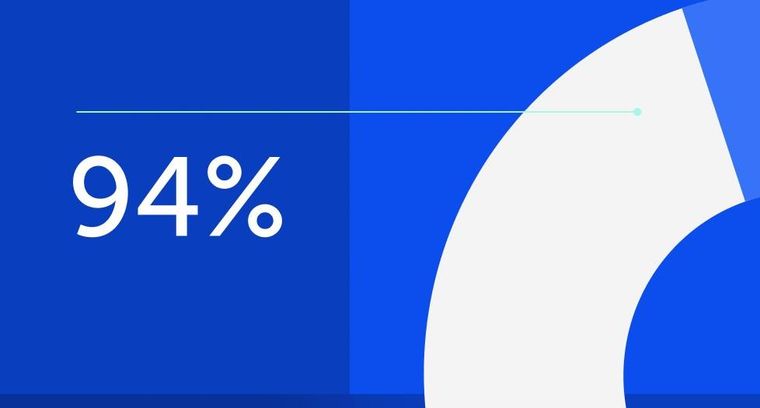
94% of researchers rate our articles as excellent or good
Learn more about the work of our research integrity team to safeguard the quality of each article we publish.
Find out more
ORIGINAL RESEARCH article
Front. Earth Sci., 05 May 2023
Sec. Quaternary Science, Geomorphology and Paleoenvironment
Volume 11 - 2023 | https://doi.org/10.3389/feart.2023.1133281
This article is part of the Research TopicBehaviors and Adaptations of Prehistoric Hunter-Gatherers in the (Sub)Tropical Rainforest AreaāArchaeology, Chronology and PaleoenvironmentView all 5 articles
The Wet Zone region of Sri Lanka has provided some of the earliest direct evidence of human utilization of rainforest resources anywhere in the world. Stable isotope analysis of human and animal remains, alongside detailed zooarchaeological analyses, have demonstrated reliance on rainforest resources as far back as 48,000 years ago. However, changes in human adaptations and the varying niches of exploited fauna, through major periods of climatic change such as during the Pleistocene-Holocene transition, remain relatively under-explored. Here, we present the results of stable carbon (δ13C) and oxygen (δ18O) isotope analyses of dental enamel from 311 animal and eight human teeth recovered from recent excavations at Fa-Hien Lena and Kitulgala Beli-lena from contexts spanning the Late Pleistocene and Holocene. Our data for human teeth from the Terminal Pleistocene and Holocene layers of Fa-Hien Lena and the Holocene layers of Kitulgala Beli-lena show little departure from rainforest resource reliance between the Pleistocene and Holocene. Meanwhile, the most dominant faunal taxa, including cercopithecid monkeys, show a similar stability in canopied forest habitation across the different species. However, δ13C data from ungulates found at Fa-Hien Lena indicate human populations did have access to forest edge and grassland habitats, suggesting either specific foraging trips or long-distance trade with other communities. In addition, our reporting of an Early Holocene presence of rhinoceros in the Wet Zone forest of Sri Lanka, the last fossil occurrence of this now regionally-extirpated taxon, highlights ongoing human interactions with large mammal communities on the island. We argue that our data demonstrate the benefits of detailed isotopic and zooarchaeological studies for detailed insights into the nature of tropical human adaptations through time.
Sri Lanka has emerged as a key region for understanding human adaptations to tropical environments from the Late Pleistocene through into the Holocene. Archaeological sites on the island have provided some of the earliest direct evidence of human utilization of rainforest resources anywhere in the world, including targeted hunting of arboreal and semi-arboreal small bodied mammals using projectile technology as early as ∼45,000 years ago (Wedage et al., 2019a), as well as gathering and processing of freshwater and terrestrial molluscs and fruits and nuts (i.e., breadfruit Artocarpus sp., and kekuna nut Canarium sp.) (Wedage et al., 2020). These activities were facilitated by microliths (Perera et al., 2011; Roberts et al., 2015a; Wedage et al., 2019b; Picin et al., 2022) and bone tools (Perera et al., 2016; Langley, 2016; Langley et al., 2020), including bow-and-arrow technology, the earliest found outside Africa so far, as well as implements for working skin and/or plant fibres (Langley et al., 2020). Archaeological investigations in the tropical rainforests of Sri Lanka have also produced the earliest Homo sapiens fossils found anywhere in South Asia (Kennedy et al., 1987; Kennedy and Deraniyagala, 1989; Kennedy, 2000).
These findings have challenged the previously held notion that tropical rainforests were ecological barriers to Pleistocene human dispersals (Bailey et al., 1989; Gamble, 1993). Moreover, the association of the earliest clear evidence for human occupation in Sri Lanka with rainforest environments questioned prevailing assumptions of the predominance of coastal settings in supporting human migrations around the Indian Ocean rim (Mellars, 2005; Mellars, 2006; Bulbeck, 2007). Renewed multidisciplinary investigations of three caves sites in Sri Lanka’s Wet Zone have continued to confirm the initial observations of Sri Lankan archaeologists from as early as the 1980s (Deraniyagala, 1992). Together, Batadomba-lena dated between∼39,000 to ∼10,000 years ago (Perera et al., 2011; Roberts et al., 2017b), Fa-Hien Lena dated ∼45,000–∼4,200 years ago (Wedage et al., 2019a) and Kitulgala Beli-lena dated ∼45,000–∼8,000 years ago (Wedage et al., 2020) provide a long-term record of human tropical forest interaction and records of early microlith and osseous technologies. Re-dating of early human occupational layers of Fa-Hien Lena and Kitulgala Beli-lena using methods that can address contamination in tropical environments (Higham et al., 2009), reassessment of the stratigraphy of the sites (Kourampas et al., 2009; Wedage et al., 2019a), detailed analyses of materials recovered from previous excavations and, in the case of Kitulgala Beli-lena, materials from new excavations, including lithic (Wedage et al., 2020) and osseous (Langley et al., 2020) artefacts, and faunal and botanical remains (Wedage et al., 2020), have been particularly important for developing more resolved insights into the specialized tropical adaptations of humans in this part of the world.
