- 1Tianjin Key Laboratory for Oceanic Meteorology, Tianjin, China
- 2Tianjin Institute of Meteorological Science, Tianjin, China
- 3State Key Laboratory of Numerical Modeling for Atmospheric Sciences and Geophysical Fluid Dynamics, Institute of Atmospheric Physics, Chinese Academy of Sciences, Beijing, China
This study analyzes the frequency characteristics of tropical cyclone (TC) genesis in the southeastern part of the western North Pacific (SEWNP) during June–November from 1965 to 2019 and investigates the possible combined effect of the solar activity and El Niño-Southern Oscillation (ENSO). Results suggest that TCs generated in the SEWNP have the longest lifetime and greatest strength, and its frequency has apparent interannual and decadal variations, which is jointly affected by the solar activity and ENSO. In El Niño years during declining phases of solar cycle (1–3 years following the solar maximum), positive TC genesis frequency anomalies in the SEWNP are significantly strong and tend to occur in extremes. While the opposite is true for La Niña during ascending phases of solar cycle (1–3 years following the solar minimum). However, there exists no significant feature in the combined effect of La Niña (El Niño) and declining (ascending) phases of solar cycle. When declining (ascending) phases of solar cycle and El Niño (La Niña) are combined, the overlapping effect leads to apparently warmer (colder) sea surface temperature (SST) anomalies in the central equatorial Pacific and colder (warmer) SST anomalies in the western Pacific, so the SST anomalies gradient are stronger. It enhances low-level westerly (easterly) wind anomalies and upper-level easterly (westerly) wind anomalies, which is favorable for the further decrease (increase) of the vertical wind shear in the eastern (most) part of SEWNP. Moreover, the stronger and more westward low-level convergence (divergence) center appears in the Pacific, causing stronger low-level convergent (divergent) flow and upper-level divergent (convergent) flow anomalies, and strengthened (suppressed) ascending movement anomalies in the SEWNP. As a result, the TC genesis frequency in the SEWNP is much higher (lower). Further diagnoses show that absolute vorticity plays a leading role in El Niño years during declining phases of solar cycle, and its contribution is obviously much greater than other environmental factors. It is not perfectly symmetrical in La Niña years during ascending phases of solar cycle when the contribution of absolute vorticity is the greatest, but vertical wind shear is also important.
1 Introduction
Tropical cyclones (TCs) could not only bring severe weather with significant casualties and economic losses, but also make an impact on the atmospheric circulation (Zhong, 2006; Ren et al., 2007; Zhong and Hu, 2007; Chen et al., 2017; Wang et al., 2019; Ling and Lu, 2022), so understanding the TC activity is of great importance. TC genesis has attracted considerable attention, but its prediction has been a difficult topic for the past few decades (Cao et al., 2022). The western North Pacific (WNP) is known to be the most prolific tropical cyclone basin, accounting for about 1/3 of all the global TCs (Chan, 2005; Huang and Chen, 2007; Woodruff et al., 2013), and the southeastern part of the WNP (SEWNP) is an important key region for the TC genesis (Wang and Chan, 2002; Camargo and Sobel, 2005; Zhang et al., 2017; Liu et al., 2019; Shan and Yu, 2021; Cao et al., 2022; Song et al., 2022). Zhang et al. (2017) found that the Atlantic meridional mode affects TC activity in the WNP mainly through changes in the TC genesis in the SEWNP. What is more, the TC genesis frequency in the SEWNP is associated with that in the eastern North Pacific and tropical North Atlantic Ocean. When more TCs generate in the SEWNP, more TCs generate in the eastern North Pacific, but less in the tropical North Atlantic Ocean (Cao et al., 2022). The sudden decrease in TC genesis frequency in the SEWNP also leads to the abrupt reduction of the frequency of TC landfall in southern China in the post-peak season, owing to the abrupt decline in westward-moving tracks (Shan and Yu, 2021). In addition, the increased TC activity in the SEWNP contributes significantly to the intensity of El Niño after 3 months by weakening the Walker circulation and strengthening the eastward propagating oceanic Kelvin waves in the tropical Pacific (Wang et al., 2019). Consequently, the TC genesis in the SEWNP is noteworthy.
As the most significant signal of interannual variability in the coupled atmosphere-ocean system, El Niño-Southern Oscillation (ENSO) plays a crucial role in TC genesis in the WNP (Pan, 1982; Chan, 1985; Li, 1987; Lander, 1994; Chen et al., 1998; Chan, 2000; Wang and Chan, 2002; Elsner and Liu, 2003; Chu, 2004; Camargo et al., 2007; Kim et al., 2011, 2016; Zhan et al., 2011; Wang et al., 2014; Zhao and Wang, 2019; Song et al., 2022). A general consensus is that the relationship between ENSO and TC genesis frequency is weak in the entire WNP, but ENSO remarkably affects the shift of TC genesis location. The TC genesis frequency increases in the SEWNP and decreases in the northwestern part of the WNP (NWWNP) during El Niño years. Wang and Chan (2002) attributed the more TCs in the SEWNP to the increase of the low-level vorticity, and fewer TCs in the NWWNP to the upper-level convergence induced by the strengthening of the East Asian trough and WNP subtropical high, which are all forced by El Niño. Camargo and Sobel (2005) suggested that more TCs generated in the SEWNP take relatively longer time to make landfall or encounter colder mid-latitude water during El Niño years, which results in more opportunities to obtain energy and enhance TC intensity.
Besides the interannual variation, the frequency of TC genesis in the WNP has the decadal feature, which is modulated by several decadal influencing factors. The solar activity has a remarkable quasi-11-year cycle and plays an important role in driving climate (Herschel, 1801; Meehl et al., 2008; Gray et al., 2010). As previous studies have shown, the solar activity exerts an influence on sea surface temperature (SST) in the central tropical Pacific (Kodera et al., 2016; Huo et al., 2021; Lin et al., 2021), and El Niño Modoki events are found within 1–3 years following the solar maximum (Huo and Xiao, 2016, 2017). Huo and Xiao (2017) proposed two mechanisms to explain it. One is the direct effect that the lagging warming response of the central tropical Pacific to the solar radiation is amplified by the coupled atmosphere-ocean processes. The other is the indirect effect that the anomalous atmospheric circulation at mid-high latitudes is modulated by the heating effect propagating from the upper atmosphere, which may trigger an El Niño Modoki event in the 1–3 years following the solar maximum through wind-evaporation-SST feedback and the seasonal footprint mechanism. Enfield and Cid (1991) found that the persistence of ENSO is shorter (longer) in low (high) solar activity years. In addition, the solar activity could also modulate the impact of ENSO on the Pacific North American teleconnection, South Asia high, East Asian winter monsoon, and precipitation in southern China (Huth et al., 2006; Zhou et al., 2013; Liu et al., 2014; Li and Xiao, 2018; Xue et al., 2020; Ma et al., 2021; Wang et al., 2021).
Although Li et al. (2019) have pointed out that the solar activity is closely related to all the global TC genesis frequency, the relationship between the solar activity and TC genesis frequency in the WNP is less studied. It is still unclear whether the influence of ENSO on the TC genesis frequency in the WNP depends on the solar activity. Considering the significance of the SEWNP, this study focuses mainly on the modulation of the effect of ENSO on the TC genesis frequency in the SEWNP by different solar activities to provide evidence for the forecast of TC genesis frequency in the SEWNP and improve the forecast accuracy.
2 Data and methods
In this study, the TC best-track data are derived from the Shanghai Typhoon Institute of the China Meteorological Administration (CMA), including the information on TC location (latitude and longitude) and intensity at 6-h intervals during 1965–2019 (Ying et al., 2014; Lu et al., 2021). Owing to the uncertainty of tropical depression, a TC here refers to reaching tropical storm intensity (17.2 m s-2) and generating in the west of 180°C in the WNP. The TC genesis location is defined as the position where the TC reaches tropical storm intensity at the first time. As suggested by Cao et al. (2022), the WNP is divided into southeastern (5°N–15°N, 140°E−180°), northeastern (15°N–30°N, 140°E−180°), northwestern (15°N–30°N, 120°E−140°E) and southwestern (5°N–15°N, 120°E−140°E) regions (Figure 1). This analysis focuses on the active TC season in the WNP, which is from June to November (JJASON).
