- 1School of Engineering and Technology, China University of Geosciences Beijing, Beijing, China
- 2College of Urban and Environmental Science, Peking University, Beijing, China
Based on field investigation, optically stimulated luminescence (OSL) dating and sedimentary analyses, this research shows that the Yinduba paleolandslide-dammed lake (PDL) was formed by a catastrophic rock avalanche damming Jinsha River before 74 ka ago. According to the blocked ancient riverbed, the lake depth was determined about 110 m and the corresponding lake length was about 22 km when the lake level reached the peak. Geomorphologic features of lacustrine terraces at Yinduba and river terraces on the residual dam body and OSL dating results indicate that Yinduba PDL is a dammed lake with multi-stage outbursts. Following the peak, due to the multi-stage breaching of the dam body, the lake level experienced two major drops until the dam breached entirely after 36 ka. The large dam size, special sedimentary structure, and semi-arid climate contribute to the long life of Yinduba PDL, which existed for approximately 38,000 years from its formation to its extinction. This study supports the possibility of a large landslide-dammed lake having the life span of up to tens of millennia, and provides new evidence regarding the evolutionary history of a dammed lake.
1 Introduction
Natural dams can be created by a variety of processes, including volcanism (Ely et al., 2012), glaciation (Scherler et al., 2014), landslides (Costa and Schuster, 1988), etc. These dams are widely distributed all over the world (Costa and Schuster, 1991; Korup, 2002), especially in mountainous terrain. Mountainous terrain host the key prerequisites for the formation of these natural dams (Korup and Tweed, 2007). Of the dams formed by landslides blocking the river, most of them (about 80%) fail within 1 year of formation (Costa and Schuster, 1988; Peng and Zhang, 2011), and only a small number of them have the ability to survive for several decades to several centuries (e.g., Chen et al., 2018) or several millennia (e.g., Liu et al., 2018) or even tens of millennia (e.g., Korup et al., 2006; Chen et al., 2016). Evolution history of these long life-span landslide-dammed lakes, including their formation, duration and drainage, can provide valuable insights into the study of geomorphological processes (Korup, 2002), fluvial evolution (Ouimet et al., 2007; Guo, 2017), tectonic activities (Wang et al., 2014; Guo et al., 2016), and sedimentary paleoenvironments (Trauth and Strecker, 1999; Zhang et al., 2011). For instance, landslide-dammed lakes impose temporary local base levels of erosion that may survive for millennia, thus retarding fluvial incision and overall landscape denudation (Fan et al., 2020).
There are three typical evolutionary processes of PDLs, namely, intermittent decline of lake level (e.g., Wu et al., 2019; Xu et al., 2020), long-term stability of lake level (e.g., Wang et al., 2019; Wang et al., 2021), and continuous decline of lake level (e.g., Wassmer et al., 2004). The intermittent decline of the lake level is caused by the multi-period breaching of the PDLs. In addition to the fluvio-lacustrine terraces (Chen et al., 2016), lacustrine terraces are also the important geomorphological evidence indicating the intermittent decline of the lake level. The formation of lacustrine terraces is only related to the lacustrine deposition, without the intervention of river and its tributaries. When the lake level declines due to the dam breach, the lacustrine sediments previously deposited in the lakeshore would be separated from the lake system and strand above the lake level as terraces. Therefore, lacustrine terraces could not only indicate the existence of PDLs (e.g., Zhang et al., 2009; Guerrero et al., 2018), but also record the lake-level fluctuations of PDLs (e.g., Ocakoğlu et al., 2013; Sharafi et al., 2019). Lacustrine terraces are often found in lakes, such as Alag Lake (Zhao and Li, 2006), Yang Lake (Liu et al., 2003; Zhao and Li, 2006), Nam Co. (Zhao et al., 2002; 2003), Bankog Co. (Zhao et al., 2011) and Peiku Co. (Kong et al., 2011), indicating the history of paleoshoreline changes and lake-level fluctuations. However, the well-preserved lacustrine terraces are rarely found in PDLs.
Yinduba PDL is located in the upper Jinsha River, at the southeastern margin of the Tibetan Plateau (Figures 1, 2). Two-level well-preserved lacustrine terraces were found in Yinduba PDL and are the subject of the present study. Although there have been some studies on Yinduba PDL, there are still some problems. For instance, Liang et al. (2022) estimated the lifetime of Yinduba PDL was ∼20.86 ka by using the linear fitting interpolation analysis of OSL dating of the residual lacustrine section. Again for instance, taking the modern riverbed as the blocked ancient riverbed, Chen and Li (2016) concluded that the lake length of Yinduba PDL is about 77 km. Aiming at these existing problems, i.e., unreliable lifetime and lake length of Yinduba PDL, this paper will: 1) use OSL dating of whole lacustrine section to provide age control of the duration of Yinduba PDL; 2) determine the elevation of the blocked old riverbed and the corresponding lake length at the highest lake level; 3) reconstruct the evolutionary history of Yinduba PDL based on lacustrine terraces and OSL ages; 4) discuss the influence of PDLs on fluvial terraces in the upper Jinsha River.
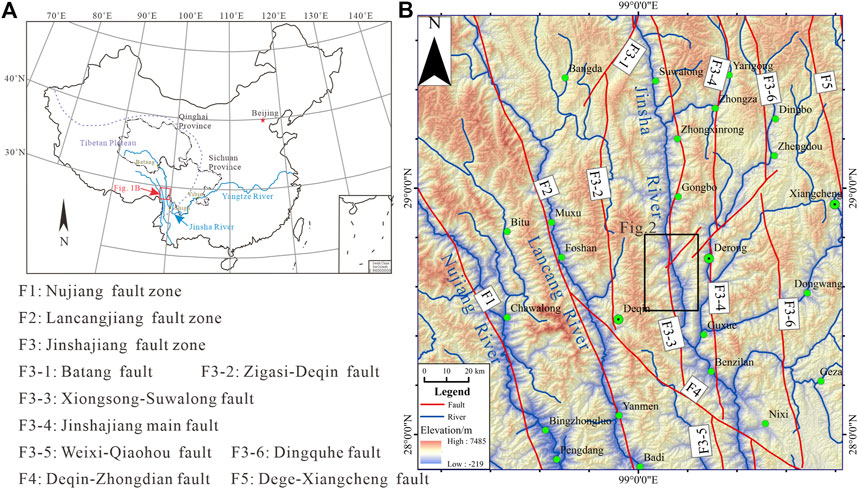
FIGURE 1. (A) Location of the study region. (B) Digital elevation model of the studied region at the Southeastern margin of the Tibetan Plateau. The black rectangle represents the location of Yinduba PDL (See Figure 2 for details).
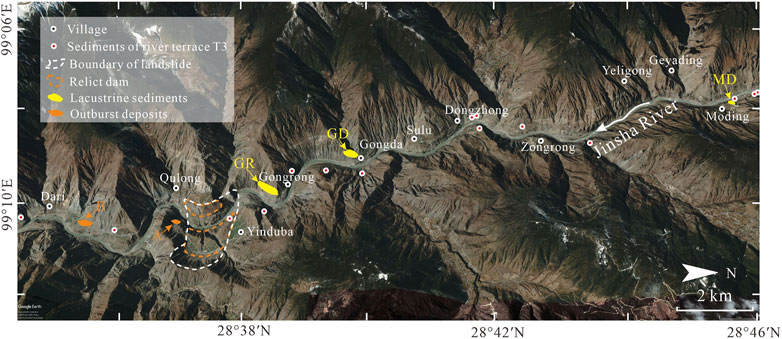
FIGURE 2. Google Earth image of Yinduba PDL, showing the distribution of lacustrine sediments, relict dam, Yinduba paleolandslide and outburst deposits (note the location in Figure 1B).
2 Geological background
The Jinsha River is located in the upper reaches of the Yangtze River. It begins at the mouth of the Batang River in Qinghai Province, and ends in Yibin, Sichuan Province, with a total length of more than 3,300 km (Figure 1A). The study area is located in the Suwalong-Benzilan segment of the upper Jinsha River (Figure 1B). This segment is characterized by typical canyon landforms, alternating between wide and narrow valleys. The narrow valleys are approximately 100–200 m wide, with the width of the wide ones being in the range of 200–400 m. Six-level fluvial terraces (T6, T5, T4, T3, T2, and T1) are intermittently distributed along both banks of the Jinsha River, with relative elevations of 300–350 m, 200–220 m, 150–180 m, 120–135 m, 60–90 m and 20–30 m above present river level (aprl), respectively (Shen, 1965). The slopes of the river valley are steep, most of them being greater than 30°.
