tailings exhibited a 70.7% higher cohesion and a 10% large IFA than that of pure full tailings after being cured for 0 days. In comparison to those cured for 0 days, the concentrated full tailings cured for 3 days and 7 days exhibited 170.2% and 149.4% higher cohesion, respectively, and a 12.1% large IFA in both cases.
(2) The shear failure pattern of the concentrated full tailings, which were added as curing agents, differs from that of pure tailings. The former tends to be brittle failure with a distinct shear zone, while the latter is a plastic failure without such a zone.
(3) With the extension of the curing age, the hydration tends to be sufficient, the brittle failure becomes more apparent, and the shear zone becomes clearer and tends to penetrate the specimen. However, the shear zone of the 7 days specimen is not apparent because the local strength is reduced by irregular internal microcracks due to the dry shrinkage deformation of the specimen. So the intermittent time between construction layers is suggested to be no longer than 3days, when intermittent time is longer than 7days, the shear strength will be reduced, which will not benefit for the overall stability of the concentrated full tailings structure, and other measurements need to be adopted to increase the shear strength of construction layers, such as the hydraulic roughing measurement.
The concentrated full tailings, which were added curing agents, have a much higher shear strength than that of the pure tailings. Even if no curing is implemented, the cohesion of concentrated full tailings is nearly twice that of the pure tailings. This suggests that the curing agents effectively work and stabilize the specimen structure. The test results show that the shear strength is positively correlated with the curing age in the early stage. After 3 days, the shear strength declines, which is related to the dry shrinkage deformation of cement and other factors. In actual construction, the specimens between construction layers are cured no longer than 3 days. In this manner, the concentrated full tailings can experience the strength growth due to hydration, while preventing the dry shrinkage deformation produced by the long drying time from weakening the structural strength.
Data availability statement
The original contributions presented in the study are included in the article/Supplementary Material, further inquiries can be directed to the corresponding authors.
Author contributions
Conceptualization, GM and WW; methodology, SW; validation, LG; formal analysis, SW; investigation, SW; resources, SW and YW; data curation, SW and YfC; writing—original draft preparation, SW; writing—review and editing, LG; visualization, SW; supervision, LG; project administration, GM; funding acquisition, GM and LG. All authors have read and agreed to the published version of the manuscript.
Funding
This research was funded by National Key Research and Development Plan of China (grant number 2018YFC0604605) and National Natural Science Foundation project of China (grant number 52274122); The APC was funded by Exploration Fund of BGRIMM Technology Group (grant number 02-2271).
Conflict of interest
SW, GM, WW, YW, YyC, LG, and YfC were employed by the company BGRIMM Technology Group.
Publisher’s note
All claims expressed in this article are solely those of the authors and do not necessarily represent those of their affiliated organizations, or those of the publisher, the editors and the reviewers. Any product that may be evaluated in this article, or claim that may be made by its manufacturer, is not guaranteed or endorsed by the publisher.
References
Agapito, L. A., and Bareither, C. A. (2018). Application of a one-dimensional large-strain consolidation model to a full-scale tailings storage facility. Miner. Eng. 119, 38–48. doi:10.1016/j.mineng.2018.01.013
Beier, N., Wilson, W., Dunmola, A., and Sego, D. (2013). Impact of flocculation-based dewatering on the shear strength of oil sands fine tailings. Can. Geotechnical J. 50, 1001–1007. doi:10.1139/cgj-2012-0262
Cui, Y., Mei, G., Chang, B., and Wang, Y. (2020). Mechanical properties of modified solidification tailings. Nonferrous Met. Sect. 072 (003), 93–98.
Dimitrova, R. S., and Yanful, E. K. (2012). Factors affecting the shear strength of mine tailings/clay mixtures with varying clay content and clay mineralogy. Eng. Geol. 125, 11–25. doi:10.1016/j.enggeo.2011.10.013
Dong, Q. (2017). Study of evolution and mechanism of the strength to sulfur content lead-zinc tailings cemented paste backfill. doctor's thesis. Fuxin City: Liaoning Technical University. (in Chinese).
