- 1National Engineering Laboratory for Exploration and Development of Low Permeability Oil and Gas Fields, Xi’an, Shaanxi, China
- 2Research Institute of Exploration and Development of PetroChina Changqing Oilfield Company, Xi’an, Shaanxi, China
“Coal measure gas,” excluding tight (low-permeability) sandstone gas, refers in this study to the self-sourcing unconventional natural gas stored in coal, shale, and fine siltstone. It is characterized by various types of occurrences and reservoirs, a distinct cyclic succession of coal measure strata, widely distributed mosaic hydrocarbon accumulations, three types of coal measure gas accumulations, and configurations of vertically multiple-stacked gas-bearing reservoirs. Coal measure gas from Shan 2 can be divided—according to the grain size of sandstone, configurations of sandstone–coal–mudstone association, thickness, and layers—into four types: thick massive sandstone–coal–mudstone (type I), fine siltstone–coal–mudstone (type II), thin sandstone–mudstone–sandstone (type III), and coal–mudstone (type IV). Comprehensive evaluation suggests that the coal measure gas in the Suide-Mizhi area of the southern Ordos Basin presents an important and favorable accumulation option, characterized by coalbed methane, shale gas, and tight gas stored in sandstone–mudstone–coal strata. Therefore, it is essential to conduct full future assessments for the coal beds, shale, and tight gas controlling exploration for the “vertical gas-bearing zone” by three-dimensional exploration.
1 Introduction
The vision for a “beautiful China” and energy-structure adjustment have significantly increased the need for natural gas consumption, which relies on imported gas to satisfy energy demands. In 2019, China’s domestic annual gas production was 1777 × 108 m3 against gas imports of 1322 × 108 m3, with dependence on external gas rising to 43.1%. Domestic annual gas production is expected to reach 3200 × 108 m3 by 2030, with natural gas consumption at 5500 × 108 m3 offset by a dependence on external gas of 64% (Zou et al., 2018; Zou et al., 2019). The increasing demand for energy and the limited amount of gas produced in China makes the exploration and exploitation of coal measure gas imperative because it could be a larger contributor to annual gas production.
Exploration for coal measure gas dates back to the 1940s in the Eames River Basin, to the west of the Weser River in the northwestern basin of Germany (Shi et al., 1985). The Groningen coal measure gas field was first discovered in the northeastern West Holland Basin, with reserves of over one trillion cubic meters; gas fields relating to coal measures with high reserves had been since found in many coal-bearing basins of Asia, Europe, Oceania, and the Americas. Coal measure gas exploration in China began in the 1970s and was first systematically reviewed by Dai (1979)—a milestone in the domestic theoretical framework of coal measure gas. By 2016, 39 coal measure gas fields were discovered, accounting for 66% of large gas fields in China. By 1978, coal measure gas accounted for 9% of total reserves and 2.5% of the annual production of natural gas in China, with these figures rising to 58.7% and 61.5% in 2017, with total reserves and annual production of 92,538.51 × 108 m3 and 902.14 × 108 m3, respectively. China’s coal measure gas production is expected to exceed 1000 × 108 m3 in 2030 (Dai et al., 2014; Zou et al., 2019). The exploration and exploitation of coal measure gas promotes the energy security, social stability, and natural gas industry development of China.
The Upper Paleozoic coal measure strata of the Ordos Basin in Western China mainly occur in the Benxi Formation, Taiyuan Formation, and Shan 2 of the Shanxi Formation. The coal beds are interbedded with dark mudstone and tight sandstone, showing highly active gas logging. Coal measure gas resources in the Ordos Basin are estimated to be around 20 trillion square meters (Fu et al., 2016), showing huge exploration potential and becoming a new natural gas exploration frontier. Research and testing for the Upper Paleozoic coal measure gas in the Ordos Basin has been conducted in recent years by the Changqing Oilfield Company. The results show that the Ordos gas content is about 0.2–1.0 m3/t for shale, 0.1–0.5 m3/t for fine siltstone, and 6–10 m3/t for coal rocks. The vertical well of the Shanxi Formation has achieved gas production of 6366–6728 m3/d, and the horizontal well of Shan 2 has achieved gas production of 14126 m3/d, confirming that coal measure strata generally contain gas and have great exploration potential.
The objective of this article is to provide a comprehensive overview of the theoretical framework and exploration achievements for Upper Paleozoic coal measure gas in the Ordos Basin by discussing the definition, types, and geological characteristics of Shan 2 (Permian). By unifying several aspects of coal measure gas, this article evaluates these favorable accumulations and helps open up new exploration and exploitation for Shan 2, promoting the large-scale exploration and exploitation of coal measure gas in China’s marine continental alteration facies.
2 Characteristics of Upper Paleozoic coal measure gas in the Ordos Basin
2.1 Geological setting
The Ordos Basin spans the five provinces of Shaanxi, Gansu, Ningxia, Inner Mongolia, and Shanxi and is a large multiple cyclic superimposed cratonic basin with general subsidence, depression migration, and simple structure. It is divided into six tectonic units: the western thrust belt, Tianhuan depression, Yishan slope, western Shanxi flexural fold belt, Yimeng uplift, and Weibei uplift (Figure 1). Its proven gas reserves are prominently distributed in the Yishan slope. The study area is the eastern Ordos Basin, developing the Upper Carboniferous Benxi Formation with barrier island-tidal flat-lagoon facies, Lower Permian Taiyuan Formation with braided stream-delta-lagoon-tidal flat-barrier island-shallow marine shelf carbonate deposits, and the Shanxi Formation with delta-tidal depositional facies. The Shanxi Formation has more stable, continuous, and thicker shale layers than the Benxi and Taiyuan formations, which is a favorable stratum for coal measure gas exploration. Shan 2 of the Shanxi Formation has high organic matter abundance and strong hydrocarbon generation ability, which is the target stratum of coal measure gas exploration in this study.
