- Department of Earth and Environmental Science, School of Human Settlements and Civil Engineering, Xi’an Jiaotong University, Xi’an, Shaanxi, China
Vegetation plays a significant role in terrestrial ecosystems due to its strong carbon absorption capability and multiple feedback effects on the climate system. The soil moisture availability determines vegetation growth, especially in the drylands. Although there has been increasing interest in issues such as the vegetation’s response to a specific climate variable, it remains unclear how soil moisture can quantitatively influence the vegetation in the drylands. In this study, we investigated the increased response of the vegetation to soil moisture and identified its key mechanism in the northern hemisphere drylands (NHD) from 1982 to 2010. The Methods included the use of the Lindeman-Merenda-Gold method. The results showed that the sensitivity of the vegetation dynamics to soil moisture significantly increased over the past 29 years (slope = 0.008, p < 0.0001), and the trend during 1996–2007 (slope = 0.025 m3/m3/yr, p < 0.0001) increased more rapidly than the trend during 1984–1995 (slope = −0.005 m3/m3/yr, p = 0.0143), which indicates increased water restrictions in recent years. Further analysis showed that atmospheric CO2 was the major contributor (27.2%) to the sensitivity changes, followed by climate change (27%), and nitrogen deposition (19%). The changes in the ecosystem structure (represented by the non-tree cover areas) and climate vacillation contributed similarly to the sensitivity change (14% and 12%). These findings can help with understanding the spatiotemporal impact of water restrictions on vegetation in the NHD and the related influencing mechanisms of vegetation growth and soil moisture in the greening and warming of the NHD.
1 Introduction
Since the industrial revolution, the ever-increasing greenhouse gases have been changing the surface radiation and energy budget processes, which has increased the surface temperature and led to climate warming, particularly in the northern hemisphere (IPCC, 2014). It is estimated that the past 30 years (1983–2012) were the warmest period in the northern hemisphere during past 1,400 years (IPCC, 2014). Vegetation plays a significant role in terrestrial ecosystems since it accounts for about 42% (∼28 PgC) of terrestrial carbon storage and assimilates approximately 20% of the annual anthropogenic CO2 emissions (Pan et al., 2011; Le Quere et al., 2016). However, not only energy and food security but also vegetation growth is vulnerable and restricted under climate warming and socio-economic changes because of water shortages (Huang et al., 2021; Qin et al., 2022), which is the most important factor in driving vegetation growth around the world. The enhanced eco-hydrosphere (Yang et al., 2021), changes in precipitation patterns (Dore, 2005; Gu and Adler, 2013; Duan et al., 2022), possible soil moisture decreases (Sherwood and Fu, 2014; Huang et al., 2016), more frequent and severe floods and droughts (Turner et al., 2020), changes in land cover/land use (Huang et al., 2018), and changes in vegetation types (Hufnagel and Garamvolgyi, 2014) due to climate change are all exacerbating the impact of water availability on vegetation (Lemordant et al., 2018; Fernandez-Martinez et al., 2019). Drylands, where rainfall is scarce and soil moisture is almost the only effective source of water availability (Wang et al., 2013; Zhao et al., 2020), are a key area where vegetation growth is subject to water stress. Thus, understanding the properties of vegetation and soil moisture, the close relationship between them, and the drivers of vegetation sensitivity to soil moisture in the drylands is important for climate and ecosystem management on a global scale.
The growth dynamics of terrestrial vegetation directly or indirectly control the energy transfer process between the surface and the atmosphere and the feedback on the climate system (Richardson et al., 2013). Although the growth of terrestrial vegetation is comprehensively regulated by environmental factors, such as light, temperature, water availability, atmospheric CO2 concentration, and nutrient availability (Cleland et al., 2007; Korner and Basler, 2010; Korner, 2018), water availability is still the main factor that controls vegetation growth in the drylands (Feng et al., 2021; Zhao et al., 2021). In recent years, with the development in remote sensing technology for hydrology analysis and water resources management (Duan et al., 2021), many studies have investigated the response of vegetation to water availability under climate warming. For example, Wu et al. (2018) and Wu et al. (2022) both assessed drought legacy effects on vegetation. Zhao et al. (2023) indicated that the ecosystem of a typical drylands was facing crucial water limitation in recent decades. Additionally, Wang et al. (2021) investigated the changes in the length of the four seasons and their impact on vegetation in the drylands. Moreover, Anderegg et al. (2018) studied vegetation resilience under and recovery from severe drought. These studies mainly focused on the interaction between vegetation and certain indicators related to drought temporally. However, the vegetation responses to water availability factors are complex and have a fierce controversy due to limited knowledge of vegetation response on a global scale (Zhao and Running, 2010). In addition, several studies have focused attention on soil moisture, a hydrological variable directly related to the water availability of vegetation in drylands, showing that soil moisture is important in controlling vegetation growth in the drylands (Vicente-Serrano et al., 2013; Knapp et al., 2015). For example, Li et al. (2022) suggested that the sensitivity of the leaf area index (LAI) to soil moisture has increased significantly in many drylands globally. Also, Dang et al. (2022) found that soil moisture had a greater impact on vegetation production in arid and semi-arid regions, while temperature changes in humid regions had a greater impact on vegetation. Nevertheless, it remains unclear what is the exact impact of soil moisture on vegetation growth within the context of climate change in the drylands. The northern hemisphere drylands (NHD) have a regulating effect on global climate change and play an important role in maintaining the balance of the ecosystem. As an arid zone spanning three continents, the NHD has research significance in terms of determining how vegetation growth is affected by drought in this region (Xu et al., 2016).
To address these, this study aimed to quantify the spatiotemporal response of the vegetation to soil water sensitivity during 1982–2010 using the growing season remote sensing normalized difference vegetation index (NDVI) and algorithm-based soil moisture (0–10 cm depth) in the NHD. The objectives of this study were to 1) investigate spatiotemporal changes in the vegetation sensitivity to soil moisture in different continents during 1982–2010, and 2) provide a northern hemisphere overview of the potential controlling factors of sensitivity changes in the drylands.