Đurđevac Ignjatović, L., Krstić, V., Radonjanin, V., Jovanović, V., Malešev, M., Ignjatović, D., et al. (2022). Application of cement paste in mining works, environmental protection, and the sustainable development goals in the mining industry. Sustainability 14, 7902–7913. doi:10.3390/su14137902
Hou, C., Zhu, W., Yan, B., Guan, K., and Du, J. (2018). Influence of binder content on temperature and internal strain evolution of early age cemented tailings backfill. Constr. Build. Mater. 189, 585–593. doi:10.1016/j.conbuildmat.2018.09.032
Hou, Y., Cao, S., Ding, P., Zhang, X., and Han, D. (2019). Effect of freeze-thaw cycle on the intensity of consolidated full tailings. Metal. Mine 1, 34–39. (in Chinese).
Indraratna, B. (1994). Geotechnical characterization of blended coal tailings for construction and rehabilitation work. Q. J. Eng. Geol. Hydrogeology 27, 353–361. doi:10.1144/GSL.QJEGH.1994.027.P4.06
Islam, S. (2021). A study on the mechanical behaviour of three different fine-grained mine tailings. Riyadh City: journal of King Saud University-Engineering Sciences. doi:10.1016/j.jksues.2021.04.001
Jiang, H., Ren, L., Gu, X., Zheng, J., and Cui, L. (2022). Synergistic effect of activator nature and curing temperature on time-dependent rheological behavior of cemented paste backfill containing alkali-activated slag. Environ. Sci. Pollut. Res. 30, 12857–12871. doi:10.1007/s11356-022-23053-1
Jin, J., Qin, Z., Lü, X., Liu, T., Zhang, G., Shi, J., et al. (2022). Rheology control of self-consolidating cement-tailings grout for the feasible use in coal gangue-filled backfill. Constr. Build. Mater. 316, 125836. doi:10.1016/j.conbuildmat.2021.125836
Ke, X. (2016). Study on structure and properties of the consolidation mass of cemented tailings backfill. Doctor's thesis. Wuhan City: Wuhan University. (in Chinese).
Kiventerä, J., Piekkari, K., Isteri, V., Ohenoja, K., Tanskanen, P., and Illikainen, M. (2019). Solidification/stabilization of gold mine tailings using calcium sulfoaluminate-belite cement. J. Clean. Prod. 239, 118008. doi:10.1016/j.jclepro.2019.118008
Li, J., Ren, W., Zhang, A., Li, S., Tan, J., and Liu, H. (2023). Mechanical properties and microstructure analysis of cement mortar mixed with iron ore tailings. Buildings 13, 1–20. doi:10.3390/buildings13010149
Li, J. (2018). The experimental research of creep characteristic and long-term strength for cemented full tailings backfill. master's thesis. Nanchang City: Jiangxi University of Science and Technology. (in Chinese).
Li, K. (2019). “Study on mechanical and acoustic emission characteristics of uniaxial compression of cemented tailings backfill subjected to temperature,”. Master's Thesis (China: Wuhan University of Science and Technology).
Libos, I. L. S., and Cui, L. (2020). Effects of curing time, cement content, and saturation state on mode-I fracture toughness of cemented paste backfill. Eng. Fract. Mech. 235, 107174. doi:10.1016/j.engfracmech.2020.107174
Paiva, H., Yliniemi, J., Illikainen, M., Rocha, F., and Ferreira, V. (2019). Mine tailings geopolymers as a waste management solution for a more sustainable habitat. Sustainability 11, 995–1015. doi:10.3390/su11040995
Qin, J., Zheng, J., and Li, L. (2021). An analytical solution to estimate the settlement of tailings or backfill slurry by considering the sedimentation and consolidation. Int. J. Min. Sci. Technol. 31, 463–471. doi:10.1016/j.ijmst.2021.02.004
Roshani, A., Fall, M., and Kennedy, K. (2017). A column study of the hydro-mechanical behavior of mature fine tailings under atmospheric drying. Int. J. Min. Sci. Technol. 27, 203–209. doi:10.1016/j.ijmst.2017.01.023
Suazo, G., Fourie, A., Doherty, J., and Hasan, A. (2016). Effects of confining stress, density and initial static shear stress on the cyclic shear response of fine-grained unclassified tailings. Géotechnique 66, 401–412. doi:10.1680/jgeot.15.P.032
Wang, B., Gan, S., Dong, P., Wang, R., Li, Q., and Gao, L. (2021). Experimental study on consolidation characteristics of tailings cemented backfill in alpine regions. Chin. J. Nonferrous Metals 32, 2446–2457. (in Chinese).