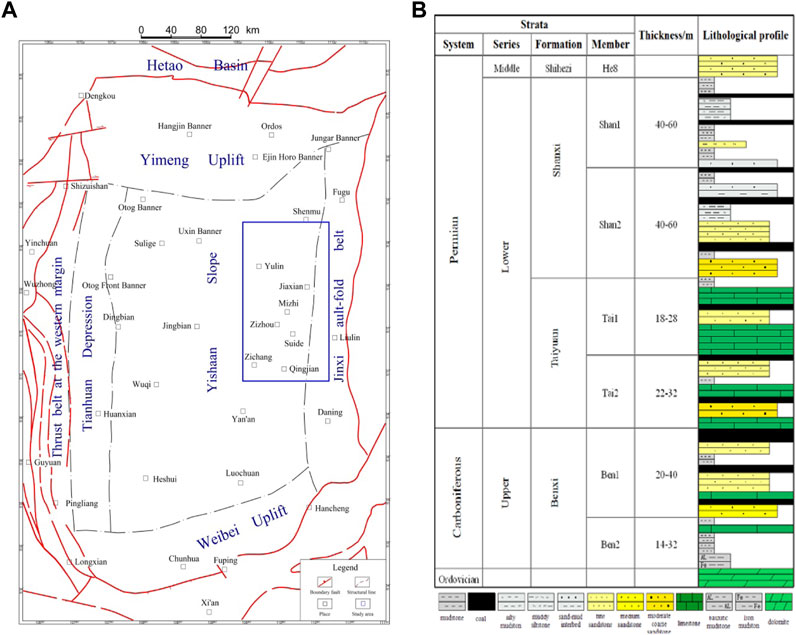
FIGURE 1. Tectonic unit division (A) and stratigraphic column (B) of the Carboniferous–Lower Permian in the Ordos Basin.
2.2 Definition of coal measure gas
“Coal measure gas,” also termed “coal-derived gas,” refers to natural gas related to coal measures. As defined by Dai (1982), coal-derived gas was generated by humic organic matter in the coal formation process. With the development of exploration and exploitation, many researchers are trying to give different definitions from the viewpoint of accumulation dynamics. However, due to the multiple storage spaces of natural gas in coal measures and varying perspectives studied by different researchers, the problem of defining coal measure gas has led to misconceptions that have, in some cases, impeded exploration efforts. Wang et al. (2014) proposed that coal measure gas was sourced from organic matter contained in coal measures during the processes of biochemistry, physical chemistry, and coalification from the perspectives of geology and deposit economy, including shale gas in coal measure shale, coalbed methane in coal beds, tight (low-permeability) gas in coal measure sandstone, and gas hydrates. Jiang et al. (2015) took Taohua mudstone as the boundary, defining the Upper Paleozoic Benxi Formation, Taiyuan Formation, Shanxi Formation, and lower Shihezi Formation coal-bearing strata in the Ordos Basin as coal measures, and the gas in them as coal measure gas. Ouyang et al. (2018) suggested that coal measure gas referred to coalbed methane from coal beds and gas from adjacent tight sandstone reservoirs; this was an economic definition. More recently, the term “coal measure gas” has been defined as stranded coalbed methane and shale gas from coal measure source rocks, such as coal and carbonaceous and dark shales, and accumulated as self-sourcing or outside-sourcing reservoirs; it can be subdivided into unconventional continuous “coalbed methane,” “shale gas,” “tight gas,” and conventional “trapped accumulations” (Zou et al., 2019). Three large coal-measure tight sandstone gas fields (Daniudi, Yulin, and Zizhou) were discovered in the eastern Ordos Basin, with proven geological reserves of over 1000×108 m3. The reservoir rocks of the main reserves of Shan 2 are composed of medium-coarse to coarse quartz sandstone and lithic sandstone (Zhao et al., 2013), with pore throat structure of mainly intergranular pores and medium-coarse throats (>0.2 μm) in the Yulin gas fields, and residual intergranular pores and thin throats in the Zizhou gas fields (Lan et al., 2007; Wang et al., 2015). However, comprehensive gas potential evaluation is needed for the siltstone interbedded with thin mudstone in the eastern Ordos Basin, characterized by and interbedded fractures under argon ion light-field emission electron microscopy and nano-sized pores and throats. The coal measure gas in this paper, excluding the coal measure tight sandstone gas in current exploration and exploitation, specifically refers to the unconventional self-sourcing natural gas stored in coal beds, shale, and fine siltstone.
2.3 Shan 2 coal measure gas types in Ordos basin
Coal measure gas is mainly stored in coal seams and the sand-mudstone partings that can be evaluated and extracted together, which can vertically expand exploration and exploitation space and increase resource abundance. Varying assemblages of coal seams and sand-mudstones lead to different coal measure gas reservoir types and mining strategies. In the context of the configurations between coal beds and adjacent sandstones defined by Ouyang et al. (2018), there are four combination relationships of coal measure gas: multi-layer coal and sandstones (type I), multi-layer coal and less layer or no sandstones (type II), monolayer/less layer coal and multi-layer sandstones (type III), as well as monolayer/less layer or no coal and less layer or no sandstones (type IV). In this article, four types of coal measure gas (Figure 2) are identified according to sandstone grain size, configurations of sandstone-coal-mudstone, thickness, and seams. Type I has already been economically exploited, while the remaining types are prioritized currently.