The monthly atmospheric fields including wind, temperature, relative humidity, specific humidity, sea level pressure, total cloud cover, and surface heat flux are obtained from the National Centers for Environmental Prediction and National Center for Atmospheric Research (NCEP/NCAR) reanalysis dataset on 2.5°C × 2.5°C grids (Kalnay et al., 1996). The monthly SST data are taken from the National Oceanic and Atmospheric Administration (NOAA)’s Extended Reconstructed SST version 5 (ERSST.v5) with a horizontal resolution of 2°C × 2°C (Huang et al., 2017). The data cover the period 1965–2019, and the linear trend is removed before the analysis. The power spectrum, Pearson correlation, linear regression, and composite analysis are used in this work, and the statistical significance is evaluated based on the Student t-test. According to Yan et al. (2004) and Yu et al. (2019), the effective degree of freedom of low-pass filtering is calculated.
The Niño3.4 index (5°S–5°N, 170°W–120°W) is from the NOAA’s Climate Prediction Center. We identify ENSO based on the ±0.5 standard deviations of the June–November-averaged Niño3.4 index. An El Niño (La Niña) event is identified when the Niño3.4 index is greater (less) than + (−) 0.5 standard deviations. The sunspot numbers (SSN) index from the World Data Center at the Royal Observatory of Belgium is used to quantify the solar activity. An 11-year fast Fourier low-pass filtering is applied to the June–November-averaged SSN index to obtain the solar maximum (minimum), which are greater (less) than 0 standard deviations. The declining (ascending) phase of solar cycle is then defined as the 1–3 years following the solar maximum (minimum).
To quantitatively diagnose the contributions of different environmental factors to TC genesis, the genesis potential index (GPI) from Emanuel and Nolan (2004) is employed, which is calculated as follows:
Where
where the bar represents the climatological mean, and the prime indicates the deviation from the climatological mean. The four terms in the right-hand side of the above equation denote the contributions of lower-level absolute vorticity, vertical wind shear between 200 hPa and 850 hPa, mid-level relative humidity, and PI, respectively.
3 Results
3.1 Frequency characteristics of TC genesis in the SEWNP and NWWNP
Characteristics of TCs generated in the four subregions of the WNP are shown in Table 1. In the NWWNP, the average TC genesis frequency in JJASON during 1965–2019 is 5.9 per year, with the highest in the four subregions of the WNP, and there is an insignificant linear decreasing trend with a value of −0.01 per year. The NWWNP is so close to East Asia that more TCs generated here tend to have a serious impact on East Asia. Compared to that in the NWWNP and northeastern part of the WNP (NEWNP), TC genesis frequency in the SEWNP of 3.8 is lower, with a linear trend of −0.04 at the 90% confidence level. However, TCs generated in the SEWNP have the longest average lifetime of 202.7 h, which far exceeds the second value of 154.4 h in the southwestern part of the WNP (SWWNP). The shortest average TC lifetime appears in the NWWNP with a value of 129.6 h. Additionally, the strongest TCs generate in the SEWNP with the average annual accumulated cyclone energy (ACE) of 2 × 105 m2 s-2, while the smallest ACE is in the SWWNP. To further confirm the feature, TC genesis frequency reaching typhoon intensity (32.7 m s-1) and super typhoon intensity (51 m s-1) are checked. It is found that in the SEWNP, there are 196 TCs reaching typhoon grade, accounting for 93% of the total number, while 131 TCs reaching super typhoon grade, accounting for 62% of the total number. It outdistances that in the other three subregions, which also verifies TCs generated in the SEWNP are the strongest in general. To sum up, TCs generated in the SEWNP have the longest lifetime and the greatest strength, while the TC genesis frequency in the NWWNP is the highest. Furthermore, ENSO has a great influence on the TC genesis in the SEWNP and NWWNP (Lander, 1994; Chen et al., 1998; Wang and Chan, 2002; Elsner and Liu, 2003; Chu, 2004; Camargo et al., 2007; Kim et al., 2011; Zhan et al., 2011; Song et al., 2022). Therefore, it is of great significance to study the TC genesis in the SEWNP and NWWNP, which is the focus of our analysis below.
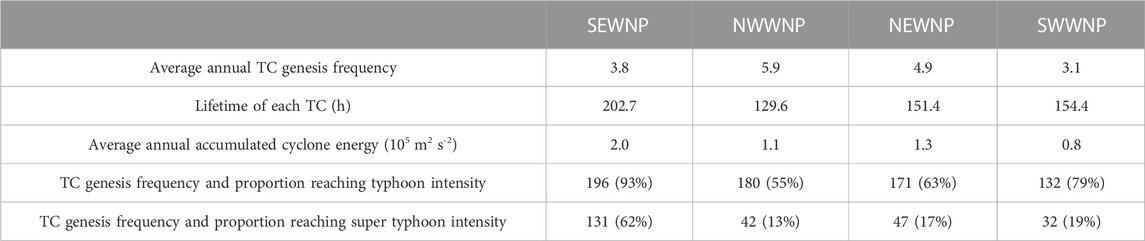
TABLE 1. Characteristics of TCs generated in the four subregions of the WNP in JJASON during 1965–2019.
As can be seen in Figure 2, there are apparent interannual and decadal variations in the TC genesis frequency in the SEWNP, with the highest TC genesis frequency occurring in 1972 and 1994, which is approximately 9 TCs. The corresponding power spectrum of TC genesis frequency in the SEWNP shows obvious peaks at cycles of 2–4 years and 11 years, in which the 11-year cycle is the most significant (Figure 3A). By means of filtering, the decadal variance contribution is proved to be significant, explaining 26% of the total variance. Therefore, in addition to the interannual influence, the TC genesis frequency in the SEWNP may be modulated by decadal factors. It is well known that solar activity has a remarkable quasi-11-year cycle and ENSO is the strongest signal of interannual variation in the coupled atmosphere-ocean system. Thus, a hypothesis naturally comes out that the TC genesis frequency in the SEWNP may be jointly affected by solar activity and ENSO. With regards to the TC genesis frequency in the NWWNP, the interannual variation is dominant and the highest TC genesis frequency occurred in 1966, 1978, 1989, and 1993, with about 10 TCs (Figure 2). The power spectrum also confirms that it has a significant peak at cycles of 3–4 years (Figure 3B). The TC genesis frequency in the NWWNP is primarily influenced by interannual factors.
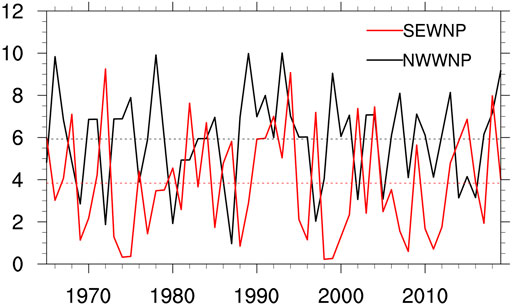
FIGURE 2. Time series of TC genesis frequency in the SEWNP (red line) and NWWNP (black line) in JJASON during 1965–2019. The dotted line denotes the average.
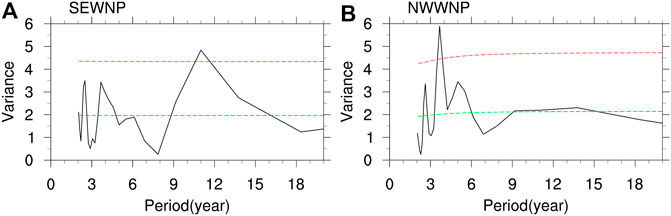
FIGURE 3. Power spectrum analysis of TC genesis frequency in the (A) SEWNP and (B) NWWNP. The green line indicates the red noise spectrum and the red line denotes statistical significance at the 95% confidence level.
