- Petro China Research Institute of Petroleum Exploration and Development, Beijing, China
Diamondoids are alkanes with cage-like structure. Their diamond-like structure makes them have high stability and anti-biodegradability, and can be preserved and enriched in complex and long geological processes. Therefore, the continuous development of quantitative detection methods for diamondoids in crude oil has deepened the research of these compounds and made them more widely used in crude oil cracking evaluation, maturity evaluation, biodegradation evaluation and other aspects.
1 Introduction
Since the 21st century, exploration work in China has changed significantly. Specifically, exploration targets have gradually shifted from structural hydrocarbon reservoirs to subtle strata and from shallow to deep strata. In addition, exploration objects have been transformed from oil to natural gas. Such changes have triggered a series of new problems in the field of oil and gas exploration, and posed great challenges to the research on oil and gas geochemistry, but also rendered a good opportunity for the development of oil and gas geochemistry.
Increasing attention has been paid to deep oil and gas reservoirs as a new exploration target. Oil and gas geochemical experts have extensively investigated natural gas, light hydrocarbons, and biomarkers, accumulated rich experience, and achieved fruitful theoretical and practical results. However, deep oil and gas reservoirs are often prone to the loss of biomarkers, or equilibrium has been reached. In the meanwhile, light hydrocarbon components are highly susceptible to secondary alteration and mixed sources, which adds to the uncertainty in their practical application. Therefore, it is urgent to develop more stably structured geochemical parameters adaptive to the high degree of evolution.
Among geological organic matter, diamondoids are small molecular alkanes with a cage-like diamond structure (Wingert, 1992; Dahl et al., 1999). Based on the number of three-dimensional (3D) cyclic structures, diamondoids are divided into adamantane, diamantane, triamantane, tetramantane, pentamantane (Figure 1), etc. Diamondoids, which generally refer to adamantane and its alkyl substitutes with strong biodegradation resistance and high thermal stability, widely exist in coal and hydrocarbon source rocks (Imuta and Ouchi, 1973; Aczel et al., 1979; Schulz et al., 2001; Wei et al., 2006b), crude oil (Bender et al., 1986; Williams et al., 1986; Wingert, 1992; Chen et al., 1996; Grice et al., 2000; Dahl et al., 2003; Azevedo et al., 2008; Ma et al., 2009), petroleum products (Wang et al., 2006), and condensate gas (Lin and Wilk, 1995; Stout and Douglas, 2004; Sassen and Post, 2008). Diamondoids have attracted much attention since they can be preserved and enriched in long and complicated geological processes, with relevant geological information carried and rendered. As revealed by previous studies, diamondoids are applicable to evaluating the thermal maturity of crude oil (Zhao et al., 1995; Chen et al., 1996; Zheng et al., 1998; Li et al., 2000; Zhang et al., 2005; Ma et al., 2017; Jiang et al., 2019, 2021, 2022; Akinlua et al., 2020; Goodwin et al., 2020; Huang et al., 2022b), judging the migration direction and charging period of oil and gas (Duan et al., 2007; Huang et al., 2022a, 2022b), assessing the cracking degree of crude oil (Dahl et al., 1999; Chai et al., 2022; Peng et al., 2022), identifying lithofacies (Schulz et al., 2001; Chai et al., 2020), evaluating the secondary alteration of oil reservoirs (Jiang et al., 2020), researching oil-source correlation (Forkner et al., 2021), assessing residual of migration fractionation (Zhu et al., 2021), exploring the thermochemical sulfate reduction reaction (Wei et al., 2011), and identifying in the sources of oil (Stout and Douglas, 2004; Wang et al., 2006; Spaak et al., 2020), especially at the over-mature stage in case of relative equilibrium reached among other biomarkers, or their failure resulting from low content.
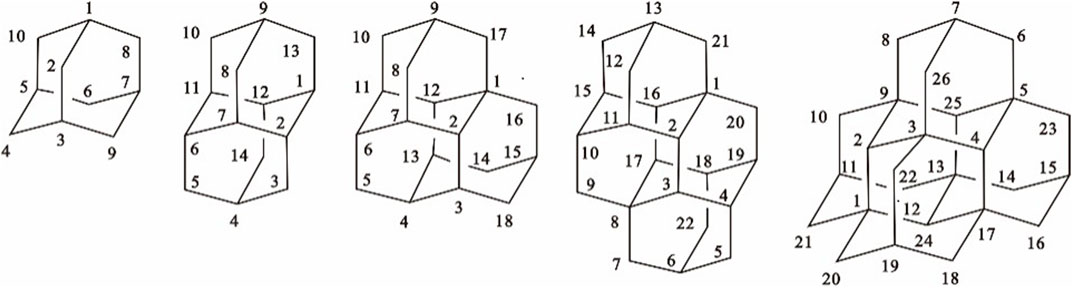
FIGURE 1. Main types of diamondods and their structure (from left to right is adamantane, diamantane, triamantane, tetramantane, pentamantane).
Among geological organic matter, easily detectable high-content adamantane and diamantane compounds have been the focus in the present research and discussion regarding oil and gas geochemistry. Therefore, this paper focuses on adamantanes and diamantanes. The following diamondoids are also used to refer to the total of adamantanes and diamantanes.
2 Quantitative detection method of diamondoids
In 1933, the most simply structured adamantane was detected in petroleum for the first time (Landa and Machacek, 1933). Since then, increasingly abundant diamondoids have been detected in natural gas (Fu and Yu, 1998; Fu and Li, 2001), crude oil and condensate gas (Wingert, 1992; Chen et al., 1996; Grice et al., 2000; Dahl et al., 2003; Stout and Douglas, 2004), petroleum products (Wang et al., 2006), and coal and hydrocarbon source rocks (Schulz et al., 2001; Wei et al., 2006b). Moreover, some diamondoids with higher molecular weights (containing three or more diamond lattice structures) have been successfully separated and identified from petroleum (Dahl et al., 2003; Atwah et al., 2021; Gadzhiev et al., 2021).
Though being detected in nearly all crude oils and most petroleum products (Wang et al., 2006), only low-concentration (several ppm or sub-ppm) diamondoids are generally contained in normal oils (Fort and Schleyer, 1964; Wingert, 1992; Dahl et al., 1999; Wei Z. B. et al., 2007), and they exist in the highly complex matrix of petroleum hydrocarbons. Therefore, high-selectivity and high-sensitivity detection methods are required for quantitatively detecting and analyzing trace components in normal crude oils and describing their minor variation characteristics.
At present, three methods have been mainly adopted to quantitatively detect and analyze diamondoids in crude oil and hydrocarbon source rock samples: the separation of group components from crude oil samples or hydrocarbon source rock extracts in combination with gas chromatography-mass spectrometry (GC-MS) (Figure 2A), the solvent dilution of crude oil samples or hydrocarbon source rock extracts in combination with GC-triple quadrupole tandem MS (GC-MS-MS) (Figure 2B), and the separation of group components from crude oil samples or hydrocarbon source rock extracts in combination with comprehensive 2D GC-time of flight-MS (GC×GC-TOFMS) (Figure 2C).