Type I is thick massive sandstone-coal-mudstone characterized by developed thick massive sandstone bodies that are coarse quartz sandstone and lithic quartz sandstone, which have good physical properties, porosity of more than 7%, permeability of more than 0.5 °mD. Hydrocarbon gas generated from coal beds, the mainly gas source rocks, is directly stored in the neighboring sandstones, forming the source-reservoir interactive coal measure gas accumulations in a combination with favorable regional seal rocks.
Type II is interbedded fine siltstone-coal-mudstone characterized by multiple assemblages of interactive, vertically cyclic superposition sandstone and coal beds, reflecting cyclical coal-forming environments (cyclothems). The sandstone is fine lithic quartz sandstone and lithic sandstone with tight structure, with porosity of 5%-7% and permeability of 0.1-0.5°mD. Coal beds can be considered as both source rocks and reservoirs, hydrocarbon gas from which is stored in the nearby sandstone, forming the combination of tight sandstone gas and coalbed methane accumulations with favorable regional seal rocks.
Type III is interbedded thin sandstone-mudstone-coal. It is marked by developed coal beds and undeveloped sandstones, indicative of a marsh depositional environment. Such type has developed mudstone and lack of terrigenous clasts, which are favorable factors equipped with regional cap rocks resulting in coalbed methane and shale gases accumulations.
Type IV is interbedded coal-mudstone, implying a deep-water sedimentary environment. It is marked by thick mudstones, thin coal beds, and undeveloped sandstone bodies, with a favorable sealing condition, supporting the formation of single coalbed methane and shale gas accumulations.
3 Geological characteristics of coal measure gas
Coal measure is a set of co-genetic coal beds or coal line-bearing sedimentary deposits formed by marine continental alteration or continental settings, occurring in residual basins with different structural properties. Coal measure gas is focused on both the genetic correlations of various reservoirs and the differences between various types of reservoirs and accumulations that result from the geological factors of coal measure gas.
3.1 Diversities of storage and reservoir
Coal measure gas occurs in three states: coal methane as absorbed gas, coal measure tight sandstone gas as free gas, and shale gas as mixed state. The abundance of organic matter gradually increases from inorganic reservoirs (sandstones) through mixed reservoirs (shale) to organic reservoirs (coal beds), forming the reservoir lithological sequence without boundary; this is the fundamental reason for the state diversities of coal measure gas that account for different mechanisms and exploitation strategies. There are many types of coal measure source rocks, such as coal beds, carbonaceous mudstone, and dark mudstone, with high accumulative thickness, high gas-generating intensity, and sufficient gas source, resulting in abundant coal measure gas resources. Three layers of coal seams at a depth of 1000–2000 m with 100 m intervals were distributed in the Fenghe Basin of Wyoming, United States, the coalbed methane and sandstone gas of which were exploited at different pressures, with coal bed–sandstones tens to hundreds of meters thick exploited simultaneously. Here, 20 vertical wells had a daily gas production of thousands of cubic meters, with a peak of 20×104 m3. In 2001–2002, a pilot test of coalbed methane and tight sandstone gas co-production was conducted in the Baihe uplift of the Pitsens Basin, United States. Coal beds were at depths of 1560–2561 m, and 65 wells targeting deep coalbed methane and tight sandstone gas were exploited simultaneously. The test showed that average daily gas production was about 1.09×104 m3, with a peak of 1.44×104 m3—60% of which was generated from coal beds (Fu et al., 2016). In 2017, a breakthrough was made in the vertical well exploitation test of coalbed methane in the daily gas production of Yangmeican 1 well, Guizhou Province, China, with target layers of coal beds, carbonaceous mudstone, and tight sandstone reaching 4656 m3 and were maintained above 3600 m3/d for 50 days—the highest record for vertical well gas production in southwest China. The resource abundance of Yangmeishu syncline coal measure gas where the well is located 4.79×108 m3/km2 is six times higher than that of pure coalbed methane. The modeling experiment shows that the exhaustion of coal rocks is very efficient compared with marine source rocks, reaching 75% at the maximum vitrinite reflectance of 1.0% and 90% at 5.5%. This indicates that the natural gas generated from coal is mainly injected into other reservoirs and is the major source of coal measure gas and adjacent non-coal measure gas accumulations.