2 Materials and methods
2.1 Study area
In this study, the drylands were classified according to the criteria of the United Nations Environment Programme. In 1977, the World Atlas of Desertification was proposed by the United Nations Food and Agriculture Organization and other institutions, and it uses the aridity index (AI; the ratio of the average annual precipitation and the average potential evapotranspiration). Areas with an AI lower than 0.65 are defined as drylands (Feng and Fu, 2013), and the drylands are divided into four zones: the dry-humid (0.5 < AI < 0.65), semiarid (0.2 < AI < 0.5), arid (0.05 < AI < 0.2), and hyper-arid regions (AI < 0.05). In this study, three (Supplementary Figure S1) parts of the Northern Hemisphere were selected as the study area and termed the Northern Hemisphere Drylands (NHD). This included the dry-humid, semiarid, and arid regions, which are mainly located at mid-latitudes (30°–60°) across Asia, Europe, Africa, and North America. Specifically, the drylands are mainly distributed in mid-latitude southwestern Europe, Central Asia, North Africa, and western North America. The hyperarid region was excluded since the seasonality of the vegetation growth in the extremely arid areas is not obvious. Globally, drylands cover 41.3% of the Earth’s land surface, and dryland vegetation provides 40% of the net primary productivity of vegetation globally (Wang et al., 2012).
2.2 Datasets
The NDVI has been widely used to reflect global-scale vegetation growth. In this study, the Global Inventory Modeling and Mapping Studies NDVI3g dataset (Tucker et al., 2005) with a spatial resolution of 0.0833° and a 15-day temporal resolution from 1981 to 2015 was used. The dataset has the characteristics of good correlation with the in situ observation data and long-term monitoring of vegetation dynamic changes. To match the meteorological and soil moisture data, the GIMMMS NDVI was spatially aggregated at a 0.5° spatial resolution.
The Global Land Evaporation Amsterdam Model soil moisture v3.5a dataset from 1980 to 2020 with a spatial resolution of 0.25° was used to obtain the soil moisture data (Miralles et al., 2011; Martens et al., 2017b). The model is a set of algorithms that separately estimate terrestrial evaporation, surface soil moisture (0–10 cm), and root-zone soil moisture (10–250 cm), and it has been widely used for estimating vegetation-climate feedback and large-scale hydrological applications (Martens et al., 2016; Martens et al., 2017a). The surface soil moisture was selected as the main focus since it largely reflects precipitation and temperature.
The CO2 concentration and nitrogen deposition from 1700 to 2010 with a spatial resolution of 0.5° were obtained from the Multi-scale Synthesis and Terrestrial Model Intercomparison Project, which was designed to provide a consistent and unified modeling framework (Liu et al., 2014; Wei et al., 2014; Huntzinger et al., 2018; Huntzinger et al., 2021).
The ecosystem structure was represented by the tree cover (percentage of pixels that were covered by the tree canopy), which was obtained from a data layer from the Vegetation Continuous Fields Version 1 data product. This layer contains the percentage of tree cover, non-tree vegetation, and bare ground from 1982 to 2015 with a spatial resolution of 0.05° (Hansen and Song, 2018).
The air temperature (°C) and precipitation were converted from the air temperature (K) and precipitation rate of the China Meteorological Forcing Dataset (CMFD; Yang and He, 2019) from 1979 to 2018 with a spatial resolution of 0.1°. The CMFD is widely used in hydrological and climate modeling research (Yang et al., 2010; He et al., 2020).
To accurately reflect the vegetation growth in the drylands, the seasons with poor vegetation growth were discarded and a time series was chosen for the growing seasons (4–10 months). For easier computer processing, all of the above data were resampled at a 0.1° × 0.1° spatial resolution using the bilinear interpolation method by Arcgis 10.2. Because of the dataset limits, the study period was chosen from 1982 to 2010. Except for the temperature and precipitation, the other data were detrended and normalized using linear de-trending (Eq. 1) and the Min-Max normalization method Eq. 2 by Python 3.8. These methods were used to emphasize the fluctuation of the data trend and make the characteristics of the different dimensions comparable. Additionally, the temperature and precipitation data were only used after linear de-trending (TEM+PR) and Min-Max normalization (TEMvar+PRvar) to represent climate change and climate vacillation, respectively. Eq. 1 is provided below:
where j represents the year from 1982 to 2010,
where
2.3 Defining the sensitivity of the vegetation to soil moisture
To quantify the sensitivity of the vegetation to soil moisture, a one-variable linear model was built. The model is defined as follows:
where
For the temporal sensitivity trend analysis, three moving windows, namely, 5-year, 10-year, and 15-year moving windows were used. For the spatial sensitivity trend analysis, 29-year data was used to calculate the overall
2.4 Attribution method
In this study, a multivariate linear regression model Eq. 5 was built to quantify the relative importance of the meteorological indexes to the sensitivity of the vegetation to soil moisture for each grid cell, assessing the factors that affect the water resources and vegetation in the drylands. The equations are as follows:
where
To obtain the importance rankings, a function was used to calculate the relative importance metrics for the linear models in the “relaimpo” package in R (https://prof.bht-berlin.de/groemping/software/relaimpo/). The Lindeman-Merenda-Gold (LMG) method was used to quantify the relative importance of the meteorological indexes to the sensitivity of the vegetation to soil moisture for each grid cell. The LMG method is typically used to avoid the order effect of the regression variables (Li et al., 2021) and evaluate the robustness of the attribution analysis (Jiao et al., 2021). The Mann-Kendall trend test, was used to analyze trends of the importance rankings over years.
3 Results
3.1 Spatial patterns of the changes in the vegetation and soil moisture
The spatial patterns of the monthly mean growing season (April to October) NDVI and soil moisture were drawn at a spatial resolution of 0.1° × 0.1° during 1982–2010 (Figures 1A–D). The NDVI exhibited generally increasing trends (Figures 1A, B), and the overall growth trend of the NDVI in the NHD was 0.035 years-1. Specifically, the NHD regions in the middle and high latitudes of Eurasia (55–65°N), Mideast of North America, Iberian Peninsula, and central China, and regions around the Black Sea and Caspian Sea, exhibited a vegetation greening trend and significantly increasing trends. Decreasing NDVI trends were observed in other regions of the NHD, such as Midwest North Asia and near Mongolia. In summary, NDVI in 70.74% of the area increased and only 29.14% of the area decreased during past three decades. The soil moisture had widely decreased trends (Figures 1C, D), and the overall descending trend of the soil moisture in the NHD was −0.015 m3/m3/year-1. Except for the NHD regions in central Russia, west regions around the Mediterranean Sea, and a small part of central and northwest China, the other regions exhibited an obvious decrease in soil moisture. Statistically, soil moisture in 59.15% of the total area of the decreased and 40.85% of the area increased during the study period.