Stable isotope analyses of human and animal tooth enamel from Batadomba-lena and Fa-Hien Lena have also provided additional, clear evidence for the overall reliance of Late Pleistocene-Holocene human populations on resources from the rainforest (Roberts et al., 2015b; Roberts et al., 2017b). This approach, by looking at “bulk” dietary contributions of resources from different environments, is able to test between scenarios of seasonal rainforest use (Deraniyagala, 1992) or past rainforest fragmentation and long-term reliance of humans on these tropical habitats. Stable carbon isotope data from human teeth recovered from ∼38–36,000 year old sedimentary layers in the site of Batadomba-lena (located ca. 25 km from Fa-Hien Lena and ca. 25 km from Kitulgala Beli-lena) showed year-long exploitation of rainforest resources (Roberts et al., 2017b). Reliance on rainforest resources apparently continued despite evidence for environmental fluctuations (i.e., development of more open forests and grasslands) during the Last Glacial Maximum up until the Mid-Holocene. The available isotope data from Batadomba-lena and Fa-Hien Lena suggest that forests were more open from ∼36–29,000 years ago to 12,000, followed by an expansion of rainforests during the Pleistocene-Holocene transition, around the same time that the Wet Zone, Intermediate Zone and Dry Zone climatic and vegetation boundaries stabilized (Roberts et al., 2017b). This raises the question of the degree to which humans, and their associated prey species, adjusted their tropical foraging strategies across this period of major change.
In this paper, we add to the existing stable isotope data for the island of Sri Lanka by conducting novel stable carbon (δ13C) and oxygen (δ18O) isotope analyses of dental enamel from human and animal teeth recovered from recent excavations of Fa-Hien Lena and Kitulgala Beli-lena. Our goal is to look at the varying niches of fauna exploited by foragers that inhabited the cave sites as an indicator of changes in human adaptations, for instance the development of new hunting technologies as revealed by recent studies looking at the lithic and osseous materials from the sites (Wedage et al., 2019b; Langley et al., 2020; Picin et al., 2022). We also focus on the materials recovered from the Terminal Pleistocene and Holocene layers to investigate whether signatures for the expansion of rainforests during the Pleistocene-Holocene transition can be detected in the fauna from the cave sites. Although emphasis has often been placed on the utilization of the sites as early as ∼45,000 years ago, the majority of the artefacts and faunal remains from both sites were recovered from archaeological layers dating to the Terminal Pleistocene and the Holocene. Whereas lithics and botanical remains were recorded in all stratigraphic units of Kitulgala Beli-lena, faunal remains were restricted to the Holocene layers, for example (Wedage et al., 2020). By focusing on these time periods, we hope to be able to shed further light on the nature of human tropical adaptations in Sri Lanka, during a time period where significant climatic and environmental changes impacted hunter-gatherer communities in different parts of tropical Asia and beyond.
We selected human and animal teeth recovered from the 2010–2012 excavations of Fa-Hien Lena and the 2017 excavations of Kitulgala Beli-lena. Both caves are located, ca. 45 km away from each other, in Sri Lanka’s Wet Zone region (Figure 1), a vegetation zone characterized by wet deciduous and tropical evergreen forests which receives around 2,200 mm–4,800 mm of rainfall annually (in contrast to the grassland-dominated Dry Zone region which receives an annual rainfall of ∼1,000 mm–1700 mm) (Ashton and Gunatilleke, 1987; Erdelen, 1988; Roberts et al., 2015b). The detailed stratigraphic sequences of both sites have been published in previous papers (Wedage et al., 2019a; Wedage et al., 2020) and we therefore provide just a brief summary description here.
FIGURE 1. Map of Sri Lanka showing the location of Fa-Hien Lena and Kitulgala Beli-lena as well as the island’s vegetation zones [redrawn based on data from (Gunatilleke et al., 2005; Erdelen, 1988)].
Fa-Hien Lena, located in the district of Kalutara in Sri Lanka’s Western Province (6°36′6″N 80°13′6″E, ca. 180 mASL and ca. 30 km away from the nearest coast), was first excavated in 1986–1988 (Deraniyagala, 1992) and yielded the earliest Homo sapiens fossils in South Asia (Kennedy et al., 1987; Kennedy and Deraniyagala, 1989). The rockshelter has an approximate surface area of 18 m2. Initially dated to ca. 38,000 years ago (Wijeyapala, 1997), renewed excavations of 2009–2012 in 3 1 m × 1 m trenches (squares N3, O3 and P3, see Supplementary Figure S1) revealed undisturbed Late Pleistocene sedimentary layers dated between ∼48,000 and ∼37,000 cal years BP (Wedage et al., 2019a) (Supplementary Figure S1, Table S1). These deposits preserved numerous faunal remains (∼30% of which showed evidence of burning), Conus shell beads, ochre fragments, quartz microliths and osseous points which represent the earliest evidence for human presence in Sri Lanka so far. Overlying the clayey silt Late Pleistocene deposits is a series of organic-rich loam layers characterized by heavy ash and charcoal concentration which represents the site’s Terminal Pleistocene occupational phase. Despite its relatively narrow temporal range (dated to 13,000–12,000 cal. BP), these deposits contained the densest concentration of artefacts and human occupational debris in the entire Fa-Hien stratigraphy. The Holocene occupational phase of the site is characterized by sandy silt and ash accumulation deposits representing undisturbed occupation floors dated between 8,000 and 4,500 years ago. Carbonized kekuna (Canarium sp.) nut endocarps were recovered in number from hearth features within these floors and some were directly dated to ca. 8,000–7,700 cal. BP (Wedage et al., 2020). The 2010–2012 excavation, which resolved the chronostratigraphy of the site, also yielded an estimated 150,000 bone fragments from which 14,485 fragments were sampled (from intact layers that can be associated with 14C dates) and analysed in detail. The number of lithic and osseous artefacts (both finished and unfinished) and the numerous faunal and botanical specimens recovered, in addition to the thick accumulations of ash and layers of occupational debris recorded, suggest that Fa Hien was utilized as a habitation site for ca., 40,000 years. We selected the human and faunal dental elements we analysed in this study from this sample so as to allow comparison of our results with those derived from the wider zooarchaeological analyses (Wedage et al., 2019a).