3.2 Distinct cyclicity of coal measures’ depositional sequence
Coal measures are deposited in continental and continental and marine alteration settings, the lithology and thickness of which are controlled by geological processes; they are characterized as thin layers and various lithologies, interbedded sandstone–mudstone–coal beds, and highly cyclicity. Such cyclicity results in both variations of coal measure gas reservoirs—leading to diversities of occurrence state—and different associations of source–reservoir–cap with various structures, moderate thickness, and multiple internal sealings that are vertically controlled by stratigraphic sequence. This results in the vertical development of several fluid pressure systems and complex gas–water correlations in the coal measure. Different fluid pressure systems in coal measures are close to each other, having a fragile dynamic energy balance and being vulnerable to disturbance by exploitation. Various correlations of associations of source–reservoir–cap lead to the same layers (such as coal beds and shale) having the functions of source rocks, reservoirs, and cap, and to the gas having the nature of self-sourcing reservoirs and outside-sourcing reservoirs—the depositional factor for the varieties of coal measure gas. The variable source–reservoir–cap combination leads to the same rock layer (such as coal seam or shale rock) having the functions of source rock, reservoir, and cap rock, resulting in natural gas in the same combination having both the characteristics of self-generation and self-storage; it is the sedimentary control factor of the types of diversity of coal measure gas reservoirs. The vertical combination of coal rock and sandstone determines the migration and accumulation mode of coal measure strata, including in situ accumulation with “source–reservoir contact” and long-distance accumulation controlled by reservoir and migration channels. The variations in reservoirs of coal measures lead to distinct differences in the mechanical properties of lithology reservoirs, which encourages effective and uniform exploitation, technical innovation, and reservoir reconstruction. The Surat Basin in Australia has recently been the most successful coal measure gas development basin in the world, with average gas production per well of 2.83–5.66×104 m3/d with a peak of 56×104 m3/d. The depositional cyclicity of the Middle Jurassic Wallon sub-group coal measure is noticeable, developing fining-upward fluvial deposition, strata thickness of 305–365 m, more than 100 coal-bearing layers (average 40 layers), and over 70% of single-layer coal beds with thicknesses of less than 1.0 m. It is inferred that thin coal beds are frequently interbedded with sandstone, carbonaceous mudstone, and mudstone, thus improving the permeability of coal-bearing layers with 5–5000 mD, designing vertical well and open hole completion technology through the whole coal measures can realize the efficient mining of coal measures gas. to effectively achieve commingling production. The varying thickness ratios of coal beds and sandstone imply that the dominant thin coal layers lead to much more frequent interbedding, thus favoring the preservation of coal measure gas, dehydration of coal beds, and coalbed methane desorption.
3.3 Characteristics of widely distributed and mosaic hydrocarbon accumulation
Coal measure is formed in a diversity of depositional settings, including alluvial fans, river–lakes, deltas, and lagoon-tidal flats. Weak hydrodynamic deposition dominated by shallow marine carbonate rocks and lagoon-tidal flat and lake mudstones occurs widely in high-stand system tracts. Strong hydrodynamic deposition dominating alluvial fan, river, and delta sandstones occurs in low-stand system tracts, while sandstone has good lateral continuity in the direction of the palaeocurrent but poor continuity in the vertical and oblique palaeocurrent direction, showing quasi-strata and lenticular shapes in the profile. The transformation stages of the two-system domains are more conducive to the wide development of weak hydrodynamic peat bogs, forming large-scale comparable main coal beds. These geometric characteristics display widely distributed mud-coated sand, mud-coated coal, mudstone, and coal beds, with three-dimensional distribution patterns of single sandstone in the mosaic of coal measure that produce mudstone and coal beds in the sediment facies change. Coal measure source rocks are superimposed with gas reservoirs, proximal source accumulations, interbedding, or even the same layers of source rocks and reservoirs, which are the contributing privileges for accumulations. In addition, the source rocks, coal reservoirs, and shale reservoirs in coal measures are distributed widely, while the sandstone reservoirs have limited distribution, but the wall rocks have strong ability in sandstone reservoirs. Such factors are of crucial importance for coal measure strata accumulation, such that gas accumulations that dominantly occur in coal measures are characterized by coal measure source rocks with small-scale segmentation and many low abundance gas fields. Furthermore, the distribution and abundance of natural gas are controlled by zones with good physical properties of heterogeneous contiguous sandstone, which is the basic geological factor as coal measure gas is a dominantly lithological gas reservoir.
3.4 Characteristics of three coal gas accumulation patterns
The reason why coal measure sandstone densifies is that sediments are enriched in plastic components, organic matter, and organic acids that lead to the accumulation process of “first densification and then charging” of coal measure sandstone. Current research and geological knowledge suggest that coal measure sandstone contains a certain amount of carbonaceous material, and organic detrital variations also affect sandstone gas accumulations. Sedimentation is the basic factor of reservoir densification controlling the original porosity features. The tight sandstone reservoir depositional settings show some common features: slow sedimentary rate, weak and steady hydrodynamic conditions, dominantly coastal zone facies relating to delta deposition, distinct sandy mudstone interbedded with coal beds, a high content of primary clay interstitial material, and lithic fragments and feldspars which easily diagenetically transform and that are not conducive to primary pore preservation. Upper Paleozoic coal measure sandstone in the northeast margin of Ordos Basin is dominated by quartz and lithic sandstones, with lithic content of 5%–50% and feldspar content of less than 25%; and the clay minerals are a significant cement and are mainly illite, kaolinite, and chlorite, with content of 5%–15% up to 35%. Considering the extent of diagenetic influence, the tight sandstone reservoirs can be divided into three types: compaction, cementation, and other origins. Mechanical compaction is the most important factor in the early diagenetic stage of induced sandstone densification, contributing to porosity decrease in the whole diagenetic process. The organic origin of sandstone densification has attracted much research attention in recent years. Large amounts of organic acids produced by the hydrocarbon generation process of coal measure source rocks enter the adjacent sandstone, and the acid diagenetic fluid environment leads to a lack of carbonate mineral cementation, which mainly forms the secondary increase of quartz particle silica precipitation, the loss of primary pores, and the densification of sandstone. In the late diagenetic stage, organic acid setting leads to distinct silica and kaolinite cementation under relatively high formation temperature and pressure, and the increase of PH values of formation fluids leads to the formation of late carbonate cementation, the further loss of residual pores, and sandstone densification. The volume of CO2 produced in the organic matter generation process of coal measure gas can reach 50%–70% of that of hydrocarbon gas, which is much higher than the current CO2 content of coal measure gas (<5%); large amounts of missing gaseous CO2 are deposited in the reservoir pores in the form of solid carbonate, and carbonate cement of 0.32 m3 can be formed by the complete conversion of 1 m3 CO2 under ideal conditions. The dynamic process of hydrocarbon gas and CO2 of coal is not synchronous, and two periods of CO2-concentrated generation exist in weak and high-mature diagenetic processes, which differ from multiple large-scale hydrocarbon gas generation. The gas generation behavior of coal measure source rocks leads the sandstone reservoir to experience serious densification in the early diagenetic stage and the coal measure strata having a pattern of “first densification and then accumulation.” The depositional site of coal measure sandstone is adjacent to that of enriched organic materials, and the early-formed organic-rich sediments are scoured by syndiagenesis or pene-contemporaneous channel, forming the preserved organic detritals mixed in the sandy sediments. Therefore, the most important lithological feature of coal measure sandstone is that it has a relatively high content of organic detritals. The gas-bearing tight sandstone of coal measures contains many coal cuttings or carbonaceous mudstone particles, the majority of which can account for 30%–40% of the view area, and the minority of which is more than 5%—generally between 10% and 20%. The organic detritals obviously affect the physical properties of sandstone. On the one hand, organic detrital increases the pore surface area of the sandstone reservoir, and the pore surface area increases 600 times when 1 m3 coal breaks into organic detritals with a diameter of 1 mm. On the other hand, the organic detrital contents and the porosity and permeability of sandstone increase. It is thus inferred that coal measure sandstone gas has the accumulation characteristics of partially self-sourcing reservoirs and has a gas production mode and pumping and producing system that differs from those of conventional sandstone reservoirs. In addition, organic detritals improve, to some extent, the physical properties of tight sandstone reservoirs, and so the related exploitation, reconstruction, and gas-producing methods must be adjusted.
