- 1School of Mines, China University of Mining and Technology, Xuzhou, China
- 2Shangyuquan Coal Mine of Shanxi Luneng Hequ Electric Coal Development Co., Ltd., Xuzhou, China
- 3State Key Laboratory of Coal Resources and Safe Mining, China University of Mining and Technology, Xuzhou, Jiangsu, China
- 4Jiangsu Engineering Laboratory of Mine Earthquake Monitoring and Prevention, Xuzhou, Jiangsu, China
During the mining of thick coal seams under the gully slope, the mining-induced fractures are prone to connect with the gully runoff, which may induce potential water hazards and affect underground production. Based on the occurrence characteristics of coal seams and the landform in the gully-developed mining area, the coal seam mining under the slope of Na’er Coal Mine and Baoshan Coal Mine was taken as the engineering background, the seasonal runoff of gullies was considered as the influencing factors of the safe mining, and the spatial relationship between the working face and the gully slope was analyzed when the mining-induced fractures were connected with the gully surface and caused the gully runoff water disaster. The research results show that the occurrence structure of the gully slope has a greater impact on the strata movement of the slope. When the key layer is incomplete, the horizontal sliding and local block overturning of the slope rock strata are strong; when the key layer is complete, the horizontal sliding and local block overturning of the slope rock strata are weakened. According to the safety distance of the working face and the generalized model of safety coal pillar setting, the method of setting the safety coal pillar was used to prevent the connection of slipping cracks of the rock strata at the slope bottom and the gully surface during the slope mining, thus preventing the occurrence of runoff hazards during the thick coal seams mining in the valley terrain. The results can provide a theoretical basis and technical support for the prevention and control of seasonal runoff hazards, as well as a reference for the prevention and control of mining-induced landslides, water conservation mining, and ecological environment protection.
1 Introduction
Research on the overburden movement and fracture development characteristics caused by coal seam mining is the theoretical basis for safe and efficient mining in gully-developed mining areas. To ensure the normal shallow coal seam mining under the gully, researchers have revealed the impact of slope under the gully on the mining pressure of the stope (Liu et al., 2021). At the same time, some researchers have summarized the disaster-causing mechanism of water disasters on the surface of coal mines (Gui et al., 2020). It is pointed out that seasonal rainfall and natural watercourses formed by valleys are the main factors of water inrush disasters in the mining process of shallow coal seams. To solve the problem of roadway water inrush and water conservation mining caused by the development of fracture zones (Zhang and López, 2019; Xie et al., 2021; Chen et al., 2022), Xie obtained five development stages of the water-conducting fracture zones. Besides, previous studies have shown that different mining methods (Du and Gao, 2017; Zhou and Yu, 2022), mining parameters (Wang et al., 2020; Qi et al., 2022) and repeated mining of coal seams (Tian et al., 2019; Cheng et al., 2020) also affect the development height of water-conducting fracture zones. Based on a large number of engineering practices, different numerical analysis models (Zhang et al., 2017; Zeng et al., 2022), mechanical theoretical models (Ning et al., 2020; Zhu et al., 2020; Yang et al., 2021; Tan et al., 2022) and monitoring methods (Wang et al., 2016; Mondal et al., 2020) have been successively introduced into the prediction and exploration of the height of water-conducting fracture zones. In addition, some researchers have effectively observed the development of water-conducting fracture zones from the perspective of strain energy (Swift, 2013; Qi et al., 2019; Sainoki et al., 2019).
In the existing studies, remarkable achievements have been made in the following aspects of the overburden movement and mining-induced fractures development characteristics, safe mining in gully-developed mining areas, and water protection (preservation) of gully water sources. However, there are still some limitations: 1) the influence of the geometry variability of the gully slope and the complexity of coal (rock) bed occurrence conditions on the overburden movement of the gully slope and the development of the mining-induced fracture is ignored; 2) the possible connection between advance fractures caused by mining-induced overburden movement and gully runoff is not fully considered. To this end, the mining of coal seams under the gully slope was taken as the engineering background, and the impact of seasonal runoff of the valley on safe mining was fully considered in this study. Based on the characteristics of overburden movement and fracture development of mining slopes with different occurrence structures, the main modes of strata movement of the slope rock, the basic manifestations and the main distribution areas of mining-induced fractures connecting the gully surface were analyzed, the basic modes, conditions and prediction methods that cause gully runoff water disasters were obtained, and the technical measures for prevention and control of runoff hazards caused by mining under gully slope were proposed.
2 Characteristics of engineering geology and hydrological environment
2.1 Characteristics of the occurrence structure of gully slope
In the mining area with valley terrain, the integrity of the overburden (soil layer) of the coal seam is damaged due to the cutting of the gully. For a typical shallow coal seam, there is only one main key layer in the overburden. With the increase of burial depth of coal seams and the change of overburden occurrence structure, the number of key layers also increases in varying degrees. Since the contact layer between the gully slope and surface runoff on both sides of the gully is mainly located at the slope bottom, there are two types of gully slope with key layers at the slope bottom: ① rock strata with an incomplete key layer at the slope bottom; ② rock strata with a complete key layer at the slope bottom, as shown in Figure 1.