Due to the foehn effect, the study area has a dry-hot valley climate, with evident vertical climate characteristics (Chen and Xu, 2013). In addition, the distribution of rainfall during a year is highly uneven, with rainfall concentrated in June to October, accounting for 78.16% of the total annual rainfall (Wei et al., 2019).
Metamorphic rocks are the most widely distributed in the study area, mainly consisting of slate, phyllite, schist and quartzite, with a small amount of marble. The exposure areas of magmatic and sedimentary rocks are relatively small. The magmatic rocks are mostly medium-basic in lithology, such as andesite, and the sedimentary rocks are mainly limestone and clastic rocks.
As detailed in Figure 1B, the main active fault in the upper Jinsha River is the Jinshajiang fault zone (F3). This fault zone is a complex structural zone trending north-south, with a total length of about 1,200 km and a width of approximately 50–70 km (Xia and Zhu, 2020). The Jinshajiang fault zone is mainly composed of the West Boundary Fault (F3-2), West Branch Fault (F3-3), Jinsha River Main Fault (F3-4), East Boundary Fault (F3-6) and Batang Fault (F3-1) (Figure 1B). The Batang Fault (F3-1) and Deqin-Zhongdian Fault (F4) divide the Jinshajiang fault zone into three segments: northern, central and southern (Figure 1B). The Weixi-Qiaohou Fault (F3-5) is in the southern segment. Seismic activities occur frequently in the Jinshajiang fault zone (Wu and Cai, 1992; Zhou et al., 2005).
3 Methodology
3.1 Field work
In the field, the location and elevation of all outcrops were measured by a high-resolution (10 cm) Global Navigation Satellite System (GNSS) handheld devices (Trimble 7x). The geomorphologic features of relict landslide dam, lacustrine sediments and outburst deposits, including morphological characteristics and sedimentary characteristics, were recorded in detail using a laser rangefinder, tape, compass, and camera. Then, the sand layers suitable for OSL dating were identified and sampled. In addition, in order to determine the elevation of the blocked ancient riverbed, the laser rangefinder was also employed to measure the elevations above present river level of relict landslide dam, lacustrine sediments and fluvial terrace deposits on both sides of the Jinsha River in the study area. Finally, based on geomorphologic features, OSL dating results and the location of blocked ancient riverbed, the duration, lake length and evolution process of the Yinduba PDL were inferred.
3.2 OSL dating
In order to determine the time at which the lake level declines during different evolutional stages, six samples were collected for OSL dating. The section of lacustrine sediments Gongrong (GR) is large and complete, and the two lacustrine terraces in Gongrong can fully reflect the evolution process of Yinduba PDL. So it is the main sampling section, with five samples from lacustrine sediments GR (Figure 4C). Outburst deposits are thin and small in scale, with one sample from the outburst deposits (Figure 5C). There are no sand layers suitable for OSL sampling in the residual dam.
The six OSL samples were taken by hammering a steel tube into the fresh profile. The tubes were sealed at both ends using aluminum foil and packed into black plastic bags, so as to protect the samples from sunlight exposure and water loss. The parts of the sample at the ends of the tube were extracted to perform water content and dose rate measurements. The remainder was used to extract quartz grains for equivalent dose (De) measurements. The remainder of each sample was first dry sieved to eliminate >300 μm fraction, followed by pretreatment with 30% HCl and 30% H2O2 to remove carbonates and organics, respectively. Then 90–125 μm grains separated by second dry sieving were etched by 40% HF to remove the feldspar, followed by 10% HCl to remove the fluoride. Finally, infrared-stimulated luminescence (IRSL) was measured to ascertain the purity of the quartz grains.
OSL dating measurements were performed by using an automated Risø TL/OSL-DA-20-C/D reader at the National Institute of Natural Hazards, Ministry of Emergency Management of China. The De for quartz of six samples were determined by using the single-aliquot regeneration (SAR) protocol (Murray and Wintle, 2000; 2003). The detailed procedure of De measurement is shown in Table 1. The environmental dose rate (shown in Table 2) was determined with various techniques at the Chinese Atomic Energy Institute in Beijing. The concentrations of U and Th were measured by NexION300D plasma mass spectrometer and the K content was measured using Z-2000 graphite furnace atomic absorption analyzer. Only sample YD-6 had a water content of 5%, while the other samples were drier, with water content of less than 1%. Considering that the water content of the samples in the historical period was higher than that at present, the water contents of all samples were taken as 5% ± 5%.
4 Geomorphologic features
Barrier dam, upstream lacustrine sediments and downstream outburst deposits constitute a trinity of sedimentary assemblages, which is the most direct evidence for identifying paleo-dammed lakes (Chen and Cui, 2015). In Yinduba and its upstream and downstream, relict paleolandslide dam, lacustrine sediments and outburst deposits were found (Figure 2).
4.1 Relict landslide dam
During a field investigation, a large paleolandslide dam was observed at Yinduba. The residual landslide dam, mainly located on the left bank of the Jinsha River (Figure 3A), is approximately 1,600 m long and 1,000 m wide, and has a dam-front thickness of 150 m. The residual landslide dam overlays the slope of the bedrock on the opposite bank (Figure 3A), with the top elevation of ∼263 m aprl. The landslide dam is mainly composed of basalt and marble. The deposits are poorly sorted and highly fragmented (Figure 3C), with the front edge of the deposits presenting the characteristics of fluidized structure (Figure 3B). The horizontal distance between the lowest point and rear edge of the landslide is approximately 2.6 km (Figure 3A), with the corresponding ΔH/L being about 0.24. The volume of this paleolandslide is estimated to 3 × 108 m3. Therefore, the Yinduba paleolandslide is considered to be a long runout rock avalanche.
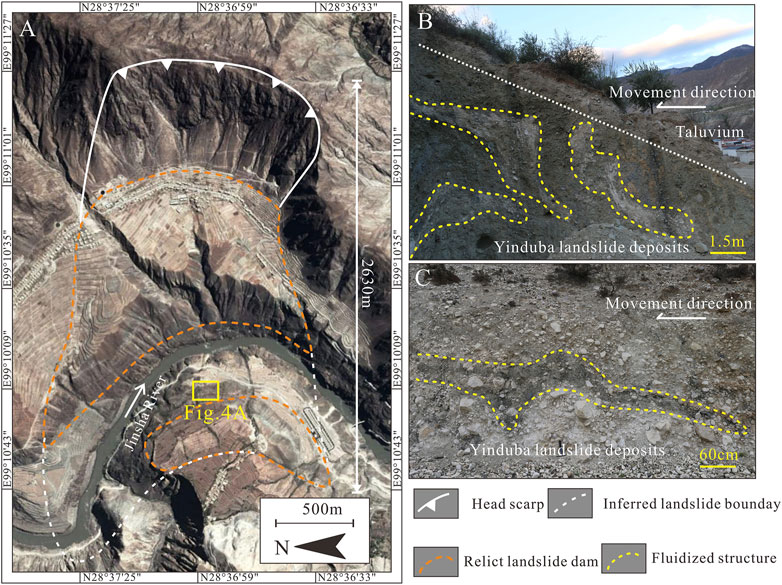
FIGURE 3. (A) Remote sensing image of the Yinduba landslide with relict landslide dam and inferred landslide boundary. (B) Fluidized structure at the front of the Yinduba landslide deposits. (C) Fragmented facies and fluidized structure of the Yinduba landslide deposits.
The remnants of two river terraces (RT2 and RT1, Figure 4A), with the respective relative heights of 200 m and 140 m aprl, were observed to occur inset onto the residual landslide dam located on the right bank of the river. The treads of the terraces that are approximately semicircular are gently inclined to river, with broad tread providing sufficient space for the preservation of river sediments. The rounded gravels are unevenly distributed on the treads, with grain size ranging from 5 to 30 cm, and a maximum particle size of up to 70 cm. These two terrace remnants were created during and after flood events and clearly indicate periodical downcutting of the landslide dam. Similar terraces are also present in landslide-dammed lakes in White Rock Canyon, New Mexico (Reneau and Dethier, 1996) and lake Gohna Tal in Birahi Ganga Valley, India (Weidinger, 2011), which record pauses in the decline of the lake level.