Coal measure gas has complicated source–reservoir relationships, and specific carrier systems reallocate the natural gas generated by source rocks, which is crucially important to co-accumulation for coal measure gas. Tight sandstone has no privilege of migration pathways and mainly migrates diffusely. Crevasse splay sandstone has a “chimney” effect on the hydrocarbon expulsion and subsequent migration to the sandstone reservoir. Natural gas in coal measure lacks large-scale lateral migration conditions, but migration in the form of episodic inrush flow driven by abnormal pressure shows vertical and short distance rapid accumulation. The coal measure free gas in the Qinshui Basin migrates in two ways: 1) a macroscopic transport system, including fault, unconformity surface, and collapse column; and 2) a micro-transport system, including micro-pores and micro-cracks of coal beds and roof sandstone. In the Zhengzhuang–Fanzhuang region, the system is micro-transport that is composed of fault, extensive coal beds “layered evaporative” plane hydrocarbon expulsion, and collapse column, which forms three coal measure gas accumulation patterns of primary, adjusted, and reformed type. A similar material modeling experiment shows that the efficiency, rate, and gas saturation of natural gas is controlled by sandstone permeability contrast. The greater the permeability heterogeneity, the greater the effective accumulation potential of coal measure strata. The distributive channel and other sandstone with good physical properties are confined by sandstone or mudstone with poor physical properties, which is conducive to the effective accumulation and preservation of natural gas. The macroscopic occurrence of a coal measure displays a huge “box,” such as the large Daniudi tight gas field in the northern Ordos Basin, which is sealed by both regional cap physical properties and abnormal pressure. The industrial gas reservoir is strictly confined in the box, superimposed with multiple large lithological traps, and consists of a “near source box type” accumulation pattern. Such a pattern has dominant source-controlling, reservoir-controlling, high-pressure sealing, and near-source accumulation geological configuration characteristics. The structure is not the reservoir-controlling factor. The breakthrough of coal measure gas exploitation in the Hangjinqi area on the northern margin of the Ordos Basin is a typical case of such accumulation. By analyzing the configuration relationship between the enrichment of coal measure gas and the sealed layer, the adjustment of the later structure, and formation occurrence, it is evident that coalbed methane is apt to accumulate in the regional mudstone cap and floor layers, and areas where it has little tectonic uplift, with smooth and equilibrium state formation occurring after the accumulation stage. We developed a concept of a closed system containing not only enriched coalbed methane but also other types of coal measure gas.
3.5 Multiple vertical gas-bearing combination features
The frequent interbedding of different lithologies in a coal measure inevitably leads to the repeated occurrence of water and gas-blocking layers or inner sealing layers in a longitudinal direction, which then forms an independent gas-bearing system in different layers of a coal measure. A superimposed gas-bearing system is defined as two or more independent gas-bearing systems overlapping each other vertically in the same coal measure. The common phenomenon that different coal measure layers in the same well have a different fluid pressure coefficient is actually the concrete manifestation of the superimposed gas-bearing system. The difference between reservoir physics and the fluid energy of a superposition gas-bearing system is that the coal measure gas co-production process shows inter-system interference and complicated geological conditions, thus constraining the full release of coal measure gas productivity.
The sequence stratigraphic framework is the physical basis for the formation of a superimposed gas-bearing system. The lack of hydrodynamic connection between a coal measure and adjacent water-bearing layers constitutes the hydrogeological basis of the system. The superimposed gas-bearing system is the product of sediment-hydrogeology-structure conditions that control the system. A superimposed gas-bearing system is controlled by depositional setting and the related sequence stratigraphic structure. Coal measures with fluvial-delta-lacustrine facies vertically display a “unified gas-bearing system,” while coal measures with “delta-tidal flat-lagoon” facies can form “superimposed gas-bearing systems”; the sequence stratigraphic structure controlling the gas bearing and physical properties of the coal measure reservoir. Low permeability strata deposited near the maximum flood surface and associated with marine mudstone have a partition barrier effect on the vertical flow of coalbed methane, which is considered the fundamental geological reason for the formation of superimposed gas-bearing systems. Such low-permeability rocks are defined as a “key layer,” which gradually develops from the delta plain facies to the delta front facies, leading to the vertical structure and superposition of the gas-bearing system becoming more complex and evident.