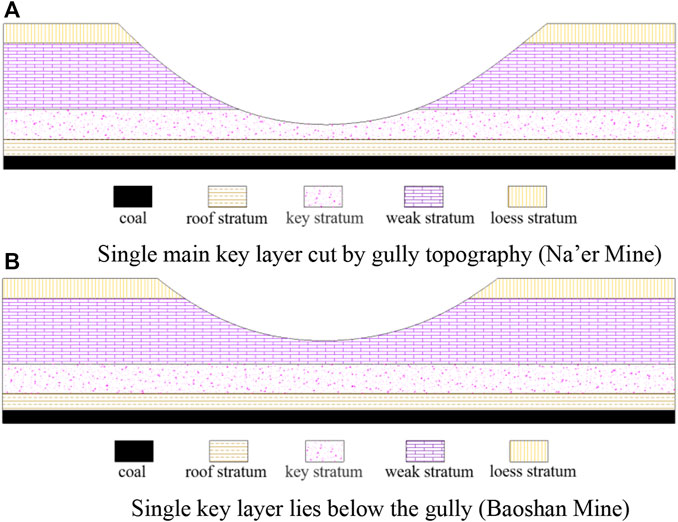
FIGURE 1. Occurrence structure of the gully slope. (A) Single main key layer cut by gully topography (Na’er Mine). (B) Single key layer lies below the gully (Baoshan Mine).
2.2 Hydrological environment characteristics
The coalfield in border areas of Shanxi, Northern Shaanxi Provinces and Inner Mongolia Autonomous Region was taken as the study area. The ecological environment of the study area is sensitive and fragile, and the hydrological environment also shows regional characteristics. In general, the study area has an arid semi-arid climate, with relatively scarce water resources, sparse vegetation, and low precipitation. The annual precipitation of this area is unevenly distributed, mainly from July to September. The precipitation in the rainy season (July–September) accounts for more than 50%–60% of the annual precipitation, as shown in Table 1.
In the gully-developed mining area, the atmospheric precipitation is mainly discharged along the valley in the form of surface runoff at the bottom of the gully. During the seasonal rainfall period, especially the concentrated rainfall, the precipitation is collected at the bottom of the gully under the conditions of valley terrain, forming seasonal rivers and even gully floods. When mining activities are conducted under the gully slope, the gully runoff will enter the stope along the crack pathway if the mining-induced fracture connects with the surface or bottom of the gully slope, as shown in Figure 2.
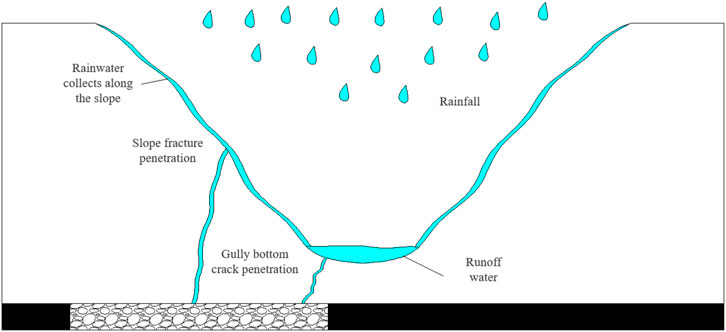
FIGURE 2. Schematic diagram of connection between valley seasonal runoff and mining-induced fractures.
3 Fracture development characteristics of the slope during the mining under the gully
Based on the landform and geological conditions of two gully slopes encountered in the mining process of Na’er Coal Mine and Baoshan Coal Mine, the physical experimental models of similar materials for two types of gully slope structures were established, including geological models of gully slope with an incomplete key layer and that with a complete key layer (as shown in Figures 3, 4). Besides, the characteristics of overburden movement caused by mining activities under the gully slope were studied and analyzed, and the main types and manifestations of the slope under the mining were focused on.
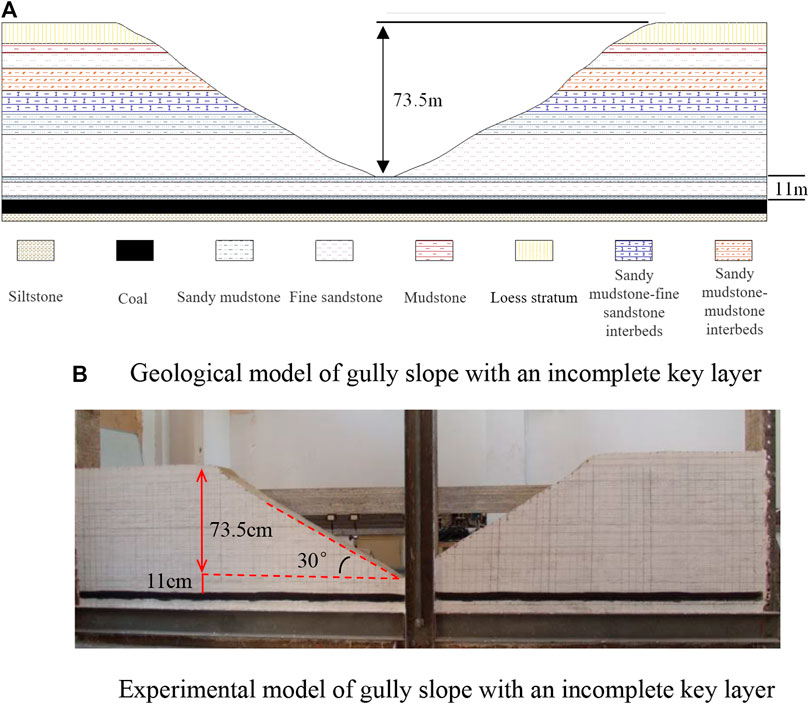
FIGURE 3. Similar physical experiment of gully slope with an incomplete key layer. (A) Geological model of gully slope with an incomplete key layer. (B) Experimental model of gully slope with an incomplete key layer.