Kitulgala Beli-lena is located in Sabaragamuwa Province (7°0′8″N 80°26′8.8″E, ca., 380 mASL, and ca., 60 km away from the nearest coast) and was first excavated by PEP Deraniyagala in 1960–1961, and from the late 1970s to the early 2010s by Sri Lanka’s Department of Archaeology (Wijeyapala, 1997). Kitulgala Beli-lena’s main chamber has an estimated floor area of ca. 1,600 m2, of which a total of 80 m2 was previously excavated (see Supplementary Figure S2). These excavations exposed a 3 m cultural sequence dated between ca. 31,000–5,000 years BP along with human remains from at least ten individuals dating to ca. 13,000 years BP as well as a wealth of quartz lithic artefacts and osseous tools and a rich assemblage of faunal remains (Wijeyapala, 1997; Kourampas et al., 2009). In 2017, new excavations in Kitulgala Beli-lena were conducted with the aim of recovering new materials that would allow refinement of the chronology as well as improved understanding of the material culture, technology and subsistence economies of the humans that utilized the site. The excavation of two 1x1 m trenches revealed ca. 1.92 m of stratified detrital sediments deposited between 44,000 and 8,000 cal. BP representing four major phases of human occupation of the site (Wedage et al., 2020) (Supplementary Figure S2, Table S2). Although lithic artefacts and plant macrobotanical remains were recovered all throughout the sequence, faunal materials were only identified in the Holocene deposits (10,577–8,029 cal., BP). This was attributed to possible differential use of cave space by the Late Pleistocene and Holocene foragers occupying the site. The taxonomic composition of the faunal remains in Kitulgala Beli-lena is similar to that recovered from contemporaneous layers in Fa-Hien Lena (Wedage et al., 2020), with cercopithecid monkeys and squirrels dominating the assemblage (accounting for 32.4% and 21.2% of the identified remains respectively) in addition to a few large mammals (suids, bovids and cervids). Although detailed analyses of lithic artefacts and faunal materials recovered from the earlier excavations of the site are yet to be conducted, the current evidence we have suggests, that akin to Fa-Hien Lena, Kitulgala Beli-lena was utilized as a habitation site from the Late Pleistocene to the Early Holocene. We selected all unburnt faunal and human dental elements recovered from the 2017 excavation of the site and included them in the current study. In the case of complete mandibles and maxilla (or for fragmentary dental elements that showed association), we selected a single element to be sampled.
Although we tried to include as many taxa as possible, the taphonomy of certain specimens prohibited inclusion in the study. For instance, several canid and felid teeth exhibited evidence of burning and remineralization and were therefore excluded from the analyses. Likewise, numerous dental elements selected from 14C-dated layers at Fa-Hien Lena were heavily covered in calcium carbonate making sampling difficult. As a result, we also included faunal dental elements recovered from undated sedimentary layers that lie between or associated with dated archaeological deposits (Supplementary Figures S1, S2). We selected a total of 311 faunal and eight human teeth for analyses (detailed in Table 1). We divided the chronology of both sites into four phases: Late Pleistocene (ca. 45,000–16,000 BP), Terminal Pleistocene (16,000–11,600 BP), Holocene1 (11,6000–9000 BP) and Holocene2 (< 9000 BP). Samples from undated Holocene contexts were included in the Holocene2 phase as there is a lack of detailed resolution of archaeological layers dated to ca. 9,000 and younger at Fa-Hien Lena (i.e., undated archaeological layers cannot be assigned to either the Early or Mid-Holocene but they are overlying sediments dated to ca. 9,000 years BP).
TABLE 1. Human and animal teeth from Fa-Hien Lena and Kitulgala Beli-lena analysed in the current study (detailed list in Supplementary Table S3) (LP- Late Pleistocene, TP- Terminal Pleistocene, H1- Holocene1, H2- Holocene2).