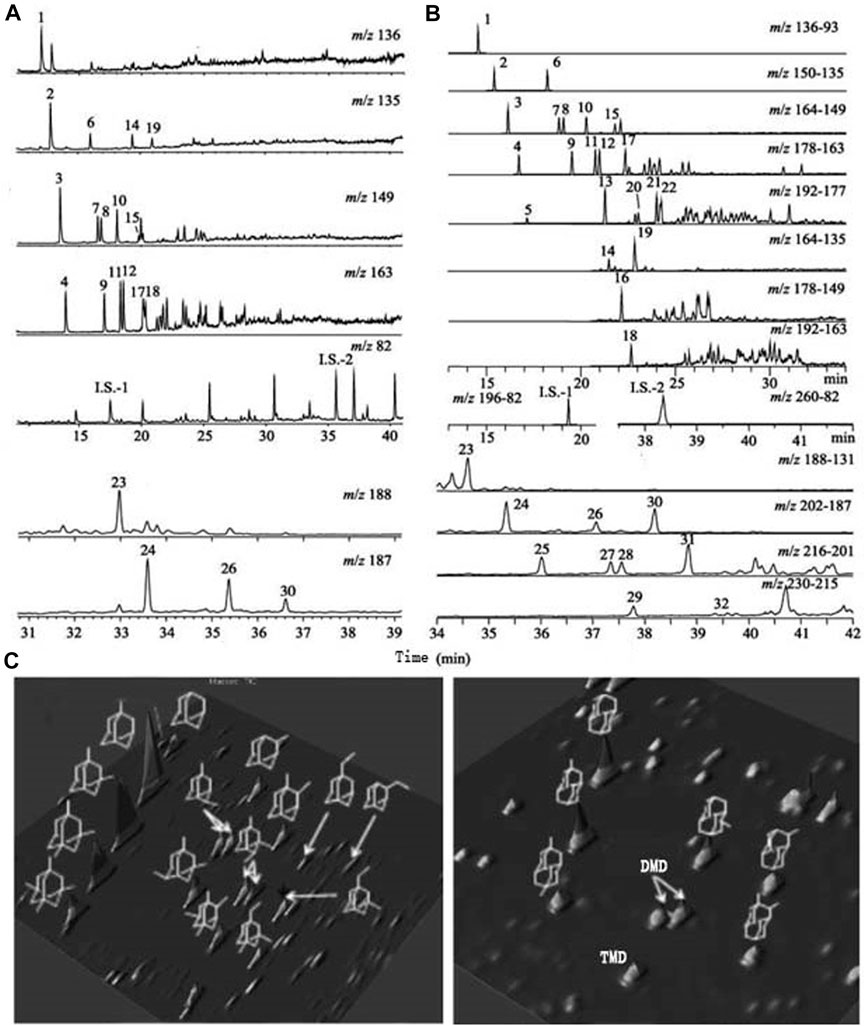
FIGURE 2. Spectrogram of diamondoids in (A) LN14 oil by GC-MS (Liang et al., 2012a), (B) LN14 oil by GC-MS-MS (Liang et al., 2012a), (C) KL205 oil by GC×GC-TOFMS (Wang et al., 2019). (1: adamantane, 2: 1-methyladamantane, 3: 1,3-dimethyladamantane, 4: 1,3,5-trimethyladamantane, 5: 1,3,5,7-tetramethyladamantane, 6: 2-methyladamantane, 7: 1,4-dimethyladamantane(cis), 8: 1,4-dimethyladamantane(trans), 9: 1,3,6-trimethyladamantane, 10: 1,2-dimethyladamantane, 11: 1,3,4-trimethyladamantane(cis), 12: 1,3,4-trimethyladamantane(trans), 13: 1,2,5,7-tetramethyladamantane, 14: 1-ethyladamantane, 15: 2,6- + 2,4-dimethyladamantane, 16: 1-ethyl-3-methyladamantane, 17: 1,2,3-trimethyladamantane, 18: 1-ethyl-3,5-dimethyladamantane, 19: 2-ethyladamantane, 20: 1,3,5,6-tetramethyladamantane, 21: 1,2,3,5-tetramethyladamantane, 22: 1-ethyl-3,5,7-trimethyladamantane, 23: diamantane, 24: 4-methyldiamantane, 25: 4,9-dimethyldiamantane, 26: 1-methyldiamantane, 27: 1,4- + 2,4-dimethyldiamantane, 28: 4,8-dimethyldiamantane, 29: 1,4,9-Trimethyldiamantane, 30: 3-methyldiamantane, 31: 3,4-dimethyldiamantane, 32: 3,4,9-trimethyldiamantane, I.S.-1: n-dodecane-d26, I.S.-2: n-hexadecane-d34).
2.1 A quantitative method integrating group component separation with GC-MS
The first method, a combination of chromatographic column-aided extract separation from actual crude oil samples and hydrocarbon source rock samples with GC-MS, is a qualitative and quantitative analysis method for diamondoids, which has been developed earliest and widely applied (Wingert, 1992; Chen et al., 1996; Grice et al., 2000; Li et al., 2000; Schulz et al., 2001; Zhang et al., 2005).
Crude oil or hydrocarbon source rock samples are usually accompanied by the interference of complex matrix components. Despite high selectivity of GC-MS, the signal of target analytical compounds will still be obscured by the interference of complex matrix components in the analysis results of samples, especially in case of the low concentration of target analytical compounds. Before the actual crude oil samples and hydrocarbon source rock extracts are analyzed via GC-MS, therefore, the interference of the complex matrix is usually eliminated by separating their group components through chromatographic column separation technology, while the target analytical compounds are concentrated and enriched using solvent volatilization (Wingert, 1992; Chen et al., 1996; Grice et al., 2000; Li et al., 2000; Schulz et al., 2001; Zhang et al., 2005). During instrumental analysis, the selective ion monitoring (SIM) mode in GC-MS can enhance the detection signal of diamondoids and improve the sensitivity of target compound analysis. Therein, ions with m/z of 136, 135, 149, 163, 177, and 191 are commonly applied to detect diamantane compounds, those with m/z of 188, 187, 201, 215, and 229 to diamantane compounds, and those with m/z of 240 and 239 to triamantane compounds.
During the separation of group components, condensate gas and gasoline can be directly injected under natural conditions, aiming to avoid the possible volatilization loss of diamondoids. The spectrogram results reveal that in the nonpolar chromatographic column, adamantane peaks earlier than n-C11 (Stout and Douglas, 2004). However, the injection system and chromatographic column of GC-MS will be polluted by complex matrix components and non-paraffinic components with high molecular weights in crude oil samples and hydrocarbon source rock extracts, leading to the reduction of column efficiency. Therefore, this detection and analysis means for diamondoids, i.e., direct injection, does not apply to crude oil and hydrocarbon source rock samples.
2.2 A quantitative method combining solvent dilution with GC-MS-MS
During chromatographic column separation and sample concentration, moreover, evaporation may result in the loss of diamondoids, or cause the deviation of quantitative results, thus affecting the analytical research based on the concentration of diamondoids. Hence, developing the quantitative detection and analysis method integrating the nondestructive pretreatment method with the high-selectivity and high-sensitivity detection method is essential for describing and exploring low-concentration diamondoids in crude oil and hydrocarbon source rock samples.
GC-MS-MS, a highly sensitive and selective instrumental analysis method, eliminates the interference of matrix ions mainly through the selected-reaction monitoring (SRM) mode, without needing any complicated sample pretreatment or purification and separation technology before injection (Frenich et al., 2005; Hernandez et al., 2005). Under the SRM mode of GC-MS-MS, the parent ion of the set target compound is selected when passing through the first quadrupole and then collides with argon molecules in the second quadrupole. Afterward, the resulting fragment ions pass through the third quadrupole, followed by the selection, detection, and analysis of daughter ions corresponding to the target compound in the fragment ions. Thanks to this selection and detection process of GC-MS-MS, the limit of quantification (LOQ) and limit of detection (LOD) of the instrument are reduced, leading to the analysis and detection results of target compounds as low as several ppb (Hernandez et al., 2005; Qu et al., 2010).
For diamondoid detection and analysis, methyl diamantane (MD) compounds in crude oil were quantitatively detected and analyzed at the earliest by monitoring the m/z202+-187+ conversion process (Dahl et al., 1999).
Afterward, Liang et al. (2012b) optimized GC-MS-MS parameters like parent and daughter ions, collision energy, and scanning time using standard diamondoid samples, and then established a complete GC-MS-MS quantitative analysis method for diamondoids. Under the optimal operating parameters of the instrument, good linear relationships were manifested in the standard curves of 10 standard diamondoid samples, and the correlation coefficient (R2) characterizing linear correlations was always higher than 0.9980. In this process, all target diamondoids presented good repeatability, with a relative standard deviation of 1.3%–5.1% (n = 5). The LODs and LOQs of all target diamondoids in oil samples obtained by this method were 0.02–0.11 and 0.08–0.37 μg/g, respectively. The above data manifested the good applicability of this method to quantitative detection and analysis of diamondoids, especially for samples with a low concentration of diamondoids.
Under the SRM mode and optimal operating parameters, GC-MS-MS displays the maximum sensitivity and selectivity for the quantitative analysis of diamondoids in crude oil samples. Besides, this technology can eliminate the interference of co-eluted compounds in crude oil samples and hydrocarbon source rock extracts by monitoring the transformation process of paired parent ions and daughter ions, with high selectivity. In the meanwhile, its high sensitivity can compensate for the low concentration of diamondoids in samples due to the absence of concentration and enrichment pretreatment. Therefore, GC-MS-MS can serve as an effective means for the reliable quantitative analysis of diamondoids in actual samples, only needing simple sample dilution.
2.3 A quantitative method combining group component separation with comprehensive 2D GC×GC-TOFMS
As a new technology developing on the basis of the traditional 2D technology in the 1990s, comprehensive 2D GC×GC characterized by good sensitivity, high resolution, large peak capacity (the product of the peak capacities of two chromatographic columns), fast analysis speed, qualitative analysis with rules to follow, family separation, and tile effect (Frysinger and Gaines, 1999; Lu et al., 2005; Ruan et al., 2002) can be used to separate complex mixtures. Specifically, this technology connects two relatively independent chromatographic columns differing in the separation mechanism and stationary phase (e.g., nonpolar column and chiral column or polar column) in series to form a 2D GC column system by using a modulator with the functions of trapping, focusing, and transmission.
TOFMS, a common MS technique, realizes separation and determination based on different time taken by moving ions with different mass-to-charge ratios and the same kinetic energy in a steady electric field to reach the receiver. This technique is characterized by a wide detectable mass range, fast response speed, and high sensitivity (Zhao and Shen, 2006). If combined with GC×GC, TOFMS is applicable to qualitative and quantitative analysis of compounds in complex systems (Lu et al., 2004b).
GC×GC and GC×GC-TOFMS have been applied to such fields as tobacco, food, wine making, petroleum geology, refined oil products, and environmental monitoring (Schoenmakers et al., 2000; Frysinger and Gaines, 2001; Hua et al., 2002; Frysinger et al., 2003; Lu et al., 2004a; Lu et al., 2007; Ventura et al., 2008). On this basis, Wang et al. (2010) established an analytical detection method for diamondoids in actual crude oil samples by optimizing parameters like column system selection, temperature program, modulation period, hot-air blowing time, and the flow rate of the carrier gas.