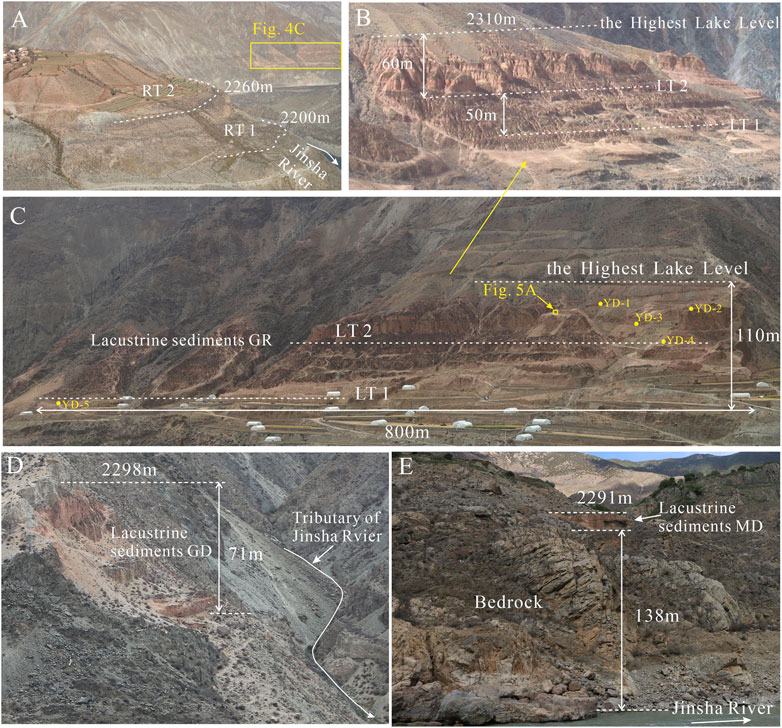
FIGURE 4. (A) Two river terraces RT 2 and RT 1 formed by fluvial erosion on dam (RT: river terrace, note the location in Figure 3A). (B) The close-up of two lacustrine terraces in Figure 4C, showing the height difference of terraces (LT: lacustrine terrace). (C) Overall view of sediments GR, showing the locations of Figure 5A and OSL samples YD-1 to YD-5. (D) Overall view of sediments GD. (E) Overall view of sediments MD.
4.2 Lacustrine sediments
Most of the lacustrine sediments of Yinduba PDL have undergone denudation, with the remaining parts only distributed in three locations, namely, Gongrong, Gongda and Moding, respectively denoted as GR, GD, and MD (Figure 2).
Sediments GR are well preserved on the convex bank of the river (Figure 2), with a thickness of about 110 m and lateral extent of up to 800 m (Figure 4C). Sediments GR consist of massive to horizontally laminated silt-rich reddish sediments (Figure 5A) and crop out in the form of terraces on valley slopes in Gongrong. Two lacustrine terraces (LT2 and LT1) can be clearly observed in the field (Figure 4B), with the relative heights of 190 m and 140 m aprl, respectively. The treads of the terraces that are benches are gently inclined to river, and terrace slopes of LT2 and LT1 are relatively smooth. Lacustrine terraces are formed during periodical lake-level declines of PDLs and thus can give evidence of several outbreaks of PDLs (Weidinger, 2011) and record the history of lake-level fluctuations (Ocakoğlu et al., 2013; Sharafi et al., 2019).
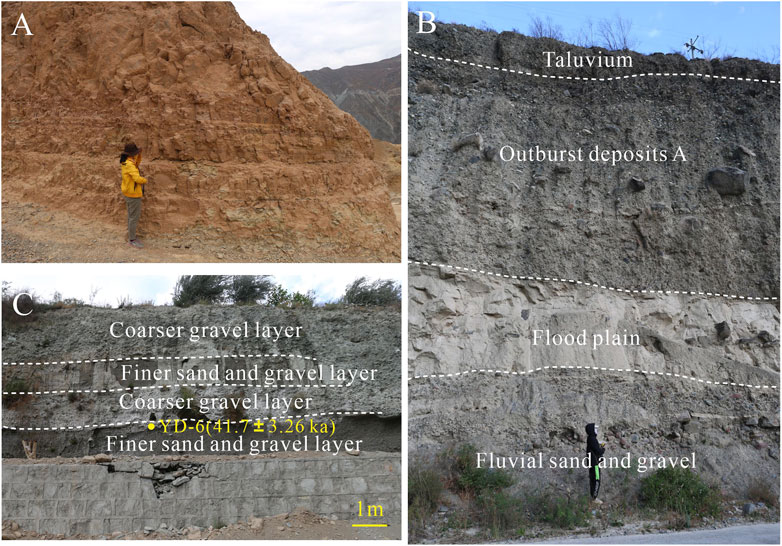
FIGURE 5. (A) Detail of inset in Figure 4C, showing horizontal lamination or massive structure in lacustrine sediments. (B) Outburst deposits A has erosional contact with underlying fluvial deposits composed of upper floodplain sediments and lower channel sediments. (C) Rhythmite interbedded structure in outburst deposits B composed of coarser gravel layer and finer sand and gravel layer, showing the location of OSL sample YD-6.
Sediments GD are located on the right bank of the tributary that used to join the Jinsha River (Figure 4D), with thinner thickness and smaller scale than sediments GR. Sediments GD consist of horizontally laminated to thinly bedded reddish silt and clayey silt, and are often interbedded with coarse sand and gravels that represents bed load of the tributary.
Sediments MD are minimal in range and thickness and located on the left bank slope of Jinsha River, with a relative height of 138 m aprl (Figure 4E). Sediments MD are composed of coarse-grained reddish sand and gravels that represent bed load of the river in the proximal part of Yinduba PDL.
4.3 Outburst deposits
In the field, two sections of outburst deposits were found at 0.5 and 5 km downstream of the dam, respectively marked as A and B (Figure 2). Outburst deposits A is 17 m thick, and laterally extends 100 m. The deposits A consists of diamicton, ranging from silt, sand to gravels. The coarse-grained gravels in deposits A are arranged linearly, thus indicating the directional movement of the deposits during the transportation process (Figure 5B). Outburst deposits B is 7 m thick and 80 m long, and is composed of cyclical superposition of coarser gravel layer and finer gravel and sand layer (Figure 5C). This special rhythmite-interbedded structure also appears in the outburst deposits of other PDLs (e.g., Chen and Cui, 2015; Chen et al., 2018; Ma et al., 2018).
5 OSL dating results
Dose rates, De values and OSL ages of the six OSL samples are shown in Table 2. The De distributions of all samples are displayed using histograms (Figure 6). The distribution of De values of sample YD-6 is relatively scattered (Figure 6), which may be caused by the rapid accumulation of outburst deposits. Therefore, the actual age of YD-6 should be smaller than the dated age (41.7 ± 3.26 ka) due to partial bleaching. With the exception of sample YD-6, the De values of all discs for every sample are normally distributed (Figure 6), meaning that the mineral grains were fully bleached prior to deposition. Therefore, the average of all measured discs of each sample except YD-6 was used as De value for the age calculation.