We build on previous zooarchaeological analyses of faunal remains recovered from Fa-Hien Lena and Kitulgala Beli-lena (Wedage et al., 2019a; Wedage et al., 2020) and identified sampled dental elements to genus whenever possible following published morphological criteria (e.g., Hillson, 1996; Swindler, 2002; Hillson, 2005; Hillson, 2016). Previously, we tried to identify the colobine teeth recovered from Fa-Hien Lena to species level (Wedage et al., 2019a). However, we acknowledge that it is not straightforward due to significant overlap in linear dental measurements and morphological features between the co-occurring grey langur (Semnopithecus priam) and purple-faced langur (Trachypithecus vetulus) (Amano et al., 2020). In contrast to Southeast Asia (Ki-Kydd and Piper, 2004; Piper et al., 2008; Ingicco et al., 2020; Amano et al., 2022), there is currently a lack of studies looking at morphological criteria for discriminating the colobines in South Asia (see Huffman et al., 2022). In a recent paper, we chose a more conservative identification method where we grouped the colobines together- and only discriminated them against macaques (Macaca sinica) (Wedage et al., 2020). We adopt the same approach here and, whenever possible, assigned the langurs as colobines and the macaques as cercopithecines. However due to the fragmentary nature, in addition to heavy wear, of the non-human primate teeth, the majority of the samples (71%) were just assigned as representing “Cercopithecidae”. Lastly, we classified the identified taxa to size class based on live weight, large mammals being animals weighing 25 kg or more [modified from (Thomas, 1969; Grayson, 1978)].
Comparison of isotopic signatures between faunal and human tissues, such as bones and dental enamel, is useful for reconstructing past vegetation and environments. The majority of plants generally employ one of two photosynthetic pathways: a C3 pathway utilized by dicots (bushes, herbs and trees) wherein a three-carbon compound is produced and the C4 pathway used by most grasses wherein carbon dioxide is initially fixed into a four-carbon molecule during photosynthesis. The C4 pathway allows for photosynthesis to proceed without significant water loss and is therefore advantageous in warm, open and dry environments (Pearcy and Ehleringer, 1984). The difference in the way carbon dioxide is fixed allows C3 and C4 plants to be distinguished based on their δ13C values which is in turn reflected in the tissues of the animals and humans that consume them. Considering stable carbon isotopes in the dental enamel, browsers usually have δ13C values lower than −8‰, grazers have δ13C values higher than −2‰ (average ∼0‰) and mixed feeders have values somewhere in between (Lee-Thorp et al., 1989; Cerling and Harris, 1999; Lee-Thorp et al., 2010). In tropical forest settings, the presence of thick canopy reduces the available carbon dioxide in the forest floor resulting in lower δ13C values. This “canopy effect” is reflected in the tissues of animals and humans relying on resources in dense forests, with their tooth enamel having δ13C values lower than −14‰ (Cerling et al., 2004) (though see (Tejada et al., 2020) for a note on baseline variation in this regard).
Stable oxygen isotope analyses of teeth can provide additional information on human and faunal paleoecology. δ18O values of tooth enamel are influenced by a wide range of factors including habitat and climate, as well as the animal’s drinking behavior, physiology and diet (Kohn, 1996; Pederzani and Britton, 2019). For example, the δ18O values in teeth of mammals that are obligate drinkers have been shown to closely reflect the δ18O values of the water they imbibe which is often strongly associated with precipitation δ18O. By contrast, non-obligate drinkers, which rely mostly on the plants they consume as their water source, have higher δ18O values indicative of the high δ18O values in leaves as a product of environmental factors influencing transpiration (Kohn, 1996). The δ18O values of vegetation in tropical ecosystems have also been shown to reflect either the source-effect of rainfall or evaporative potential, which can be used to infer canopy density and environmental change (Buchmann et al., 1997; Buchmann and Ehleringer, 1998).
The details of the archaeological animal and human teeth analysed in this study are presented in Supplementary Table S3, including the specimen number, excavation context, associated chronology, taxon identification, and element. Dental enamel records isotopic information related to diet and hydrological context over the period of enamel formation and therefore will vary depending on the species and the tooth sampled. For humans, the third molar, which develops between 7 and 13 years until the mid to late teenage years (Hillson, 1996) is an ideal representative of the late juvenile/adult diet. By contrast, first molars are generally avoided due to the temporal overlap between their formation and weaning (Wright and Schwarcz, 1998). For the current study, however, we were limited by the scarcity of human remains recovered from both sites (Fa-Hien Lena, n = 7; Kitulgala Beli-lena, n = 1) and no third molars were available (see Supplementary Table S3). Nonetheless, this does not hinder exploration of the significant δ13C differences between signatures of consumers relying on closed canopy C3, intermediate/open C3 and C4 resources, which is the primary focus of our study.
All selected samples were first cleaned using air abrasion to remove sediments and other minerals (i.e., manganese oxide and calcium carbonate) adhering to the tooth surface. We employed bulk sampling of dental enamel by gently and evenly abrading the full length of the buccal surface of the teeth with a diamond-tipped drill, ensuring a representative sample for the whole axis of enamel mineralization. Organic and secondary contaminates were removed from the obtained enamel powder by pre-treatment following established protocols (Sponheimer et al., 2005; Lee-Thorp et al., 2012; Roberts et al., 2015b; Roberts et al., 2017b; Roberts et al., 2018; Ventresca Miller et al., 2018) which also allows for comparison between obtained isotopic measurements and those in the existing literature for Sri Lanka. This involved washing the samples with 1.5% NaOCl for 60 min followed by repeated rinsing with purified water. The samples were then washed with 0.1 M acetic acid for 10 min followed again by repeated rinsing with purified water, after which the samples were lyophilized for 24 h. Phosphoric acid (100%) was then added to ∼2 mg of the lyophilized samples at 70°C and the resulting gasses were measured by stable carbon and oxygen isotope analysis using a Thermo Gas Bench 2 connected to a Thermo Delta V Advantage Mass Spectrometer at the Stable Isotopes Laboratory, Max Planck Institute for Geoanthropology (formerly for the Science of Human History). The obtained δ13C and δ18O values were compared against international standards [IAEA-603 (δ13C = 2.5‰; δ18O = −2.4‰); IAEA-CO-8 (δ13C = 5.8‰; δ18O = −22.7‰); USGS44 (δ13C = −42.2‰)] and an in-house standard [MERCK (δ13C = −41.3‰; δ18O = −14.4‰)] using the Isodat 3.0 software from Thermo Electron Corporation. Machine measurement error is c. + 0.1‰ for δ13C and + 0.2‰ for δ18O, as determined by replicate analysis of MERCK carbonate standards.