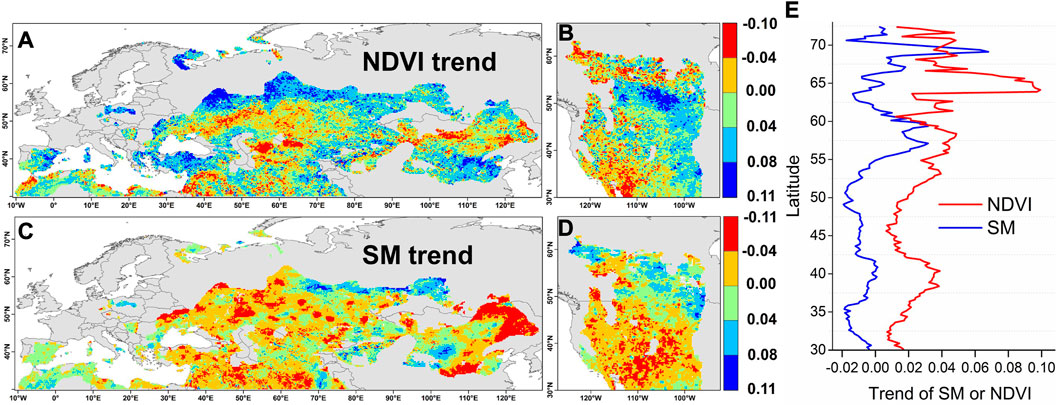
FIGURE 1. (A, B) Spatiotemporal trend of the normalized difference vegetation index (NDVI) and soil moisture (SM) in the drylands of the Northern Hemisphere from 1982 to 2010 and (C,D) The spatial distribution trend of the NDVI and SM, respectively. (E) The average trend of the NDVI and SM per pixel per continent in the different latitudes.
The average trends of the NDVI and soil moisture in terms of pixels per continent in the different latitudes are shown in Figure 1E. Overall, the NDVI in the different latitudes was generally increasing, while the soil moisture was decreasing below 55°N and fluctuated in the high latitudes. Importantly, there was an apparent positive correlation between vegetation greening and soil moisture between 30°N and 60°N (Figure 1E). This indicated the increased response of the vegetation to soil moisture in the major areas of the drylands in the northern hemisphere, which suggests a water constraint.
3.2 Increasing sensitivity of the vegetation to soil moisture
To further support the potential water constraint based on the response of the vegetation to soil moisture, the significant positive and negative trends in the vegetation-soil moisture sensitivity (
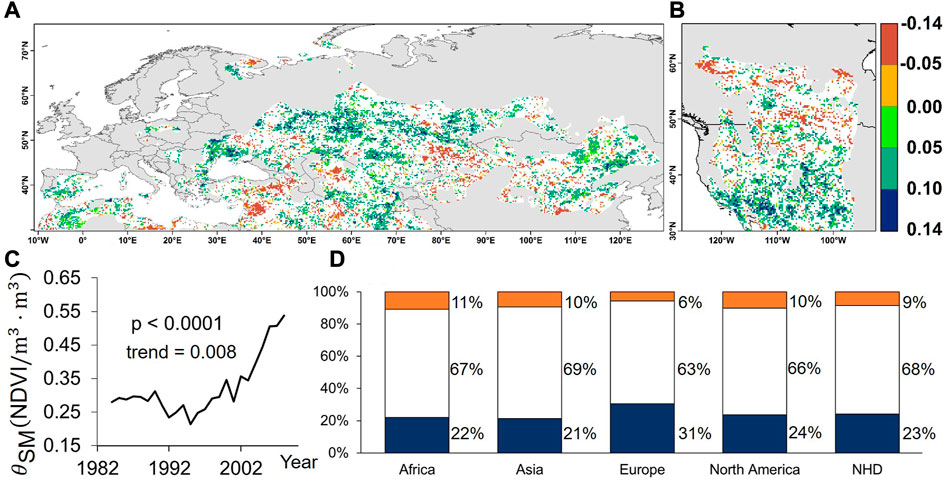
FIGURE 2. Significant positive and negative trends in vegetation-soil moisture sensitivity (Mann-Kendall test, p < 0.05) across the Northern Hemisphere drylands (NHD). The normalized difference vegetation index and soil moisture were represented by NDVI and SM. (A, B) The spatial distribution of the vegetation-soil moisture sensitivity trends across the NHD. (C) A time series of the vegetation-soil moisture sensitivity during 1982–2010. (D) The fractions (%) of significant positive (dark blue) and negative (orange) pixels per continent. The blanks in (A, B, D) are land fractions that underwent a non-significant change.
Spatially, 70% and 20% of the NHD area were dominated by significant positive and negative trends (p < 0.05) during the study period, respectively, whereas only 10% of the NHD land fractions had a non-significant change (Figure 2A). Moreover, higher positive sensitivity of the vegetation to soil moisture was found in southern North America and central Eurasia. This indicated widespread increased sensitivity to soil moisture.
Temporally, a significantly increasing trend (p < 0.0001) was identified for
In addition, the sensitivity of the vegetation to soil moisture in the different continents in the NHD is shown in Figure 2D. The continents with significantly increased sensitivity were: Europe, North America, Africa, and Asia. Europe was the most sensitive to moisture, with 31% of the drylands showing a significant increase in sensitivity and only 6% of the drylands showing a significant decrease in sensitivity, which was slightly different from the other continents. In contrast, 23% of the NHD had significantly increased vegetation sensitivity, 9% had significantly decreased vegetation sensitivity, and 68% had no significant change in vegetation sensitivity. This result was also consistent with the results in Figure 1A, where a considerable part of the drylands faced productivity constraints.