4 The exploration potential of coal measure gas
Coalbed methane, shale gas, and fine sandstone gas reservoirs have different controlling factors for accumulation, which determine how different favorable gas zones have different controlling factors. Therefore, it is essential to consider different parameters to select favorable zones.
4.1 Coalbed methane favorable zone
The most direct and crucial parameters for target selection and evaluation of coalbed methane are thickness, gas-bearing contents, resource abundance, adsorption saturation, permeability, and coal-bed depth (Wang et al., 2006; Tian et al., 2010); these are important to the recoverability and economy of coalbed methane. The coalbed methane of Shan 2 has high thermal maturity with Ro generally higher than 1.6%, which is in a high coal rank. The cumulative thickness of coal beds is 6–10 m, with a high range of 7 m3/t−20 m3/t gas content. The coalbed methane abundance in the Daning-Jixian region adjacent to the study area is 2.85 × 108 m3/km2, and the adsorption saturation of coalbed methane wells with daily gas production of more than 1000 m3 is greater than 50% (Tian et al., 2010). The coal beds in the currently exploited coalbed methane blocks are mainly buried at a shallow depth of 1300 m, while the coal bed of Shan 2 in the study area has a high depth of 1900–2400 m and low permeability of almost lower than 0.5 mD, which is medium-to low-permeability coal. The practice of coalbed methane exploration and development shows that the single coalbed layer is more than 3 m thick, and the coalbed methane reservoir has good resource potential when the accumulative thickness is over 10 m and commercial development value when the coalbed methane has content of over 6 m3/t and daily gas production of over 1000 m3. Research shows that, worldwide, coalbed methane resources are dominantly distributed in coal beds with depths of 1500 m (Kuuskraa and Wyman, 1993). A new round of coalbed methane resource evaluation in China suggests that the coalbed methane resource is 30×1012 m3 at a depth of 1500–3000 m, accounting for 54.5% of the total resource, with abundant deep coalbed methane resource (Li et al., 2016). As a consequence, according to the criteria of thickness, gas-bearing concentration, resource abundance, and adsorption saturation of high-rank coalbed methane proposed by Wang et al. (2006), we determined the evaluation parameter of the favorable zone (Table 1) and selected the favorable coalbed methane zones of Shan 2 (Figure 5). We thus show that the favorable zones of coalbed methane are distributed in the Yulin-Suide region, and the thick coal beds distribute zonally in a N–S trend. Although coal is developed in the south and east of the region, the single layer has a negligible thickness and thus little exploration and development potential as coalbed methane. In addition, in the current economic and technical conditions, it is economically difficult to separately exploit coalbed methane because of its high burial depth, high drilling cost, and low permeability.
4.2 Favorable zones of shale gas
The critical parameters for shale gas zone evolution include organic content, maturity, thickness, gas-bearing content, physics, and brittle mineral content (Li et al., 2011; Wang et al., 2013). The shale of the Shan 2 study area has a wide range of TOC of 0.08%–37.11% (average 2.0%), while the Shanxi Formation has Ro ranging 1.45%–2.37% (average 1.89%), suggesting that the organic maturity of the target shale layer in the high maturity stage is higher than the lowest standard (Ro = 1.1%) of commercial exploitation of shale gas, which is beneficial to the formation of shale gas accumulation. The thickness of a single layer of shale gas reservoir is generally less than 10 m, but its cumulative thickness is generally greater than 30 m; therefore, the shale gas of Shan 2 has exploitation potential. The field test showed that the shale has low gas content, varying 0.19 m3/t−2.16 m3/t (average 0.64 m3/t), and the adsorption experiment implies that the Shanxi Formation shale has a high absorbed gas content of 0.56 m3/t−15.28 m3/t (average 3.28 m3/t). This demonstrates that the Shanxi Formation has a high potential for absorbed gas content. The Shan 2 shale has a permeability of 0.0019 mD−0.317 mD (average 0.0415 mD)—higher than that of commercial shale gas reservoirs with lowest values of 0.00001 mD—and has low porosity ranging 0.2%–4.7% (average 1.7%)—lower than that of commercial shale gas reservoir with lowest values of 2%. Brittle minerals include quartz, feldspar, and carbonates (calcite, siderite, and ferridolomite,) and pyrite with contents of 10%–71% (average 49.9%), while the clay minerals have relatively high contents of 29%–90% (50.1%), which has an adverse impact on reservoir fracturing.
Considering the commercial exploitation quantitative technical indicators of shale gas in the current technical conditions, we determined the evaluation parameter of the favorable zone (Table 2), and the favorable zone distribution of Shan 2 shale gas (Figure 5) is in the south of the Jiaxian region.
4.3 Favorable zone of fine siltstone gas
Shan 2 fine siltstone is tight and so has worse properties than those of medium-coarse sandstones, but has similar key factors of accumulation, which determine that the key parameters of the favorable area are reservoir thickness, physical properties, and gas content. The thickness of the Shan 2 siltstone reservoir is controlled by depositional micro-facies types, including a delta plain distributary channel flanking zone, delta front underwater distributary channel flanking zone, mouth bar far end, and shore shallow lake bar. There are limited studies on the lowest thickness of sandstone gas, gas testing, and core physical property analysis, considering the possible co-production of such sandstone and other reservoirs; we define the lower range of sandstone thickness as 1 m. Because the good reservoir physical properties of Shan 2 fine siltstone are lithic quartz sandstone, we determine for physical criteria that porosity is greater than 3.5%, permeability is more than 0.1 mD, and the lower limit of gas saturation is 55%, based on the previous studies (Table 3). Therefore, this study selects the favorable zone of Shan 2 siltstone gas reservoir that is zonally distributed in the southern Jiaxian region of N–S trending, with a small single layer thickness and distribution area.