3.2 Association between ENSO and TC genesis frequency in the SEWNP and NWWNP during different solar cycle phases
Previous studies have verified that ENSO plays an important role in TC genesis frequency in the SEWNP and NWWNP (Lander, 1994; Chen et al., 1998; Wang and Chan, 2002; Elsner and Liu, 2003; Chu, 2004; Camargo et al., 2007; Kim et al., 2011; Zhan et al., 2011; Song et al., 2022). The researchers suggested that El Niño contributes to the increase of TC genesis frequency in the SEWNP and the decrease in the NWWNP, while the effect of La Niña is roughly opposite. We calculated the correlation coefficient again and found a highly strong correlation between TC genesis frequency in the SEWNP and Niño3.4 index in JJASON during 1965–2019, with a value of 0.74 at the 99% confidence level. The TC genesis frequency in the NWWNP has a significant negative correlation with Niño3.4 index, with a value of −0.41 at the 99% confidence level. These results are consistent with the previous findings. Then what are the relationships between the SSN index and TC genesis frequency in the SEWNP and NWWNP on the decadal time scale? As demonstrated in the lead-lag correlation on the decadal time scale, TC genesis frequency in the SEWNP has a significant positive correlation with the SSN index in the leading 1–3 years (Table 2). When the SSN index leads 2–3 years, the correlation coefficients of 0.77 and 0.75 are the largest, both at the 99% confidence level. In addition, no obvious correlations are found between the TC genesis frequency in the NWWNP and the SSN index in the leading 1–3 years. In consequence, the relationship between the SSN index and TC genesis frequency in the SEWNP is different from that in the NWWNP, meaning that the declining phase of solar cycle only favors increasing TC genesis frequency in the SEWNP. It is unlike El Niño, which also has an impact on the TC genesis frequency in the NWWNP.

TABLE 2. Correlation coefficients of TC genesis frequency in the SEWNP with the SSN index in the leading 0–3 years on the decadal time scale in JJASON during 1965–2019. * (***) denotes the 90% (99%) confidence level.
According to the above analysis, the TC genesis frequency in the SEWNP has a significant positive correlation with the SSN index on the decadal time scale. Furthermore, the decadal variance contribution of the TC genesis frequency in the SEWNP is large, so can the influence of ENSO on TC genesis frequency in the SEWNP be modulated by the solar activity? The previous studies indicated that the influence of solar activity on the tropical Pacific SST lags for more than 1 year (Huo and Xiao, 2016, 2017), and TC genesis frequency in the SEWNP is significantly related to the SSN index in the leading 1–3 years. Thus, the following study focuses mainly on the situation of the SSN index in the leading 1–3 years in detail. As described in Part 2 of this study, the declining (ascending) phase of solar cycle is defined as the 1–3 years following the solar maximum (minimum). During declining phases of solar cycle, El Niño events are associated with significantly strong positive TC genesis frequency anomalies in the SEWNP. It tends to occur in extreme value, and the standardized anomalies exceed +1.0 in the overwhelming majority of El Niño years. TCs in the SEWNP are more numerous, with the mean of +1.1, +1.0, and +1.3 in the 1–3 years following the solar maximum, passing the 95% or 99% confidence level, respectively (Table 3). During ascending phases of solar cycle, there are negative or relatively weak positive TC genesis frequency anomalies in the SEWNP in El Niño years, and the mean of +0.6, +0.5, and +0.3 are all not significant. On the contrary, in La Niña years, there are generally obviously fewer TCs and the average anomalies are −0.9, −1.2, and −1.2 at the 99% confidence level in the 1–3 years following the solar minimum. However, in La Niña years during declining phases of solar cycle, there are nearly half more-TC years and the average negative anomalies of −0.4, 0, and −0.3 are all weak.
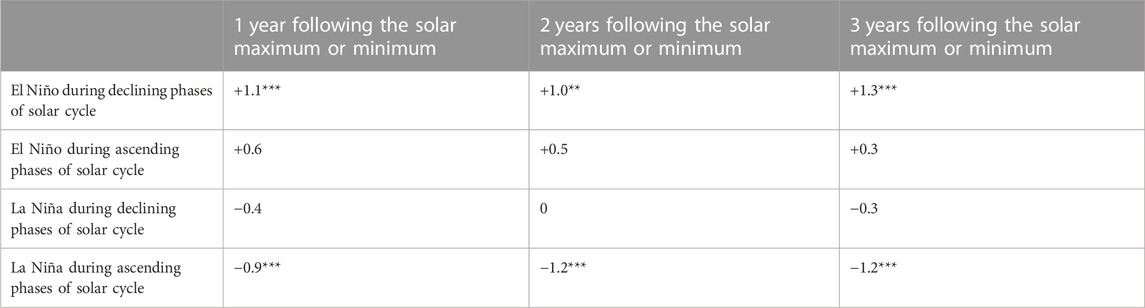
TABLE 3. Mean standardized TC genesis frequency in the SEWNP in El Niño and La Niña years during different solar cycle phases from 1965 to 2019. ** (***) denotes the 95% (99%) confidence level.
For the combined impact of ENSO and solar cycle phases, it is the most obvious in the 3 years following the solar maximum and minimum, so take this as an example to show the detailed anomalous TC genesis frequency in the SEWNP (Table 4). As can be seen, during El Niño in the 3 years following the solar maximum, TC genesis frequency anomalies are greater than or equal to +0.8 in all years, except 2006 when it is only −0.1. As a result, the positive anomalies are quite high. However, during El Niño in the 3 years following the solar minimum, TC genesis frequency anomalies are smaller than −1.0 for 2 years, and weak positive anomalies also appear for 2 years in all 8 years. Similarly, La Niña events are associated with all the negative TC genesis frequency anomalies smaller than or equal to −0.9 in the 3 years following the solar minimum, while this feature is weak in the 3 years following the solar maximum. To sum up, with regards to the TC genesis frequency in the SEWNP influenced by ENSO, solar activity is an important modulation in the decadal background. In El Niño (La Niña) years during declining (ascending) phases of solar cycle, there are significantly strong positive (negative) TC genesis frequency anomalies in the SEWNP, which may be synergistic. Resulting from the opposite effect of El Niño (La Niña) and ascending (declining) phases of solar cycle, TC genesis frequency anomalies in the SEWNP have no significant feature.

TABLE 4. Standardized TC genesis frequency in the SEWNP during ENSO in the 3 years following the solar maximum and solar minimum from 1965 to 2019.
Although there is no significant lead-lag relationship between TC genesis frequency in the NWWNP and the SSN index on the decadal time scale, it is hard to judge whether solar activity modulates the effect of ENSO on the TC genesis frequency in the NWWNP or not. It is essential to carry out statistical analysis in detail. In El Niño years during ascending phases of solar cycle, the TCs in the NWWNP are significantly reduced. However, the effects of El Niño and ascending phases of solar cycle are out of phase, so TC genesis frequency in the NWWNP is dominated by El Niño, which is less modulated by ascending phases of solar cycle. In addition, the TC genesis frequency anomalies in the NWWNP are all not significant in the combined effects of La Niña and ascending or declining phases of solar cycle. Consequently, the modulation of ENSO’s influence on the TC genesis frequency in the NWWNP by solar activity is little.