3.3 Mechanisms for sensitivity changes
Finally, we evaluated the order of importance of the CO2 concentration, nitrogen deposition, ecosystem structure, climate change, and climate vacillation for
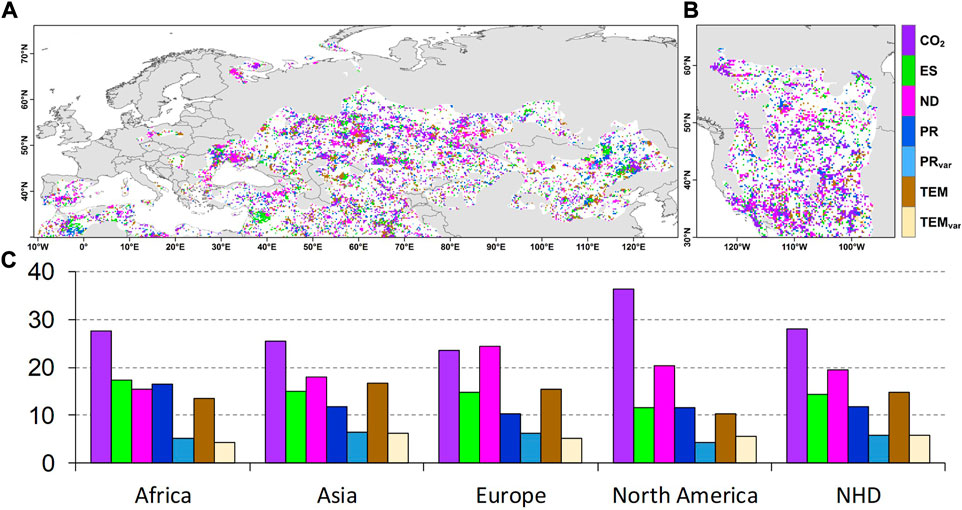
FIGURE 3. Main drivers of the significant trends (Mann-Kendall, p < 0.05) in the vegetation-soil moisture sensitivity per pixel. (A, B) The spatial distribution of the drivers of the vegetation-soil moisture sensitivity in the Northern Hemisphere drylands (NHD). (C) The area fractions of the lands that are dominated by each factor (%). Abbreviations: CO2, ES, ND, PR, PRvar, TEM, and TEMvar are the CO2 concentration, ecosystem structure, nitrogen deposition, precipitation, variation in precipitation, air temperature, and variation in air temperature, respectively.
The trend and fluctuation values of the precipitation and temperature were combined to visualize the climate and vegetation contributions to the enhanced response with the LMG method (Figure 4). The results (Figure 4A) showed that the most important controlling factor in the whole NHD region was the CO2 concentration (28%). The second most important controlling factor was climate change (27%), which was followed by nitrogen deposition (19%), ecosystem structure (14%), and climate vacillation (12%). All of the controlling factors played a positive role in the sensitivity increase (Figure 4B), which indicated strong water limitation in the future.
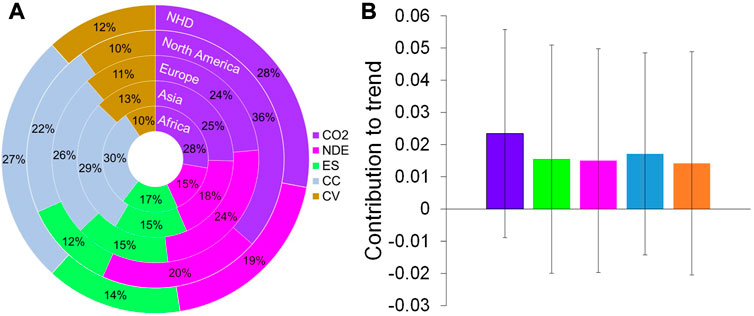
FIGURE 4. Climate and vegetation contributions to the enhanced response in the Northern Hemisphere drylands (NHD). (A) The proportional contribution of the control factors based on the Lindeman-Merenda-Gold method. (B) The contribution to the total trend for the five major control factors. Abbreviations: CO2, NDE, ES, CC, and CV are the CO2 concentration, nitrogen deposition, ecosystem structure, climate change, and climate vacillation, respectively.
4 Discussion
In this study, an obvious increase in the NDVI and decrease in the soil moisture was observed regionally and latitudinally (in the mid-latitudes) during the study period. Generally, the large-scale greening of vegetation is consistent with other studies, for example, Xu et al. (2016); Lian et al. (2020) both found that the growing season in the middle latitudes of the northern hemisphere was brought forward and that the region was greening. In addition, this greening also reduced soil moisture and aggravated soil moisture limitation (Deng et al., 2020), especially in several semi-arid and arid regions of the world. These findings were also consistent with this study, that is, the soil moisture decreased significantly in the NHD.
Additionally, the NDVI showed a trend of a steady fluctuation and then a gradual increase in response to the soil moisture at the corresponding latitude, reflecting the response of the vegetation growth to water availability. However, there were unique climate conditions that should also be considered. For example, due to the westerly and polar circulation, the climate in the central and northern parts of Eurasia was wetter than that in the other arid regions during the study period, so the vegetation growth was less affected by the water constraint. Moreover, the vegetation changes in the NHD were also affected by human activities, especially land use/land cover changes (de Beurs and Henebry, 2004). For example, in 1991, after the disintegration of the former Soviet Union, a large amount of farmland was abandoned, and livestock production declined sharply (Propastin et al., 2008). Also, Kazakhstan’s grassland fires were among the most serious in the world from 2001 to 2009 (Loboda et al., 2012). The Loess Plateau, the world’s largest and deepest deposit region of loess located in China, has undergone significant greening since the implementation of the Grain for Green Project in 1999 (Feng et al., 2016; Fu et al., 2017). Thus plowing, fire, grazing, and urbanization have all changed the drylands’ NDVI. Overall, vegetation change is the comprehensive result of various factors, except in the drylands, where the soil moisture plays a more important role.
The results of this study showed that there was a large area with a significant increase in vegetation sensitivity to soil moisture in the drylands of each continent. Similar results were also reported by other researchers. For example, Papagiannopoulou et al. (2017) suggested that water availability was the most powerful factor that drove vegetation growth globally (about 61% of the vegetated surface was primarily water-limited) during 1981–2010. Jiao et al. (2021) suggest that there was a strong increase in water constraints between 1982 and 2015 in the extratropical Northern Hemisphere. Furthermore; Li et al. (2022) indicated that the sensitivity of the LAI to water availability has increased significantly, especially in many arid and semi-arid regions globally in the past 40 years. These results were also supported by studies on the drylands in Australia, China, and America (Ma et al., 2015; Feng et al., 2016; Maurer et al., 2020).