However, the pretreatment method can not only remove the interference of other compounds on diamondoids, but also improve the concentration of diamondoids. Therefore, scholars are also constantly improving the pretreatment process of this method to reduce the loss of adamantanes in the pretreatment process (Wang et al., 2019). In addition, scholars have been developing quantitative analysis of diamondoids with higher carbon number (such as triamantane, tetramantane) and more complex structure (such as ethanodiamondoids) (Wang et al., 2019; Ma et al., 2022).
3 Main sources of diamondoids
Diamondoids have been extensively valued, investigated, and applied in oil and gas geochemistry because of the nature and characteristics vested in their own stable structures. Nevertheless, the source, origin, and evolutionary characteristics of diamondoids in geological bodies remain unclear yet. Diamondoids are considered to be abiotic since they have not been detected in modern organisms and sediments (Wei et al., 2006c; Wei et al., 2007 Z. B.). It is guessed that diamondoids are produced in the early diagenetic stage of organic matter because they have been detected in immature and low-mature peats and sedimentary rocks (Schulz et al., 2001; Wei et al., 2006c, Wei et al., 2007 Z. B.). Dahl et al. (1999) thought that once formed, diamondoids will be neither destroyed nor produced again. With the deepening research, however, scholars have proved by laboratory simulations that diamondoids can be generated and cracked in the process of high-temperature thermal evolution, with highly extensive sources of parent materials (Giruts et al., 2006; Giruts and Gordadze, 2007; Fang et al., 2012), and their cracking products include their own isomers and liquid hydrocarbons such as aromatics (Wei et al., 2006b). In the meanwhile, it has been verified by the above research that the formation mechanism of diamondoids is not only restricted to the early proposed catalytic rearrangement of polycyclic naphthenes (Dahl et al., 2010) but also includes high-temperature cracking.
As revealed by predecessors’ results, diamondoids with lower molecular weights are formed mainly through two mechanisms: The first is Lewis acid-catalyzed rearrangement of polycyclic hydrocarbons (Schneide et al., 1966; Wingert, 1992; Lin and Wilk, 1995). The second is high-temperature cracking of higher-molecular-weight components (Giruts et al., 2006; Giruts and Gordadze, 2007; Gordadze and Giruts, 2008). As verified by laboratory thermal cracking simulation experiments, diamondoids with lower molecular weights can be formed in immature sedimentary rocks and peats (Wei et al., 2006c; Wei et al., 2007 Z.), kerogen (Gordadze, 2002), crude oil (Fang et al., 2012), as well as their different family components (Fang et al., 2013), including saturated hydrocarbon components (Giruts et al., 2006) and polar components (nonhydrocarbons and asphaltenes) (Giruts and Gordadze, 2007), and other compounds, such as C16, C19, C22, C34, and C36 (Gordadze and Giruts, 2008) and β-aryl ketone (Berwick et al.). Moreover, all possible isomers of adamantane and diamantane compounds can be detected in the cracking products of the above components. Furthermore, it has been experimentally found that adamantane compounds with lower molecular weights, such as adamantane and diamantane compounds, will crack at high temperatures (Wei et al., 2006b; Fang et al., 2012).
Some thermal cracking experiments manifest that the formation of diamondoids in modern sedimentary rocks is catalyzed or inhibited by minerals under water-bearing conditions, and those in ketone can be catalyzed or inhibited by such minerals as montmorillonite, aluminosilicate, kaolinite, and illite (Wei et al., 2006a, c, 2007b). Some pyrolysis experiments, moreover, show that diamondoids can be produced in the thermal cracking of crude oil samples and their high-molecular-weight components, saturated hydrocarbon components, aromatic hydrocarbon components, and polar components even in the absence of catalysts (Giruts et al., 2006; Giruts and Gordadze, 2007; Fang et al., 2012; Fang et al., 2013).
Through the gold tube thermal simulation experiments of saturated hydrocarbon, aromatic hydrocarbon, asphaltene and nonhydrocarbon in crude oil, it is proved that diamondoids mainly derive from the thermal cracking of saturated hydrocarbon components (Figure 3), as proved by gold tube thermal simulation experiments on saturated hydrocarbons, aromatic hydrocarbons, asphaltenes, and nonhydrocarbons (Fang et al., 2013). Light hydrocarbon components make certain contributions to the formation of diamondoids, as demonstrated by gold tube thermal simulation experiments on the original crude oil and the crude oil after the volatilization of light hydrocarbon components (Fang et al., 2016). In addition, existing studies have shown that diamondoids can be produced during the thermal cracking of kerogen with different types of sedimentary organic matter, but their content and composition characteristics are different (Jiang et al., 2018). In the meanwhile, it has been found through simulation experiments that the content of diamondoids in geological bodies is influenced by volatilization and dissipation (Fang et al., 2016) and biodegradation of crude oil in the early formation stage.
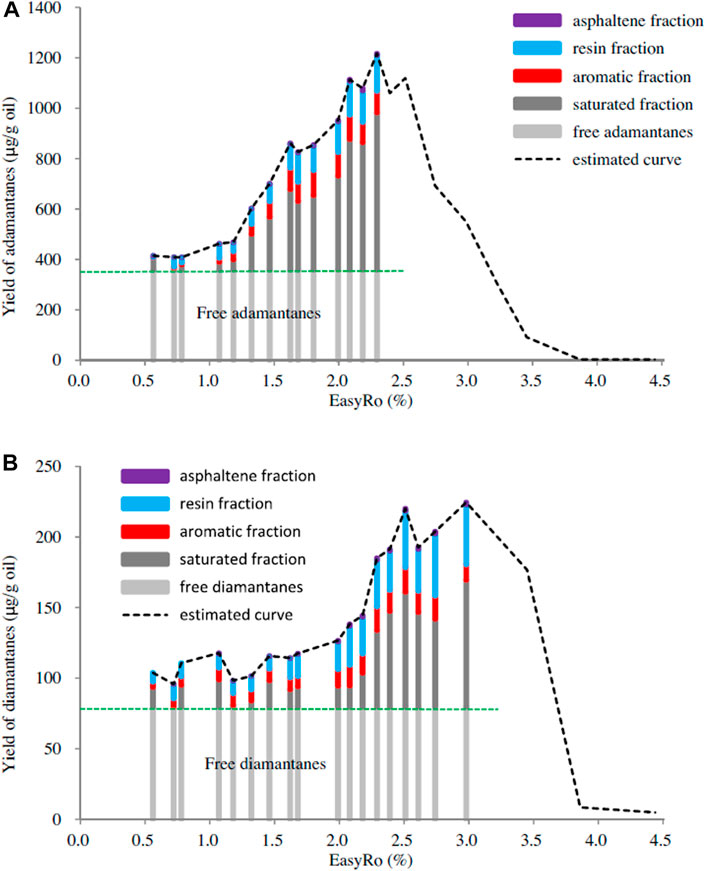
FIGURE 3. The calculated yield curves and source contributions of adamantanes and diamantanes generated from the Tarim oil. (A) Adamantanes; (B) diamantanes (Fang et al., 2013).
In addition, it has been proved through the gold tube thermal simulation experiment on soluble organic matter components in marine shale and kerogen after extraction that diamondoids in organic matter are mainly attributed to the thermal cracking of soluble organic matter components (Figure 4), and the same to coal measures (Fang et al., 2015; Zhai et al., 2022). Furthermore, a thermal cracking experiment was continued on the same kerogen sample after the soluble organic matter was extracted and removed under easy reflectance of vitrinite (EasyRo) of 0.8%, 1.0%, and 1.3%. On this basis, the staged cumulative generation results of diamondoids in kerogen and its resulting asphalt in three stages (EasyRo0.8%–1.0%, 1.0%–1.3%, and 1.3%) were discussed. The results showed that diamondoids were mainly produced in the secondary cracking of asphalt, and the upper limit of the contribution of kerogen thermal cracking to diamondoids was EasyRo1.3% (Li et al., 2015).
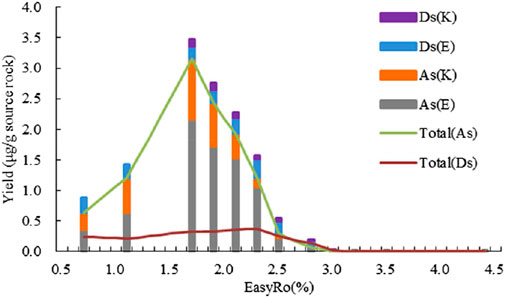
FIGURE 4. Estimated yields (mg/g) of different types diamondoids during source rock maturation. As(K) and As(E) stand for the adamantane contributions from the kerogen pyrolysate and extract pyrolysate, respectively. Ds(K) and Ds(E) stand for the diamantine contributions from the kerogen pyrolysate and extract pyrolysate, respectively (Fang et al., 2015).
4 Application of diamondoids in the geochemical field
Diamondoids in crude oil are applied in the geochemical field mainly through their concentration and parameters.
4.1 Evaluation of crude oil cracking degree
The concentration of diamondoids in crude oil and source rock extracts has been investigated in oil and gas geochemistry. For instance, the relative abundance of diamondoids in light oil and condensate gas can serve as an important “fingerprint” tool to identify the sources of oil spill events (Stout and Douglas, 2004).