3.3 Physical mechanism for the solar activity to modulate the effect of ENSO on TC genesis frequency in the SEWNP
According to the previous studies, the solar activity has an effect on the anomalous SST in the central equatorial Pacific (Huo and Xiao, 2016, 2017; Kodera et al., 2016; Huo et al., 2021; Lin et al., 2021), so the SST in the central equatorial Pacific may be the key modulated by solar activity in the influencing process of ENSO on TC genesis frequency in the SEWNP. To explain it, the anomalous SST, cloud cover, and surface heat fluxes in the 1–3 years following the solar activity are regressed onto the SSN index on the decadal time scale (Figure 4), which are primarily alike from the lagging 1 year to the lagging 3 years. The downward (upward) surface heat flux is defined as the positive (negative). During the declining phases of solar cycle, the negative shortwave radiation flux anomalies appear in the western equatorial Pacific and positive anomalies in the central-eastern equatorial Pacific (Figure 4A), which contributes to the negative and positive SST anomalies in the western and central-eastern equatorial Pacific, respectively (Figure 4B). It is well known that the shortwave radiation flux is dominantly controlled by the cloud cover. The distribution of the cloud cover anomalies is also essentially in agreement with that of the shortwave radiation flux anomalies in the equatorial Pacific (Figure 4D). Meanwhile, the westerly wind anomalies over the central equatorial Pacific on account of the zonal SST anomalies gradient reduce the climatological trade winds, which reduces the surface evaporation and upward latent heat flux (Figure 4C). Besides, the longwave radiation flux anomalies are negative and sensible heat flux anomalies are positive in the central equatorial Pacific (Figure omitted). Thus, the positive net heat flux anomalies (Figure 4E) contribute to the warm SST anomalies in the central equatorial Pacific. In consequence, by means of the direct radiative effect of solar activity and wind-evaporation-SST feedback, the declining phases of the solar cycle cause warm SST anomalies in the central equatorial Pacific, which verifies the findings of Huo and Xiao (2017) and Huo et al. (2021).
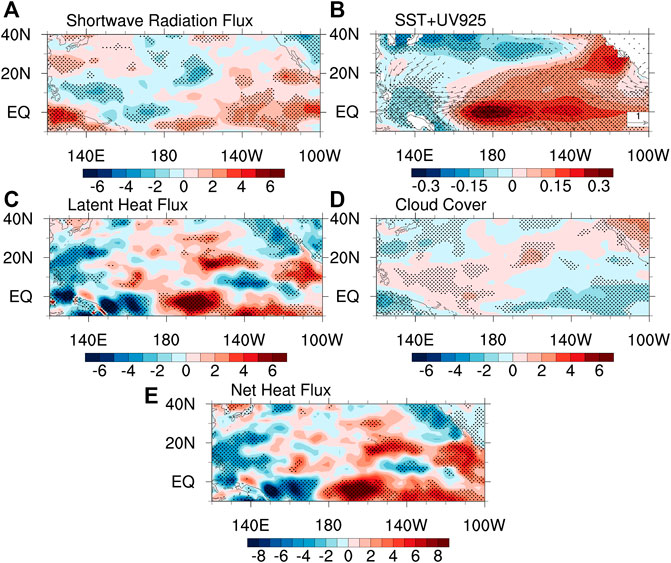
FIGURE 4. Regression of (A) shortwave radiation flux anomalies (units: W m−2), (B) SST anomalies (shaded; units: °C) and wind anomalies at 925 hPa (vector; units: m s−1; vectors denote statistical significance at the 95% confidence level), (C) latent heat flux anomalies (units: W m−2), (D) cloud cover anomalies (units: %), and (E) net heat flux anomalies (units: W m−2) in the lagging 2 years onto the SSN index on the decadal time scale. Dots indicate statistical significance at the 95% confidence level.
Then how does the solar activity modulate the effect of ENSO on TC genesis frequency in the SEWNP through anomalous atmospheric circulation? Because the distributions of anomalous SST and atmospheric variables during ENSO in the 1–3 years following the solar maximum or solar minimum are basically similar, the physical fields from the following 1 year to the following 3 years are averagely composited. As shown in the composited SST and wind anomalies at 925 hPa (Figures 5A, B), owing to the effect of solar cycle phases, compared to El Niño during ascending phases of solar cycle, there are apparently warmer SST anomalies in the central equatorial Pacific and colder SST anomalies in the western Pacific in El Niño years during declining phases of solar cycle, along with the stronger SST gradient anomalies between the central equatorial Pacific and western Pacific. Therefore, the overlapping of declining phases of solar cycle and El Niño possibly enhances the low-level westerly wind anomalies in the SEWNP, where the upper-level easterly wind anomalies are also stronger. In the climatological mean, the SEWNP is dominated by the easterly wind at low levels, while dominated by the westerly wind at eastern high levels and the easterly wind at western high levels. As a result, the vertical wind shear between 200 hPa and 850 hPa in the eastern SEWNP is further reduced, which is more favorable for TC genesis in the eastern SEWNP. Additionally, it can be seen in the low-level velocity potential and divergent wind anomalies (Figures 6A, B) that a stronger and more westward convergence center exists in the Pacific in the combined effect of El Niño and declining phases of solar cycle, which is conducive to the stronger low-level convergent flow and high-level divergent flow anomalies in the SEWNP (Figures 7A, B). Correspondingly, the ascending movement and convection anomalies are strengthened, leading to more TCs in the SEWNP.
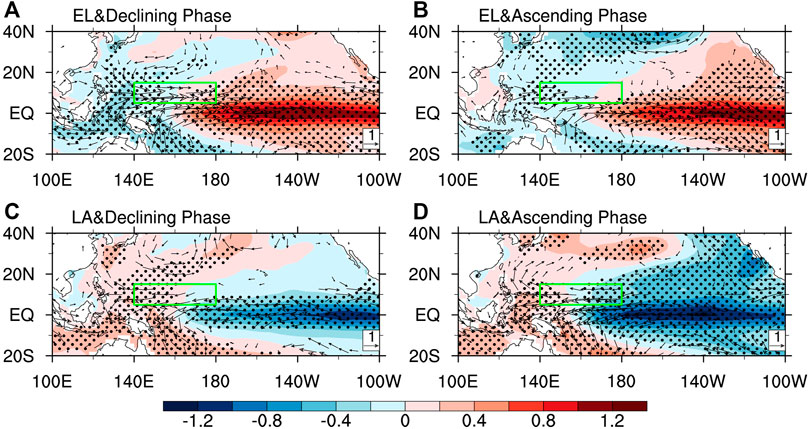
FIGURE 5. Composite SST anomalies (shaded; units: °C) and wind anomalies at 925 hPa (vector; units: m s−1) in JJASON in (A), (B) El Niño and (C), (D) La Niña years during different solar cycle phases. Dots (vectors) indicate SST (wind) anomalies are significant at the 99% confidence level.
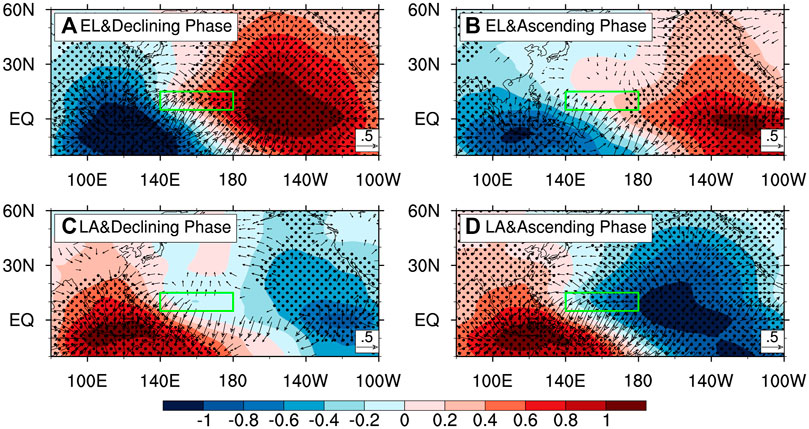
FIGURE 6. Composite velocity potential anomalies (shaded; units: 106 m2 s−1) and divergent wind anomalies at 925 hPa (vector; units: m s−1) in JJASON in (A), (B) El Niño and (C), (D) La Niña years during different solar cycle phases. Dots (vectors) indicate velocity potential (divergent wind) anomalies are significant at the 99% confidence level.