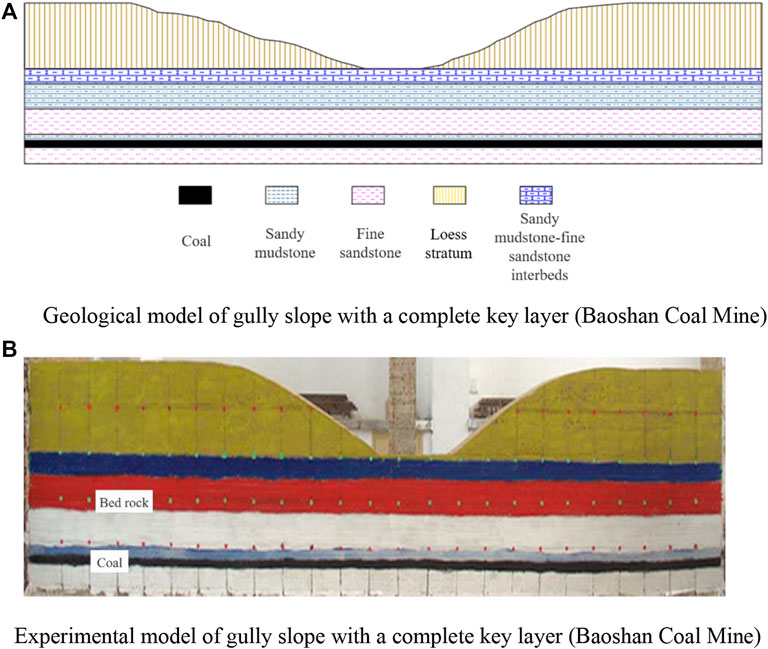
FIGURE 4. Similar physical experiment of gully slope with an incomplete key layer. (A) Geological model of gully slope with a complete key layer (Baoshan Coal Mine). (B) Experimental model of gully slope with a complete key layer (Baoshan Coal Mine).
3.1 Mining under the gully slope with an incomplete key layer
In terms of the overall development height of mining-induced fractures, under the geological condition of Na’er Coal Mine, mining-induced fractures develop to the surface, that is, there are only a caving zone and fracture zone on the vertical section of overburden. The heights of the caving zone and the fracture zone are about 23.4 m and 61.1 m, which are similar to those in the mining of typical shallow buried coal seams.
As shown in Figure 5, most of the adjacent areas collapse in layered form. The development height of mining-induced fractures in the adjacent area of the slope rises slowly, while it develops rapidly in the area below the slope. When the working face is advanced to 30 m, the roof begins to cave, and a small amount of longitudinal cracks is produced. When the working face is advanced to 60 m, there is a massive collapse of rock beneath the main roof, and cracks with different development degrees are generated. As the working face advances forward and enters the mining area below the gully, the collapsed strata continue to develop upward, and the cracks near the slope develop rapidly. When the working face is advanced to 160 m, a large tensile crack appears at the upper part of the slope section because one side of the overburden of the working face is a free surface, and it continues to develop downward with the increase of mining space. When the working face is advanced to 190 m, the surface crack is connected with the longitudinal crack of overburden. During the whole mining process, the surface subsidence gradually deviates to the gully direction, and mining-induced fractures develop to the surface.
After coal seam mining, the development of mining-induced fractures and overburden movement are related and promote each other. The development and distribution characteristics of mining-induced fractures in overburden after mining are treated with digital binarization, as shown in Figure 6. Besides, the shape and distribution of the mining-induced fractures in overburden are highly consistent with the overburden movement, which is mainly reflected in the following two aspects:
① During the mining toward the gully, separated layers or horizontally staggered cracks are the main mining-induced fractures in overburden, and there are some vertical cracks connecting separated layers or horizontally staggered cracks in different layers. The mining-induced fractures in the overburden of the Xianggou mining area are closely related and promote the overburden movement. This phenomenon is particularly obvious at the bottom of the slope. Consequently, the goaf below the slope bottom is directly connected with the gully bottom, with a large degree of connectivity. The maximum width of the cracks is about 1.9 m. If there is perennial or seasonal surface runoff at the bottom of the gully, there will be a large risk of water inrush.
② During the mining backward the gully, tensile cracks are the main mining-induced fractures in overburden, which directly connect the goaf and the slope surface. The experimental results show that there are three large tensile cracks in the overburden of the slope area. The maximum width of the tensile cracks can reach 3.6 m and these cracks hardly close. As a result of the crack cutting, the overburden presents a massive structure, and the goaf is connected with the slope surface. If the surface runoff level at the bottom of the gully is large, it can also lead to a greater risk of water inrush.
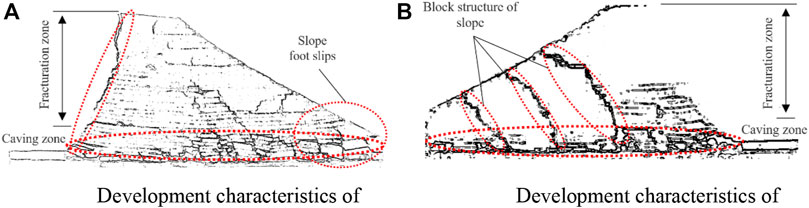
FIGURE 6. Final state of overburden fracture development in the slope model of Na’er Coal Mine during the mining under the gully. (A) Development characteristics of fractures during the mining toward the gully. (B) Development characteristics of fractures during the mining backward the gully.
3.2 Mining under the gully slope with a complete key layer
Figure 7 shows the development characteristics of mining-induced fractures under the conditions of the Baoshan Coal Mine. The overburden in the adjacent area is dominated by layered collapse. When the working face is advanced to 20 m, the strata directly under the immediate roof generate separation, and longitudinal cracks appear at both ends of the strata directly under the immediate roof simultaneously. When the working face is advanced to 30 m, the stratification phenomenon is intensified, and the crack in the immediate roof further increases and gradually extends to the coal wall. When the working face is advanced to 60 m, the rock strata under the main roof collapse in a large area, the separation layer between the direct roof and the basic roof increases, and the longitudinal cracks gradually become connected around. As the working face advances to the gully mining area, the crack development characteristics are similar to those of Na’er Coal Mine, and the cracks are gradually developed. When the working face is advanced to 110 m, the advanced tensile cracks are developed on the surface. When the working face is advanced to 140 m, the tensile crack is connected with the crack in the caving zone. When the working face advances to 160 m, new cracks are generated on the surface. As the working face advances, the longitudinal cracks gradually close and generate new tensile cracks. After the excavation of the working face, the cracks develop to the surface, and the development law of surface cracks is consistent with the activity law of periodic roof breaking.