According to the theory of Dahl et al. (1999), moreover, once formed, diamondoids will be neither destroyed nor produced again. It is believed, therefore, that the increase in the concentration of diamondoids in oil results from the thermal degradation of most other non-diamondoids in oil (Dahl et al., 1999). Given this, Dahl et al. (1999) proposed that the cracking degree of crude oil can be evaluated by an effective index, the concentration of 3-+4-MD (Figure 5) (Dahl et al., 1999), which has been applied in some studies (Wei Z. B. et al., 2007; Springer et al., 2010). Here, we need to pay attention to the selection of baseline concentration for different regions (Springer et al., 2010).
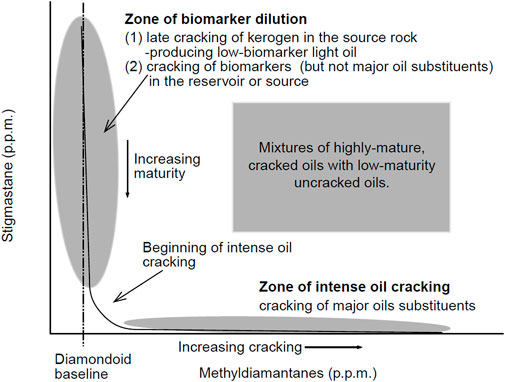
FIGURE 5. Diagram from the concentrations of methyldiamantanes(3-+4-methyldiamantane) and stigmastane (Dahl et al., 1999).
However, Wei et al. (2006b) explored the thermal maturity-dependent changes in the concentration of diamondoids in a set of coal and sedimentary rock samples with a maturity range of Ro=0.20%–6.40%. The quantitative results of diamondoids in coal and source rock extracts manifested that the concentration of diamondoids began to decrease after Ro>4.0% (Wei et al., 2006b). It was also found that some diamondoids can also be thermally cracked to aromatic hydrocarbons under high temperatures (Oya et al., 1981; Schoell and Carlson, 1999; Wei et al., 2006b). As the research deepens, scholars have found that the diamondoids continuously enriched in the cracking process of crude oil may be ascribed to two aspects: the cracking of relatively unstable hydrocarbons in crude oil (Dahl et al., 1999), and the sustained production of diamondoids (Wei et al., 2006c; Fang et al., 2012). Dahl et al. (1999) evaluated the cracking degree of crude oil based on the cracking of relatively unstable hydrocarbons in crude oil, in which the total amount of diamondoids is assumed to be constant. Therefore, this parameter is still applicable at the stage of constant content (EasyRo<2.0%) (Fang et al., 2013), while it needs to be carefully judged at the stage of changing content.
4.2 Maturity evaluation
Among diamondoids, the homologous compounds differing in thermal stability are formed due to the different substitutions on each substituent. For homologous diamondoids formed by the same alkyl substitution, for example, the compounds substituted at “bridge” carbon positions (positions 1, 3, 5, and 7 of adamantane in Figure 1) display higher thermal stability than those substituted at the corresponding “quaternary” carbon positions (positions 2, 4, 6, and 8 of adamantane in Figure 1). In other words, for adamantane compounds with the same methyl substitution, 1- methyl adamantane (MA) formed by methyl substitution at the “bridge” carbon position shows higher thermal stability than 2-MA (Wingert, 1992) (Figure 1). For diamantane compounds with the same methyl substitution, 4-MD formed by methyl substitution at the “bridge” carbon position presents better thermal stability than the other two homologous isomers (1-MD and 3-MD) (Wingert, 1992; Chen et al., 1996).
Based on the aforesaid thermal stability relationships, Chen et al. (1996) also established the ratio parameters of diamondoids, namely, MA index [MAI=1-MA/(1-MA + 2-MA)] and MD index [MDI=4-MD/(1-MD + 3-MD + 4-MD)]. In the meanwhile, they pointed out that these ratios increased with the increase in the thermal maturity of crude oil and hydrocarbon source rocks. Then, such indexes were used to evaluate the maturity of condensate oil samples with a high evolutionary degree in Tarim Basin, and the corresponding Ro was 1.6%–1.7%. The Ro of condensate oil in Ying-Qiong Basin was obtained in a similar fashion as 1.6%–2.0%.
Following this rule, many adamantane maturity parameters have been established in the existing studies and applied in practice. For instance, MAI and MDI have been adopted to evaluate crude oil and hydrocarbon source rocks (Chen et al., 1996; Schulz et al., 2001; Wei et al., 2006b, Wei et al., 2007 Z.). In addition, dimethyl adamantane index-1 (DMAI-1), DMAI-2, ethyl adamantane index (EAI), trimethyl adamantane index-1 (TMAI-1), TMAI-2, dimethyl diamantane index-1 (DMDI-1), and DMDI-2 have been applied in simulation experiments and actual geological samples (Schulz et al., 2001; Zhang et al., 2005; Wei Z. et al., 2007). According to the simulation results of crude oil cracking, the relative changes in TMAI-1 and DMA/MD (DMA stands for DMA compounds, including the sum of adamantane compounds formed by dimethyl substitution at different positions. MD denotes MD compounds, including the sum of diamantane compounds formed by methyl substitution at different positions), and DMAI-1 and DMA/MD are also applicable parameters for crude oil maturity (Figure 6) (Fang et al., 2013).
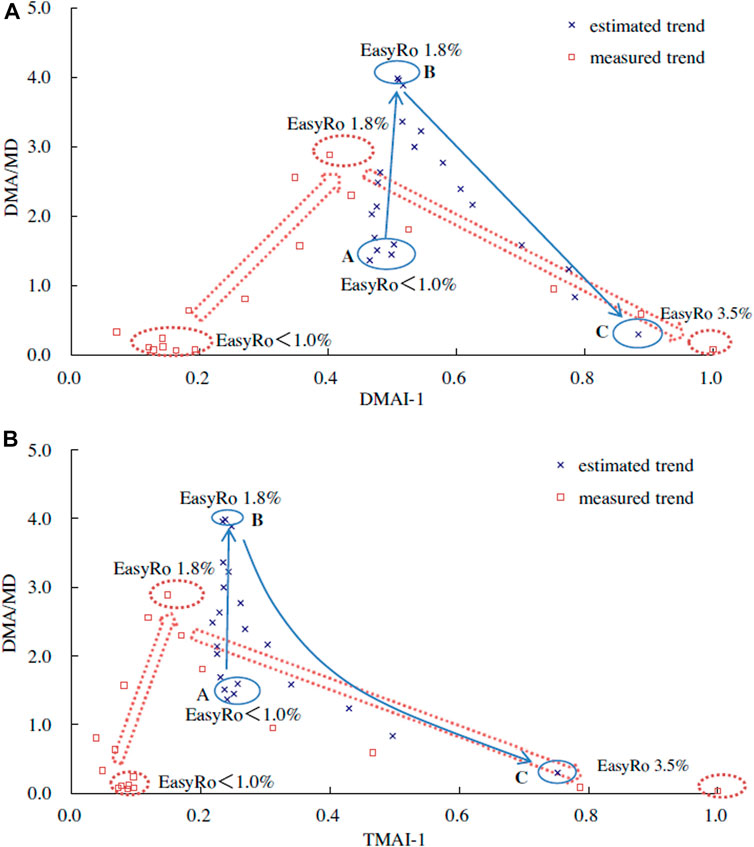
FIGURE 6. The plots of concentration ratios vs. isomerization ratios of diamondoids for the Tarim oil cracking. (A) DMA/MD vs. DMAI-1; (B) DMA/MD vs. TMAI-1 (Fang et al., 2013).
Taking advantages of diamondoids in maturity evaluation, Duan et al. (2007) investigated the distribution characteristics of diamantane parameters of crude oil in Tahe Oilfield, Tarim Basin, analyzed and discussed the charging period and migration direction of oil and gas in this oilfield, and explored the migration direction of crude oil. Bao et al. (2015) analyzed the distribution characteristics of alkyl adamantane and alkyl diamantane compounds in condensate oil in Zhujiadun, Yancheng Depression, Subei Basin, and that in upper Cretaceous mature hydrocarbon source rocks in Taizhou Formation, as well as their parameters. On this basis, the source of condensate oil was explored, and the comparison with the measured Ro showed that adamantane compounds are practical, to some extent, in the maturity evaluation of crude oil and hydrocarbon source rocks.
Problems exist despite the extensive practical application of adamantane parameters in maturity evaluation. As suggested by the existing studies, MAI and MDI are only applicable in a narrow range of Ro. Li et al. (2000) analyzed the adamantane parameters obtained from the extracts of the hydrocarbon source rocks in lower Ordovician Majiagou Formation in the central gas field of Ordos Basin, China, and thought that MDI changes within 44%–65%, without obvious changes in the area of Ro>2.0%, so it is only applicable in a Ro range of 0.9%–2.0%. However, Schulz et al. (2001) and Wei Z. B. et al. (2007) conducted thermal cracking experiments on actual samples under water-bearing conditions, and held that MAI and MDI are only applicable to the maturity evaluation of samples with Ro>1.3%.
On the other hand, Li et al. (2000) also pointed out no obvious correlation between MDI and sample depth or Ro, which contradicts with the previous conclusion drawn by Chen et al. (1996). As discovered through laboratory simulation of MDI-Ro correlation thermal maturation of hydrocarbon source rocks in different horizons of Ying-Qiong Basin, samples from different horizons are correlated with maturity differently, which also highlights the reliability problem and limitations of adamantane parameters in practical application (Li et al., 2000; Wei Z. et al., 2007).