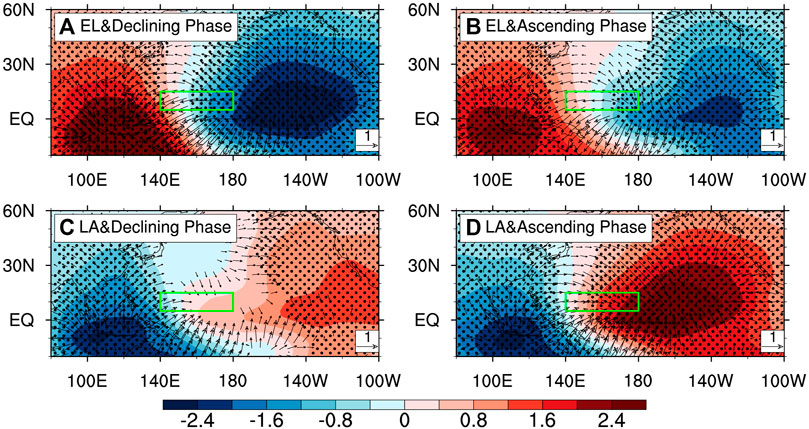
FIGURE 7. Composite velocity potential anomalies (shaded; units: 106 m2 s−1) and divergent wind anomalies at 200 hPa (vector; units: m s−1) in JJASON in (A), (B) El Niño and (C), (D) La Niña years during different solar cycle phases. Dots (vectors) indicate velocity potential (divergent wind) anomalies are significant at the 99% confidence level.
Compared to the declining phases of solar cycle, La Niña brings the obviously colder SST anomalies in the central equatorial Pacific and warmer SST anomalies in the western Pacific during ascending phases of solar cycle (Figures 5C, D). As a result, the SST anomalies gradient are stronger and low-level easterly wind and upper-level westerly anomalies are also stronger in the SEWNP. It further increases the vertical wind shear between 200 hPa and 850 hPa in most parts of the SEWNP, resulting in the further suppression of TC genesis. In La Niña years during ascending phases of solar cycle, the low-level divergent and high-level convergent flow anomalies in the SEWNP become more pronounced (Figures 6C, 6D, 7C, 7D) and the descending movement anomalies become more enhanced, accompanied with weaker humidity and convection, which suppress TC genesis in the SEWNP in further.
The vertical wind shear, low-level vorticity, and middle-level relative humidity are three important environment factors for TC genesis (Gao et al., 2018; Liu and Chan, 2018). In El Niño years during declining phases of solar cycle, the warmer SST anomalies in the central equatorial Pacific induce a stronger Rossby wave response in the northwest compared to the ascending phases of solar cycle, causing the stronger cyclonic circulation anomalies. As a result, the positive low-level relative vorticity anomalies are further intensified in the SEWNP (Figures 8A, B), which is beneficial to more TCs. It exhibits different characteristics of the vertical wind shear anomalies in the eastern and western SEWNP, that is the stronger positive anomalies are in the western SEWNP, while the stronger negative anomalies are in the eastern SEWNP (Figures 8E, F). Thus, the vertical wind shear favors TC genesis in the eastern SEWNP and suppresses TC genesis in the western SEWNP. In terms of middle-level relative humidity, during declining phases of solar cycle, El Niño events are associated with stronger positive relative humidity anomalies in most parts of the SEWNP compared to the ascending phases of solar cycle (Figures 8I, J). It is more favorable for TC genesis and leads to higher TC genesis frequency in the SEWNP. In La Niña years during ascending phases of solar cycle, the negative relative vorticity anomalies are stronger in the SEWNP (Figures 6C, D), and the positive vertical wind shear anomalies (Figures 8G, H) and the negative relative humidity anomalies (Figures 8K, L) are also stronger in most parts of the SEWNP compared to the declining phases of solar cycle. These environment conditions further inhibit TC genesis, resulting in the lower TC genesis frequency in the SEWNP.
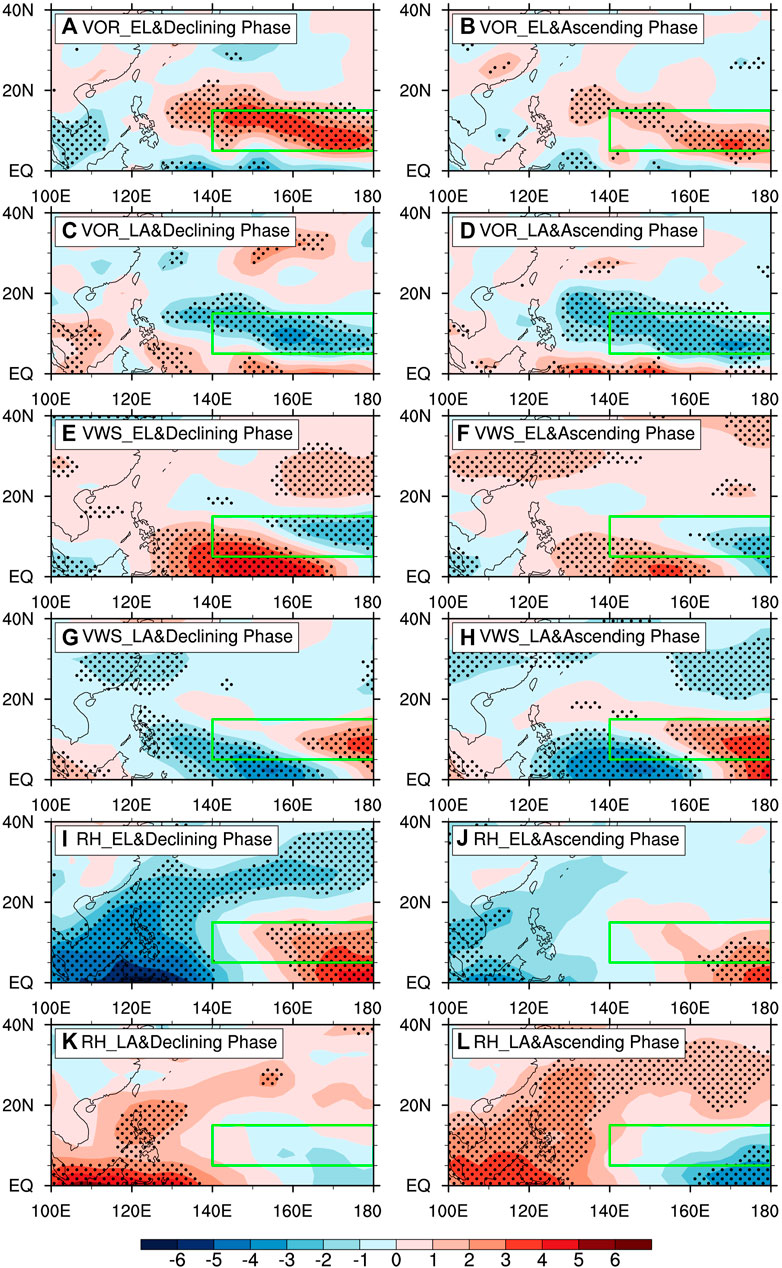
FIGURE 8. Composite (A–D) relative vorticity anomalies (units: 10–6 s−1), (E–H) vertical wind shear anomalies between 200 hPa and 850 hPa (units: m s−1), and (I–L) relative humidity anomalies at 600 hPa (units: %) in JJASON during ENSO in different solar cycle phases. Dots indicate statistical significance at the 99% confidence level.
