- 1College of Safety Science and Engineering, Henan Polytechnic University, Jiaozuo, China
- 2State Key Laboratory Cultivation Base for Gas Geology and Gas Control (Henan Polytechnic University), Jiaozuo, China
- 3State Collaborative Innovation Center of Coal Work Safety and Clean-efficiency Utilization, Jiaozuo, China
- 4Chongqing Vocational Institute of Safety and Technology, Chongqing, China
- 5School of Mechanical and Power Engineering, Henan Polytechnic University, Jiaozuo, China
Introduction: The initial velocity of gas diffusion (ΔP) of coal is an important index to characterize the outburst risk of coal, and temperature is an important factor affecting the determination of initial velocity of gas diffusion. However, how to control the temperature during ΔP determination remains a significant challenge. Methods: In this paper, an experimental system for ΔP testing under temperature-controlled conditions was constructed, and the effect of temperature on the ΔP determination results was further explored by accurately controlling the temperature of the testing system. Results: The results show that the temperature change will affect the determination result of ΔP in the range of 13°C–40°C, and the determination results do not show obvious regularity. Discussion: The main reasons for the analysis are as follows: during the determination of ΔP, although the higher the temperature is, the faster the speed of gas diffusion and the greater the amount of gas diffusion and the pressure produced in the first 60 s, but the smaller the amount of gas adsorption before release. There is a situation of mutual offset between the two, resulting in the fluctuation of ΔP determination results. It is concluded that the temperature change will affect the determination of the initial velocity of gas diffusion, and when ΔP is measured, the temperature of the experimental system should be consistent with the actual temperature of the sampled coal body as far as possible, so as to increase the reliability of the measured results for the prediction of coal and gas outburst. This paper provides an experimental basis for clarifying the industry standard of temperature related to ΔP.
1 Introduction
The initial velocity of gas diffusion of coal is an index that indicates how quickly the coal containing gas changes from adsorption state to free state under the condition of complete exposure. The greater the initial velocity of gas diffusion, the stronger the initial gas release capacity of coal body, and the greater the outburst risk of coal seam (Liu and Wang, 2013; Liang et al., 2018; Sun, 2018). This index has been used as one of the indexes to judge the danger degree of coal and gas outburst in most countries in the world, and has been widely used (AQ1080-2009, 2009; Wang and Shi, 1991; Lu, 1992).
Many scholars have studied the influencing factors of this index and achieved fruitful results (Chen et al., 2012; Meng and Li, 2016; Du et al., 2020; Li et al., 2022; Lu et al., 2022). The effects of adsorption pressure, firmness coefficient, volatile matter, adsorption equilibrium time, and particle size on the initial velocity of gas diffusion have also been studied (Jianning, 2010; Xu and Jiang, 2011; Tan et al., 2012; Xu and Jiang, 2012; Yanwei and Mingju, 2015; Yang et al., 2022). Temperature is one of the important factors. Li et al. (Li and Jiang, 2009) pointed out that there is a quadratic relationship between the initial velocity of gas diffusion and the temperature difference through experiments and curve fitting in the temperature range of 20°C–24°C, and the higher the temperature is, the smaller the initial velocity of gas diffusion is Guo, (2018) draws the conclusion that the initial velocity of gas diffusion decreases with the increase of temperature in the temperature range of 5°C–30°C through experimental analysis, and points out that the extent of the decrease is related to the initial velocity of gas diffusion of coal sample itself Ma, (2017) thinks that there is a quadratic function relationship between temperature and initial velocity of gas diffusion in the temperature range of 15°C–35°C, and the initial velocity of gas diffusion increases with the increase of temperature before the extreme point, and decreases with the increase of temperature after the extreme point.Huang et al. (2019) pointed out that the error of the same coal sample is large when the experimental temperature is 10°C–30°C, which is caused by the influence of room temperature, and the follow-up study should fully consider the effect of room temperature on the initial gas diffusion velocity of coal.Guo et al. (2020) pointed out that the initial velocity of gas diffusion does not decrease in a straight line with the increase of temperature, and the change of temperature may affect the internal pressure of gas or the structure of coal matrix.