Temporally, the
To find out what caused the increased
Such effects could be even more serious in drylands due to the enhanced changes in the lengths of spring, summer, and autumn. Global mean surface warming reached 1.08C above the preindustrial period (1850–1900) in 2017 (Hoegh-Guldberg et al., 2019). The trend in temperature might be amplified in the future with the rising radiative forcing. Accordingly, the changes of the four seasons would be intensified (Wang et al., 2021), resulting in a series of severer impacts and risks in physical and biological systems. The changes in the four seasons and related influences in drylands could be further enhanced because of their high sensitivity to temperature changes. Therefore, future investigations should consider the effects of seasonal variations in drylands.
Overall, our findings highlight a strong dependence of global vegetation on water availability. These results suggest that vegetation development may be more susceptible to future fluctuation of soil water availability. Also, most of these areas are projected to face more severe hydrological conditions (Satoh et al., 2022). Due to the high sensitivity of drylands vegetation to soil moisture, the related impact of water availability may be further enhanced.
5 Conclusion and perspectives
This study investigated the increased response of vegetation to soil moisture and identified its key mechanisms in the NHD from 1982 to 2010. The results identified significant greening in the NHD. The NDVI showed a trend of steady fluctuation and then a gradual increase in response to the soil moisture at the corresponding latitude, reflecting the response of the vegetation growth to water availability. The increased sensitivity of the vegetation to soil moisture (
This study has limitations and uncertainties that should be acknowledged. Eq. 5 was used to explain the sensitivity of vegetation change to soil moisture, and CO2, ND, ES, CC, and CV were selected as the relevant factors. However, many other variables also play a role in this relationship and some of them may not yet be fully understood. As a result, the focus on these five factors alone may not accurately reflect the complexities of the relationship and should be kept in mind in future studies. Additionally, the study only considered surface soil moisture (0–10 cm) data, as it is more positively related to vegetation growth in typical drylands in the North Hemisphere (Yu et al., 2022). However, the relationship between deep soil moisture and vegetation growth is also important and should be considered in future research (Schenk and Jackson, 2002).
Data availability statement
Publicly available datasets were analyzed in this study. These data can be found here: The GIMMMS NDVI is downloaded from https://data.tpdc.ac.cn/en/data/9775f2b4-7370-4e5e-a537-3482c9a83d88/. The GLEAM soil moisture data are available online at https://www.gleam.eu/. The CMFD climate data are available at https://www.tpdc.ac.cn/zh-hans/data. The atmospheric CO2 concentration and nitrogen deposition data can be obtained from https://nacp.ornl.gov/MsTMIP.shtml. The tree cover data are available at https://lpdaac.usgs.gov/products/vcf5kyrv001/.
Author contributions
Conceptualization, XW, FZ, and YW; formal analysis, XW, FZ, and YW; funding acquisition, FZ and YW; methodology, FZ and YW; validation, XW; visualization, XW; writing—original draft, XW and FZ; writing—review and editing, FZ and YW. All authors have read and agreed to the published version of the manuscript.
Funding
This study was funded by the National Science Foundation of China (42101029 and 42271025), the China Postdoctoral Science Foundation (2020M683451 and 2022T150513), the National Key Research and Development Program of China (2022YFF1302200).
Acknowledgments
We appreciate the detailed suggestions and comments from the editor and reviewers.
Conflict of interest
The authors declare that the research was conducted in the absence of any commercial or financial relationships that could be construed as a potential conflict of interest.
Publisher’s note
All claims expressed in this article are solely those of the authors and do not necessarily represent those of their affiliated organizations, or those of the publisher, the editors and the reviewers. Any product that may be evaluated in this article, or claim that may be made by its manufacturer, is not guaranteed or endorsed by the publisher.
Supplementary material
The Supplementary Material for this article can be found online at: https://www.frontiersin.org/articles/10.3389/feart.2023.1144410/full#supplementary-material
References
Anderegg, W. R. L., Konings, A. G., Trugman, A. T., Yu, K. L., Bowling, D. R., Gabbitas, R., et al. (2018). Hydraulic diversity of forests regulates ecosystem resilience during drought. Nature 561 (7724), 538–541. doi:10.1038/s41586-018-0539-7
Adams, H. D., Guardiola-Claramonte, M., Barron-Gafford, G. A., Villegas, J. C., Breshears, D. D., Zou, C. B., et al. (2009). Temperature sensitivity of drought-induced tree mortality portends increased regional die-off under global-change-type drought. Proceedings of the national academy of sciences 106 (17), 7063–7066.