To ascertain which environmental factor plays a dominant role in TC genesis, the GPI is employed to quantitatively diagnose the relative contributions of environmental factors to TC genesis in the SEWNP. The detailed calculation method of the anomalous GPI is described in Part 2 of this study, which consists of the low-level absolute vorticity, vertical wind shear between 200 hPa and 850 hPa, mid-level relative humidity, and PI terms. Cao et al. (2022) pointed out that absolute vorticity contributes the most to the GPI anomalies in the SEWNP, along with the secondary contribution of relative humidity and vertical wind shear terms. How is it in our study? On account of the overlapping effect of the declining (ascending) phases of solar cycle and El Niño (La Niña), the regional averages of the four terms in the SEWNP are composited in El Niño (La Niña) years during declining (ascending) phases of solar cycle (Figure 9). As shown in Figure 9A, the positive GPI anomalies appear in the SEWNP, corresponding to the increase of TC genesis frequency in El Niño years during declining phases of solar cycle. The absolute vorticity term makes the largest contribution to the GPI anomalies, and it is obviously much larger than the other terms. The contributions of the other terms are different in El Niño years during declining phases of solar cycle. The second largest contribution is the vertical wind shear term in the 1 year following the solar maximum, and the relative humidity term in the 2–3 years following the solar maximum. In conclusion, the absolute vorticity term plays a leading role in the GPI anomalies, whose contribution is obviously much larger than the other terms. In the meanwhile, it is not perfectly symmetrical with the combined effect of La Niña and ascending phases of solar cycle. During this period, the negative GPI anomalies are accordant to the decrease in TC genesis frequency (Figure 9B). The absolute vorticity term makes the largest contribution to the GPI anomalies and the second largestt contribution is the vertical wind shear term. It is different from that in El Niño during declining phases of solar cycle because the vertical wind shear term is also important. The above conclusions are basically consistent with the analysis in Figure 8.
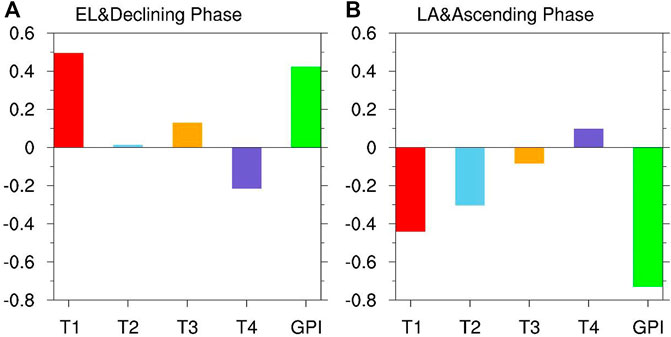
FIGURE 9. Composite regional mean absolute vorticity term (T1), vertical wind shear term (T2), relative humidity term (T3), potential intensity term (T4), and GPI anomalies in the SEWNP in (A) El Niño years during declining phases of solar cycle and (B) La Niña years during ascending phases of solar cycle.
4 Summary and discussion
Previous studies have suggested that ENSO has an important impact on the TC genesis frequency in the SEWNP and NWWNP (Lander, 1994; Chen et al., 1998; Wang and Chan, 2002; Elsner and Liu, 2003; Chu, 2004; Camargo et al., 2007; Kim et al., 2011; Zhan et al., 2011; Song et al., 2022). However, few studies have focused on the influence of solar activity on TC genesis frequency in the WNP. In this study, the combined effect of solar activity and ENSO on the TC genesis frequency in the SEWNP during JJASON from 1965 to 2019 has been primarily investigated. The results indicate that as for the four subregions in the WNP, TCs generated in the SEWNP have the longest lifetime and greatest strength, with an average lifetime of 202.7 h and an average annual ACE of 2 × 105 m2 s-2, far larger than that in the other subregions in the WNP. The overwhelming majority of TCs generated in the SEWNP reach typhoon intensity and super typhoons accounting for 62% of the total number. Additionally, The TC genesis frequency in the SEWNP has apparent interannual and decadal variations, reflecting both the interannual influencing factors and decadal background factors.
The lead-lag correlation shows that TC genesis frequency in the SEWNP has a significant positive correlation with the SSN index in the leading 1–3 years on the decadal time scale, and it is the largest when the SSN index leads 2–3 years. However, no significant correlations are found between the TC genesis frequency in the NWWNP and the SSN index in the leading 1–3 years. Further analysis indicates that the effect of ENSO on TC genesis frequency in the SEWNP is modulated by the decadal background of the solar activity. In El Niño (La Niña) years during declining (ascending) phases of solar cycle, there are significantly strong positive (negative) TC genesis frequency anomalies in the SEWNP, which tends to occur in extreme value. Resulting from the opposite effect of El Niño (La Niña) and ascending (declining) phases of solar cycle, TC genesis frequency anomalies in the SEWNP have no significant feature.
For El Niño (La Niña) modulated by declining (ascending) phases of solar cycle, the overlapping effect leads to apparently warmer (colder) SST anomalies in the central equatorial Pacific and colder (warmer) SST anomalies in the western Pacific, along with the stronger SST anomalies gradient between the central (western) equatorial Pacific and western (central) equatorial Pacific. It enhances low-level westerly (easterly) wind anomalies and upper-level easterly (westerly) wind anomalies, which is favorable for the further decrease (increase) of vertical wind shear between 200 hPa and 850 hPa in the eastern (most) parts of SEWNP. Moreover, there exists a stronger and more westerly low-level convergence (divergence) center in the Pacific, resulting in stronger low-level convergent (divergent) flow and high-level divergent (convergent) flow anomalies in the SEWNP. Correspondingly, the ascending movement and convection anomalies are strengthened (suppressed). As a result, more (fewer) TCs generate in the SEWNP.
The TC genesis factors including vertical wind shear, low-level vorticity, and middle-level relative humidity are examined. In El Niño years during declining phases of solar cycle, the stronger cyclonic circulation anomalies bring about the stronger positive low-level vorticity anomalies in the SEWNP, which is beneficial to more TCs. The stronger positive vertical wind shear anomalies appear in the western SEWNP, while the stronger negative anomalies are in the eastern SEWNP. El Niño events are associated with stronger positive middle-level relative humidity anomalies in most parts of the SEWNP. In La Niña years during ascending phases of solar cycle, the negative vorticity anomalies, positive vertical wind shear anomalies, and negative relative humidity anomalies are stronger in most parts of the SEWNP, resulting in the lower TC genesis frequency in the SEWNP.
The relative contributions of environmental factors to TC genesis in the SEWNP are further quantitatively diagnosed by the GPI. The major characteristic in the combined effect of El Niño and declining phases of solar cycle is different from that in the combined effect of La Niña and ascending phases of solar cycle. During El Niño years in declining phases of solar cycle, the absolute vorticity plays a leading role in the GPI anomalies, whose contribution is obviously much greater than other terms. In La Niña during ascending phases of solar cycle, the absolute vorticity term has the greatest contribution to GPI anomalies, but the vertical wind shear is also important, which is secondary.
In this study, the combined effect of solar activity and ENSO on the TC genesis frequency in the SEWNP is primarily investigated. It helps us to make better forecasts for the TC genesis frequency in the SEWNP. However, the influence of solar activity on the TC genesis frequency in the other subregions of WNP is unknown. Especially, the feature of TC genesis in the South China Sea is different from that in the WNP, how is the combined effect of solar activity and ENSO? The questions deserve to be carried out in the following work.
Data availability statement
The TC best-track data from the CMA can be found from http://tcdata.typhoon.org.cn/dlrdqx_sm.html. The NCEP/NCAR reanalysis data and ERSST.v5 sea surface temperature data are available at https://www.psl.noaa.gov/data/gridded/. The SSN index can be accessed via http://www.sidc.be/silso/datafiles. The Niño3.4 index can be downloaded from https://www.cpc.ncep.noaa.gov/data/indices/ersst5.nino.mth.81-10.ascii.
Author contributions
ShL and ZL contributed to conception and design of the study. SiL calculated the GPI index. ShL wrote the first draft of the manuscript. All authors contributed to the revision of the manuscript.
Funding
This work was supported by the National Key Basic Research and Development Program of China (2012CB957800) and the National Natural Science Foundation of China (U1902209).