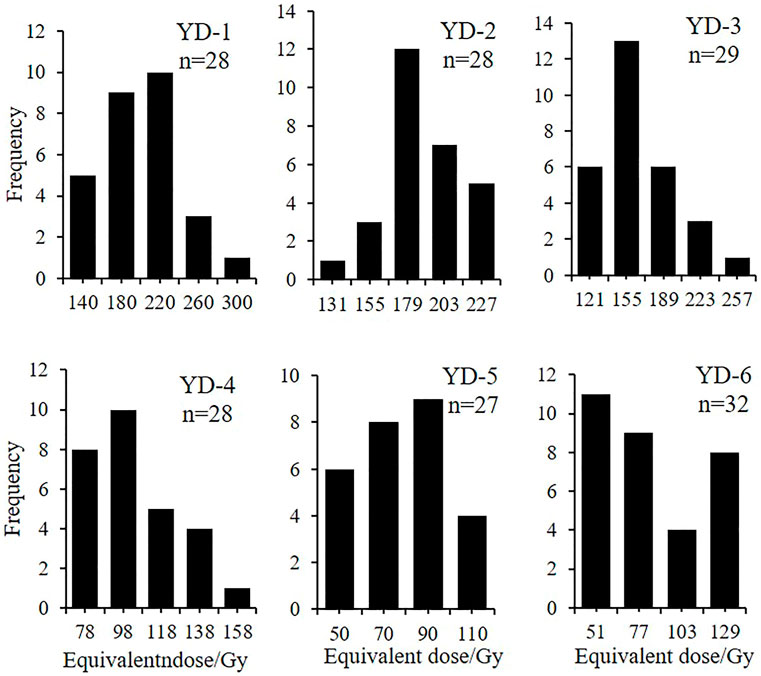
FIGURE 6. Histograms of De distributions for all luminescence dating samples. With the exception of sample YD-6, the De values of each sample are normally distributed.
The saturation dose limit for quartz is ∼300 Gy (Fan, 2010). A review of OSL dating practices by Murray and Olley. (2002) shows that OSL dating of quartz grains is capable of providing reliable ages ranging from the last century to 350 ka, by comparison with independent ages derived from various types of sediments. The De value of sample YD-1 was lower than 300 Gy, and the test dose corrected natural OSL signal level (Lx/Tx) was much lower than saturation level (Figure 7B). The OSL signals of sample YD-1 decrease very quickly during the first 2 s of stimulation (Figure 7A), indicating that the OSL signal is dominated by the fast component. And the recycling ratio of the sample YD-1 is close to 1 (Figure 7C). Therefore, the OSL age of YD-1 was reliable, without being underestimated.
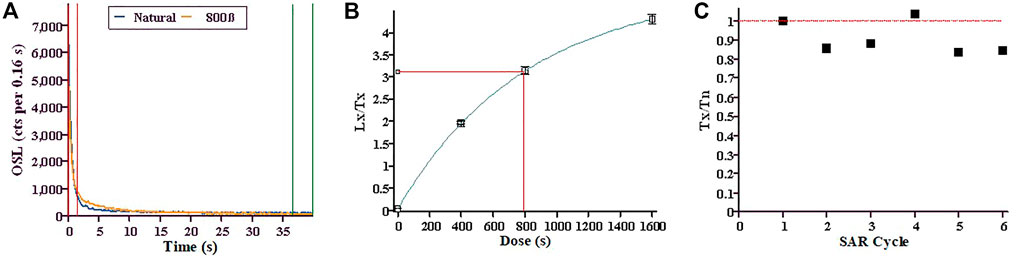
FIGURE 7. OSL decay curve (A), growth curve (B) and recycling ratio (C) of sample YD-1. (A) The OSL signal was quickly bleached to the measurement background within about 1 s, suggesting that the sample is dominated by the fast component. (B) The test dose corrected natural OSL signal level (Lx/Tx) was much lower than the saturation level. (C) The recycling ratio of the sample is in the range of 1.02 ± 0.04.
6 Discussion
6.1 Duration of the Yinduba PDL
The top and bottom ages of lacustrine sediments GR are 73.96 ± 4.26 ka and 35.95 ± 2.28 ka, respectively (Figure 4C; Table 2), and there is no interlayer of fluvial sediments appearing in the 110 m thick lacustrine sediments. Therefore, the dam was not be breached completely between 74–36 ka and the river system was not re-established during this period. That is, the duration of up to 38,000 years of Yinduba PDL was not caused by repetitive blockages. Due to being partly bleached, the actual age of sample YD-6 is smaller than 41.7 ± 3.26 ka, thus suggesting that outburst deposits B was likely deposited after 36 ka B. P. After the last dam breaching, the dammed lake disappeared, and the fluvial system was re-established. Therefore, the Yinduba PDL was formed at the late stage of Late Pleistocene (ca. 74 ka) and disappeared after ca. 36 ka B.P., with longevity of up to 38,000 years.
The previous studies illustrated that about 80% landslide dams failed within the first year after formation (Costa and Schuster, 1988; Peng and Zhang, 2011). However, a landslide dam is statistically likely to survive for several centuries or several millennia or even longer (e.g., Korup et al., 2006; Chen et al., 2016). For instance, Phoksundo Lake, originating from a river-damming rockslide in western Nepal, still exists after 30–40 ka (Yagi, 1997). For another example, a dammed lake was formed by a rockslide during 56.36 ± 6.98 and 55.84 ± 5.9 ka B. P. in Kali Gandhaki valley, Nepal and disappeared within late to postglacial times, with duration of approximate 40 ka (Baade et al., 1998).
Long-lived Yinduba PDL may result from the favourable combination of several factors such as large dam size, special sedimentary structure, semi-arid climate in headwaters.
1. Large dam size. The Yinduba rock avalache is estimated to be 1,700 m long and 2,200 m wide, with the average thickness of 100 m. The volume of deposit is ca. 3 × 108 m3. Due to the extraordinarily large volume of Yinduba rock avalanche, the natural dam existed for relatively long time.
2. Special sedimentary structure. Rock avalanche deposits can be simplified as three distinct facies: a surface and near surface carapace facies, the main interior body facies, and a basal facies (Dunning and Armitage, 2011). The carapace facies is of critical importance to rock-avalanche dam stability because it is the material forming the dam crest (Dunning and Armitage, 2011). The clast-supported carapace facies of large angular rock fragments armors the dam surface, adds roughness, dissipates stream energy, and inhibits erosion of the dam during even the highest flows (Ouimet et al., 2007). In addition, flowing and seeping water can stabilize dam by depositing sediment between large gravels. Similar cases are observed in dams formed by Bawa La rock avalanche in the high Himalayan Crystalline Zone and Cui Hua rock avalanche in the Qin Ling mountains of China, respectively (Weidinger, 2011). The body facies, forming the bulk of most rock avalanche deposits, is composed of compact diamicton (Dunning et al., 2005). The diamicton will be further compacted and cemented under the weight of the overlying sediments. Therefore, the dam increased its stability over time.
3. Semi-arid climate in headwaters. The climate is an important factor for the stability and life span of these natural rock blockages. Semi-arid to arid climate seem to offer a high preservation potential for PDLs, which is reflected in similar studies (e.g., Wayne, 1999). For instance, due to low rate of sedimentation into the reservoir and low rates of erosion caused by dry weather conditions, a post-glacial dammed lake, formed by rock avalanche blocking tributary of Tsarap Chu in Zanskar, still exists (Weidinger, 2011). The timing and pattern of lake-level changes of 48 lakes on the Tibetan Plateau reveal a long-term trend of relative lake-level fall from at least MIS5, which is caused by a sustained drying since the Last Interglacial (Yu et al., 2019). Additionally, the Yinduba PDL mainly survived during MIS4, in which the temperature dropped and the rainfall decreased sharply. Therefore, the rate of sedimentation into the Yinduba PDL is low during duration, thereby extending Yinduba PDL’s life span.
6.2 Elevation of the blocked riverbed and the maximum lake length of the Yinduba PDL
Usually, the current riverbed can be used as the blocked old riverbed to estimate the size of lake, because the most PDLs were formed in the Holocene (e.g., Chen et al., 2013; Wang et al., 2014; Chen et al., 2018; Liu et al., 2018) and the topography of the ancient channel has not changed much. However, Yinduba PDL was formed at the late stage of Late Pleistocene (ca. 74 ka), and the bottom of the lacustrine sediments GR, GD and MD are all more than 100 m above the present river level. If the size of the Yinduba PDL is estimated by using the current riverbed as the blocked riverbed, the estimated size is much higher than the actual size. Therefore, the height of the blocked riverbed should be determined based on the evolution history of Jinsha River.