All δ13C and δ18O measurements were tested for normality using the Shapiro-Wilk Test. Following observations of non-normality, the significance of δ13C and δ18O between different time periods and between different faunal groups was tested using Kruskal–Wallis tests and, if significant, the tests were followed by a post hoc Dunn test to determine which groups were significantly different from each other. The same approach was employed to make comparisons between different taxa in all time periods as well as between cercopithecids in different time periods. Analysis of variance (ANOVA) followed by post hoc Bonferroni/Tukey pairwise comparisons were conducted in cases where normality is observed. All statistical analyses were conducted using R (R Core Team, 2016).
δ13C of faunal teeth sampled from Late Pleistocene to Holocene layers of Fa-Hien Lena and the Holocene layers of Kitulgala Beli-lena showed the predominance of taxa with closed-canopy C3 signatures (Figures 2–7). Considering all samples from both sites, cercopithecid monkeys (including both macaques and langurs) have a δ13C range of −17.8‰ to −11.1‰ (n = 178, mean = −14.9‰) (Figures 4–6). Giant (Ratufa macroura) and flying (Petinomys/Petaurista) squirrels have a range of −16.3‰ to −10.9‰ δ13C (n = 46, mean = −13.7‰) and −15.3‰ to −11‰ (n = 4, mean = −12.9‰), respectively (Figures 4, 7). Viverrids (including Paradoxurus and Viverricula) have δ 13C ranging from to −15.9‰ to −10.7‰ (n = 54, mean = −13.4‰) (Figures 4, 7). Similarly, smaller-bodied artiodactyls including mouse-deer (Moschiola) (−12.9‰ to −11.5‰, n = 5, mean = −12.2‰) and muntjac (Muntiacus) (n = 2, mean = −12.3‰) exhibited low δ13C values (Figures 4, 8). By contrast, larger bodied artiodactyls such as suids (−12.9‰ to −5.8‰, n = 5, mean = −9.84‰), and elephants (n = 2, mean = −7.5‰) exhibited higher δ13C values (Figures 2, 4, 8). The same is the case for the single rhinoceros specimen having a δ13 of −3.3‰. Cervids (Rusa/Axis) have the highest δ 13C observed, with values ranging from −0.96‰ to 2.2‰ (n = 9, mean = 1.13) (Figures 4, 8).
FIGURE 2. δ13C measurements for human and faunal teeth from Fa-Hien Lena and Kitulgala Beli-lena analysed in this study. (A) Data for all taxa (including humans) in different time periods. (B) Data for animal taxa (in all time periods) separated by class size (Large mammals weighing > 25 kg) compared to human samples. Boxplots show median and interquartile range, as well as data points including outliers. All data presented in detail in Supplementary Table S3. (LP- Late Pleistocene, TP- Terminal Pleistocene, H1- Holocene1, H2- Holocene2).
FIGURE 3. Stable carbon (δ13C) and oxygen (δ18O) isotope data for human and animal tooth enamel samples from Fa-Hien Lena and Kitulgala Beli-lena.
FIGURE 4. δ13C measurements for human and faunal tooth from Fa-Hien Lena and Kitulgala Beli-lena analysed in this study grouped by taxon. Boxplots show median and interquartile range, as well as data points including outliers. All data presented in detail in Supplementary Table S3. (LP- Late Pleistocene, TP- Terminal Pleistocene, H1- Holocene1, H2- Holocene2) (Animal silhouettes from phylopic.org, modified under Creative Commons license).
FIGURE 5. Stable carbon (δ13C) and oxygen (δ18O) isotope data from tooth samples of non-human primates from Fa-Hien Lena and Kitulgala Beli-lena (datapoints with cross +).
FIGURE 6. δ13C measurements for cercopithecid monkey (including both langurs and macaques) teeth from Fa-Hien Lena and Kitulgala Beli-lena grouped based on time period. Boxplots show median and interquartile range, as well as data points including outliers. All data presented in detail in Supplementary Table S3. (LP- Late Pleistocene, TP- Terminal Pleistocene, H1- Holocene1, H2- Holocene2).
FIGURE 7. Stable carbon (δ13C) and oxygen (δ18O) isotope data from tooth samples of small mammals (live weight < 25 kg) from Fa-Hien Lena and Kitulgala Beli-lena (datapoints with cross +).
FIGURE 8. Stable carbon (δ13C) and oxygen (δ18O) isotope data from tooth samples of artiodactyls from Fa-Hien Lena and Kitulgala Beli-lena (datapoints with cross +). Datapoints with silhouettes indicate specimens shown in Figure 9. (Animal silhouettes from phylopic.org, modified under Creative Commons license).