Conflict of interest
The authors declare that the research was conducted in the absence of any commercial or financial relationships that could be construed as a potential conflict of interest.
Publisher’s note
All claims expressed in this article are solely those of the authors and do not necessarily represent those of their affiliated organizations, or those of the publisher, the editors and the reviewers. Any product that may be evaluated in this article, or claim that may be made by its manufacturer, is not guaranteed or endorsed by the publisher.
References
Bister, M., and Emanuel, K. A. (2002). Low frequency variability of tropical cyclone potential intensity 1. Interannual to interdecadal variability. J. Geophys. Res. Atmos. 107, D24. doi:10.1029/2001JD000776
Camargo, S. J., Emanuel, K. A., and Sobel, A. H. (2007). Use of a Genesis potential index to diagnose ENSO effects on tropical cyclone Genesis. J. Clim. 20, 4819–4834. doi:10.1175/JCLI4282.1
Camargo, S. J., and Sobel, A. H. (2005). Western North Pacific tropical cyclone intensity and ENSO. J. Clim. 18, 2996–3006. doi:10.1175/JCLI3457.1
Cao, X., Wu, R., Xu, J., Sun, Y., Bi, M., Dai, Y., et al. (2022). Coherent variations of tropical cyclogenesis over the North Pacific and North Atlantic. Clim. Dyn. doi:10.1007/s00382-022-06381-3
Chan, J. C. L. (1985). Tropical cyclone activity in the northwest pacific in relation to the El niño/southern oscillation phenomenon. Mon. Weather Rev. 113, 599–606. doi:10.1175/1520-0493(1985)113<0599:TCAITN>2.0.CO;2
Chan, J. C. L. (2000). Tropical cyclone activity over the Western North Pacific associated with El nino and La nina events. J. Clim. 13, 2960–2972. doi:10.1175/1520-0442(2000)013<2960:TCAOTW>2.0.CO;2
Chan, J. C. L. (2005). Interannual and interdecadal variations of tropical cyclone activity over the Western North Pacific. Meteorol. Atmos. Phys. 89, 143–152. doi:10.1007/s00703-005-0126-y
Chen, T. C., Weng, S. P., Yamazaki, N., and Kiehne, S. (1998). Interannual variation in the tropical cyclone formation over the Western North Pacific. Mon. Weather Rev. 126, 1080–1090. doi:10.1175/1520-0493(1998)126<1080:IVITTC>2.0.CO;2
Chen, X., Zhong, Z., and Lu, W. (2017). Association of the poleward shift of East Asian subtropical upper-level jet with frequent tropical cyclone activities over the Western North Pacific in summer. J. Clim. 30, 5597–5603. doi:10.1175/JCLI-D-16-0334.1
Chu, P. S. (2004). “ENSO and tropical cyclone activity,” in Hurricanes and typhoons, past, Present and future. Editors R. J. Murnane, and K. B. Liu (Columbia University Press), 297–332.
Elsner, J. B., and Liu, K. B. (2003). Examining the ENSO-typhoon hypothesis. Clim. Res. 25, 43–54. doi:10.3354/cr025043
Emanuel, K. A., and Nolan, D. S. (2004). “Tropical cyclone activity and the global climate system,” in Preprints, 26th conf. On hurricanes and tropical meteorology (Miami, FL: Amer. Meteor. Soc.), 10A.2.
Enfield, D. B., and Cid, L. S. (1991). Low-frequency changes in El nino-southern oscillation. J. Clim. 4, 1137–1146. doi:10.1175/1520-0442(1991)004<1137:LFCIEN>2.0.CO;2
Gao, S., Chen, Z., and Zhang, W. (2018). Impacts of tropical North Atlantic SST on western North Pacific landfalling tropical cyclones. J. Clim. 31, 853–862. doi:10.1175/JCLI-D-17-0325.1
Gray, L. J., Beer, J., Geller, M., Haigh, J. D., Lockwood, M., Matthes, K., et al. (2010). Solar influences on climate. Rev. Geophys. 48. doi:10.1029/2009RG000282
Herschel, W. (1801). Observations tending to investigate the nature of the sun, in order to find the causes or symptoms of its variable emission of light and heat: With remarks on the use that may possibly be drawn from solar observations. Phil. Trans. Roy. Soc. Lond. 91, 265–318. doi:10.1098/rstl.1801.0015
Huang, B., Thorne, P. W., Banzon, V. F., Boyer, T., Chepurin, G., Lawrimore, J. H., et al. (2017). Extended reconstructed Sea surface temperature, Version 5 (ERSSTv5): Upgrades, validations, and intercomparisons. J. Clim. 30, 8179–8205. doi:10.1175/JCLI-D-16-0836.1
Huang, R., and Chen, G. (2007). Research on interannual variations of tracks of tropical cyclones over Northwest Pacific and their physical mechanism (in Chinese). Acta Meteor. Sin. 65, 683–694.
Huo, W., and Xiao, Z. (2016). The impact of solar activity on the 2015/16 El Niño event. Atmos. Ocean. Sci. Lett. 9, 428–435. doi:10.1080/16742834.2016.1231567
Huo, W., and Xiao, Z. (2017). Modulations of solar activity on El Niño Modoki and possible mechanisms. J. Atmos. Solar-Terrestrial Phys. 160, 34–47. doi:10.1016/j.jastp.2017.05.008
Huo, W., Xiao, Z., Wang, X., and Zhao, L. (2021). Lagged responses of the tropical pacific to the 11-yr solar cycle forcing and possible mechanisms. J. Meteorol. Res. 35, 444–459. doi:10.1007/s13351-021-0137-8
Huth, R., Pokorná, L., Bochníček, J., and Hejda, P. (2006). Solar cycle effects on modes of low-frequency circulation variability. J. Geophys. Res. Atmos. 111, D22107. doi:10.1029/2005JD006813
Kalnay, E., Kanamitsu, M., Kistler, R., Collins, W., Deaven, D., Gandin, L., et al. (1996). The NCEP/NCAR 40-year reanalysis project. Bull. Amer. Meteor. Soc. 77, 437–472. doi:10.1175/1520-0477(1996)077,0437:TNYRP.2.0.CO;2
Kim, H. M., Webster, P. J., and Curry, J. A. (2011). Modulation of North Pacific tropical cyclone activity by three phases of ENSO. J. Clim. 24, 1839–1849. doi:10.1175/2010JCLI3939.1
Kim, J. S., Kim, S. T., Wang, L., Wang, X., and Il Moon, Y. (2016). Tropical cyclone activity in the northwestern pacific associated with decaying central pacific El niños. Stoch. Environ. Res. Risk Assess. 30, 1335–1345. doi:10.1007/s00477-016-1256-0
Kodera, K., Thiéblemont, R., Yukimoto, S., and Matthes, K. (2016). How can we understand the global distribution of the solar cycle signal on the Earth’s surface? Atmos. Chem. Phys. 16, 12925–12944. doi:10.5194/acp-16-12925-2016
Lander, M. A. (1994). An exploratory analysis of the relationship between tropical storm formation in the Western North Pacific and ENSO. Mon. Weather Rev. 122, 636–651. doi:10.1175/1520-0493(1994)122<0636:AEAOTR>2.0.CO;2
Li, C. (1987). A study on the influence of El Nino upon typhoon action over Western Pacific. Acta Meteor. Sin. 45, 229–236. (in Chinese).