Wang, S., Zhang, C., and Zhu, W. (2008), Influence of solidified tailing concentration on initial fluidity and strength. Metal. Mine 2, 136–145. (in Chinese).
Wang, S., Zhang, C., and Zhu, W. (2009), Influence of solidifying additive on the initial fluidity and strength of solidified tailings. Metal. Mine 2, 30–33.
Xiu, Z., Wang, S., Ji, Y., Wang, F., and Ren, F. (2022). Experimental study on the triaxial mechanical behaviors of the cemented paste backfill: Effect of curing time, drainage conditions and curing temperature. J. Environ. Manag. 301, 113828. doi:10.1016/j.jenvman.2021.113828
Xiu, Z., Wang, S., Ji, Y., Wang, F., and Wang, P. (2021). An analytical model for the triaxial compressive Stress-strain relationships of Cemented Pasted Backfill (CPB) with different curing time. Constr. Build. Mater. 313, 125554. doi:10.1016/j.conbuildmat.2021.125554
Xu, W., Li, Q., and Tian, M. (2019). Strength and deformation properties of polypropylene fiber-reinforced cemented tailings backfill. Chin. J. Eng. 41, 1618–1626. (in Chinese). doi:10.13374/j.issn2095-9389.2018.12.14.002
Yan, B., Han, L., Xiao, H., Zhang, J., Huang, J., Hu, W., et al. (2019). Rapid dewatering and consolidation of concentrated colloidal suspensions: Mature fine tailings via self-healing composite hydrogel. acs Appl. Mater. interfaces 11 (24), 21610–21618. doi:10.1021/acsami.9b05692
Yao, Y., Van Tol, A. F., and Van Paassen, L. A. (2012). “The effect of flocculant on the geotechnical properties of mature fine tailings: An experimental study,” in IOSTC 2012: Proceedings of the 3rd International Oil Sands Tallings Conference, Edmonton, Canada (Edmonton: university of Alberta).
Yilmaz, E., Belem, T., Bussière, B., Mbonimpa, M., and Benzaazoua, M. (2015). Curing time effect on consolidation behaviour of cemented paste backfill containing different cement types and contents. Constr. Build. Mater. 75, 99–111. doi:10.1016/j.conbuildmat.2014.11.008
Yin, S., Liu, J., Shao, Y., Zhang, H., Armelle, B., and Kou, Y. (2020). Influence rule of early compressive strength and solidification mechanism of full tailings paste with coarse aggregate. J. Central South Univ. Sci. Technol. 51, 11–17. (in Chinese).
Yu, X., Song, W., Tan, Y., Kemeny, J., and Wang, J. (2022), Energy dissipation and 3d fracturing of Backfill-encased-rock under triaxial compression. Constr. Build. Mater. 341, 127877. doi:10.1016/j.conbuildmat.2022.127877
Zhao, S., Su, D., Zhang, Y., and Wu, W. (2016). Study on creep test of cemented tailings backfill and statistical damage model. Metal. Mine 5, 26–30. (in Chinese).
Keywords: concentrated full tailings, triaxial shear test, curing age, shear strength, stress-strain curve
Citation: Wang S, Mei G, Wang W, Wang Y, Cui Y, Guo L and Chu Y (2023) Experimental study of triaxial shear features of concentrated full tailings considering the effect of curing age. Front. Earth Sci. 11:1158715. doi: 10.3389/feart.2023.1158715
Received: 04 February 2023; Accepted: 10 April 2023;
Published: 21 April 2023.
Edited by:
Xing Wang, Guangzhou University, ChinaReviewed by:
Yi Shan, Guangzhou University, ChinaShuai Cao, University of Science and Technology Beijing, China
Yue Qin, Wuhan University of Technology, China
Copyright © 2023 Wang, Mei, Wang, Wang, Cui, Guo and Chu. This is an open-access article distributed under the terms of the Creative Commons Attribution License (CC BY). The use, distribution or reproduction in other forums is permitted, provided the original author(s) and the copyright owner(s) are credited and that the original publication in this journal is cited, in accordance with accepted academic practice. No use, distribution or reproduction is permitted which does not comply with these terms.
*Correspondence: Guodong Mei, meiguodong@bgrimm.com; Lijie Guo, guolijie@bgrimm.com