4.3 Identification of genetic types
At present, the research on the genetic types of organic matter in diamondoids is still insufficient. Schulz et al. (2001) proposed for the first time that dimethyl diamantane compounds (4,9-dimethyl diamantane, 4,8-dimethyl diamantine, and 3,4-dimethyl diamantane) show different distribution characteristics in different types of hydrocarbon source rocks (Figure 7). To be specific, marine siliceous clastic rocks of type II kerogen are rich in 4,9-dimethyl diamantane and carbonate rocks are rich in 4,8-dimethyl diamantine, while type III carbon mudstone and coal contain abundant 3,4-dimethyl diamantane. Accordingly, it was pointed out that different types of organic matter can be distinguished using a triangle diagram characterizing the relative content of dimethyl diamantane compounds.
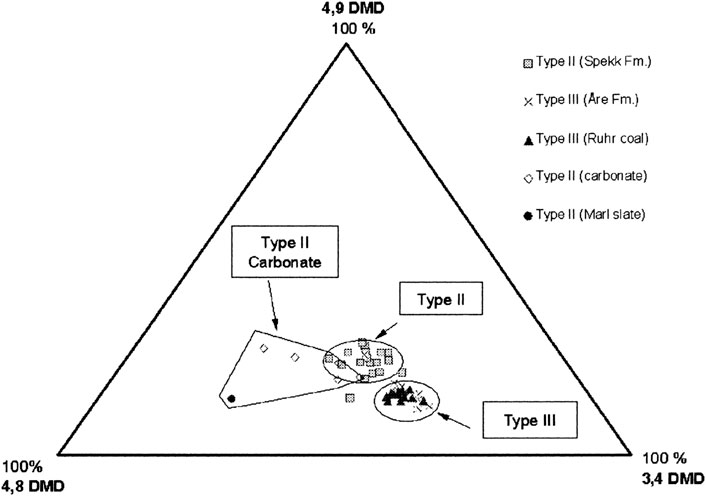
FIGURE 7. Triangular plot of the relative distribution of 4,9-dimethyldiamantane (4,9-DMD), 4,8-dimethyldiamantane (4,8-DMD) and 3,4-dimethyldiamantane (3,4-DMD) (Schulz et al., 2001).
With this triangle diagram, which was considered an effective identification method, Chen et al. (2008) identified the genetic types of organic matter of condensate oil in Jiyang Depression, and concluded that the condensate oil in Jiyang Depression originates from the mixture of coal bed and lake facies.
The existing studies have shown that the difference in some maturity parameters may result from different sources or causes of organic matter during hydrocarbon generation (Li et al., 2015; Jiang et al., 2018). For instance, DMAI-1 and DMAI-2 may be different due to the primary cracking of kerogen at different stages and the secondary cracking of its resulting asphalt (Li et al., 2015). Among the gold tube thermal cracking products of type I and type II kerogen, two bivariant parameters (DMA/MD and TMAI-1, and DMA/MD and DMAI-1) of diamondoids also display different evolutionary characteristics. Even the loss of volatile components of crude oil in the low-mature stage will generate an influence on the maturity parameters of adamantane in the later stage (Fang et al., 2016).
Some scholars have suggested that the application of diamondoids can be extended by deeply exploring their evolutionary characteristics during the thermal evolution of different types of hydrocarbon source rocks (Ma, 2016). As aforementioned, the influence of organic matter types on the composition characteristics of diamondoids has been analytically investigated through gold tube cracking simulation experiments on different types of kerogens (Jiang et al., 2018). However, the difference in composition characteristics is attributed to the combined effects of the primary cracking of kerogen and the secondary cracking of asphalt, which goes against the situation under actual geological conditions. Efforts have been made to distinguish the two effects, but what has been obtained is the staged cumulative generation result of diamondoids in kerogen and its resulting asphalt in three stages: EasyRo0.8%–1.0%, 1.0%–1.3%, and >1.3% (Li et al., 2015).
4.4 Evaluation of biodegradation degree
Biodegradation, a significant transformation process of crude oil in underground oil and gas-bearing basins (Connan, 1984; Peters and Moldwan, 1993; Head et al., 2003), will influence the physical and chemical molecular characteristics of crude oil (Winters and Williams, 1969; Hunt, 1979; Connan, 1984; Peters and Moldowan, 1993) and further affect oil exploitation and refining costs. Moreover, biodegradation can generate important economic impacts on oil (Connan, 1984; Wilhelms et al., 2001; Larter et al., 2006) and strategic impacts on oil exploration (Larter et al., 2012).
A few studies regarding the biodegradation process and degree of crude oil by diamondoids have been reported. Generally, hydrocarbons present consistent overall variation trends and different individual rates in the process of biodegradation (Larter et al., 2006). Accordingly, the evaluation indexes of biodegradation degree have been established in past studies based on the differences in relative abundance and sequentially sensitive structures among different series of compounds (Volkman et al., 1984; Peters and Moldowan, 1993). Following the same principle, Grice et al. (2000) quantitatively detected diamondoids in actual crude oil samples with different degrees of biodegradation, and the results manifested that MA/A (MA stands for MA compounds, including the sum of MA at each substitution position, and A denotes adamantane) will change regularly with the variation of the biodegradation degree, which can serve as an evaluation index of the biodegradation degree and can also be used to identify the mixture of severely biodegradable oil and non-biodegradable oil. Besides, MA/A will not be significantly influenced by the difference in maturity. Wei Z. B. et al. (2007) already applied this parameter in related studies.
Wang et al. (2006), Yang et al. (2013), Grice et al. (2000), Wei Z. B. et al. (2007), and Chai et al. (2022) quantitatively detected diamondoids in crude oil biodegradation products during indoor and outdoor simulations and those in actual crude oil biodegradation products. Results revealed that diamondoids will be affected by biodegradation in actual oil reservoirs, with their content presenting an overall declining trend (Grice et al., 2000; Wang et al., 2006; Wei Z. B. et al., 2007; Yang et al., 2013; Chai et al., 2022). Therein, the decreasing trend of the content of adamantane resembles that of total diamondoids, which is decided by the dominant position of adamantane compounds in diamondoids. The content of diamantane compounds, however, shows a less obvious decreasing trend than that of adamantane compounds, reflecting the better anti-biodegradation ability of diamantane compounds than adamantane compounds. In the meanwhile, triamantane compounds are subjected to minor content changes (Wang et al., 2006; Wei Z. B. et al., 2007). Even if the biodegradation degree reaches the eighth grade defined by Peters and Moldowan (1993), crude oil will still contain diamondoids with a certain concentration (Wei Z. B. et al., 2007), which also effectively guarantees the application of diamondoids in biodegradable oils, especially in highly biodegradable oils.
The application of other parameters in biodegradable oils has also been studied by scholars. Dahl et al. (1999) proposed an effective index, namely, the concentration of 3-+4-MD, to evaluate the cracking degree of crude oil and mixed oil. This method is considered immune to biodegradation (Wei Z. B. et al., 2007). Grice et al. (2000) calculated the MAI and MDI in actual crude oil samples biodegraded to different degrees, and found that the MAI is consistent well, reflecting their similar maturity. However, the MDI varies a lot, which may be associated with the mineral content in source rocks (Grice et al., 2000). In addition, Xu (2007) tested the stability of MAI and MDI in biodegradation, both of which indicated a good anti-biodegradation ability. And the research results of Chai et al. (2022) indicate that no significant biodegradation impact on MAI, EAI, MDI, DMDI-1 and DMDI-2.
5 Summary
This paper summarizes the research of adamantanes in crude oil. (1) There are three main methods for quantitative analysis of diamondoids in crude oil, pretreatment combined with GC-MS, crude oil dilution combined with GC-MS-MS, and pretreatment combined with GC×GC-TOFMS. (2) The main sources of diamondoids in crude oil are the thermal cracking of saturated hydrocarbon components or the soluble organic matter components in source rocks. (3) Diamondoids can be applied to evaluate the crude oil cracking degree, maturity degree, biodegradation degree, and identificate the genetic types. However, attention should also be paid to the scope of application and assumptions.
Author contributions
CF: writing—original draft preparation, validation. GH: conceptualization, writing—reviewing and editing, validation. YY: investigation, arrangement. DG: investigation, supervision. All authors contributed to the article and approved the submitted version.
Funding
This work was financially supported by the National Natural Science Foundation of China (Grant Nos 42172184 and 42272188), the Scientific Research and Technological Development Project of China National Petroleum Corporation (Grant No. 2021DJ0601), Basic research and strategic reserve technology research fund project of institutes directly under CNPC (Grant No. 2020D-5008-02).
Conflict of interest
The authors were employed by Petro China Research Institute of Petroleum Exploration and Development.
Publisher’s note
All claims expressed in this article are solely those of the authors and do not necessarily represent those of their affiliated organizations, or those of the publisher, the editors and the reviewers. Any product that may be evaluated in this article, or claim that may be made by its manufacturer, is not guaranteed or endorsed by the publisher.