In terms of δ18O values, cercopithecid monkeys exhibited values ranging from of −6.4‰ to −1.9‰ (n = 178, mean = −4.4‰), giant squirrels between −8.3‰ and −3.0‰ δ13O (n = 46, mean = −4.6‰), flying squirrels between −6.3‰ and −4.1‰ (n = 4, mean = −5‰), civet cats and porcupines between −7.3‰ and −4.1‰ (n = 54, mean = −4.7‰) and −6.6‰ to −3.1‰ (n = 5, mean =−5.8‰) respectively and mouse-deer between −7.5‰ and −4.5‰ (n = 5, mean = −5.6‰). With the exception of muntjacs, which have the lowest δ18O values in the sample set analyzed (mean = −7.4‰, n = 2), larger bodied taxa exhibited higher δ18O values. Suids exhibited a wide range of δ18O values ranging from −7.3‰ to −2.1‰ (n = 5, mean = −4.8‰). The two elephant samples have an average δ18O values of −2.7‰ while the single rhinoceros specimen yielded a value of −3.8‰. In comparison, the larger cervids have δ18O between −5.2‰ and −0.9‰ (n = 8, mean = 3.9‰).
The six human specimens from the Terminal Pleistocene of Fa-Hien Lena have δ13C values ranging from −14.2‰ to −12.8‰ (mean = −13.6‰) and δ18O values between −6.0‰ and −4.4‰ (mean = −5.1‰). By comparison, the single specimen from the Early Holocene phase of the site has a δ13C of −13.3‰ and δ18O of −5.3‰. The δ13C and δ18O values of the human molar fragment from the Holocene layers of Kitulgala Beli-lena fall within the range of the values of obtained from the Fa-Hien specimens (δ13C= 12.7‰ and δ18O= −4.2‰).
A Shapiro-Wilk test for normality indicates that the δ13C (p = <0.05) of the dataset when grouped according to taxon follows a normal distribution (Supplementary Table S4). An ANOVA test shows that taxonomic grouping significantly influences δ13C [F (10,306)= 1.86, p<0.05], Supplementary Table S5) and post hoc Bonferroni and Tukey pairwise comparisons suggest that larger ungulates, specifically cervids, suids and elephants have significantly different δ13C from the smaller-bodied taxa (Supplementary Tables S6, S7). The post hoc tests likewise show that the δ13C of the human specimens are distinct from cercopithecid monkeys, mouse-deer, suids and larger cervids (Supplementary Tables S6, S7; Figures 3, 4). Like the δ13C values, the δ18O values are also different between taxonomic groups as shown by an ANOVA test [F (10,306)= 4.76, p<0.05], Supplementary Table S7). Post hoc pairwise comparisons point to the δ18O of muntjacs and elephants being different from some of the other taxa, though this could be an artifact of the small sample size for both group (Supplementary Tables S9, S10).
In terms of the difference of δ13C and δ18O datasets between different occupational phases within Fa-Hien Lena and Kitulgala Beli-lena, a Shapiro-Wilk test indicated that the values, when grouped according to chronology, do not follow a normal distribution. Kruskal–Wallis analyses indicate there to be significant δ13C (Hchi2: 94.83, df= 4, p<0.05) differences between chronological phases within the sites. Post hoc Dunn tests suggest that there is a significant difference in the δ13C between the Late Pleistocene and the later Holocene (H2) phase of Fa-Hien Lena (Supplementary Table S11). The tests also indicate significant δ13C differences between the Terminal Pleistocene and the Holocene (both H1 and H2) phases of site occupation. The difference appears to be driven by the lower δ13C values for H1 and H2 (Figure 2A). Interestingly, the post hoc Dunn tests indicate that the Holocene phase of Kitulgala (H2) has distinct δ13C values from the Late Pleistocene and Holocene (H1 and H2) phases of Fa-Hien Lena. Visually (Figure 2A), it appears that this is driven by the higher δ13C values for Kitulgala Beli-lena, which is within the range of values for the Terminal Pleistocene of Fa-Hien. Kruskal–Wallis analyses also indicate significant differences (Hchi2: 10.22, df= 4, p<0.05) in δ18O values between the different chronological phases of the sites. Post hoc Dunn tests suggest that the δ18O values for the later Holocene (H2) phase of Fa-Hien Lena are distinct from the earlier Holocene and Terminal Pleistocene phases with the box plot (Supplementary Figure S4) showing lower δ18O values for the former. Likewise, the Holocene phase (H2) in Kitulgala Beli-lena has significantly lower δ18O values compared to the earlier Holocene (H1) phase of Fa-Hien (Supplementary Table S12, Figure S4).
The δ13C and δ18O values for cercopithecid monkeys are good indicator of the types of environments exploited by the forager communities that inhabited Fa-Hien Lena and Kitulgala Beli-lena as they were the most common animals exploited in all phases of occupation in both sites. Shapiro-Wilk tests indicate that the δ13C (p<0.05) and δ18O (p<0.05) values for monkeys, when grouped according to chronology, do not follow a normal distribution. Kruskal–Wallis analyses indicate there to be significant δ13C (Hchi2: 71.97, df = 4, p<0.05) and δ18O (Hchi2: 16.95, df = 4, p<0.05) differences between chronological phases within the sites. Post hoc Dunn tests suggest that there is a significant difference in δ13C values of monkeys from Late Pleistocene compared to the Holocene phases of Fa-Hien Lena. Similarly, the cercopithecid samples from the Terminal Pleistocene phase have distinct δ13C values compared to those from H1 and H2. The δ13C values of monkey teeth from the Holocene phase (H2) of Kitulgala Beli-lena are significantly different from the values from all occupational phases of Fa-Hien Lena except for the Terminal Pleistocene (p= 0.072) (Supplementary Table S13). This mirrors the results obtained when all taxa are grouped according to chronological phase, which the scatter (Figure 5) and box (Figure 6) plots suggest is due to lower δ13C values of monkey teeth in the Holocene levels of Fa-Hien Lena. By contrast, the monkey teeth from the Holocene layers of Kitulgala Beli-lena have higher δ13C values (−15.1‰ to −11.1‰, mean= −13.5‰) compared to those from the early (−17.1‰ to −13.8‰, ave= −15.3‰) and later (−16.9‰ to −12.4‰, mean= −15.6‰) Holocene phases of Fa-Hien Lena.