Although many people at home and abroad have made great contributions to the study of the influence of temperature on the determination results of the initial velocity of gas diffusion, in general, the precise control of temperature during ΔP determination has rarely been reported. With the continuous increase of coal mining depth, the phenomenon of coal seam temperature increase occurs in many mines (Zheng et al., 2011), and the influence of temperature factors on coal and gas outburst is increasing. As the main prediction index of coal and gas outburst, the initial velocity of gas diffusion has no clear requirement for temperature in the industry standard for its determination, so there is an urgent need to study the influence of temperature factors on its determination and define the standard.
This paper constructs the ΔP determination system under the condition of temperature based on the instrument for measuring the initial velocity of gas diffusion developed by Henan Polytechnic University, and realizes the accurate control of the temperature of the test system. The experiment was carried out strictly according to the technical standard of AQ 1080-2009 (AQ1080-2009, 2009), and the effect of temperature on the initial velocity of gas diffusion was further studied. It is concluded that in the temperature range of 13°C–40°C, the temperature change will affect the determination results of ΔP, and the determination results do not show obvious regularity. This paper provides an experimental model and some data support for the further study on the temperature influencing factors of ΔP determination.
2 Experimental
2.1 Coal sample collection
Four coal samples were selected for experiment in this paper in order to make the experimental results comparable. Coal sample 1 is a soft coal collected from the excavation head of 16041 transportation roadway in Jiaozuo Jiulishan Coal Mine, which is numbered JR. Coal sample 2 is hard coal collected from 16021 transportation roadway in Jiaozuo Jiulishan Coal Mine, numbered JY. Coal sample 3 is a soft coal collected from 3101 working face of Hebi No. 8 Coal Mine, which is numbered HR. Coal sample 4 is hard coal collected from 3103 working face of Hebi No. 8 Coal Mine, numbered HY. The specific sampling locations is shown in Figure 1.
In order to fully understand the properties of coal samples, four coal samples were analyzed industrially according to the industrial analysis method of coal (GB/T212-2008). The results are as follows, as shown in Table 1.
2.2 Sample preparation
According to the regulations of GB474 and GB477 in China, the collected coal samples were crushed and sieved with particle size of 0.2–0.25 mm. The production process of coal sample is shown in Figure 2. Two specimens are taken from each coal sample, each weighing 3.5 g. Meanwhile, keep the spare coal sample for repeated experimental verification.
2.3 Experimental installation
The initial velocity of gas diffusion determination system under temperature-controlled conditions constructed in this paper is mainly composed of five parts: the initial velocity of gas diffusion determination instrument, glass water tank, constant temperature water bath, gas cylinder and vacuum pump. The instrument for measuring the initial velocity of gas diffusion is developed by Henan Polytechnic University. The glass water tank is self-built. Constant temperature water bath is produced by Chongqing Huida Experimental instrument Co., Ltd. Other auxiliary experimental equipment include WY-98B adsorption constant tester, electronic scale, distiller, sealed hammer crusher, mercury thermometer, and so on.
In order to clearly show how to accurately control the temperature of the system, the system model diagram is drawn, as shown in Figure 3. The realization process of temperature control: Appropriate amount of water is injected into the glass tank, and then the well-sealed initial velocity of gas diffusion determination instrument is placed in it to maintain immersion. The constant temperature water bath is connected with the glass water tank, and the temperature of the water in the tank is controlled by the constant temperature water bath, so as to realize the accurate control of the temperature of the ΔP determination system. Two thermometers are placed vertically on both sides of the tank to compare and monitor whether the water temperature is stable at the set temperature.