4.4 Favorable zone of coal measure gas
The coupling accumulation of coal measure gas is facilitated by the high content of organic matter in coal-bearing rock series, the strong cycle, the overlapping of reservoir space, and the difference in the development of reservoir pore structure. In the Shanxi formation of the Ordos Basin, the transitional phase of marine and continental coal measure source rocks are mainly gas generation, and this formation features thin interbedded development with fine sand. Therefore, its hydrocarbon expulsion efficiency is high, organic matter nanopores are not developed, and the gas from the coal seam and coal measure shale rock, as the source rock, can accumulate and form tight coal measure sandstone gas in the adjacent coal measure sandstone layer. In the formation of vertical three-dimensional gas geological characteristics, each reservoir space overlap formed a natural cap conducive to the preservation of coal measures gas (Figure 3).
Due to the low content of brittle minerals in mudstone and coal seam are difficult to reform in the coal measures of the Shan 2 member, it is difficult to reform. Given the low gas content of fine silty sand rock, it is difficult to industrially develop a single type of coalbed methane, mud shale gas, and tight sandstone gas in coal measures. Therefore, considering coal measure gas as a whole and simultaneously using a single well to conduct integrated exploration and development of coalbed methane gas, mud shale gas, and tight sandstone gas would not only reduce the exploration and development cost of unconventional natural gas but also improve the use efficiency and profit of a gas well, as well as production life. The combination of sand and coal in the study area forms a V-shaped curve (Figure 4) with both free gas and adsorbed gas, which can accommodate both large and micro-pores, thus achieving high production by fracturing horizontal or highly deviated wells. According to the comprehensive evaluation, sand and peat interbedding developed in the south; through the superposition of coalbed methane, shale gas, and tight gas favorable areas, it is believed that there is a favorable area of coal measure gas accumulation in the Suide-Mizhi area in the south (Figure 5), with an area of 3,265.4 km2 and predicted resources of 322.821 billion square meters.
5 Conclusion
“Coal measure gas,” excluding tight sandstone gas, refers in this study to self-sourcing unconventional natural gas stored by coal, shale, and fine siltstone, characterized by various reservoir and accumulation modes, distinct cyclical coal measure sedimentary sequences, widely distributed and mosaic hydrocarbon accumulation, the three coal gas accumulations, and multiple configurations of vertical gas-bearing reservoirs. Based on the grain-size distribution of sandstone, configurations of sandstone-coal-mudstone association, and strata thickness and layers, coal measure gas from Shan 2 can be divided into four types: thick massive sandstone-coal-mudstone (type I), fine siltstone-coal-mudstone (type II), thin sandstone-mudstone-sandstone (type III), and coal-mudstone (type IV). Type I has already been economically exploited, while the remaining types are currently prioritized.
The favorable coalbed methane area is mainly distributed in the Yulin-Suide area, and the thick coal seam is zonally distributed from south to north. The favorable area of mountain shale gas is distributed in the south of Jiashan County. The favorable areas of silty sand gas reservoir are mainly distributed in the south of Jiaxian. The comprehensive evaluation has shown that sand and peat interbedding developed in the south, and favorable areas of coalbed methane, shale gas, and tight gas are superimposed in the Suide-Mizhi area in the south. The comprehensive evaluation of coal seam, shale, and tight gas should be strengthened in exploration, and three-dimensional exploration should be conducted based on the vertical gas bearing zone.
Data availability statement
The datasets presented in this study can be found in online repositories. The names of the repository/repositories and accession number(s) can be found in the article/supplementary material.
Author contributions
W-BZ wrote the full text, HZ and Y-HS were responsible for the relevant experimental operation and appropriate relevant data, X-XF and JH drew the figure, and X-YJ completed the statistical data.
Funding
This research was supported by “Research on strategy and evaluation technology of new area and new strata and new field of coal-bed methane” (Grant No. 2021DJ2303) and “Study on sweet spots evaluation and yield-increasing mechanism of Upper Paleozoic coal-bed methane in central and eastern Ordos Basin” (Grant No. 2022D-JB01).
Conflict of interest
Authors W-BZ, HZ, Y-HS, X-XF, JH, and X-YJ were employed by the Research Institute of Exploration and Development of PetroChina Changqing Oilfield Company.
Publisher’s note
All claims expressed in this article are solely those of the authors and do not necessarily represent those of their affiliated organizations, or those of the publisher, the editors, and the reviewers. Any product that may be evaluated in this article, or claim that may be made by its manufacturer, is not guaranteed or endorsed by the publisher.
References
Dai, J. X. (1979). Natural gas and oil formation in coalification processes. Petroleum Exploitation Dev. 6 (3), 10–17.