As Baoshan Coal Mine is a typical shallow coal seam, there is only a single key layer in the bedrock, and the mining thickness of the coal seam is about 6 m. The development height of mining-induced fracture in the Baoshan Coal Mine is the same as that of the mining slope test in Na’er Coal mine. As shown in Figure 8, there are only two zones (the caving zone and fracture zone) on the vertical profile. The experimental results show that the height of the caving zone is about 21.2 m, and the fracture zone is developed to the surface, with a height of about 68.8 m. The experimental results show that Baoshan Coal Mine has the following development and distribution characteristics of mining-induced fractures during the mining under the slope.
① During the mining toward the gully, overburden separation and staggered cracks are developed, and are penetrated by vertical cracks layer by layer. During the mining backward the gully, the vertical cracks are developed, and the separation and staggered cracks are only developed in the bedrock. Besides, the overburden mining-induced fractures during the mining of the Xianggou and Beigou are similar to those of Na’er Coal Mine. At the same time, it also shows that the overburden movement and the crack development characteristics during the coal seam mining toward and backward the gully slope have certain particularity and homogeneity.
② The mining-induced fractures of the slope are not directly connected with the goaf. After the coal seam is mined, the mining-induced fracture in overburden also develops to the surface (or gully surface). Different from the mining in the Na’er coal mine, after the mining under the slope of Baoshan coal mine, the slope cannot be directly involved in the roof movement of coal seam because the main key layer is still a certain distance (45 m) from the bottom of the gully. Combined with the buffering effect of the soft rock between the main key layer and the slope, the mining-induced fractures in the slope area do not directly connect with the goaf, but indirectly connect with the goaf through the mining-induced fractures in the rock layer under the slope.
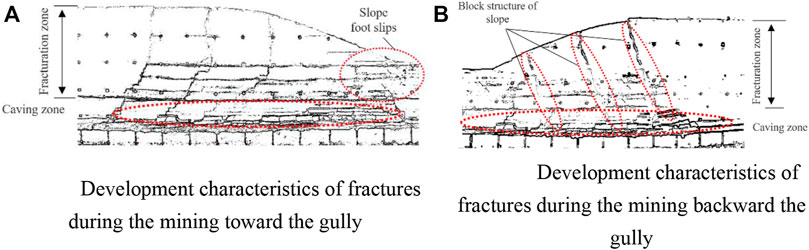
FIGURE 8. Final state of overburden fracture development in the slope model of Baoshan Coal Mine during the mining under the gully. (A) Development characteristics of fractures during the mining toward the gully. (B) Development characteristics of fractures during the mining backward the gully.
3.3 Prediction of mining-induced fracture development height in overburden
The physical simulation experiment shows that when the development height of mining-induced fractures develops to the slope, the slope rock strata directly or indirectly participate in the roof activities of the coal seam, resulting in the unique strata movement of the slope rock and the characteristics of crack development. When applying the key layer theory to analyze the development height of the water-conducting fractures in overburden, it is generally believed that if the key layer is broken, the rock strata controlled by the key layer will be broken successively, and the mining-induced fractures will penetrate the strata under their control. The coal seam produces relatively large space after mining, and the roof strata have a certain dilatancy after caving and can reduce the free space under the key layer. Nevertheless, if the lower key layer is broken and the higher key layer still reaches the breaking condition, the cracks will pass through the higher key layer and continue to develop upward, leading to the increase in the height of mining-induced fractures, as shown in Figure 9.
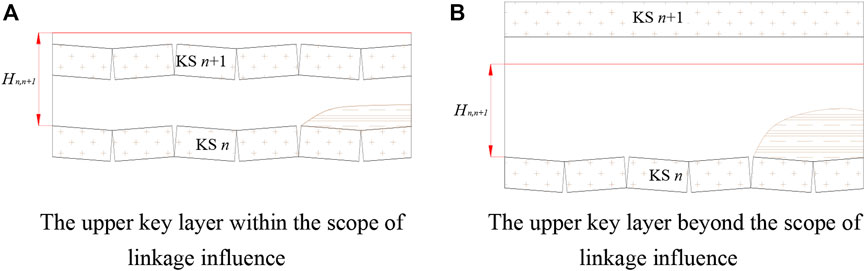
FIGURE 9. Linkage influence height of key layers. (A) The upper key layer within the scope of linkage influence. (B) The upper key layer beyond the scope of linkage influence.
According to whether there is free space below the key layer, the spatial position of the key layer to be broken can be analyzed. It is assumed that H’i-1,i is the actual thickness of the rock stratum between the key layers i-1 and key layer i; ki-1,i is the crushing expansion coefficient of the rock stratum between the key layers i-1 and key layer i; hi is the thickness of the key layer i; H’0,1 and k0,1 are the actual thickness and the crushing expansion coefficient the rock stratum between the coal seam and key layer 1. Therefore, Eq. 1 can be obtained as follows:
Besides, Hn,n+1 is defined as the spatial linkage influence height of the key layer breaking (referred to as the linkage height of the key layer). The linkage height is explained as follows: after key layer n is broken, if its upper adjacent key layer (key layer n+1) is within the linkage height range, it can cause the breakage of key layer n+1, as shown in Figure 9A. Otherwise, as there are enough thick rock layers with strong deformation resistance between the two key layers, the breakage of key layer n cannot cause the breakage of key layer n+1, as shown in Figure 9B. Due to the linkage height of the key layer, when the relative spatial position of the key layer in overburden meets the conditions, the breakage of the lower key layer can induce the breakage of the adjacent key strata, thus resulting in the breakage of the upper key layer. When mining-induced fractures pass through the upper key layer, the mining-induced fractures continue to develop upward in overburden.