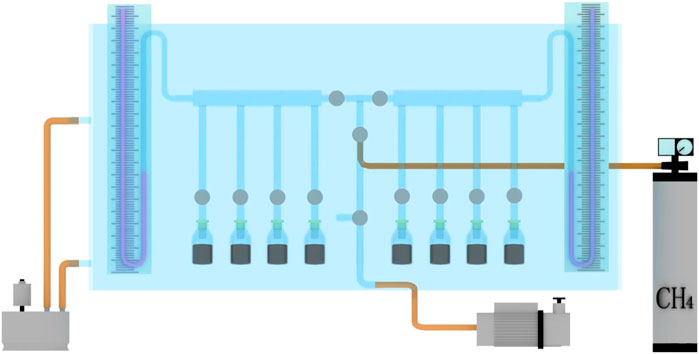
FIGURE 3. System model for measuring the initial velocity of gas diffusion under temperature control.
2.4 Experimental process and results
The experiment was carried out strictly according to the technical standard of AQ 1080-2009 (Liang et al., 2018). In order to accurately describe the process of the experiment, this paper makes a schematic diagram of the determination system, as shown in Figure 4. The specific experimental process is as follows:
2.4.1 Sample preparation
The coal sample with particle size of 0.2–0.25 mm was prepared according to the 2.2 method. In this experiment, there are 4 coal samples. In order to make the experimental results reliable, two samples are taken from each kind of coal sample, one of which is used as a parallel comparison of coal samples. A total of 8 samples, each sample weighs 3.5 g.
2.4.2 Sample loading
Eight samples were packed in 8 sample bottles by funnel. Sample bottle 1 and 5 are filled with JR, sample bottle 2 and 6 are filled with JY, sample bottle 3 and 7 are filled with HR, and sample bottle 4 and 8 are filled with HY. The latter is the parallel comparison sample of the former.
2.4.3 Temperature selection
The temperature range selected in the experiment is 13°C–40°C. A total of 6 temperature points were selected, which are 13°C, 18.5°C, 23°C, 28°C, 35°C and 40°C. There are three factors to consider: the first is the temperature environment of the coal mine around 20°C in the actual production; the second is the development trend of temperature rising with the increase of mine mining depth; the third is to find the linear law of ΔP changing with temperature.
2.4.4 Test
Step 1:. Check the test system, set the first temperature point to be investigated at 13°C, and start heating. Enter the second step after it is constant at 13°C. The temperature of water in the tank was observed by the two thermometers.
Step 2:. Open all coal sample channels, open valves 9 and 10, rotate three-way valves 11 and 12 to the position where they are connected up and down, and start the vacuum pump to degas the coal sample for 90 min.
Step 3:. After 90 min of degassing, turn off the vacuum pump, and then rotate valve 11 to connect methane bottles and all coal sample bottles. Gas is charged to make coal samples absorb gas for 90 min, and the charging pressure is maintained at 0.1 MPa.
Step 4:. Close the methane bottle and all coal sample bottles in order to isolate the coal sample bottle from the methane bottle.
Step 5:. Rotate the three-way valves 11 and 12 to the position of the upper and lower connection, and start the vacuum pump to degas the dead space of the instrument, so that the liquid level of the mercury column at both ends of the U-shaped mercury column meter is level.
Step 6:. Close valve 12, stop the vacuum pump, then close valve 10. Open the coal sample bottle 1, start the stopwatch timing when the coal sample bottle is connected to the dead space of the instrument, close valve 1 at 10s, write down the upper and lower liquid level readings of the mercury column meter on the left, and get the P1 value. Open the valve 1 again at 45 s and close valve 1 again at 60 s, read out the upper and lower liquid level readings of the mercury column meter again, get the P2 value, and then calculate the ΔP value of coal sample 1 at the temperature of 13°C.
Step 7:. Close the coal sample bottle 1 and complete the coal sample 1 test. Open valve 10 and 12, start the vacuum pump to degas the dead space of the instrument, so that the mercury column surfaces at both ends of the U-shaped mercury column meter are level. The same method is used to complete the test of coal sample 2, 3 and 4 ΔP value in turn. The same steps are used to complete the test of ΔP values of four parallel coal samples 5, 6, 7 and 8 on the right.