References
Aczel, T., Gorbaty, M. L., Maa, P. S., and Schlosberg, R. H. (1979). Stability of adamantane and its derivatives to coal-liquefaction conditions, and its implications toward the organic structure of coal. Fuel 58, 228–230. doi:10.1016/0016-2361(79)90123-6
Akinlua, Akinsehinwa, Ibeachusim, B. I., Adekola, S. A., Adedosu, T. A., Li, Y., and Xiong, Y. Q. (2020). Diamondoid geochemistry of Niger delta source rocks: Implication for petroleum exploration. Energy Sources, Part A Recovery, Util. Environ. Eff., 1–11. doi:10.1080/15567036.2020.1840672
Atwah, I., Moldowan, J. M., Koskella, D., and Dahl, J. (2021). Application of higher diamondoids in hydrocarbon mudrock systems. Fuel 284, 118994. doi:10.1016/j.fuel.2020.118994
Azevedo, D. A., Tamanqueira, J. B., Dias, J. C. M., Carmo, A. P. B., Landau, L., and Goncalves, F. T. T. (2008). Multivariate statistical analysis of diamondoid and biomarker data from Brazilian basin oil samples. Fuel 87, 2122–2130. doi:10.1016/j.fuel.2007.11.005
Bao, J. P., Liang, X. Y., Zhu, C. S., and Jiang, X. C. (2015). Diamondoid hydrocarbons and their geochemical significances in condensate from the Zhujiadun gas reservoir in the Yancheng sag. Nat. Gas. Geosci. 26 (3), 513–523.
Bender, A. O., Said, E. Z., and Abdulsada, A. K. (1986). Gas chromatographic identification of adamantanes in some Iraqi crude oils. Analyst 111, 575–576. doi:10.1039/an9861100575
Chai, Z., Chen, Z. H., Liu, H., Cao, Z. C., Cheng, B., Wu, Z. P., et al. (2020). Light hydrocarbons and diamondoids of light oils in deep reservoirs of shuntuoguole low uplift, Tarim Basin: Implication for the evaluation on thermal maturity, secondary alteration and source characteristics. Mar. Petroleum Geol. 117, 104388. doi:10.1016/j.marpetgeo.2020.104388
Chai, Z., Chen, Z., Patience, R., Wen, Z., Tang, Y., Cheng, B., et al. (2022). Light hydrocarbons and diamondoids in deep oil from tabei of Tarim Basin: Implications on petroleum alteration and mixing. Mar. Petroleum Geol. 138, 105565. doi:10.1016/j.marpetgeo.2022.105565
Chen, J., Fu, J., Sheng, G., Liu, D., and Zhang, J. (1996). Diamondoid hydrocarbon ratios: Novel maturity indices for highly mature crude oils. Org. Geochem. 25 (3), 179–190. doi:10.1016/s0146-6380(96)00125-8
Chen, Z. L., Liu, X., Jin, H. R., Wang, Z., and Zhang, L. Y. (2008). Study on condensate maturity and type using methyl diamantane parameter. Acta Sedimentol. Sin. 26 (4), 705–708. CNKI:SUN:CJXB.0.2008-04-025
Connan, J. (1984). “Biodegradation of crude oils in reservoirs,” in Advances in petroleum geochemistry. Editors J. Brooks, and D. H. Welte (London, UK: Academic Press), 299–335.
Dahl, J. E., Liu, S. G., and Carlson, R. M. K. (2003). Isolation and structure of higher diamondoids, nanometer-sized diamond molecules. Science 299 (5603), 96–99. doi:10.1126/science.1078239
Dahl, J. E., Moldowan, J. M., Peters, K. E., Claypool, G. E., Rooney, M. A., Michael, G. E., et al. (1999). Diamondoid hydrocarbons as indicators of natural oil cracking. Nature 399 (6731), 54–57. doi:10.1038/19953
Dahl, J. E. P., Moldowan, J. M., Wei, Z. B., Lipton, P. A., Denisevich, P., Gat, R., et al. (2010). Synthesis of higher diamondoids and implications for their formation in petroleum. Angew. Chemie-International Ed. 49, 9881–9885. doi:10.1002/anie.201004276
Duan, Y., Wang, C. Y., Zheng, C. Y., and Wu, B. X. (2007). Diamantane distribution characteristics in crude oil from Tahe Oilfield in Tarim Basin and oil and gas migration. Nat. Gas. Geosci. 18 (5), 693–696. doi:10.1016/S1872-5813(07)60034-6
Fang, C. C., Xiong, Y. Q., Li, Y., Chen, Y., and Tang, Y. J. (2015). Generation and evolution of diamondoids in source rock. Mar. Petroleum Geol. 67, 197–203. doi:10.1016/j.marpetgeo.2015.05.018
Fang, C. C., Xiong, Y. Q., Li, Y., Liang, Q. Y., Wang, T. S., and Li, Y. X. (2016). The effect of volatile components in oil on evolutionary characteristics of diamondoids during oil thermal pyrolysis. Sci. China Earth Sci. 59, 362–370. doi:10.1007/s11430-015-5163-x
Fang, C., Xiong, Y., Li, Y., Chen, Y., Liu, J., Zhang, H., et al. (2013). The origin and evolution of adamantanes and diamantanes in petroleum. Geochimica Cosmochimica Acta 120, 109–120. doi:10.1016/j.gca.2013.06.027
Fang, C., Xiong, Y., Liang, Q., and Li, Y. (2012). Variation in abundance and distribution of diamondoids during oil cracking. Org. Geochem. 47, 1–8. doi:10.1016/j.orggeochem.2012.03.003
Forkner, R., Fildani, A., Ochoa, J., and Moldowan, J. M. (2021). Linking source rock to expelled hydrocarbons using diamondoids: An integrated approach from the northern gulf of Mexico. J. Petroleum Sci. Eng. 196, 108015. doi:10.1016/j.petrol.2020.108015
Fort, R. C., and Schleyer, P. V. (1964). Adamantane: Consequences of the diamondoid structure. Chem. Rev. 64, 277–300. doi:10.1021/cr60229a004
Frenich, A. G., Gonzalez-Rodriguez, M. J., Arrebola, F. J., and Vidal, J. L. M. (2005). Potentiality of gas chromatography-triple quadrupole mass spectrometry in vanguard and rearguard methods of pesticide residues in vegetables. Anal. Chem. 77, 4640–4648. doi:10.1021/ac050252o
Frysinger, G. S., and Gaines, R. B. (1999). Comprehensive two-dimensional gas chromatography with mass spectrometric detection (GC × GC/MS) applied to the analysis of petroleum. J. High Resolut. Chromatogr. 22 (5), 251–255. doi:10.1002/(sici)1521-4168(19990501)22:5<251:aid-jhrc251>3.0.co;2-v
Frysinger, G. S., and Gaines, R. B. (2001). Separation and identification of petroleum biomarkers by comprehensive two-dimensional gas chromatography. J. Sep. Sci. 24 (2), 87–96. doi:10.1002/1615-9314(20010201)24:2<87:aid-jssc87>3.0.co;2-0
Frysinger, G. S., Gaines, R. B., Xu, L., and Reddy, C. M. (2003). Resolving the unresolved complex mixture in petroleum-contaminated sediments. Environ. Sci. Technol. 37 (8), 1653–1662. doi:10.1021/es020742n
Fu, N., and Li, Y. C. (2001). Diamondoid hydrocarbon ratios as indicators of maturity in natural gas. Acta Sedimentol. Sin. 19 (1), 145–149. doi:10.3969/j.issn.1000-0550.2001.01.025
Fu, N., and Yu, X. G. (1998). Detection and application of diamondoids in natural gas. Petroleum Geol. Exp. 20 (3), 267–271.
Gadzhiev, G. A., Badmaev, C. M., Gordadze, G. N., and Giruts, M. V. (2021). Thermal diffusion separation of petroleum diamondoids and protodiamondoids. Pet. Chem. 61, 147–151. doi:10.1134/s0965544121020110
Giruts, M. V., and Gordadze, G. N. (2007). Generation of adamantanes and diamantanes by thermal cracking of polar components of crude oils of different genotypes. Pet. Chem. 47 (1), 12–22. doi:10.1134/s0965544107010021
Giruts, M. V., Rusinova, G. V., and Gordadze, G. N. (2006). Generation of adamantanes and diamantanes by thermal cracking of high-molecular-mass saturated fractions of crude oils of different genotypes. Pet. Chem. 46 (4), 225–236. doi:10.1134/s0965544106040025
Goodwin, N. R. J., Abdullayev, N., Javadova, A., Volk, H., and Riley, G. (2020). Diamondoids and basin modelling reveal one of the world's deepest petroleum systems, south caspian basin, Azerbaijan. J. Petroleum Geol. 43 (2), 133–149. doi:10.1111/jpg.12754
Gordadze, G. N., and Giruts, M. V. (2008). Synthesis of adamantane and diamantane hydrocarbons by high-temperature cracking of higher n-alkanes. Pet. Chem. 48, 414–419. doi:10.1134/s0965544108060029
Gordadze, G. N., 2002. Termoliz organicheskogo veshchestva v neftegazopoiskovoĭ geokhimii. Institut geologii i razrabotki goriru Chikh iskopaemykh IGiRGI, Moskva.