4 The influence of slope occurrence and mining conditions on the development of mining-induced fractures in overburden
The change of slope occurrence and mining conditions of coal seams will greatly affect the movement of the gully slope and the crack development. In this section, the discrete element program 3DEC was used to conduct numerical simulation experiments, and the influencing factors (slope angle, slope height, coal seam mining thickness, burial depth of gully bottom) on the movement and destruction of the overburden of the gully slope with two structural types, namely, the absence of key layers and the integrity of the sequence, It lays a foundation for the prevention and control of gully runoff. Based on the two models and the analysis results of slope occurrence characteristics in Section 3, the actual typical slope of Na’er Coal Mine was taken as a reference. In the above two slope models, the slope angle was set as 30°, the slope height as 60 m, and the coal seam mining thickness as 6 m. Table 2 shows test schemes of the basic slope model, and Figure 10 shows the numerical model. Based on the test schemes of the two slope models, the simulation schemes for the influence characteristics of main factors are formulated as shown in Tables 3, 4.
In this section, according to the main influencing factors of mining under the slope, the slope terrain and the occurrence conditions of coal and rock, based on the 3DEC discrete element program, a total of 26 slope numerical models of nine groups of two types have been established, simulation experiments have been carried out, Table 5 and the fitting formulas between the horizontal safety distance from the working face of Class I and Class II mining slope to the slope bottom and the slope angle, slope height, and the minimum buried mining ratio at the gully bottom have been obtained, as shown in Figure 11.
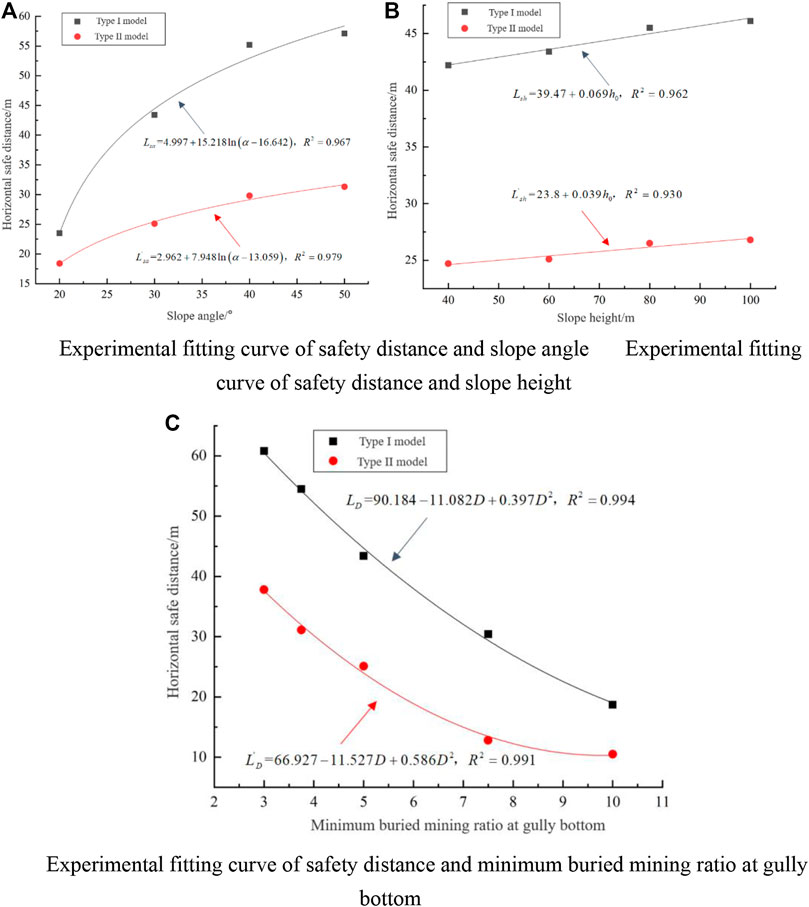
FIGURE 11. Safe distance of working face under different slope parameters. (A) Experimental fitting curve of safety distance and slope angle (B) Experimental fitting curve of safety distance and slope height. (C) Experimental fitting curve of safety distance and minimum buried mining ratio at gully bottom.
The analysis results show that: 1) the changes of slope angle, slope height, coal seam mining height and buried depth of gully bottom coal seam have different degrees of influence on the Class I slope model (with an incomplete key layer) and Class II slope model (with a complete key layer). The experimental results show that the damage degree of slope rock with an incomplete key layer is more significant. 2) Under the same slope structure type, the response degree of slope rock strata movement and failure to the four influencing factors (slope angle, slope height, coal seam mining height, and coal seam burial depth of gully bottom) is different. The larger the slope angle, coal seam mining height, and slope height, the more significant the influence on strata movement and failure of the slope rock, while the influence degree of slope height is relatively weaker than that of slope angle and mining height. The larger the coal seam buried at the bottom of the gully, the smaller the strata movement and damage degree of the slope rock.
5 Prevention and control measures for water hazard of valley runoff during coal seam mining under the slope
5.1 Conditions of gully runoff water hazard caused by mining under slope
Due to the different engineering geology and technical conditions of coal seam mining, the mining-induced damage degree and scope of rock strata in the slope are different. Based on the previous research on the mining of coal seams under the gully slope, the conditions that cause the water inrush hazard of gully runoff during the slope mining can be summarized as follows: 1) water source conditions 2) catchment channel conditions 3) water inrush pathway conditions (as shown in Figure 12). When the conditions of water source, catchment channel and water inrush pathway are reached together, the gully runoff will pose a threat to safe mining when the working face is advanced under the gully slope. In addition, due to the fragile ecological environment in the gully development area and the scarcity of surface runoff, the gully runoff is the main water source for surface vegetation and animal husbandry activities. Therefore, mining under the slope not only causes dangerous mining production, but also damages the ecological environment of the gully runoff basin, even causes the cutoff of downstream rivers.