Step 8:. After all the tests are completed, the difference between the left coal sample and the corresponding right parallel coal sample ΔP is calculated. If the difference between the two is greater than 1, the test result of this group is invalid, and the sample is reloaded for testing. If the difference is less than 1, the maximum value of the two will be taken as the determination result of the coal sample. Finally, the determination results of 4 groups of coal samples under 13 °C temperature condition are obtained.The ΔP values of four coal samples at 18.5°C, 23°C, 28°C, 35°C and 40°C are obtained by re-setting the temperature of thermostatic water bath and repeating the above steps. The results are shown in Table 2.
3 Discussion and results
3.1 Experimental analysis
From Figure 3 we can see that the instrument for determination of initial velocity of gas diffusion is always immersed in a constant temperature tank in this experiment, the water temperature is controlled by a thermostatic water bath. The temperature difference is less than 1 °C up and down, and the temperature is relatively constant. The difference between the experimental process and the previous experiments is that when measuring the ΔP value at a certain temperature point, the constructed constant temperature system can not only ensure that the temperature of the coal sample is the set temperature, but also ensure that the temperature of the whole test system is constant at the set temperature. It is not affected by room temperature. This scheme greatly improves the reliability and accuracy of the temperature condition in the determination of ΔP.
According to the experimental results of 2.4, the variation curves of initial velocity of gas diffusion at different temperatures of JR, JY, HR, and HY are drawn respectively, as shown in Figure 5.
From Figure 5 we can see that in the temperature range of 13°C–40°C, the initial velocity of gas diffusion jumps up and down with the increase of temperature, showing no obvious regularity. From the span, JR is 3, JY is 5, HR is 3, HY is 1.5, compared with the critical value 10, this is enough to affect people’s judgment on the risk of coal and gas outburst. From a macro point of view, the initial gas emission rate of soft coal in Jiaozuo Jiulishan Mine and Hebi No. 8 Coal Mine shows a decreasing trend with the increase of temperature, while their corresponding hard coal shows a rising trend. Preliminary judgment: for hard coal, the effect of temperature rise on gas diffusion rate is greater than that on adsorption, while soft coal is the opposite.
3.2 Discussion
This paper focuses on the influence of temperature on the determination of the initial velocity of gas diffusion. According to the definition of ΔP, ΔP is the difference between the pressure P1 generated by gas diffusion of coal sample in the first 10s and the pressure P2 produced by gas diffusion of coal sample within 45–60 s, provided that the basic measurement conditions such as adsorption equilibrium pressure and particle size remain unchanged. In general, the determination result is reflected in the amount of gas emission in the first 60 s of coal sample, which is related to the gas emission rate and the initial adsorption capacity of coal sample. Therefore, the discussion of the influence of temperature on the initial velocity of gas diffusion becomes the discussion of the influence of temperature on the rate of gas emission and the initial gas adsorption capacity in the first 60 s.
3.2.1 Analysis of the influence of temperature on gas diffusion rate
Liu et al. (2013) concluded that the increase of temperature greatly improves the diffusion ability of gas in coal particles by enhancing the activity of methane molecules and strengthening the expansion of pores, especially small pores. Gao, (2013) proved that in the temperature range of 25°C–45°C, under the same pressure condition, the higher the temperature, the greater the rate of gas desorption, and the maximum rate is in the first 60 s, and tends to be consistent after 3 min. Li (Hong, 2011) believes that in the temperature range of 20°C–60°C, under the same pressure, the higher the temperature, the greater the gas desorption rate, and the maximum gas desorption rate is in the first 60 s. He, (2010) demonstrated that in the temperature range of 10°C–40°C, the higher the temperature, the higher the gas desorption rate and the greater the desorption amount in the same time. Based on the above research, it can be concluded that the higher the temperature, the faster the gas emission rate, and the greater the gas emission amount in the first 60 s, which means that the effect of temperature on the determination results of initial velocity of gas diffusion is positively correlated from the perspective of gas disffusion rate.