Cleland, E. E., Chuine, I., Menzel, A., Mooney, H. A., and Schwartz, M. D. (2007). Shifting plant phenology in response to global change. Trends Ecol. Evol. 22 (7), 357–365. doi:10.1016/j.tree.2007.04.003
Dang, C. Y., Shao, Z. F., Huang, X., Qian, J. X., Cheng, G., Ding, Q., et al. (2022). Assessment of the importance of increasing temperature and decreasing soil moisture on global ecosystem productivity using solar-induced chlorophyll fluorescence. Glob. Change Biol. 28 (6), 2066–2080. doi:10.1111/gcb.16043
de Beurs, K. M., and Henebry, G. M. (2004). Land surface phenology, climatic variation, and institutional change: Analyzing agricultural land cover change in Kazakhstan. Remote Sens. Environ. 89 (4), 497–509. doi:10.1016/j.rse.2003.11.006
Denissen, J. M. C., Teuling, A. J., Pitman, A. J., Koirala, S., Migliavacca, M., Li, W. T., et al. (2022). Widespread shift from ecosystem energy to water limitation with climate change. Nat. Clim. Change. 12 (7), 677-684. doi:10.1038/s41558-022-01403-8
Deng, Y. H., Wang, S. J., Bai, X. Y., Luo, G. J., Wu, L. H., Chen, F., et al. (2020). Vegetation greening intensified soil drying in some semi-arid and arid areas of the world. Agric. For. Meteorology 292, 108103. doi:10.1016/j.agrformet.2020.108103
Dore, M. H. I. (2005). Climate change and changes in global precipitation patterns: What do we know? Environ. Int. 31 (8), 1167–1181. doi:10.1016/j.envint.2005.03.004
Duan, W. L., Maskey, S., Chaffe, P. L. B., Luo, P. P., He, B., Wu, Y. P., et al. (2021). Recent advancement in remote sensing technology for hydrology analysis and water resources management. Remote Sens. 13 (6), 1097. doi:10.3390/rs13061097
Duan, W. L., Zou, S., Christidis, N., Schaller, N., Chen, Y. N., Sahu, N., et al. (2022). Changes in temporal inequality of precipitation extremes over China due to anthropogenic forcings. Npj Clim. Atmos. Sci. 5 (1), 33. doi:10.1038/s41612-022-00255-5
Feng, S., and Fu, Q. (2013). Expansion of global drylands under a warming climate. Atmos. Chem. Phys. 13 (19), 10081–10094. doi:10.5194/acp-13-10081-2013
Feng, X., Fu, B., Piao, S., Wang, S., Ciais, P., Zeng, Z., et al. (2016). Revegetation in China’s Loess Plateau is approaching sustainable water resource limits. Nat. Clim. Change 6 (11), 1019–1022. doi:10.1038/nclimate3092
Feng, X., Fu, B., Zhang, Y., Pan, N., Zeng, Z., Tian, H., et al. (2021). Recent leveling off of vegetation greenness and primary production reveals the increasing soil water limitations on the greening Earth. Sci. Bull. 66 (14), 1462–1471. doi:10.1016/j.scib.2021.02.023
Fernandez-Martinez, M., Sardans, J., Chevallier, F., Ciais, P., Obersteiner, M., Vicca, S., et al. (2019). Global trends in carbon sinks and their relationships with CO2 and temperature. Nat. Clim. Change 9 (1), 73–79. doi:10.1038/s41558-018-0367-7
Fu, B. J., Wang, S., Liu, Y., Liu, J. B., Liang, W., and Miao, C. Y. (2017). Hydrogeomorphic ecosystem responses to natural and anthropogenic changes in the Loess Plateau of China. Annu. Rev. Earth Planet. Sci. 45, 223–243. doi:10.1146/annurev-earth-063016-020552
Gonsamo, A., Ciais, P., Miralles, D. G., Sitch, S., Dorigo, W., Lombardozzi, D., et al. (2021). Greening drylands despite warming consistent with carbon dioxide fertilization effect. Glob. Chang. Biol. 27 (14), 3336–3349. doi:10.1111/gcb.15658
Gu, G. J., and Adler, R. F. (2013). Interdecadal variability/long-term changes in global precipitation patterns during the past three decades: Global warming and/or Pacific decadal variability? Clim. Dyn. 40 (11-12), 3009–3022. doi:10.1007/s00382-012-1443-8
Hansen, M., and Song, X. (2018). Data from: Vegetation Continuous Fields (VCF) Yearly Global 0.05 Deg Sioux Falls. NASA EOSDIS Land Processes DAAC. doi:10.5067/MEaSUREs/VCF/VCF5KYR.001
He, J., Yang, K., Tang, W., Lu, H., Qin, J., Chen, Y., et al. (2020). The first high-resolution meteorological forcing dataset for land process studies over China. Sci. Data 7 (1), 25–11. doi:10.1038/s41597-020-0369-y
Hoegh-Guldberg, O., Jacob, D., Taylor, M., Bolanos, T. G., Bindi, M., Brown, S., et al. (2019). The human imperative of stabilizing global climate change at 1.5 degrees C. Science 365 (6459). doi:10.1126/science.aaw6974
Huang, J. P., Yu, H. P., Guan, X. D., Wang, G. Y., and Guo, R. X. (2016). Accelerated dryland expansion under climate change. Nat. Clim. Change 6 (2), 166–171. doi:10.1038/Nclimate2837
Huang, K., Xia, J. Y., Wang, Y. P., Ahlstrom, A., Chen, J. Q., Cook, R. B., et al. (2018). Enhanced peak growth of global vegetation and its key mechanisms. Nat. Ecol. Evol. 2 (12), 1897–1905. doi:10.1038/s41559-018-0714-0
Huang, W. J., Duan, W. L., and Chen, Y. N. (2021). Rapidly declining surface and terrestrial water resources in Central Asia driven by socio-economic and climatic changes. Sci. Total Environ. 784, 147193. doi:10.1016/j.scitotenv.2021.147193
Hufnagel, L., and Garamvolgyi, A. (2014). Impacts of climate change on vegetation distribution No. 2-Climate change induced vegetation shifts in the new world. Appl. Ecol. Environ. Res. 12 (2), 355–422. doi:10.15666/aeer/1202_355422
Hufnagel, L., and Garamvölgyi, Á. (2014). Impacts of climate change on vegetation distribution No. 2-climate change induced vegetation shifts in the new world. Appl. Ecol. Environ. Res. 12 (2), 355–422. doi:10.15666/aeer/1202_355422
Huntzinger, D. N., Schwalm, C. R., Wei, Y., Cook, R. B., Michalak, A. M., Schaefer, K., et al. (2018). NACP MsTMIP: Global 0.5-degree model outputs in standard format, version 1.0. Oak Ridge, Tennessee: ORNL Distributed Active Archive Center.
Huntzinger, D. N., Schwalm, C. R., Wei, Y., Shrestha, R., Cook, R. B., Michalak, A. M., et al. (2021). NACP MsTMIP: Global 0.5-degree model outputs in standard format, version 2.0. Oak Ridge, Tennessee: ORNL Distributed Active Archive Center.