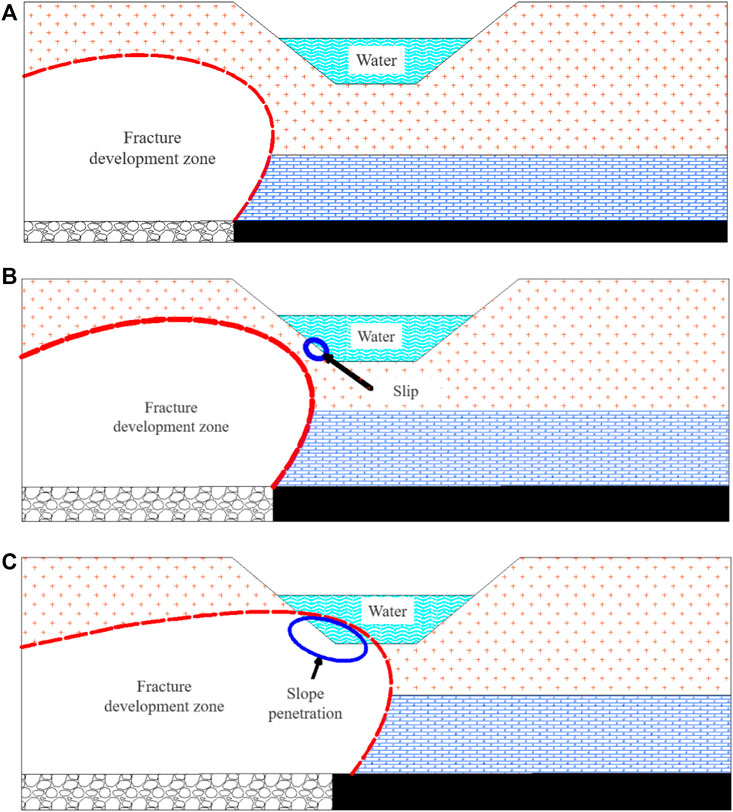
FIGURE 12. Development process of mining-induced fractures. (A) Early stage of cross-gully mining (B) Interim stage of cross-gully mining (C) Late stage of cross-gully mining.
5.2 Setting of safety protection pillar for mining under slope
Through the experimental analysis of the movement characteristics of the mining slope, when the development height of mining-induced fractures in overburden (Hmax) after coal mining is greater than the burial depth of the coal seam (hb) at the gully bottom, the overburden movement of the slope are obviously different from those of conventional mining, and the Kmax can be calculated as follows:
If Kmax < 1, the mining-induced fracture of overburden in the stope has not developed to the bottom of the gully. The slope is located in the bending subsidence zone, which belongs to the subsidence deformation mining slope, and the surface water at the bottom of the gully is not connected with the fractured zone. In addition, when there is a certain thickness of rock (soil) protective layer under the gully bottom, the seasonal surface runoff of the valley poses a small threat to the safety of coal mining below.
If Kmax ≥ 1, the development height of mining-induced fractures in the overlying strata of the stope is above the gully bottom. When the burial depth of the coal seam is shallow, the mining-induced fracture can develop to the surface. The slope-valley system is seriously affected by mining and belongs to the fractured mining slope. When the height of the caving zone is greater than the burial depth of the coal seam at the gully bottom, the slope-valley system is more seriously affected by mining. Part of the rock strata at the lower slope will collapse, forming the caved mining slope. Whether it is a fractured or caved mining slope, the surface water at the bottom of the gully will be connected with the fracture zone, threatening the safety of the working face.
In the actual mining process, only the coal seam mining factors are fixed, the valley shape, overburden structure combination, hydrogeology and other characteristics are changeable. Based on the simulation results, a generalized model of safety coal pillar setting under the slope is established, as shown in Figure 13, and the following simplified assumptions are made:
(1) The gully bottom is the flat bottom interface of surface runoff, and the upper boundary of the water body is determined according to the highest flood level in history.
(2) The overburden and rock strata at the bottom of the slope are horizontal layers.
(3) In the advancing process from the adjacent area to the slope, the development height of mining-induced fractures in overburden has exceeded the bottom surface of valley surface runoff.
In Figure 13, v is the vertical height from the gully bottom interface to the mining seam roof; α1 and α2 are the slope angles of the slopes on both sides of the valley; W1 and W2 are the horizontal widths of the water body overflowing the slope bottom on both sides of the valley when the valley surface runoff reaches the highest flood level; W3 is the valley width; L1 and L2 refer to the horizontal safe distance between the working face and the slope bottom when mining the coal seam under the slope, and L3 refers to the width of the waterproof protective coal pillar.