3.2.2 Analysis of the influence of temperature on initial adsorption amount of gas
Wang et al. (2016); (Zhang et al., 2009); (Chen et al., 1995); (Zhong et al., 2002); (Wang et al., 2011); (Liu et al., 2012); (Cai et al., 2019); (Wang et al., 2022) and others found that the amount of gas adsorption gradually decreased with the increase of temperature. It is generally accepted that the adsorption amount decreases by 8% for a 1 °C temperature increase. Based on the previous research results, from the perspective of gas adsorption, the higher the temperature, the smaller the initial adsorption capacity of coal sample before ΔP determination. From this point of view, the effect of temperature on the determination of the initial velocity of gas diffusion is negatively correlated.
3.2.3 Analysis of the influence of temperature on gas diffusion pressure
The temperature has an effect on the pressure under the condition of constant diffusion space. The initial velocity of gas diffusion determination instrument used in this paper is of variable volume and pressure type. According to the research results of Lu, (1992), ΔP is not only related to the amount of gas diffusion to be measured, but also related to the volume of the dead space of the instrument and the tube diameter of the manometer. The sum of the dead space of the instrument and the space produced by the change of the pressure gauge is the diffusion space of the experimental coal sample. Here, according to the ideal gas state equation (PV=nRT), assuming that the diffusion space V and the gas diffusion quantity n remain unchanged, it can be seen that the temperature is proportional to the pressure. That is to say, the higher the temperature, the greater the pressure, the greater the corresponding p-value. Therefore, from this point of view, the change of temperature will directly affect the values of P1 and P2, thus affecting the results of the determination of the initial velocity of gas diffusion.
To sum up, in the process of ΔP determination, although the higher the temperature is, the greater the gas diffusion volume and its generating pressure are in the first 60 s, but the smaller the adsorption capacity before release is, and there is a mutual offset between the two cases. Therefore, the measurement results of ΔP under different temperature conditions failed to show the corresponding regularity. The analytical results are consistent with the experimental results.
According to the above discussion, it is suggested that the industry standard for the determination of ΔP related temperature should be clarified. Reason: In actual production, the actual temperature of coal seams in different mining areas and different depths is different, but most of the experimental measurements are carried out at room temperature (20°C), so the results of ΔP may deviate from the real results. In the actual test, not all laboratories have constant temperature conditions either, and the same coal sample may have different test results in winter and summer, and inconsistent test results in day and night. The result will directly affect people’s judgment of coal and gas outburst.
4 Conclusion
1. The test system for the initial velocity of gas diffusion under different temperature conditions constructed in this paper provides an experimental reference for further research.
2. The temperature change will affect the ΔP determination results in the range of 13°C–40°C, and the results do not show obvious regularity. In the process of ΔP determination, the temperature of the experimental system should be kept consistent with the actual temperature of the sampled coal body as far as possible, so as to increase the reliability of the determination results for the prediction of coal and gas outburst.
3. From a macro point of view, hard coal shows an increasing trend of ΔP with the increase of temperature, and soft coal shows a decreasing trend. It is preliminarily speculated that the effect of temperature increase on gas diffusion rate of hard coal is greater than the effect on its adsorption, and the opposite is true for soft coal.
4. This paper also provides an experimental basis for clarifying the industry standard for the determination of ΔP related temperature. Therefore, the industry standard for determining the temperature of ΔP should be defined, in order to increase the comparability and stability of ΔP determination results.
Data availability statement
The original contributions presented in the study are included in the article/Supplementary Material, further inquiries can be directed to the corresponding authors.
Author contributions
XS: Conceptualization, funding acquisition, methodology, investigation, writing—original draft, writing—review and editing. JW: Conceptualization, funding acquisition, methodology, writing—review and editing. ZW: Conceptualization, funding acquisition, methodology, investigation, writing—review and editing. LS: Investigation, writing—original draft. JY: Investigation, Writing—original draft.
Funding
This work was supported by the National Natural Science Foundation of China (Grant No: 51974109), Project funded by China Postdoctoral Science Foundation (2021M700132), Basic Research Funds of Henan Polytechnic University (NSFRF220205).
Conflict of interest
The authors declare that the research was conducted in the absence of any commercial or financial relationships that could be construed as a potential conflict of interest.