In terms of differences in δ18O values of the primate samples analysed, post hoc Dunn pairwise comparison only draws out the later Holocene phase (H2) as being significantly different from the earlier Holocene (H1) and Terminal Pleistocene phases (Supplementary Figure S5, Table S12). Previously, it was shown that T. vetulus has lower δ13C than M. sinica in Fa-Hien Lena (Roberts et al., 2017a). We originally aimed to evaluate if this is the case with the new samples from the site as well as in Kitulgala Beli-lena, but our adoption of stricter identification methods reduced our sample size significantly. Of the 178 cercopithecid dental elements selected for the study, only 51 can be identified as either langur (T. vetulus/S. priam) or macaque (M. sinica). Grouping langur (−17‰ to 12.1‰, ave= −15.08) and macaque (−16.93 to −12.3‰, ave= −14.36) specimens together without consideration of the sites’ occupational phases, T-tests showed that there is no significant difference between their δ13C (df= 49, t= −1.92, p>0.05) and δ18O (df= 49, t= 0.01, p>0.05) values.
The fauna recovered from the different occupational phases of Fa-Hien Lena and the Holocene layers of Kitulgala Beli-lena can be broadly divided into three different groups based on their δ 13C signatures: taxa with low δ 13C values suggestive of high proportion of C3 biomass in their diets and therefore dependence on forests or closed environments which include small bodied mammals such as monkeys, squirrels, civet cats and porcupines as well as smaller ungulates such as mouse-deer and muntjacs (between −17.5‰ and −12‰); taxa including pigs, elephants and rhinos, with higher δ 13C values (between −12‰ and −3‰) which is indicative of feeding on a slightly more mixed C3/C4 biomass and therefore representative of animals inhabiting mixed closed-canopy to intermediate open environments; and taxa with high δ 13C (> −3‰), which include larger cervids (Axis/Rusa), indicative of high proportion of C4 in their diet, and therefore representing animals in grasslands/open environments. A similar distribution of habitats for prey animals was observed at the site of Balangoda Kuragala (Roberts et al., 2015b) which today sits within the Intermediate Rainforest Zone of the island (Roberts et al., 2015a). Isotope data at this site highlighted human access to forest dwelling primates and squirrels as well as open dwelling elephants and lagomorphs (Roberts et al., 2015b), with a clear preference for the former and forested habitats. Interestingly, while Balangoda Kuragala is located at the edge of a forest-grassland ecotone today, our new data demonstrate that the access to open-dwelling animals was even maintained by Wet Zone rainforest communities across the Terminal Pleistocene and Holocene.
A single rhinoceros specimen, a broken piece of an upper premolar/molar (buccal side), too fragmented to be confidently assigned to element (Figure 9B), from the Early Holocene layers of Fa-Hien Lena has a δ13C of −3.3‰ ± 0.1‰, suggesting mixed forest/open environment foraging. Previously, isolated teeth identified as representing “Rhinoceros sivalensis sinhaleyus” were reported from the Ratnapura beds of Western Sri Lanka, associated with hippopotamus (Hexaprotodon sivalensis) and elephant fossils and presumed to be from the Upper Pleistocene, although no dates are available (Deraniyagala, 1938; Deraniyagala, 1965). To our knowledge, there are no other reported occurrences of rhinoceros in any Pleistocene/Holocene archaeological sites in the island and the tooth fragment we recorded at Fa-Hien Lena is the evidence for the last appearance of the taxon in Sri Lanka. Although there are no direct dates for the specimen, the archaeological layer where it was recovered (c. 168) is sandwiched by layers dated between 7,955 and 7,791/8,595–8,430 cal. BP., (c. 153/138) and 12,575–12,150 cal. BP. (c. 174) [see (Wedage et al., 2019a) for details]. The δ13C value for the Fa-Hien Lena rhino is slightly higher compared to the range of reported Indian rhino (R. unicornis) δ13C values from the Late Middle Pleistocene of Thailand (Suraprasit et al., 2018) and lines up with known diets of modern Indian rhino populations which include mostly grasses but also leaves, shrubs, tree branches and fruits (Laurie, 1982; Nowak and Walker, 1999). By contrast, the δ13C values for the more forest-adapted Javan rhinoceros (R. sondaicus) from the Middle Pleistocene to Early Late Pleistocene of Thailand ranged from −17.8‰ to −12.0‰ (Suraprasit et al., 2018). Overall, the presence of the rhinoceros remain in the site highlights the longevity of human interactions with large mammals in Sri Lanka, even as they focused primarily on small-bodied arboreal and semi-arboreal prey.
FIGURE 9. Example of large mammal specimens analysed in the study. (A) Elephant (Elephas) molar fragment (BYP 216) (B) Rhinoceros molar fragment (BYP 208) (C) Suid (Sus scrofa) mandibular M3 (BYP240) (D) Cervid (Munitacus muntjak) mandible fragment (BYP206B) (E) Cervid (?Rusa/Axis) maxillary M2 (BYP204) (F) Cervid (?Rusa/Axis) mandibular M3. (Animal silhouettes from phylopic.org, modified under Creative Commons license).