According to the generalized model of safety coal pillar setting for mining under the slope, the most important thing is to determine the width of the safety coal pillar (L3) for gully runoff. T prevent the connection of the gully surface and overburden mining-induced fractures through horizontal slipping cracks at the rock stratum at the slope bottom, there is a safe distance between the working face and the slope. The safe distance of the working face is taken as the index to determine the L3. The overburden movement and mining-induced fracture development characteristics of coal seam mining under slope are the comprehensive results of various influencing factors. In this study, the influence characteristics of four main factors on overburden movement and mining-induced fracture development are analyzed, and the single factor expression between the main factors and the safety distance is fitted based on the results of numerical simulation. To ensure the safety and reliability of the fitting, the maximum value calculated by the above three fitting formulas is taken as the safety distance of the working face. It is assumed that the working face under the gully slope on both sides of the gully is mined towards the gully. According to the gully topography and the generalization model of safety coal pillar width under gully surface runoff in Figure 13, the above analysis of the safety distance is considered, and the width of the safety coal pillar (L3) can be expressed as follows:
① Class I slope:
② Class II slope
The precondition for water hazard threat caused by gully runoff is that the development height of mining-induced fractures has exceeded the gully bottom interface, that is, Kmax ≥ 1. Although local block overturning of the slope will produce reverse fractures to connect with the gully surface during the mining backward the gully, the areas of local block overturning of the slope mainly occur in the middle and upper parts of the slope, and the vertical distance from the gully bottom is large. Therefore, reverse fractures are not the main channel for gully runoff water inrush. Therefore, referring to the Code for coal pillar retention and coal mining in buildings, water bodies, railways and main shafts, the width of the protective coal pillar can be designed according to the rock movement angle δ during the mining backward the gully.
Assuming that there are gully slopes on both sides of the valley, the mining toward the gully is conducted for one side, and the mining backward the gully is conducted for the other side. According to the generalized model of safety coal pillar width established in Figure 13, the safety coal pillar width L3 can be expressed as:
① Class I slope:
② Class II slope
where
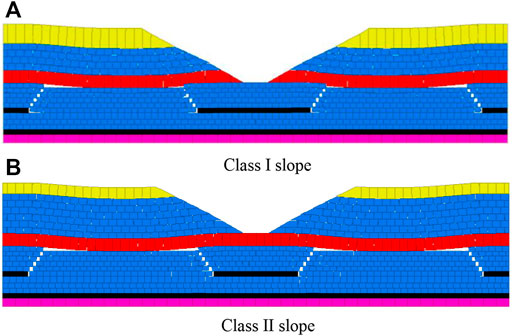
FIGURE 14. Overburden evolution characteristics with safety pillar. (A) Class I slope. (B) Class II slope.
6 Conclusion
(1) In this study, the location of the key layer in the overburden and the cutting characteristics of the overburden are considered, and the occurrence structure characteristics of the gully slope are classified based on the control of the key layer on the rock strata at the bottom of the slope. The Class I slope is represented by the slope with an incomplete key layer, and the Class II slope is represented by the slope with a complete key layer.
(2) The changes in slope angle, slope height, coal seam mining height, and burial depth of coal seam in the gully bottom have different effects on the Class I slope model (with an incomplete key layer) and Class II slope model (with a complete key layer). When there is an incomplete key layer, the damage degree of slope rock is more significant. The greater the slope angle, coal seam mining height and slope height, the more obvious the influence on the strata movement and damage of the slope rock, but the influence of the slope height is weaker than that of the slope angle and mining height. The larger the buried depth of coal seams at the bottom of the gully, the smaller the damage degree caused by the strata movement of the slope rock, and the smaller the scope of horizontal sliding cracks in the slope bottom.
(3) Based on the results of numerical simulation, the relations between the safety distance of the mining face under Class I and Class II slope and the required slope angle α, slope height h0 and the D ratio of the buried depth of the coal seam under the gully bottom and the mining height. Specifically, the safety distance has a non-linear logarithmic positive correlation with the slope angle α, a linear positive correlation with the slope height h0, and a quadratic polynomial negative correlation with the ratio D of the buried depth to the mining height of coal seam under the gully bottom. Based on the generalized model of the safe distance of the working face and the coal pillar setting, the method of setting the safety coal pillar is proposed in this study.
Data availability statement
The datasets presented in this study can be found in online repositories. The names of the repository/repositories and accession number(s) can be found in the article/Supplementary Material.
Author contributions
Conceptualization, YZ and CQ; methodology, CQ and XC; software, XC; formal analysis, ZC; data collection, ZC and JW; writing—original draft preparation, XW, CQ, and ZN; writing-review and editing, ZN.
Funding
This work was supported by the financial supports of General Program of The National Nature Science Foundation of China (51474206, 51874282, and 51874278).
Conflict of interest
The author YZ was employed by Shangyuquan Coal Mine of Shanxi Luneng Hequ Electric Coal Development Co., Ltd.
The remaining authors declare that the research was conducted in the absence of any commercial or financial relationships that could be construed as a potential conflict of interest.
Publisher’s note
All claims expressed in this article are solely those of the authors and do not necessarily represent those of their affiliated organizations, or those of the publisher, the editors and the reviewers. Any product that may be evaluated in this article, or claim that may be made by its manufacturer, is not guaranteed or endorsed by the publisher.