Dai, J. X., Ni, Y. Y., Huang, S. P., Liao, F. R., Yu, C., Gong, D. Y., et al. (2014). Significant function of coal-derived gas study for natural gas industry development in China. Nat. Gas. Geosci. 25 (1), 1–22. doi:10.11764/j.issn.1672-1926.2014.01.0001
Fu, X. H., Deleqiati, J., Zhu, Y. M., Shen, J., and Li, G. (2016). Resources characteristics and separated reservoirs’ drainage of unconventional gas in coal measures. Earth Sci. Front. 23 (3), 36–40. doi:10.13745/j.esf.2016.03.005
Jiang, T., Liu, Z. Y., Wang, T., and Song, H. Z. (2015). Discussion on upper paleozoic coal measures gas reservoiring mechanism in northeastern Ordos Basin. Coal Geol. China 27 (7), 43–47. doi:10.3969/j.issn.1674-1803.2015.07.10
Kuuskraa, V. A., and Wyman, R. E. (1993). Deep coal seams: An overlooked source for long-term natural gas supplies. Gas technology symposium. Soc. Petroleum Eng. 26196, 587–596.
Lan, C. L., He, S. L., Men, C. Q., Zhang, J. F., and Lan, Y. F. (2007). A study of deliverability variations in low-pressure, low-permeability reservoirs: Paleozoic reservoir in Ordos basin. Nat. Gas. Ind. 27 (12), 99–101.
Li, X. Z., Wang, Y. H., Jiang, Z. C., Chen, Z. L., Wang, L. Z., and Wu, Q. (2016). Progress and study on exploration and production for deep coalbed methane. J. China Coal Soc. 41 (1), 24–31. doi:10.13225/j.cnki.jccs.2015.9003
Li, Y. J., Liu, H., Liu, J. X., Cao, L. C., and Jia, X. C. (2011). Geological regional selection and an evaluation method of resource potential of shale gas. J. Southwest Petroleum Univ. 33 (2), 28–34. doi:10.3863/j.issn.1674-5086.2011.02.004
Ouyang, Y. L., Tian, W. G., Sun, B., Wang, B., Qi, L., Sun, Q. P., et al. (2018). Characteristics of coal measure gas accumulation and such gas exploration strategies in China. Nat. Gas. Ind. 38 (3), 15–23. doi:10.3787/j.issn.1000-0976.2018.03.002
Shi, X. Z., Dai, J. X., Wang, Z. M., Zhu, J. W., and Liu, J. Q. (1985). A study of carbon isotope of coal-formed gas in FRG and its inspiration to us. Nat. Gas. Ind. 5 (2), 1–9.
Tian, W. G., Li, W. Z., and Sun, B. (2010). “Preliminary study on the evaluation parameter standards of Chinese coal-bed gas constituency,” in Theory and technology of coalbed methane exploration and development. Editors F. J. Sun, S. L. Feng, Q. B. Zhao, B. A. Xian, W. Z. Li, and J. P. Ye (Beijing: Petroleum Industry Press), 25–31.
Wang, R. G., Li, W. H., Liao, Y. Y., Feng, Y. J., Guo, Y. Q., Ma, Y., et al. (2015). Study on the characteristics of the upper paleozoic Shanxi and xiashihezi formation in Zizhou gas field, Ordos basin. Chin. J. Geol. 50 (1), 249–261. doi:10.3969/j.issn.0563-5020.2015.01.16
Wang, S. Q., Wang, S. Y., Man, L., Dong, D. Z., and Wang, Y. M. (2013). Appraisal method and key parameters for screening shale gas play. J. Chengdu Univ. Technol. 40 (6), 609–620. doi:10.3969/j.issn.1671-9727.2013.06.01
Wang, T., Wang, Q. W., and Fu, X. H. (2014). The significance and the systematic research of the unconventional gas in coal measures. Coal Geol. Explor. 42 (1), 24–27. doi:10.3969/j.issn.1001-1986.2014.01.005
Wang, Y. B., Tian, W. G., and Li, W. Z. (2006). Criteria for the evaluation of coalbed methane area selection in China. Geol. Bull. China 25 (9-10), 1104–1107.
Zhao, D. F., Zhao, J. Z., and Chen, Y. (2013). An analysis on the characteristics of the Shan 2 formation gas pools in Yulin-Zizhou area of Ordos basin. Nat. Gas. Geosci. 24 (2), 320–328.
Zou, C. N., Yang, Z., He, D. B., Wei, Y. S., Li, J., Jia, A., et al. (2018). Theory, technology and prospects of conventional and unconventional natural gas. Petroleum Exploitation Dev. 45 (4), 604–618. doi:10.1016/s1876-3804(18)30066-1
Keywords: Ordos Basin, Upper Paleozoic, Shan 2, coal measure gas, exploration potential
Citation: Zhao W-B, Zhang H, Shi Y-H, Fu X-X, Hui J and Jing X-Y (2023) Geological characteristics and exploration potential of the coal measure gas from Shan 2 of the Shanxi formation in the eastern Ordos Basin. Front. Earth Sci. 11:1142141. doi: 10.3389/feart.2023.1142141
Received: 11 January 2023; Accepted: 22 February 2023;
Published: 10 March 2023.
Edited by:
Qingqiang Meng, SINOPEC Petroleum Exploration and Production Research Institute, ChinaReviewed by:
Changyu Fan, Northwest University, ChinaYinghai Guo, China University of Mining and Technology, China
Copyright © 2023 Zhao, Zhang, Shi, Fu, Hui and Jing. This is an open-access article distributed under the terms of the Creative Commons Attribution License (CC BY). The use, distribution or reproduction in other forums is permitted, provided the original author(s) and the copyright owner(s) are credited and that the original publication in this journal is cited, in accordance with accepted academic practice. No use, distribution or reproduction is permitted which does not comply with these terms.
*Correspondence: Hui Zhang, emhhbmdodWkwMV9jcUBwZXRyb2NoaW5hLmNvbS5jbg==; Jie Hui, MTIyOTM0ODYxOEBxcS5jb20=