Jiao, W., Wang, L., Smith, W. K., Chang, Q., Wang, H., and D'Odorico, P. (2021). Observed increasing water constraint on vegetation growth over the last three decades. Nat. Commun. 12 (1), 3777. doi:10.1038/s41467-021-24016-9
Knapp, A. K., Carroll, C. J. W., Denton, E. M., La Pierre, K. J., Collins, S. L., and Smith, M. D. (2015). Differential sensitivity to regional-scale drought in six central US grasslands. Oecologia 177 (4), 949–957. doi:10.1007/s00442-015-3233-6
Korner, C., and Basler, D. (2010). Phenology under global warming. Science 327 (5972), 1461–1462. doi:10.1126/science.1186473
Korner, C. (2018). Concepts in empirical plant ecology. Plant Ecol. Divers. 11 (4), 405–428. doi:10.1080/17550874.2018.1540021
Le Quere, C., Andrew, R. M., Canadell, J. G., Sitch, S., Korsbakken, J. I., Peters, G. P., et al. (2016). Global carbon budget 2016. Earth Syst. Sci. Data 8 (2), 605–649. doi:10.5194/essd-8-605-2016
Lemordant, L., Gentine, P., Swann, A. S., Cook, B. I., and Scheff, J. (2018). Critical impact of vegetation physiology on the continental hydrologic cycle in response to increasing CO2. Proc. Natl. Acad. Sci. U. S. A. 115 (16), 4093–4098. doi:10.1073/pnas.1720712115
Li, H., Wu, Y., Liu, S., and Xiao, J. (2021). Regional contributions to interannual variability of net primary production and climatic attributions. Agric. For. Meteorology 303, 108384. doi:10.1016/j.agrformet.2021.108384
Li, W., Migliavacca, M., Forkel, M., Denissen, J. M. C., Reichstein, M., Yang, H., et al. (2022). Widespread increasing vegetation sensitivity to soil moisture. Nat. Commun. 13 (1), 3959–9. doi:10.1038/s41467-022-31667-9
Lian, X., Piao, S. L., Li, L. Z. X., Li, Y., Huntingford, C., Ciais, P., et al. (2020). Summer soil drying exacerbated by earlier spring greening of northern vegetation. Sci. Adv. 6 (1), eaax0255. doi:10.1126/sciadv.aax0255
Liu, S., Wei, Y., Post, W. M., Cook, R. B., Schaefer, K., and Thornton, M. M. (2014). Data from: NACP MsTMIP: Unified North American soil map. Oak Ridge, Tennessee: ORNL Distributed Active Archive Center. doi:10.3334/ORNLDAAC/1242
Loboda, T. V., Giglio, L., Boschetti, L., and Justice, C. O. (2012). Regional fire monitoring and characterization using global NASA MODIS fire products in dry lands of Central Asia. Front. Earth Sci. 6 (2), 196–205. doi:10.1007/s11707-012-0313-3
Ma, X., Huete, A., Moran, S., Ponce-Campos, G., and Eamus, D. (2015). Abrupt shifts in phenology and vegetation productivity under climate extremes. J. Geophys. Research-Biogeosciences 120 (10), 2036–2052. doi:10.1002/2015jg003144
Martens, B., Miralles, D. G., Lievens, H., Fernandez-Prieto, D., and Verhoest, N. E. C. (2016). Improving terrestrial evaporation estimates over continental Australia through assimilation of SMOS soil moisture. Int. J. Appl. Earth Observation Geoinformation 48, 146–162. doi:10.1016/j.jag.2015.09.012
Martens, B., Miralles, D. G., Lievens, H., van der Schalie, R., de Jeu, R. A. M., Fernandez-Prieto, D., et al. (2017a). GLEAM v3: Satellite-based land evaporation and root-zone soil moisture. Geosci. Model. Dev. 10 (5), 1903–1925. doi:10.5194/gmd-10-1903-2017
Martens, B., Miralles, D. G., Lievens, H., van der Schalie, R., de Jeu, R. A. M., Férnandez-Prieto, D., et al. (2017b). GLEAM v3: Satellite-based land evaporation and root-zone soil moisture. Geosci. Model. Dev. 10 (5), 1903–1925. doi:10.5194/gmd-2016-162
Maurer, G. E., Hallmark, A. J., Brown, R. F., Sala, O. E., and Collins, S. L. (2020). Sensitivity of primary production to precipitation across the United States. Ecol. Lett. 23 (3), 527–536. doi:10.1111/ele.13455
Miralles, D. G., Holmes, T. R. H., De Jeu, R. A. M., Gash, J. H., Meesters, A. G. C. A., and Dolman, A. J. (2011). Global land-surface evaporation estimated from satellite-based observations. Hydrology Earth Syst. Sci. 15 (2), 453–469. doi:10.5194/hess-15-453-2011
Pan, Y. D., Birdsey, R. A., Fang, J. Y., Houghton, R., Kauppi, P. E., Kurz, W. A., et al. (2011). A large and persistent carbon sink in the world's forests. Science 333 (6045), 988–993. doi:10.1126/science.1201609
Papagiannopoulou, C., Miralles, D. G., Dorigo, W. A., Verhoest, N. E. C., Depoorter, M., and Waegeman, W. (2017). Vegetation anomalies caused by antecedent precipitation in most of the world. Environ. Res. Lett. 12 (7), 074016. doi:10.1088/1748-9326/aa7145
Propastin, P. A., Kappas, M., and Muratova, N. R. (2008). Inter-annual changes in vegetation activities and their relationship to temperature and precipitation in central Asia from 1982 to 2003. J. Environ. Inf. 12 (2), 75–87. doi:10.3808/jei.200800126
Qin, J. X., Duan, W. L., Chen, Y. N., Dukhovny, V. A., Sorokin, D., Li, Y. P., et al. (2022). Comprehensive evaluation and sustainable development of water-energy-food-ecology systems in Central Asia. Renew. Sustain. Energy Rev. 157, 112061. doi:10.1016/j.rser.2021.112061
Richardson, A. D., Keenan, T. F., Migliavacca, M., Ryu, Y., Sonnentag, O., and Toomey, M. (2013). Climate change, phenology, and phenological control of vegetation feedbacks to the climate system. Agric. For. Meteorology 169, 156–173. doi:10.1016/j.agrformet.2012.09.012
Satoh, Y., Yoshimura, K., Pokhrel, Y., Kim, H., Shiogama, H., Yokohata, T., et al. (2022). The timing of unprecedented hydrological drought under climate change. Nat. Commun. 13 (1), 3287. doi:10.1038/s41467-022-30729-2
Schenk, H. J., and Jackson, R. B. (2002). Rooting depths, lateral root spreads and below-ground/above-ground allometries of plants in water-limited ecosystems. J. Ecol. 90 (3), 480–494. doi:10.1046/j.1365-2745.2002.00682.x
Sherwood, S., and Fu, Q. (2014). A drier future? Science 343 (6172), 737–739. doi:10.1126/science.1247620
Tucker, C. J., Pinzon, J. E., Brown, M. E., Slayback, D. A., Pak, E. W., and Mahoney, R. (2005). An extended AVHRR 8-km NDVI dataset compatible with MODIS and SPOT vegetation NDVI data. International journal of remote sensing 26 (20), 4485–4498.