Li, D., and Xiao, Z. (2018). Can solar cycle modulate the ENSO effect on the Pacific/North American pattern? J. Atmos. Solar-Terrestrial Phys. 167, 30–38. doi:10.1016/j.jastp.2017.10.007
Li, Z., Yu, W., Li, T., Murty, V. S. N., and Tangang, F. (2013). Bimodal character of cyclone climatology in the bay of bengal modulated by monsoon seasonal cycle. J. Clim. 26, 1033–1046. doi:10.1175/JCLI-D-11-00627.1
Li, H., Wang, C., He, S., Wang, H., Tu, C., Xu, J., et al. (2019). Plausible modulation of solar wind energy flux input on global tropical cyclone activity. J. Atmos. Solar-Terrestrial Phys. 192, 104775. doi:10.1016/j.jastp.2018.01.018
Lin, Y. F., Yu, J. Y., Wu, C. R., and Zheng, F. (2021). The footprint of the 11-year solar cycle in northeastern pacific SSTs and its influence on the central pacific El Niño. Geophys. Res. Lett. 48, e2020GL091369. doi:10.1029/2020GL091369
Ling, S., and Lu, R. (2022). Tropical cyclones over the western North Pacific strengthen the East asia—pacific pattern during summer. Adv. Atmos. Sci. 39, 249–259. doi:10.1007/s00376-021-1171-2
Liu, Z., Yoshimura, K., Buenning, N. H., and He, X. (2014). Solar cycle modulation of the Pacific-North American teleconnection influence on North American winter climate. Environ. Res. Lett. 9, 024004. doi:10.1088/1748-9326/9/2/024004
Liu, C., Zhang, W., Stuecker, M. F., and Jin, F. F. (2019). Pacific meridional mode-western North Pacific tropical cyclone linkage explained by tropical pacific quasi-decadal variability. Geophys. Res. Lett. 46, 13346–13354. doi:10.1029/2019GL085340
Liu, K. S., and Chan, J. C. L. (2018). Changing relationship between La Niña and tropical cyclone landfalling activity in South China (La Niña and TC landfalling activity in South China). Int. J. Climatol. 38, 1270–1284. doi:10.1002/joc.5242
Lu, X., Yu, H., Ying, M., Zhao, B., Zhang, S., Lin, L., et al. (2021). Western North pacific tropical cyclone database created by the China meteorological administration. Adv. Atmos. Sci. 38, 690–699. doi:10.1007/s00376-020-0211-7
Ma, H., Wang, R., Lai, A., Li, X., Wang, F., Zhou, Z., et al. (2021). Solar activity modulates the El Niño-Southern Oscillation-induced precipitation anomalies over southern China in early spring. Int. J. Climatol. 41, 6589–6601. doi:10.1002/joc.7214
Meehl, G. A., Arblaster, J. M., Branstator, G., and van Loon, H. (2008). A coupled air-sea response mechanism to solar forcing in the Pacific region. J. Clim. 21, 2883–2897. doi:10.1175/2007JCLI1776.1
Pan, Y. (1982). The effect of the thermal state of equatorial eastern Pacific on the frequency of typhoons over Western Pacific (in Chinese). Acta Meteor. Sin. 40, 24–34.
Ren, S., Liu, Y., and Wu, G. (2007). Interactions between typhoon and subtropical anticyclone over Western Pacific revealed by numerical experiments (in Chinese). Acta Meteor. Sin. 65, 329–340. doi:10.11676/qxxb2007.032
Shan, K., and Yu, X. (2021). Variability of tropical cyclone landfalls in China. J. Clim. 34, 9235–9247. doi:10.1175/JCLI-D-21-0031.1
Song, J., Klotzbach, P. J., Wang, Y. F., and Duan, Y. (2022). Influence of different La Niña decay types on tropical cyclone Genesis over the Western North Pacific. Atmos. Res. 280, 106419. doi:10.1016/j.atmosres.2022.106419
Wang, B., and Chan, J. C. L. (2002). How strong ENSO events affect tropical storm activity over the Western North Pacific. J. Clim. 15, 1643–1658. doi:10.1175/1520-0442(2002)015<1643:HSEEAT>2.0.CO;2
Wang, X., Zhou, W., Li, C., and Wang, D. (2014). Comparison of the impact of two types of El Niño on tropical cyclone Genesis over the South China Sea. Int. J. Climatol. 34, 2651–2660. doi:10.1002/joc.3865
Wang, Q., Li, J., Jin, F. F., Chan, J. C. L., Wang, C., Ding, R., et al. (2019). Tropical cyclones act to intensify El Niño. Nat. Commun. 10, 3793. doi:10.1038/s41467-019-11720-w
Wang, R., Ma, H., Xiao, Z., Li, X., Gao, C., Gao, Y., et al. (2021). The combined effects of ENSO and solar activity on mid-winter precipitation anomalies over southern China. Front. Earth Sci. 9, 1–15. doi:10.3389/feart.2021.771234
Woodruff, J. D., Irish, J. L., and Camargo, S. J. (2013). Coastal flooding by tropical cyclones and sea-level rise. Nature 504, 44–52. doi:10.1038/nature12855
Xue, X., Chen, W., and Zhou, Q. (2020). Solar cycle modulation of the connection between boreal winter ENSO and following summer South Asia high. J. Atmos. Solar-Terrestrial Phys. 211, 105466. doi:10.1016/j.jastp.2020.105466
Yan, H., Zhong, M., and Zhu, Y. (2004). Determination of the degree of freedom of digital filtered time series with an application to the correlation analysis between the length of day and the Southern Oscillation index. Chin. Astron. Astrophys. 28, 120–126. doi:10.1016/S0275-1062(04)90014-8
Ying, M., Zhang, W., Yu, H., Lu, X., Feng, J., Fan, Y. X., et al. (2014). An overview of the China meteorological administration tropical cyclone database. J. Atmos. Ocean. Technol. 31, 287–301. doi:10.1175/JTECH-D-12-00119.1
Yu, M., Zhu, C., and Jiang, N. (2019). Subseasonal mode of cold and wet climate in South China during the cold season: A climatological view. Atmos. Ocean. Sci. Lett. 12, 73–79. doi:10.1080/16742834.2019.1568164
Zhan, R., Wang, Y., and Lei, X. (2011). Contributions of ENSO and East Indian ocean SSTA to the interannual variability of northwest pacific tropical cyclone frequency. J. Clim. 24, 509–521. doi:10.1175/2010JCLI3808.1
Zhang, W., Vecchi, G. A., Villarini, G., Murakami, H., Rosati, A., Yang, X., et al. (2017). Modulation of Western North Pacific tropical cyclone activity by the atlantic meridional mode. Clim. Dyn. 48, 631–647. doi:10.1007/s00382-016-3099-2
Zhao, H., and Wang, C. (2019). On the relationship between ENSO and tropical cyclones in the Western North Pacific during the boreal summer. Clim. Dyn. 52, 275–288. doi:10.1007/s00382-018-4136-0
Zhong, Z. (2006). A possible cause of a regional climate model’s failure in simulating the east Asian summer monsoon. Geophys. Res. Lett. 33, L24707. doi:10.1029/2006GL027654
Zhong, Z., and Hu, Y. (2007). Impacts of tropical cyclones on the regional climate: An East Asian summer monsoon case. Atmos. Sci. Lett. 8, 93–99. doi:10.1002/asl.158
Keywords: tropical cyclone genesis, solar cycle phases, El Niño-southern Oscillation, combined effect, environmental factors
Citation: Li S, Li Z and Ling S (2023) Combined effect of the solar activity and ENSO on the tropical cyclone genesis frequency in the southeastern part of the western North Pacific. Front. Earth Sci. 11:1139699. doi: 10.3389/feart.2023.1139699
Received: 07 January 2023; Accepted: 24 February 2023;
Published: 14 March 2023.
Edited by:
Hiroko Miyahara, Musashino Art University, JapanReviewed by:
Limin Zhou, East China Normal University, ChinaXin Wang, South China Sea Institute of Oceanology (CAS), China
Copyright © 2023 Li, Li and Ling. This is an open-access article distributed under the terms of the Creative Commons Attribution License (CC BY). The use, distribution or reproduction in other forums is permitted, provided the original author(s) and the copyright owner(s) are credited and that the original publication in this journal is cited, in accordance with accepted academic practice. No use, distribution or reproduction is permitted which does not comply with these terms.
*Correspondence: Zhangqun Li, lizhangqun@lasg.iap.ac.cn