Terraces T2 and T3 in the upper Jinsha River are estimated to have respective formation ages of 15,670 ± 500 a and 149.65 ± 11.97 ka (Zhang, 1993; Zhang et al., 1998). Based on the OSL dating results, it is inferred that the river was blocked during the river downcutting following the aggradation of terrace T3. The respective relative heights of the bottom of lacustrine sediments GR, GD, and MD are 120–140 m, 165 m and 138 m aprl. Because lacustrine sediments GD is located on the slope of the tributary, the actual relative height of the paleo-lake bottom in the main channel should be lower than 165 m aprl. Therefore, the relative heights of bottom of the relict lacustrine sediments are all similar to that of terrace T3 in the upper Jinsha River (120–135 m aprl, Shen, 1965). Therefore, the surface of terrace T3 can be roughly regarded as the blocked old riverbed. Taking the elevation of the topmost lacustrine sediments as the highest lake level, the maximum lake length of the Yinduba PDL is determined to be approximately 22 km (Figure 8). This lake length is significantly smaller than that estimated by taking the modern riverbed as the blocked ancient riverbed (77 km, Chen and Li, 2016).
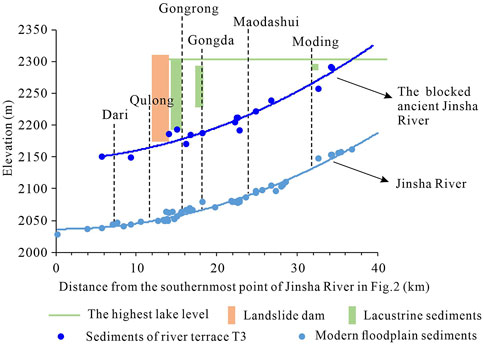
FIGURE 8. Longitudinal profile of the Yinduba PDL, showing the lake length at the highest lake level and the elevation of the blocked old riverbed.
6.3 Evolution process of the Yinduba PDL
In a closed lake basin, a lacustrine terrace represents a temporary stillstand of lake level as a result of a precipitation-evaporation balance (Yu et al., 2019). Similarly, in a dammed lake, the terraces represent a temporary stillstand of lake surface caused by inflow-outflow balance during the stable period of dam body. After the dam breaches partially, the lake level drops to a stable elevation, and a new lacustrine terrace will be formed, the elevation of which is lower than that of the previous one. When the lake stays at a stable level for a long time, the dam deposits will be eroded and transported by river. Meanwhile, the fluvial sediments are accumulated on the dam deposits, forming river terraces (e.g., Reneau and Dethier, 1996; Weidinger, 2011). Therefore, a series of such lacustrine terraces and river terraces can provide useful evidence for the evolution history of the dammed lakes.
Two-level lacustrine terraces at Gongrong, marked as LT2 and LT1 (Figures 4B, C), record two temporary stillstands during the lake level dropping. The lake-level fluctuations of the Yinduba PDL controlled the developments of lacustrine terraces at Gongrong (Figures 4B, C) and river terraces onto the residual landslide dam (Figure 4A). These terraces, in turn, record evolution history of Yinduba PDL. Therefore, the evolution process of the Yinduba PDL can be divided into four stages as follow (Figures 9, 10).
(1) Stage 1 (>74 ka): Before 74 ka ago, a rock avalanche occurred at Yinduba and blocked the Jinsha River, forming a dammed lake (Figure 9A). The lake water level behind the dam then gradually rose, eventually reaching its maximum elevation of 2,310 m (Figure 10).
(2) Stage 2 (∼74 ka to ∼47 ka): After the peak, the lake level dropped to 2,260 m due to the partial dam breach (Figure 10), and the topmost lacustrine sediments had already emerged from the lake surface (Figure 9B). Then, the lake level stabilized for long time, and the dam was eroded by the water flow, thereby forming terrace RT2 (Figure 9B).
(3) Stage 3 (∼47 ka to ∼36 ka): The dam breached again, and the lake level dropped by about 60 m. Then, the lake level remained stable at 2,200 m (Figure 10), with being close to the bottom of dammed lake. The lacustrine sediments previously deposited at lakeshore could remain stranded as terrace LT2 (Figure 9C). The dam was eroded by the water flow again, thereby forming terrace RT1 (Figure 9C).
(4) Stage 4 (<36 ka): Finally, the dam breached completely, and Yinduba PDL died out. The lacustrine sediments previously deposited at lakeshore emerged from water level, forming terrace LT1 (Figure 9D). Following this, the fluvial regime was re-established and Jinsha river continued to cutdown, thus forming the present landform (Figure 9D).
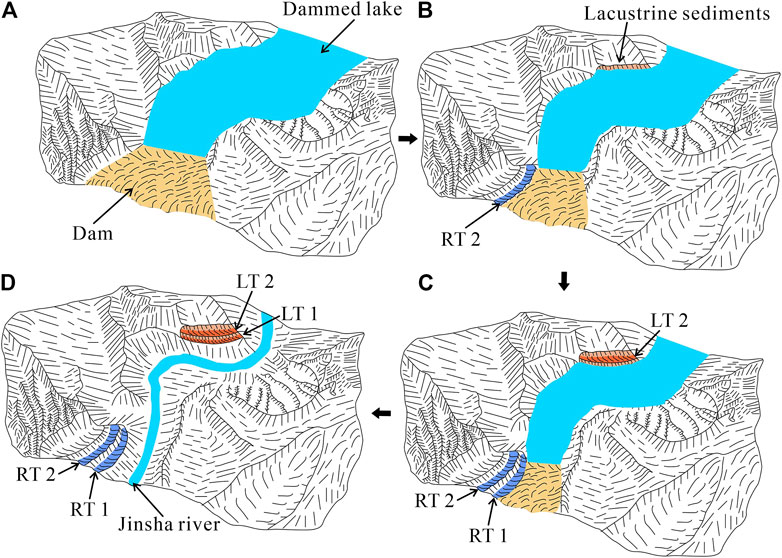
FIGURE 9. Schematic model of evolution process of Yinduba PDL. (A) The landslide blocked the Jinsha River, forming a dammed lake. (B) After the first outburst, topmost lacustrine sediments emerged from the lake surface, and terrace RT2 was formed by the water flow eroding the dam. (C) After the second outburst, terrace LT2 emerged from the lake surface, and terrace RT1 was formed by the water flow eroding the dam. (D) The dam breached completely and terrace LT1 emerged from the lake surface. Following this, Jinsha river continued to cutdown, thus forming the present landform.
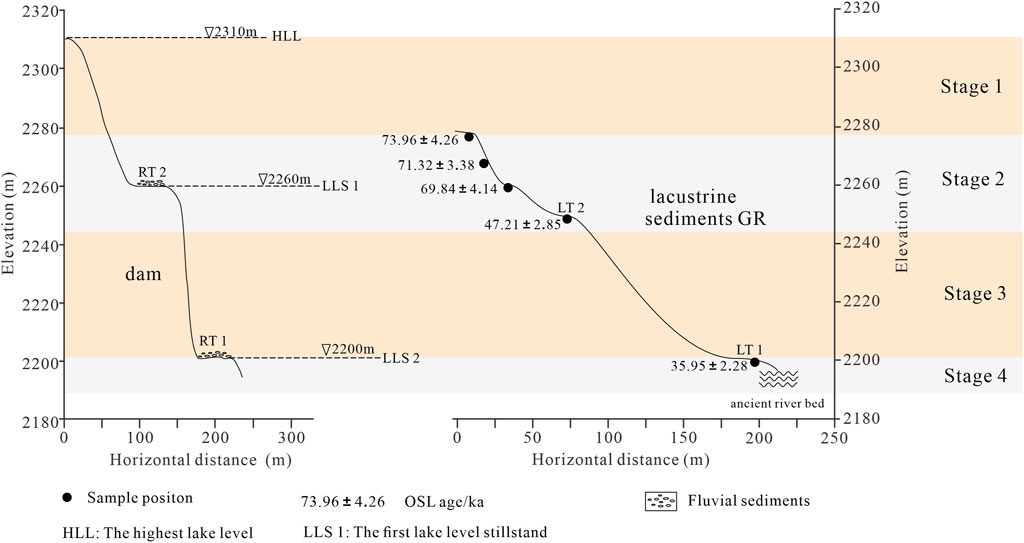
FIGURE 10. Comparison of river terraces and lacustrine terraces, showing the morphology of the terraces and history of lake-level fluctuations of the Yinduba PDL (the ancient river bed with a relative height of 120–140 m aprl).