Grice, K., Alexander, R., and Kagi, R. I. (2000). Diamondoid hydrocarbon ratios as indicators of biodegradation in Australian crude oils. Org. Geochem. 31 (1), 67–73. doi:10.1016/s0146-6380(99)00137-0
Head, I. M., Jones, D. M., and Larter, S. R. (2003). Biological activity in the deep subsurface and the origin of heavy oil. Nature 426 (6964), 344–352. doi:10.1038/nature02134
Hernandez, F., Portoles, T., Pitarch, E., Lopez, F. J., Beltran, J., and Vazquez, C. (2005). Potential of gas chromatography coupled to triple quadrupole mass spectrometry for quantification and confirmation of organohalogen xenoestrogen compounds in human breast tissues. Anal. Chem. 77, 7662–7672. doi:10.1021/ac050874+
Hua, R. X., Ruan, C. H., Wang, J. H., Lu, X., Liu, J., Xiao, K., et al. (2002). Research of group separation of petroleum fractions by comprehensive two-dimensional gas chromatography. Acta Chim. Sin. 12, 2185–2191. doi:10.3321/j.issn:0567-7351.2002.12.018
Huang, W., Yu, S., Zhang, H., Xiao, Z., Liu, D., and Pan, C. (2022a). Diamondoid fractionation and implications for the kekeya condensate field in the southwestern depression of the tarim basin, nw China. Mar. Petroleum Geol. 138, 105551. doi:10.1016/j.marpetgeo.2022.105551
Huang, W., Zhang, H., Xiao, Z., Yu, S., and Pan, C. (2022b). Generation, expulsion and accumulation of diamondoids, aromatic components and gaseous hydrocarbons for gas fields in Kuqa Depression of the Tarim Basin, NW China. Mar. Petroleum Geol. 145, 105893. doi:10.1016/j.marpetgeo.2022.105893
Imuta, K., and Ouchi, K. (1973). Isolation of adamantane from coal extract. Fuel 52, 301–302. doi:10.1016/0016-2361(73)90062-8
Jiang, W., Chen, C., Long, Z., Li, Y., Yang, C., and Xiong, Y. (2022). Geochemical characteristics and possible sources of crude oils in the baiyun deep-water area of the pearl river mouth basin, south China sea. Mar. Petroleum Geol. 135, 105410. doi:10.1016/j.marpetgeo.2021.105410
Jiang, W., Li, Y., Fang, C., Yu, Z., and Xiong, Y. (2021). Diamondoids in petroleum: Their potential as source and maturity indicators. Org. Geochem. 160, 104298. doi:10.1016/j.orggeochem.2021.104298
Jiang, W., Li, Y., and Xiong, Y. (2020). Reservoir alteration of crude oils in the junggar basin, northwest China: Insights from diamondoid indices. Mar. Petroleum Geol. 109, 104451. doi:10.1016/j.marpetgeo.2020.104451
Jiang, W., Li, Y., and Xiong, Y. (2019). Source and thermal maturity of crude oils in the junggar basin in northwest China determined from the concentration and distribution of diamondoids. Org. Geochem. 128, 148–160. doi:10.1016/j.orggeochem.2019.01.004
Jiang, W., Li, Y., and Xiong, Y. (2018). The effect of organic matter type on formation and evolution of diamondoids. Mar. Petroleum Geol. 89, 714–720. doi:10.1016/j.marpetgeo.2017.11.003
Landa, S., and Machacek, V. (1933). Sur l'adamantane, nouvel hydrocarbure extrait du naphte. Collect. Czechoslov. Chem. Commun. 5, 1–5. doi:10.1135/cccc19330001
Larter, S., Huang, H., Adams, J., Bennett, B., Jokanola, O., Oldenburg, T., et al. (2006). The controls on the composition of biodegraded oils in the deep subsurface: Part II—geological controls on subsurface biodegradation fluxes and constraints on reservoir-fluid property prediction. AAPG Bull. 90 (6), 921–938. doi:10.1306/01270605130
Larter, S., Huang, H., Adams, J., Bennett, B., and Snowdon, L. R. (2012). A practical biodegradation scale for use in reservoir geochemical studies of biodegraded oils. Org. Geochem. 45, 66–76. doi:10.1016/j.orggeochem.2012.01.007
Li, J. G., Paul, P., and Cui, M. Z. (2000). Methyl diamantane index (MDI) as a maturity parameter for Lower Palaeozoic carbonate rocks at high maturity and overmaturity. Org. Geochem. 31 (4), 267–272. doi:10.1016/s0146-6380(00)00016-4
Li, Y., Chen, Y., Xiong, Y. Q., Wang, X. T., Fang, C. C., Zhang, L., et al. (2015). Origin of adamantanes and diamantanes in marine source rock. Energy & Fules 29, 8188–8194. doi:10.1021/acs.energyfuels.5b01993
Liang, Q., Xiong, Y., Fang, C., and Li, Y. (2012a). Comparison of two methods for the determination of diamondoids in crude oil. Geochemica 41, 433–441. doi:10.1007/s11783-011-0280-z
Liang, Q., Xiong, Y., Fang, C., and Li, Y. (2012b). Quantitative analysis of diamondoids in crude oils using gas chromatography–triple quadrupole mass spectrometry. Org. Geochem. 43, 83–91. doi:10.1016/j.orggeochem.2011.10.008
Lin, R., and Wilk, Z. A. (1995). Natural occurrence of tetramantane (C22H28), pentamantane (C26H32) and hexamantane (C30H36) in a deep petroleum reservoir. Fuel 74, 1512–1521. doi:10.1016/0016-2361(95)00116-m
Lu, H. L., Zhao, M. Y., Liu, H. M., and Bo, Y. C. (2005). Principle and application of comprehensive two dimensional gas chromatography-mass spectroscopy: A review. Tob. Sci. Technology/Tobacco Chem. 3, 22–25. CNKI:SUN:YCKJ.0.2005-03-007
Lu, H. L., Zhao, M. Y., Liu, H. M., Gong, A. D., Yu, J., Zheng, H. N., et al. (2007). Determination of neutral chemical constituents in flue-cured tobacco by comprehensive two-dimensional gas chromatography and time-of flight mass spectrometry. Chin. J. Chromatogr. 25 (1), 30–34. doi:10.3321/j.issn:1000-8713.2007.01.006
Lu, X., Cai, J. L., Wu, J. F., Kong, H. W., Zhao, M. Y., Hua, R. X., et al. (2004a). Characterization of phenols in cigarette mainstream smoke condensate by using comprehensive two-dimensional gas chromatography/time-of-flight mass spectrometry. Acta Chim. Sin. 62 (8), 804–810. doi:10.3321/j.issn:0567-7351.2004.08.011
Lu, X., Wu, J. F., Wu, J. H., Kong, H. W., Hua, R. X., Tao, W. S., et al. (2004b). Characterization and quantitative determination of diesel fractions by comprehensive two-dimensional gas chromatography/time of flight mass spectrometry. Chin. J. Chromatogr. 22 (1), 5–11. doi:10.3321/j.issn:1000-8713.2004.01.002
Ma, A. L., Jin, Z. J., and Zhu, C. S. (2017). Maturity and oil-cracking of the ordovician oils from Tahe oilfield, Tarim Basin, NW China. Nat. Gas. Geosci. 28 (2), 313–323. doi:10.1016/j.jnggs.2017.12.001
Ma, A. L., Jin, Z. J., Zhu, C. S., Peng, S. T., and Zhang, W. B. (2009). Quantitative analysis on absolute concentration of diamondoids in oils from Tahe Oilfield. Acta Pet. Sin. 30 (2), 214–218. doi:10.7623/syxb200902009
Ma, A. L., Lin, H. X., Yun, L., Qiu, N. S., Zhu, X. X., and Wu, X. (2022). Detection of ethanodiamantanes in the Ordovician crude oil from Shuntuoguole area in Tarim Basin and its significance. Acta Pet. Sin. 43 (6), 788–803. doi:10.7623/syxb202206004
Ma, A. L. (2016). New advancement in application of diamondoids on organic geochemistry. Nat. Gas. Geosci. 27 (005), 851–860. doi:10.11764/j.issn.1672-1926.2016.05.0851
Oya, A., Nakamura, H., Otani, S., and Marsh, H. (1981). Carbonization of adamantane to a graphitizable carbon. Fuel 60, 667–669. doi:10.1016/0016-2361(81)90215-5
Peng, Y., Cai, C., Fang, C., Wu, L., Liu, D., Sun, P., et al. (2022). Diamondoids and thiadiamondoids generated from hydrothermal pyrolysis of crude oil and TSR experiments. Sci. Rep. 12, 196. doi:10.1038/s41598-021-04270-z
Peters, K. E., and Moldowan, J. M. (1993). The biomarker guide: Interpreting molecular fossils in petroleum and ancient sediments. Englewood Cliffs, NJ, USA: Prentice Hall.