Publisher’s note
All claims expressed in this article are solely those of the authors and do not necessarily represent those of their affiliated organizations, or those of the publisher, the editors and the reviewers. Any product that may be evaluated in this article, or claim that may be made by its manufacturer, is not guaranteed or endorsed by the publisher.
References
AQ1080-2009 (2009). “State Adminstration of work safety,” in Determination method for index (ΔP) of initial velocity of diffusion of coal gas, 12.
Cai, T., Feng, Z., and Jiang, Y. (2019). Thermodynamic characteristics of methane adsorption of coal with different initial gas pressures at different temperatures[J]. Adv. Mater. Sci. Eng. 2019, 4751209.
Chen, C., Xian, X., and Zhang, D. (1995). The dependence of temperature to the adsorption of methane on anthracite coal and its char[J]. Coal Convers. 18 (3), 88–92.
Chen, X., Cheng, Y., and Wang, L. (2012). Moisture content affected to initial gas emission velocity of different ranking coal[J]. Coal Sci. Technol. 40 (12), 62–65.
Du, F., Wang, K., Zhang, X., Xin, C., Shu, L., and Wang, G. (2020). Experimental study of coal–gas outburst: Insights from coal–rock structure, gas pressure and adsorptivity. Nat. Resour. Res. 29, 2481–2493. doi:10.1007/s11053-020-09621-7
Gao, B. (2013). Study on gas desorption laws of coal particle at high temperature[D]. Beijing: China University of Geosciences.
Guo, P., Gao, J., and Zhang, C. (2020). Research progress and analysis on influence factors of initial velocity of gas emission[J]. Saf. Coal Mines 51 (7), 176–182.
Guo, Z. (2018). Influences of temperature and dissipate space volume on determination of gas initial emission velocity by isobaric variable pressure method[J]. China Energy Environ. Prot. 40 (12), 42–44.
He, Z. (2010). Research on the impact of temperature on gas desorption law of technically coal[D]. Jiaozuo: Henan Polytechnic University.
Hong, Li (2011). Experimental research on influence of ambient temperature on gas desorption laws of particle coal[D]. Jiaozuo: Henan Polytechnic University.
Huang, D., Zhu, H., and Chen, G. (2019). Effect of temperature on initial velocity of coal gas release[J]. Mod. Min. 11 (11), 236–262.
Jianning, K. (2010). Influence study on initial speed of methane diffusion by adsorption pressure[J]. Saf. Coal Mines 41 (4), 4–5.
Li, X., and Jiang, C. (2009). Study on effect of temperature on initial speed of methane emission[J]. Saf. Coal Mines 40 (1), 1–3.
Li, X., Wang, C., Chen, Y., and Li, H. (2022). Influence of temperature on gas desorption characterization in the whole process from coals and its application analysis on outburst risk prediction. Fuel 321, 124021. doi:10.1016/j.fuel.2022.124021
Liang, Y., Wang, F., Luo, Y., and Hu, Q. (2018). Desorption characterization of methane and carbon dioxide in coal and its influence on outburst prediction. Adsorpt. Sci. Technol. 36 (7-8), 1484–1495. doi:10.1177/0263617418781903
Liu, G., Zhang, Z., and Song, Z. (2012). Adsorption experiments on CH4 under the conditions of high temperature and pressure and equilibrium water[J]. J. China Coal Soc. 37 (5), 794–797.
Liu, J., and Wang, Z. (2013). Relation between metamorphic grade and initial velocity of gas diffusion. J. Liaoning Tech. Univ. Sci. 32 (6), 745–748.
Liu, Y., Jianping, W., and He, Z. (2013). Influence rules and mechanisms of temperature on dynamic process of gas diffusion from coal particles[J]. J. China Coal Soc. 38 (S1), 100–105.
Lu, Z., Wang, L., Lv, M., Lei, Y., Hao, W., and Liu, Q. (2022). Experimental study on coal and gas outburst risk in strong outburst coal under different moisture content[J]. Front. Earth Sci. 10, 782372. doi:10.3389/feart.2022.782372
Lu, Z. (1992). Problems and improvement in determination of initial velocity of gas diffusion △P [J]. Industrial Saf. Dust Prot. (9), 39–41.