6.4 Implications for the PDL effect on fluvial terraces
The terraces formed by the erosion base level changes caused by tectonic movements or climate changes are widely distributed and exhibit good continuity. Compared with the fluvial terraces in the middle and lower reaches of the Jinsha River, the terraces in the upper reaches are not well developed, with poor continuity. Therefore, more studies regarding the fluvial terraces of the Jinsha River are concentrated in the middle and lower reaches of the river (e.g., Su et al., 2019; Liu et al., 2020a; 2020b). One of the main reasons for the poor development of terraces in the upper reaches of the Jinsha River is the landslide dams and the dammed lakes behind the dams.
The upper reaches of the Jinsha River are prone to landslides blocking the river, forming dammed lakes. For instance, in the Zhongza-Batang segment of the upper Jinsha River, there are eight landslide dams formed during the period of about 122 AD-1100 AD (Chen et al., 2013). Generally, Landslide obstructions impose temporary local base levels of erosion and protect riverbed from the incision of the river and its tributaries, thereby interrupting development and continuity of fluvial terraces in the upper Jinsha River. The Yinduba PDL has existed for approximately 38,000 years from its formation to final extinction, and its dam belongs to long-term eroded dam according to the classification of landslide dam stability in Fan et al. (2020). During these 38,000 years, the lake surface served as the erosion base level and prevented the river from further downcutting, thereby influencing the development of fluvial terraces. In addition to the Yinduba PDL, there are many other PDLs present in the upper Jinsha River (e.g., Chen et al., 2013; Bao et al., 2020; Li et al., 2021), with life-spans ranging from centuries to millennia (Wang et al., 2014; Chen et al., 2018), or even longer (Zhang et al., 2011). The presence of these paleo-dammed lakes appeared to impede valley incision and interfered with river evolution.
There are fewer PDLs in the middle and lower Jinsha River, and their duration is relatively short, mostly in the range of centuries to millennia (Liu et al., 2018; Hu et al., 2021). Though there is a moraine-dammed lake with a life span of more than 10,000 years in the middle reaches of the Jinsha River, the average downcutting rate of the moraine dam is 7.6 m/ka (Kong et al., 2009), which is nearly four times faster than the Yinduba dam (1.7 m/ka, Figure 11). These fewer paleo-dammed lakes with short duration have less influence on the development and continuity of fluvial terraces in the middle and lower Jinsha River. Therefore, we suggest that paleo-dammed lakes should be taken into account when studying the factors that control the fluvial terraces in the upper Jinsha River.
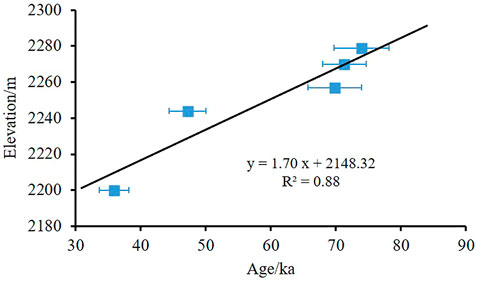
FIGURE 11. Plot of elevations of different samples vs. their corresponding OSL ages, indicating an average long-term landslide-dam incision rate of 1.70 m/ka.
7 Conclusion
In this study, six OSL dating results from lacustrine and outburst deposits, combined with sedimentological evidence and the geomorphic features of river terraces and lacustrine terraces, reveal the evolution processes of the Yinduba PDL at the late stage of Late Pleistocene. The main conclusions are as follows.
(1) Yinduba PDL was formed at the late stage of Late Pleistocene (ca. 74 ka) and disappeared after ca. 36 ka B. P., with longevity of up to 38,000 years. The large dam size, special sedimentary structure, and semi-arid climate contribute to the long life of Yinduba PDL.
(2) The estimation of the extent of Yinduba PDL is based on the determination of the blocked ancient riverbed. The blocked ancient riverbed was close to the surface of terrace T3 in height, with a relative elevation of 120–140 m aprl. When the lake level reached the peak, the lake depth was about 110 m and the corresponding lake length was about 22 km.
(3) Geomorphologic features of lacustrine terraces at Yinduba and river terraces on the residual dam body and OSL dating results indicate that Yinduba PDL is a dammed lake with multi-stage outbursts. Before 74 ka ago, a rock avalanche blocked the Jinsha River, forming Yinduba PDL. The lake water level behind the dam gradually rose, eventually reaching its peak of 2,310 m. After the peak, due to the multi-stage breaching of the dam body, the lake level dropped by 50 m and 60 m between ∼74 ka to ∼47 ka and ∼47 ka to ∼36 ka, respectively. After 36 ka, the dam breached completely and Yinduba PDL died out.
(4) There are many long-standing PDLs in the upper reaches of Jinsha River and they imposed a temporary local base level of erosion that survived from centuries to millennia, or even longer, thus affecting the development of fluvial terraces. Therefore, we suggest that paleo-dammed lakes should be taken into account when studying the factors that influence development characteristics and evolution of the fluvial terraces in the upper Jinsha River.
Data availability statement
The original contributions presented in the study are included in the article/supplementary material, further inquiries can be directed to the corresponding author.
Author contributions
HX: Field investigation, particle size measurement, analyzing results, writing original manuscript, review and editing. JC: Field investigation, review and editing, content layout, project administration. RC: Field investigation, drawing graphs, review and editing ZC: Field investigation, review and editing DM and LS: Field investigation, sampling, review and editing. All authors listed have made a substantial, direct, and intellectual contribution to the work and approved it for publication.
Funding
This work is supported by the Special Foundation for National Science and Technology Basic Research Program of China (grant number 2019FY101605), the National Key Research and Development Program of China (grant number 2018YFC1505003), and the National Natural Science Foundation of China (grant number 41571012).
Acknowledgments
We are greatly indebted to Linlin Li for his assistance in particle size measurement, and also to Fuchu Dai for his guidance in field work.
Conflict of interest
The authors declare that the research was conducted in the absence of any commercial or financial relationships that could be construed as a potential conflict of interest.
Publisher’s note
All claims expressed in this article are solely those of the authors and do not necessarily represent those of their affiliated organizations, or those of the publisher, the editors and the reviewers. Any product that may be evaluated in this article, or claim that may be made by its manufacturer, is not guaranteed or endorsed by the publisher.
References
Baade, J., Lang, A., Mäusbacher, R., and Wagner, G. (1998). “Quaternary lake deposits in the Thakkhola graben, Mustang, Nepal,” in 13th himalaya-karakoram-tibet international workshop (Mustang, Nepal: Spinger), 20–22.
Bao, Y., Zhai, S., Chen, J., Xu, P., Sun, X., Zhan, J., et al. (2020). The evolution of the Samaoding paleolandslide river blocking event at the upstream reaches of the Jinsha River, Tibetan Plateau. Geomorphology 351, 106970. doi:10.1016/j.geomorph.2019.106970
Chen, J., and Cui, Z. J. (2015). Discovery of outburst deposits induced by the Xuelongnang paleolandslide-dammed lake in the Upper Jinsha River, China and its environmental and hazard significance. Acta Sedimentol. Sin. 33, 275–284. doi:10.14027/j.cnki.cjxb.2015.02.007
Chen, J., Dai, F., Lv, T., and Cui, Z. (2013). Holocene landslide-dammed lake deposits in the Upper Jinsha River, SE Tibetan Plateau and their ages. Quat. Int. 298, 107–113. doi:10.1016/j.quaint.2012.09.018
Chen, J. P., and Li, H. Z. (2016). Genetic mechanism and disasters features of complicated structural rock mass along the rapidly uplift section at the upstream of Jinsha River. J. Jilin Univ. Earth Sci. Ed. 46, 1153–1167. doi:10.13278/j.cnki.jjuese.201604202
Chen, J., and Xu, P. (2013). Considerations on the scientific investigation of Jinsha River. J. Yangtze River Sci. Res. Inst. 30, 1–6. doi:10.3969/j.issn.1001-5485.2013.07.001
Chen, J., Zhou, W., Cui, Z., Li, W., Wu, S., and Ma, J. (2018). Formation process of a large paleolandslide-dammed lake at xuelongnang in the upper Jinsha River, SE Tibetan plateau: Constraints from OSL and 14C dating. Landslides 15, 2399–2412. doi:10.1007/s10346-018-1056-3
Chen, Y., Aitchison, J. C., Zong, Y., and Li, S. H. (2016). OSL dating of past lake levels for a large dammed lake in southern Tibet and determination of possible controls on lake evolution. Earth Surf. Process. Landforms 41, 1467–1476. doi:10.1002/esp.3907
Costa, J. E., and Schuster, R. L. (1991). Documented historical landslide dams from around the world. US Geological Survey Open-File Report 91-239.