Qu, L. J., Zhang, H., Zhu, J. H., Yang, G. S., and Aboul-Enein, H. Y. (2010). Rapid determination of organophosphorous pesticides in leeks by gas chromatography-triple quadrupole mass spectrometry. Food Chem. 122, 327–332. doi:10.1016/j.foodchem.2010.02.038
Ruan, C. H., Ye, F., Kong, H. W., Lu, X., and Xu, G. W. (2002). Separation characteristics of comprehensive two-dimensional gas chromatography in analyzing petroleum sample. Chin. J. Anal. Chem. 30 (5), 548–551. doi:10.1016/S0956-5663(01)00303-7
Sassen, R., and Post, P. (2008). Enrichment of diamondoids and 13C in condensate from hudson canyon, US atlantic. Org. Geochem. 39, 147–151. doi:10.1016/j.orggeochem.2007.10.004
Schneide, A., Warren, R. W., and Janoski, E. J. (1966). Formation of perhydrophenalenes and polyalkyladamantanes by isomerization of tricyclic perhydroaromatics. J. Org. Chem. 31, 1617–1625. doi:10.1021/jo01343a070
Schoell, M., and Carlson, R. M. K. (1999). Diamondoids and oil are not forever. Nature 399, 15–16. doi:10.1038/19847
Schoenmakers, P. J., Oomen, J. L. M. M., Blomberg, J., Genuit, W., and Velzen, G. V. (2000). Comparison of comprehensive two-dimensional gas chromatography and gas chromatography-mass spectrometry for the characterization of complex hydrocarbon mixtures. J. Chromatogr. A 892, 29–46. doi:10.1016/s0021-9673(00)00744-5
Schulz, L. K., Wilhelms, A., Rein, E., and Steen, A. S. (2001). Application of diamondoids to distinguish source rock facies. Org. Geochem. 32 (3), 365–375. doi:10.1016/s0146-6380(01)00003-1
Spaak, G., Edwards, D. S., Grosjean, E., Scarlett, A. G., and Grice, K. (2020). Identifying multiple sources of petroleum fluids in browse basin accumulations using diamondoids and semi-volatile aromatic compounds. Mar. Petroleum Geol. 113, 104091. doi:10.1016/j.marpetgeo.2019.104091
Springer, M. V., Garcia, D. F., Goncalves, F. T. T., Landau, L., and Azevedo, D. A. (2010). Diamondoid and biomarker characterization of oils from the llanos orientales basin, Colombia. Org. Geochem. 41, 1013–1018. doi:10.1016/j.orggeochem.2010.03.002
Stout, S. A., and Douglas, G. S. (2004). Diamondoid hydrocarbons—Application in the chemical fingerprinting of natural gas condensate and gasoline. Environ. Forensics 5 (4), 225–235. doi:10.1080/15275920490886734
Ventura, G. T., Kenig, F., Reddy, C. M., Frysinger, G. S., Gaines, R. B., Mooy, B. V., et al. (2008). Analysis of unresolved complex mixtures of hydrocarbons extracted from Late Archean sediments by comprehensive two-dimensional gas chromatography (GC×GC). Org. Geochem. 39, 846–867. doi:10.1016/j.orggeochem.2008.03.006
Volkman, J. K., Alexander, R., Kagi, R. I., Rowland, S. J., and Sheppard, P. N. (1984). Biodegradation of aromatic hydrocarbons in crude oils from the Barrow Sub-basin of Western Australia. Org. Geochem. 6, 619–632. doi:10.1016/0146-6380(84)90084-6
Wang, H. T., Weng, N., Zhang, S. C., Chen, J. P., and Wei, C. Y. (2010). Characteristics and identification of saturated hydrocarbons by comprehensive two-dimensional gas chromatography coupled to time-of-flight mass spectrometry. J. Chin. Mass Spectrom. Soc. 31, 18–27. doi:10.1016/S1872-2067(10)60128-3
Wang, H. T., Weng, N., Zhang, S. C., Wei, C. Y., and Zhang, C. J. (2019). A novel method for quantitative analysis of diamondoids in petroleum samples. Petroleum Geol. Exp. 41 (3), 443–450. doi:10.11781/sysydz201903443
Wang, Z., Yang, C., Hollebone, B., and Fingas, M. (2006). Forensic fingerprinting of diamondoids for correlation and differentiation of spilled oil and petroleum products. Environ. Sci. Technol. 40 (18), 5636–5646. doi:10.1021/es060675n
Wei, Z. B., Moldowan, J. M., Peters, K. E., Wang, Y., and Xiang, W. (2007a). The abundance and distribution of diamondoids in biodegraded oils from the San Joaquin Valley: Implications for biodegradation of diamondoids in petroleum reservoirs. Org. Geochem. 38, 1910–1926. doi:10.1016/j.orggeochem.2007.07.009
Wei, Z., Mankiewicz, P., Walters, C., Qian, K., Phan, N. T., Madincea, M. E., et al. (2011). Natural occurrence of higher thiadiamondoids and diamondoidthiols in a deep petroleum reservoir in the Mobile Bay gas field. Org. Geochem. 42 (2), 121–133. doi:10.1016/j.orggeochem.2010.12.002
Wei, Z., Michael Moldowan, J., Dahl, J., Goldstein, T. P., and Jarvie, D. M. (2006a). The catalytic effects of minerals on the formation of diamondoids from kerogen macromolecules. Org. Geochem. 37 (11), 1421–1436. doi:10.1016/j.orggeochem.2006.07.006
Wei, Z., Moldowan, J. M., Jarvie, D. M., and Hill, R. (2006b). The fate of diamondoids in coals and sedimentary rocks. Geology 34 (12), 1013–1016. doi:10.1130/g22840a.1
Wei, Z., Moldowan, J. M., and Paytan, A. (2006c). Diamondoids and molecular biomarkers generated from modern sediments in the absence and presence of minerals during hydrous pyrolysis. Org. Geochem. 37 (8), 891–911. doi:10.1016/j.orggeochem.2006.04.008
Wei, Z., Moldowan, J. M., Zhang, S., Hill, R., Jarvie, D. M., Wang, H., et al. (2007b). Diamondoid hydrocarbons as a molecular proxy for thermal maturity and oil cracking: Geochemical models from hydrous pyrolysis. Org. Geochem. 38 (2), 227–249. doi:10.1016/j.orggeochem.2006.09.011
Wilhelms, A., Larter, S. R., Head, I., Farrimond, P., Di-Primio, R., and Zwach, C. (2001). Biodegradation of oil in uplifted basins prevented by deep-burial sterilization. Nature 411 (6841), 1034–1037. doi:10.1038/35082535
Williams, J. A., Bjoroy, M., Dolcater, D. L., and Winters, J. C. (1986). Biodegradation in south Texas eocene oils — effects on aromatics and biomarkers. Org. Geochem. 10, 451–461. doi:10.1016/0146-6380(86)90045-8
Wingert, W. S. (1992). GC-MS analysis of diamondoid hydrocarbons in Smackover petroleums. Fuel 71 (1), 37–43. doi:10.1016/0016-2361(92)90190-y
Winters, J. C., and Williams, J. A. (1969). “September Microbiological alteration of crude oil in the reservoir,” in Symposium on petroleum transformation in geologic environments (Washington, D.C, United States: American Chemical Society), E22–E31.
Xu, X. Q. (2007). Analysis and tracer technology of organic pollutants in the marine environment. Xiamen, college of chemistry and chemical engineering. Xiamen, China: Xiamen University.
Yang, B. J., Zheng, L., Cui, Z. S., Gao, W., Li, Q., and Wang, X. R. (2013). Changes of diamondoid compounds in crude oil from the west of Bohai sea by biodegradation in shorelines. Mar. Environ. Sci. 4, 481–485. CNKI:SUN:HYHJ.0.2013-04-001
Zhai, J., Cao, Z., Fang, C., Yuan, Y., Wu, W., and Liu, J. (2022). The evolution characteristics of diamondoids in coal measures and their potential application in maturity evaluation. Front. Earth Sci. 10. doi:10.3389/feart.2022.1031799
Zhang, S., Huang, H., Xiao, Z., and Liang, D. (2005). Geochemistry of palaeozoic marine petroleum from the Tarim Basin, NW China. Part 2: Maturity assessment. Org. Geochem. 36 (8), 1215–1225. doi:10.1016/j.orggeochem.2005.01.014
Zhao, B., and Shen, X. J. (2006). The development of TOFMS analysis technique. Mod. Sci. Instrum. 4, 30–33. doi:10.3969/j.issn.1003-8892.2006.04.006
Zhao, H., Wang, P. R., Chen, Q., Yao, H. X., and Zhu, J. Z. (1995). Adamantane and its application to studying the maturity of crude oils from Tarim Basin. J. Jianghan Petroleum Inst. 17 (3), 24–30.
Zheng, L. J., Cao, J. P., Xue, J. H., and Bie, D. Z. (1998). A new index for the maturity of crude oil and hydrocarbon source rocks-methyl diamantane index. Petroleum Geol. Exp. 20 (4), 411–416.
Keywords: diamondoids, detection method, main source, geological application, crude oil
Citation: Fang C, Hu G, Yuan Y and Gong D (2023) Review on detection method, main source and geological application of diamondoids in crude oil. Front. Earth Sci. 11:1141209. doi: 10.3389/feart.2023.1141209
Received: 10 January 2023; Accepted: 10 July 2023;
Published: 25 August 2023.
Edited by:
Qingqiang Meng, SINOPEC Petroleum Exploration and Production Research Institute, ChinaReviewed by:
Zeyu Yang, Environment and Climate Change Canada, CanadaTaohua He, Yangtze University, China
Copyright © 2023 Fang, Hu, Yuan and Gong. This is an open-access article distributed under the terms of the Creative Commons Attribution License (CC BY). The use, distribution or reproduction in other forums is permitted, provided the original author(s) and the copyright owner(s) are credited and that the original publication in this journal is cited, in accordance with accepted academic practice. No use, distribution or reproduction is permitted which does not comply with these terms.
*Correspondence: Guoyi Hu, aHVndW95aTY5QHBldHJvY2hpbmEuY29tLmNu