Ma, C. (2017). Reasearch on calibration technique and device of initial velocity of coal gas measuring device[D]. Xuzhou, Jiangsu: China University of Mining and Technology.
Meng, Y., and Li, Z. (2016). Experimental study on diffusion property of methane gas in coal and its influencing factors. Fuel 185, 219–228. doi:10.1016/j.fuel.2016.07.119
Sun, W. (2018). Research on initial speed of methane diffusion on initial gas emission in driving face[J]. Coal Technol. 37 (7), 163–164.
Tan, R., Wang, Z., and Yang, H. (2012). Adsorption equilibrium time affected to initial velocity of gas emission[J]. Coal Sci. Technol. 40 (5), 46–48.
Wang, J., Zhang, L., and Zhao, D. (2011). Effect of temperature and moisture on raw coal adsorption characteristics[J]. J. China Coal Soc. 36 (12), 2086–2091.
Wang, K., Ren, H., Wang, Z., and Wei, J. (2022). Temperature-pressure coupling effect on gas desorption characteristics in coal during low-variable temperature process. J. Petroleum Sci. Eng. 211, 110104. doi:10.1016/j.petrol.2022.110104
Wang, Y., and Shi, B. (1991). Quantitative analysis of influence on △P-meter measurements[J]. J. Huainan Min. Inst. 11 (3), 21–30.
Wang, Z., Xie, C., and Qi, C. (2016). Inhibitory effect of low temperature on methane diffusion in coal[J. ]. Saf. Coal Mines 47 (6), 16–19.
Xu, L., and Jiang, C. (2012). Influence factors analysis on fastness coefficient and initial speed of methane emission determination[J]. Coal Technol. 31 (1), 93–94.
Xu, L, and Jiang, C (2011). Study on relation between coal's volatility and initial speed of methane emission[J]. Saf. Coal Mines 42 (7), 21–22.
Yang, X., Wang, G., Du, F., Jin, L., and Gong, H. (2022). N2 injection to enhance coal seam gas drainage (N2-ECGD): Insights from underground field trial investigation[J]. Energy 239, 122247. doi:10.1016/j.energy.2021.122247
Yanwei, L., and Mingju, L. (2015). Effect of particle size on difference of gas desorption and diffusion between soft coal and hard coal[J]. J. China Coal Soc. 40 (3), 579–587.
Zhang, T., Xu, H., and Li, S. (2009). The effect of temperature on the adsorbing capability of coal[J]. J. China Coal Soc. 34 (6), 802–805.
Zheng, G., Luan, Y., and Bai, L. (2011). Discussion on the zonations of shallow geothermal energy resources in China[J]. City Geol. 6 (1), 12–16.
Keywords: gas diffusion, initial velocity, temperature, determination, coal
Citation: Shang X, Wei J, Wen Z, Shang L and Yuan J (2023) Determination of initial velocity of gas diffusion under temperature control. Front. Earth Sci. 11:1138828. doi: 10.3389/feart.2023.1138828
Received: 06 January 2023; Accepted: 02 February 2023;
Published: 13 February 2023.
Edited by:
Xuelong Li, Shandong University of Science and Technology, ChinaReviewed by:
Feng Du, China University of Mining and Technology, Beijing, ChinaYabin Gao, Taiyuan University of Technology, China
Copyright © 2023 Shang, Wei, Wen, Shang and Yuan. This is an open-access article distributed under the terms of the Creative Commons Attribution License (CC BY). The use, distribution or reproduction in other forums is permitted, provided the original author(s) and the copyright owner(s) are credited and that the original publication in this journal is cited, in accordance with accepted academic practice. No use, distribution or reproduction is permitted which does not comply with these terms.
*Correspondence: Xianguang Shang, shangxianguang@hpu.edu.cn; Zhihui Wen, wenzhihui@hpu.edu.cn