Costa, J. E., and Schuster, R. L. (1988). The formation and failure of natural dams. Geol. Soc. Am. Bull. 100, 1054–1068. doi:10.1130/0016-7606(1988)100<1054:tfafon>2.3.co;2
Dunning, S. A., and Armitage, P. (2011). “The grain-size distribution of rock-avalanche deposits: Implications for natural dam stability,” in Natural and artificial rockslide dams (Berlin, Heidelberg: Springer), 479–498.
Dunning, S., Petley, D., Rosser, N., and Strom, A. (2005). “The morphology and sedimentology of valley confined rock-avalanche deposits and their effect on potential dam hazard,” in Proceedings of the international conference on landslide risk management. Editors O. Hungr, R. Fell, R. Couture, and E. Eberhardt (Balkema, London, UK: Taylor and Francis), 691–701.
Ely, L. L., Brossy, C. C., House, P. K., Safran, E. B., O'Connor, J. E., Champion, D. E., et al. (2012). Owyhee River intracanyon lava flows:Does the river give a dam? Geol. Soc. Am. Bull. 124, 1667–1687. doi:10.1130/B30574.1
Fan, A. C. (2010). Quartz OSL dating of quaternary sediments from China. PhD Thesis. Hong Kong SAR: The University of Hong Kong.
Fan, X., Dufresne, A., Siva, S. S., Strom, A., Hermanns, R., Tacconi, S. C., et al. (2020). The formation and impact of landslide dams - state of the art. Earth-Science Rev. 203, 103116. doi:10.1016/j.earscirev.2020.103116
Guerrero, J., Gutiérrez, F., García-Ruiz, J. M., Carbonel, D., Lucha, P., and Arnold, L. J. (2018). Landslide-dam paleolakes in the central pyrenees, upper gállego river valley, NE Spain: Timing and relationship with deglaciation. Landslides 15, 1975–1989. doi:10.1007/s10346-018-1018-9
Guo, X. H. (2017). Relationship between landslide-dammed lakes with the evolution of upper stream of the yellow river from longyang gorge to liujia gorge on northeast margin of tibet plateau. PhD Thesis. Xi'an: Chang’an University.
Guo, X., Sun, Z., Lai, Z., Lu, Y., and Li, X. (2016). Optical dating of landslide-dammed lake deposits in the upper Yellow River, Qinghai-Tibetan Plateau, China. Quat. Int. 392, 233–238. doi:10.1016/j.quaint.2015.06.021
Hu, M., Wu, Z., Reicherter, K., Ali, S., Huang, X., and Zuo, J. (2021). A historical earthquake-induced landslide damming event at the qiaojia reach of the Jinsha River, SE Tibetan plateau: Implication for the seismic hazard of the xiaojiang fault. Front. Earth Sci. 9, 649543. doi:10.3389/feart.2021.649543
Kong, P., Na, C., Brown, R., Fabel, D., Freeman, S., Xiao, W., et al. (2011). Cosmogenic 10Be and 26Al dating of paleolake shorelines in Tibet. J. Asian Earth Sci. 41, 263–273. doi:10.1016/j.jseaes.2011.02.016
Kong, P., Na, C., Fink, D., Zhao, X., and Xiao, W. (2009). Moraine dam related to late Quaternary glaciation in the Yulong Mountains, southwest China, and impacts on the Jinsha River. Quat. Sci. Rev. 28, 3224–3235. doi:10.1016/j.quascirev.2009.08.005
Korup, O. (2002). Recent research on landslide dams – A literature review with special attention to New Zealand. Prog. Phys. Geogr. 26, 206–235. doi:10.1191/0309133302pp333ra
Korup, O., Strom, A. L., and Weidinger, J. T. (2006). Fluvial response to large rock-slope failures: Examples from the himalayas, the tien Shan, and the southern alps in New Zealand. Geomorphology 78, 3–21. doi:10.1016/j.geomorph.2006.01.020
Korup, O., and Tweed, F. (2007). Ice, moraine, and landslide dams in mountainous terrain. Quat. Sci. Rev. 26, 3406–3422. doi:10.1016/j.quascirev.2007.10.012
Li, Y., Feng, X., Yao, A., Lin, S., Wang, R., and Guo, M. (2021). A massive ancient river-damming landslide triggered by buckling failure in the upper Jinsha River, SE Tibetan Plateau. Bull. Eng. Geol. Environ. 80, 5391–5403. doi:10.1007/s10064-021-02293-4
Liang, L. J., Zhang, Z. R., and Dai, F. C. (2022). A Late Pleistocene landslide damming event and its implications for the evolution of river valley landforms in the upper Jinsha River, southeastern Tibetan Plateau. Quat. Int. 622, 97–109. doi:10.1016/j.quaint.2022.01.006
Liu, A. M., Yu, J. X., He, Y. Z., Lu, D. B., Mu, S. Y., Yue, L., et al. (2003). Environmental changes in the Yang Lake area of shengzha county, north tibet since 140 ka B.P. Quat. Sci. 23, 83–91.
Liu, F. L., Gao, H. S., Li, Z. M., Pan, B. T., and Su, H. (2020a). Terraces development and their implications for valley evolution of the Jinsha River from Qiaojia to Menggu. Acta Geogr. Sin. 75, 1095–1105. doi:10.11821/dlxb202005015
Liu, F. L., Gao, H. S., Li, Z. M., Pan, B. T., and Su, H. (2020b). Terraces development and their implications for valley evolution of the Jinsha River since late Pleistocene near longjie, yunnan. Adv. Earth Sci. 35, 431–440. doi:10.11867/j.issn.1001-8166.2020.039
Liu, W., Hu, K., Carling, P. A., Lai, Z., Cheng, T., and Xu, Y. (2018). The establishment and influence of Baimakou paleo-dam in an upstream reach of the Yangtze River, southeastern margin of the Tibetan Plateau. Geomorphology 321, 167–173. doi:10.1016/j.geomorph.2018.08.028
Ma, J., Chen, J., Cui, Z., Zhou, W., Liu, C., Guo, P., et al. (2018). Sedimentary evidence of outburst deposits induced by the Diexi paleolandslide-dammed lake of the upper Minjiang River in China. Quat. Int. 464, 460–481. doi:10.1016/j.quaint.2017.09.022
Murray, A. S., and Olley, J. M. (2002). Precision and accuracy in the optically stimulated luminescence dating of sedimentary quartz: A status review. Geochronometria 21, 1–16.
Murray, A. S., and Wintle, A. G. (2000). Luminescence dating of quartz using an improved single-aliquot regenerative-dose protocol. Radiat. Meas. 32, 57–73. doi:10.1016/S1350-4487(99)00253-X
Murray, A. S., and Wintle, A. G. (2003). The single aliquot regenerative dose protocol: Potential for improvements in reliability. Radiat. Meas. 37, 377–381. doi:10.1016/s1350-4487(03)00053-2
Ocakoğlu, F., Kır, O., Yılmaz, İ. Ö., Açıkalın, S., Erayık, C., Tunoğlu, C., et al. (2013). Early to Mid-Holocene Lake level and temperature records from the terraces of Lake Sünnet in NW Turkey. Palaeogeogr. Palaeoclimatol. Palaeoecol. 369, 175–184. doi:10.1016/j.palaeo.2012.10.017
Ouimet, W. B., Whipple, K. X., Royden, L. H., Sun, Z., and Chen, Z. (2007). The influence of large landslides on river incision in a transient landscape: Eastern margin of the Tibetan Plateau (Sichuan, China). Geol. Soc. Am. Bull. 119, 1462–1476. doi:10.1130/b26136.1
Peng, M., and Zhang, L. M. (2011). Breaching parameters of landslide dams. Landslides 9, 13–31. doi:10.1007/s10346-011-0271-y
Reneau, S. L., and Dethier, D. P. (1996). Late Pleistocene landslide-dammed lakes along the rio grande, white rock canyon, new Mexico. Geol. Soc. Am. Bull. 108, 1492–1507. doi:10.1130/0016-7606(1996)108<1492:LPLDLA>2.3.CO;2
Scherler, D., Munack, H., Mey, J., Eugster, P., Wittmann, H., Codilean, A. T., et al. (2014). Ice dams, outburst floods, and glacial incision at the Western margin of the Tibetan plateau: A >100 k.y. chronology from the shyok valley, karakoram. Geol. Soc. Am. Bull. 126, 738–758. doi:10.1130/b30942.1
Sharafi, S., Yamani, M., and Ehteshami-Moinabadi, M. (2019). Evidence of the formation of landslide-dammed lakes in the Zagros Mountains range, Iran. J. Mt. Sci. 16, 2389–2403. doi:10.1007/s11629-019-5434-7
Shen, Y. C. (1965). Landforms of the upper reaches of the Yangtze river. Beijing: Science Press, 162.