Turner, M. G., Calder, W. J., Cumming, G. S., Hughes, T. P., Jentsch, A., LaDeau, S. L., et al. (2020). Climate change, ecosystems and abrupt change: Science priorities. Philosophical Trans. R. Soc. B-Biological Sci. 375 (1794), 20190105. doi:10.1098/rstb.2019.0105
Vicente-Serrano, S. M., Gouveia, C., Camarero, J. J., Begueria, S., Trigo, R., Lopez-Moreno, J. I., et al. (2013). Response of vegetation to drought time-scales across global land biomes. Proc. Natl. Acad. Sci. U. S. A. 110 (1), 52–57. doi:10.1073/pnas.1207068110
Wang, J. M., Guan, X. D., Guan, Y. P., Zhu, K. W., Shi, R., Kong, X. N., et al. (2021). Changes in lengths of the four seasons over the drylands in the northern hemisphere midlatitudes. J. Clim. 34 (20), 8181–8190. doi:10.1175/Jcli-D-20-0774.1
Wang, L., D'Odorico, P., Evans, J. P., Eldridge, D. J., McCabe, M. F., Caylor, K. K., et al. (2012). Dryland ecohydrology and climate change: Critical issues and technical advances. Hydrology Earth Syst. Sci. 16 (8), 2585–2603. doi:10.5194/hess-16-2585-2012
Wang, Y. Q., Shao, M. A., and Liu, Z. P. (2013). Vertical distribution and influencing factors of soil water content within 21-m profile on the Chinese Loess Plateau. Geoderma 193, 300–310. doi:10.1016/j.geoderma.2012.10.011
Wei, Y., Liu, S., Huntzinger, D. N., Michalak, A. M., Viovy, N., Post, W. M., et al. (2014). Data from: NACP MsTMIP: Global and NorthNorth American driver data for multi-model Intercomparison. Oak Ridge, Tennessee: ORNL Distributed Active Archive Center. doi:10.3334/ORNLDAAC/1220
Wu, M. C., Manzoni, S., Vico, G., Bastos, A., de Vries, F. T., and Messori, G. (2022). Drought legacy in sub-seasonal vegetation state and sensitivity to climate over the northern hemisphere. Geophys. Res. Lett. 49 (15). doi:10.1029/2022GL098700
Wu, X., Liu, H., Li, X., Ciais, P., Babst, F., Guo, W., et al. (2018). Differentiating drought legacy effects on vegetation growth over the temperate Northern Hemisphere. Glob. Chang. Biol. 24 (1), 504–516. doi:10.1111/gcb.13920
Xu, C., Liu, H., Williams, A. P., Yin, Y., and Wu, X. (2016). Trends toward an earlier peak of the growing season in Northern Hemisphere mid-latitudes. Glob. Change Biol. 22 (8), 2852–2860. doi:10.1111/gcb.13224
Yang, D. W., Yang, Y. T., and Xia, J. (2021). Hydrological cycle and water resources in a changing world: A review. Geogr. Sustain. 2 (2), 115–122. doi:10.1016/j.geosus.2021.05.003
Yang, K., and He, J. (2019). Data from: China meteorological forcing dataset (1979-2018). National Tibetan Plateau Data Center, Beijing. doi:10.11888/AtmosphericPhysics.tpe.249369.file
Yang, K., He, J., Tang, W., Qin, J., and Cheng, C. C. K. (2010). On downward shortwave and longwave radiations over high altitude regions: Observation and modeling in the Tibetan Plateau. Agric. For. Meteorology 150 (1), 38–46. doi:10.1016/j.agrformet.2009.08.004
Yu, Z. X., Wang, T. Y., Wang, P., and Yu, J. J. (2022). The spatiotemporal response of vegetation changes to precipitation and soil moisture in drylands in the North temperate mid-latitudes. Remote Sens. 14 (15), 3511. doi:10.3390/rs14153511
Zhang, Y., Gentine, P., Luo, X., Lian, X., Liu, Y., Zhou, S., et al. (2022). Increasing sensitivity of dryland vegetation greenness to precipitation due to rising atmospheric CO2. Nat. Commun. 13 (1), 4875. doi:10.1038/s41467-022-32631-3
Zhao, F. B., Wu, Y. P., Yao, Y. Y., Sun, K., Zhang, X. S., Winowiecki, L., et al. (2020). Predicting the climate change impacts on water-carbon coupling cycles for a loess hilly-gully watershed. J. Hydrology 581, 124388. doi:10.1016/j.jhydrol.2019.124388
Zhao, F., Ma, S., and Wu, Y. (2021). Changes in dry-season water availability and attributions in the yellow river basin, China. Front. Environ. Sci. 9. doi:10.3389/fenvs.2021.762137
Zhao, F., Wang, X., Ma, S., Wu, Y., Qiu, L., Sun, P., et al. (2023). Widespread increasing ecosystem water limitation during the past three decades in the yellow river basin, China. J. Geophys. Res. Biogeosciences 128 (2). doi:10.1029/2022jg007140
Keywords: climate change, north hemisphere, sensitivity, soil moisture, vegetation growth, water availability
Citation: Wang X, Zhao F and Wu Y (2023) Increased response of vegetation to soil moisture in the northern hemisphere drylands. Front. Earth Sci. 11:1144410. doi: 10.3389/feart.2023.1144410
Received: 14 January 2023; Accepted: 13 February 2023;
Published: 01 March 2023.
Edited by:
Weili Duan, Xinjiang Institute of Ecology and Geography (CAS), ChinaReviewed by:
Qiang Li, Northwest A and F University, ChinaDengfeng Liu, Xi’an University of Technology, China
Copyright © 2023 Wang, Zhao and Wu. This is an open-access article distributed under the terms of the Creative Commons Attribution License (CC BY). The use, distribution or reproduction in other forums is permitted, provided the original author(s) and the copyright owner(s) are credited and that the original publication in this journal is cited, in accordance with accepted academic practice. No use, distribution or reproduction is permitted which does not comply with these terms.
*Correspondence: Fubo Zhao, emZ1Ym83ODlAMTYzLmNvbQ==; Yiping Wu, cm9ja3kueXB3dUBnbWFpbC5jb20=