References
Chen, J., Liu, L., Zeng, B., Tao, K., Zhang, C., Zhao, H., et al. (2022). A constitutive model to reveal the anchorage mechanism of fully bonded bolts. Rock Mech. Rock Eng. 11, 8. doi:10.1007/s00603-022-03160-8
Cheng, G., Yang, T., Liu, H., Wei, L., Zhao, Y., Liu, Y., et al. (2020). Characteristics of stratum movement induced by downward longwall mining activities in middle-distance multi-seam. Int. J. Rock Mech. Min. Sci. 136, 104517. doi:10.1016/j.ijrmms.2020.104517
Du, F., and Gao, R. (2017). Development patterns of fractured water-conducting zones in longwall mining of thick coal seams—a case study on safe mining under the zhuozhang river. Energies 10 (11), 1856. doi:10.3390/en10111856
Gui, H., Qiu, H., Chen, Z., Ding, P., Zhao, H., and Li, J. (2020). An overview of surface water hazards in China coal mines and disaster-causing mechanism. Arabian J. Geosciences 13 (2), 67. doi:10.1007/s12517-019-5046-0
Liu, Y., Qi, Q., Wang, A., and Jiang, B. (2021). Influence of valleys terrain on pressure of fully mechanized working faces in shallow coal seams. Shock Vib. 2021, 1–11. doi:10.1155/2021/8880041
Mondal, D., Roy, P. N. S., and Kumar, M. (2020). Monitoring the strata behavior in the Destressed Zone of a shallow Indian longwall panel with hard sandstone cover using Mine-Microseismicity and Borehole Televiewer data. Eng. Geol. 271, 105593. doi:10.1016/j.enggeo.2020.105593
Ning, J., Wang, J., Tan, Y., and Xu, Q. (2020). Mechanical mechanism of overlying strata breaking and development of fractured zone during close-distance coal seam group mining. Int. J. Min. Sci. Technol. 30 (2), 207–215. doi:10.1016/j.ijmst.2019.03.001
Qi, X. R., Li, D., Chu, F., Zhang, J., and Zhang, J. (2019). Study of the height of water flowing fracture zone based on strain energy failure criterion. Earth Environ. Sci. 330, 022065. doi:10.1088/1755-1315/330/2/022065
Qi, Y., Wang, W., Ge, J., Yang, Z., and Qi, Q. (2022). Development characteristics of the rock fracture field in strata overlying a mined coal seam group. PLoS One 17 (10), e0268955. doi:10.1371/journal.pone.0268955
Sainoki, A., Mitri, H. S., Chinnasane, D., and Schwartzkopff, A. K. (2019). Quantitative energy-based evaluation of the intensity of mining-induced seismic activity in a fractured rock mass. Rock Mech. Rock Eng. 52 (11), 4651–4667. doi:10.1007/s00603-019-01861-1
Swift, G. (2013). Relationship between joint movement and mining subsidence. Bull. Eng. Geol. Environ. 73 (1), 163–176. doi:10.1007/s10064-013-0539-7
Tan, Y., Cheng, H., Lv, W., Yan, W., Guo, W., Zhang, Y., et al. (2022). Calculation of the height of the water-conducting fracture zone based on the analysis of critical fracturing of overlying strata. Sustainability 14 (9), 5221. doi:10.3390/su14095221
Tian, C., Liu, Y., Yang, X., Hu, Q., Wang, B., and Yang, H. (2019). Development characteristics and field detection of overburden fracture zone in multiseam mining: A case study. Energy Sci. Eng. 8 (3), 602–615. doi:10.1002/ese3.536
Wang, F., Tu, S., Zhang, C., Zhang, Y., and Bai, Q. (2016). Evolution mechanism of water-flowing zones and control technology for longwall mining in shallow coal seams beneath gully topography. Environ. Earth Sci. 75 (19), 1309. doi:10.1007/s12665-016-6121-4
Wang, Z., Song, G., and Ding, K. (2020). Study on the ground movement in an open-pit mine in the case of combined surface and underground mining. Adv. Mater. Sci. Eng. 2020, 1–13. doi:10.1155/2020/8728653
Xie, X., Hou, E., Wang, S., Sun, X., Hou, P., Wang, S., et al. (2021). Formation mechanism and the height of the water-conducting fractured zone induced by middle deep coal seam mining in a sandy region: A case study from the xiaobaodang coal mine. Adv. Civ. Eng. 2021, 1–11. doi:10.1155/2021/6684202
Yang, T., Yang, Y., Zhang, J., Gao, S., Li, T., and Wu, H. (2021). Study on development law of mining-induced slope fracture in gully mining area. Adv. Civ. Eng. 2021, 1–9. doi:10.1155/2021/9990465
Zeng, Y., Lian, H., Du, X., Tan, X., and Liu, D. (2022). An analog model study on water–sand mixture inrush mechanisms during the mining of shallow coal seams. Mine Water Environ. 41 (2), 428–436. doi:10.1007/s10230-022-00870-x
Zhang, C., Mitra, R., Oh, J., Canbulat, I., and Hebblewhite, B. (2017). Numerical analysis on mining-induced fracture development around river valleys. Int. J. Min. Reclam. Environ. 32 (7), 463–485. doi:10.1080/17480930.2017.1293495
Zhang, Q., and López, D. L. (2019)., 38. Ohio, 566–580. doi:10.1007/s10230-019-00619-zUse of time series analysis to evaluate the impacts of underground mining on the hydraulic properties of groundwater of dysart woods, OhioMine Water Environ.3
Zhou, Y., and Yu, X. (2022). Study of the evolution of water-conducting fracture zones in overlying rock of a fully mechanized caving face in gently inclined extra-thick coal seams. Appl. Sci. 12 (18), 9057. doi:10.3390/app12189057
Keywords: valley terrain, rock strata evolution, mining-induced fractures, runoff water, water-conducting fracture zone
Citation: Zhang Y, Qian C, Wang X, Chen X, Chang Z, Wang J and Niu Z (2023) Study on prevention and control mechanism of runoff water hazard in thick coal seam mining in valley terrain. Front. Earth Sci. 11:1140491. doi: 10.3389/feart.2023.1140491
Received: 09 January 2023; Accepted: 10 February 2023;
Published: 21 February 2023.
Edited by:
Danqi Li, Curtin University, AustraliaReviewed by:
Jianhang Chen, China University of Mining and Technology, Beijing, ChinaSaisai Wu, Xi’an University of Architecture and Technology, China
Copyright © 2023 Zhang, Qian, Wang, Chen, Chang, Wang and Niu. This is an open-access article distributed under the terms of the Creative Commons Attribution License (CC BY). The use, distribution or reproduction in other forums is permitted, provided the original author(s) and the copyright owner(s) are credited and that the original publication in this journal is cited, in accordance with accepted academic practice. No use, distribution or reproduction is permitted which does not comply with these terms.
*Correspondence: Chenlong Qian, Y2hlbmxvbmdfcWlhbkAxNjMuY29t