Su, H., Dong, M., and Hu, Z. (2019). Late Miocene birth of the Middle Jinsha River revealed by the fluvial incision rate. Glob. Planet. Change 183, 103002. doi:10.1016/j.gloplacha.2019.103002
Trauth, M. H., and Strecker, M. R. (1999). Formation of landslide-dammed lakes during a wet period between 40,000 and 25,000 yr B.P. in northwestern Argentina. Palaeogeogr. Palaeoclimatol. Palaeoecol. 153, 277–287. doi:10.1016/S0031-0182(99)00078-4
Wang, H., Cui, P., Liu, D., Liu, W., Bazai, N. A., Wang, J., et al. (2019). Evolution of a landslide-dammed lake on the southeastern Tibetan Plateau and its influence on river longitudinal profiles. Geomorphology 343, 15–32. doi:10.1016/j.geomorph.2019.06.023
Wang, H., Wang, P., Hu, G., Ge, Y., and Yuan, R. (2021). An early Holocene river blockage event on the Western boundary of the namche barwa syntaxis, southeastern Tibetan plateau. Geomorphology 395, 107990. doi:10.1016/j.geomorph.2021.107990
Wang, P., Chen, J., Dai, F., Long, W., Xu, C., Sun, J., et al. (2014). Chronology of relict lake deposits around the Suwalong paleolandslide in the upper Jinsha River, SE Tibetan Plateau: Implications to Holocene tectonic perturbations. Geomorphology 217, 193–203. doi:10.1016/j.geomorph.2014.04.027
Wassmer, P., Schneider, J. L., Pollet, N., and Schmitter-Voirin, C. (2004). Effects of the internal structure of a rock–avalanche dam on the drainage mechanism of its impoundment, Flims sturzstrom and Ilanz paleo-lake, Swiss Alps. Geomorphology 61, 3–17. doi:10.1016/j.geomorph.2003.11.003
Wayne, W. J. (1999). The alemania rockfall dam: A record of a mid-holocene earthquake and catastrophic flood in northwestern Argentina. Geomorphology 27, 295–306. doi:10.1016/S0169-555X(98)00080-4
Wei, X. Y., Huang, X. R., Pan, L., and Zhang, L. (2019). Response of runoff change to Climate in the upper reaches of Jinsha River in past 60 years. Water Power 45, 12–17.
Weidinger, J. (2011). “Stability and life span of landslide dams in the himalayas (India, Nepal) and the Qin ling mountains (China),” in Natural and artificial rockslide dams (Berlin, Heidelberg: Springer), 243–277.
Wu, L. Z., Deng, H., Huang, R. Q., Zhang, L. M., Guo, X. G., and Zhou, Y. (2019). Evolution of lakes created by landslide dams and the role of dam erosion: A case study of the jiajun landslide on the dadu river, China. Quat. Int. 503, 41–50. doi:10.1016/j.quaint.2018.08.001
Wu, X. G., and Cai, C. X. (1992). The neotectonic activity along the central segment of Jinshajiang fault zone and the epicentral determination of Batang M 6.5 earthquake. J. Seismol. Res. 15, 401–410.
Xia, J. W., and Zhu, M. (2020). Study on tectonic characteristics and activity of middle section of Jinshajiang Main Fault Zone. Yangtze River 51, 131–137. doi:10.16232/j.cnki.1001-4179.2020.05.022
Xu, H., Chen, J., Cui, Z., and Chen, R. (2020). Sedimentary facies and depositional processes of the diexi ancient dammed lake, upper minjiang river, China. Sediment. Geol. 398, 105583. doi:10.1016/j.sedgeo.2019.105583
Yagi, H. (1997). Origin of the Phoksundo tal (lake), dolpa district, Western Nepal. J. Nepal Geol. Soc. 15, 1–7. doi:10.3126/jngs.v15i0.32115
Yu, S. Y., Colman, S. M., and Lai, Z. P. (2019). Late-Quaternary history of ‘great lakes’ on the Tibetan Plateau and palaeoclimatic implications - a review. Boreas 48, 1–19. doi:10.1111/bor.12349
Zhang, X., Walling, D. E., He, X., and Long, Y. (2009). Use of landslide-dammed lake deposits and pollen tracing techniques to investigate the erosional response of a small drainage basin in the Loess Plateau, China, to land use change during the late 16th century. Catena 79, 205–213. doi:10.1016/j.catena.2009.05.001
Zhang, Y. C., Li, J. J., Zhu, J. J., Chen, Y., Pan, B. T., and Kuang, M. S. (1998). Studies on development of Jinshajiang river during late cenozoic. Yunnan Geogr. Environ. Res. 10, 43–48.
Zhang, Y. C. (1993). Study on development of the Jinsha River and uplift of the Tibetan plateau since the late cenozoic. PhD Thesis. Lanzhou: Lanzhou University.
Zhang, Y., Zhao, X., Lan, H., and Xiong, T. (2011). A Pleistocene landslide-dammed lake, Jinsha River, yunnan, China. Quat. Int. 233, 72–80. doi:10.1016/j.quaint.2010.10.020
Zhao, X. T., Zhao, Y. Y., Zheng, M. P., Ma, Z. B., Cao, J. K., and Li, M. H. (2011). Late Quaternary lake development and denivellation of Bankog Co as well as lake evolution of Southeastern North Tibetan Plateau during the last great lake period. Acta Geoscientica Sinica 32, 13–26. doi:10.3975/cagsb.2011.01.03
Zhao, X. T., Zhu, D. G., Wu, Z. H., and Ma, Z. B. (2002). The development of Nam Co lake in tibet since late Pleistocene. Acta Geosci. Sin. 23, 329–334.
Zhao, X. T., Zhu, D. G., Yan, F. H., Wu, Z. H., Ma, Z. B., and Mai, X. S. (2003). Climatic change and lakelake-level variation of Nam Co, xizang since the last interglacial stage. Quat. Sci. 23, 41–52.
Zhao, Z. M., and Li, A. R. (2006). Evolutionary characteristics of river and lake terraces in different areas of the Northern Qinghai-Tibet Plateau. Geol. Bull. China 25, 221–225.
Keywords: lacustrine terraces, evolutional process, landslide-dammed lake, Tibetan Plateau, Late Pleistocene
Citation: Xu H, Chen J, Chen R, Cui Z, Mi D and Shi L (2023) Evolution process of the Yinduba paleolandslide-dammed lake in the upper Jinsha River, SE Tibetan Plateau. Front. Earth Sci. 11:1144992. doi: 10.3389/feart.2023.1144992
Received: 15 January 2023; Accepted: 02 May 2023;
Published: 12 May 2023.
Edited by:
Hans-Balder Havenith, University of Liège, BelgiumCopyright © 2023 Xu, Chen, Chen, Cui, Mi and Shi. This is an open-access article distributed under the terms of the Creative Commons Attribution License (CC BY). The use, distribution or reproduction in other forums is permitted, provided the original author(s) and the copyright owner(s) are credited and that the original publication in this journal is cited, in accordance with accepted academic practice. No use, distribution or reproduction is permitted which does not comply with these terms.
*Correspondence: Jian Chen, amlhbmNoZW5AY3VnYi5lZHUuY24=