Similarly, cervids identified as belonging to the genus Rusa or Axis exhibited distinct open/grassland C4 signatures with δ 13C ranging from −1.0 to 2.2 (n = 9, mean = 1.13) (Figures 4, 8). On-going work (Amano et al., In Prep) aims to identify the dental element to specific taxon but the current lack of comparative data for Sri Lanka in addition to the fragmentary nature of the Fa-Hien Lena specimens limits our current identification. Three species of deer (in addition to muntjacs), the sambar (Rusa unicolor), the chital (Axis axis) and the hog deer (Axis porcinus) are currently present in Sri Lanka, albeit it is still uncertain whether the hog deer is native to the island (Vishvanath et al., 2014; Timmins et al., 2015). Throughout its wide distribution range, the sambar is known for its highly adaptive ecology and diets that consists of grasses, foliage, fruits and submerged water plants (Geist, 1998). In Sri Lanka, sambar deer from the Horton Plains National Park were found to be more dependent on graminoid grasses, though food compositions varied heavily depending on the season (Padmalal et al., 2003). By contrast, the chital is known to be more of a grazer, feeding mostly on grasses, although it is also known to feed on flowers and fruits and will occasionally browse when necessary (Moe and Wegge, 1994). In Sri Lanka, the chital is currently inhabiting the shrubby areas in the Dry Zone (Balasubramanian et al., 1980).
The δ13C values of the large cervids from the Terminal Pleistocene and Holocene layers of Fa-Hien Lena are much higher than the δ13C values for R. unicolor (−12‰ to −4.1‰, ave= −7.74‰) and A. axis (−12.1‰ to −11.7‰, ave= −11.9‰) reported from contemporaneous archaeological layers in Balangoda Kuragala mentioned above (Roberts et al., 2015b). The high δ 13C values of these animals suggest that the people that utilized Fa-Hien Lena during the Terminal Pleistocene/Early Holocene had access to forest edges or grassland environments where they hunted cervids in presumably long expedition trips. Given the overall forest reliance recorded in human δ13C values at these rainforest sites, it is possible that the foragers that inhabited Fa-Hien Lena employed a mobility organization and/or hunting strategy wherby small groups undertook some specialized long-distance (ca. >60 km) hunting trips. Alternatively, it is also possible that the deer skeletal elements were sourced from other groups which had access to more open environments though contact and trade networks. Interestingly, cervid specimens were present in very low frequencies (< 1.5%) in Fa-Hien indicating that they did not play a significant role in the subsistence of the people that settled the site. The cervids (and all other large ungulates for that matter) were mostly represented by tooth or long bone fragments, suggesting that they were not butchered on-site (Wedage et al., 2019a). Instead, cervid skeletal and dental elements were most likely brought to the site as finished tools or as materials for tool production as evidenced by the high proportion of worked fragments (Langley et al., 2020). Of the 72 deer skeletal and dental elements, 25% were worked metapodial and antler fragments. This suggests that certain parts of the deer carcass, the skull with the antler and the metapodials, were transported or traded over long distance. Although this remains to be ascertained, contact between groups focusing on different ecologies is also supported by the presence of shell beads manufactured from marine taxa as well as isolated shark teeth which were hypothesized to have been traded or exchanged with groups living in the coast (Langley et al., 2020). It is possible that coastal resources were sourced through expedition trips as Fa-Hien is only ca., 30 km away from the nearest coast, though, as noted above, human δ13C implies rainforest residence (Roberts et al., 2015b; Roberts et al., 2017b). Future work and discovery of coastal sites contemporaneous to Fa-Hien would be necessary to investigate these hypotheses.
The δ18O values we observed for the different taxa in Fa-Hien Lena and Kitulgala Beli-lena generally track their feeding position in the forest (i.e., “vertical stratification” (Cerling et al., 2004; Krigbaum et al., 2013; Carter and Bradbury, 2016), with animals feeding in the canopy having higher δ18O than animals such as mouse-deer and muntjacs which forage in the forest floor (Supplementary Figure S3). Specifically, post hoc Tukey pairwise comparisons point to muntjacs having significantly lower δ18O values compared to canopy-dwelling taxa such as monkeys, civet cats and squirrels. However, as with the δ 13C, we do not see any difference with the δ18O between langurs and macaques. We presume that this is brought about by our failure to discriminate between the obligate-drinker, canopy-dwelling T. vetulus which is expected to have higher δ18O than the semi-terrestrial, also obligate-drinking S. priam. But as pointed out by Roberts et al. (Roberts et al., 2017b), this could also be an issue of equifinality, with the elevated δ18O values in T. vetulus resulting from them feeding up high in the canopy and in S. priam (and M. sinica) possibly resulting from them drinking from open, 18O-enriched water sources in the ground. The latter could also explain the high δ18O values we observed for taxa in mixed/open environments including elephants and larger cervids. It would also fit with observations that the primate isotopic values track overall environmental changes through the occupation sequences of the site.
The δ13C values from the different occupational phases within Fa-Hien Lena hint at slight differences in the local environment in the site at different time periods. The difference appears to be driven by the lower δ13C values for the Holocene (H1 and H2), which is consistent with the previously reported increase in the canopy cover in/or around the site following the onset of the Holocene (Roberts et al., 2017b). This can be seen in the box plot considering all taxa (Figure 2A) and in the plot for δ13C of cercopithecid monkeys (Figure 6). By contrast, the δ13C values for human and fauna in the Holocene (H2) layers of Kitulgala Beli-lena is higher compared to contemporaneous layers in Fa-Hien Lena. This suggests that environment in/or around (or at least where the foragers hunted the fauna) Kitulgala Beli-lena is more open than in Fa-Hien Lena during the Early to Mid-Holocene (i.e., 9,000 years BP and later). This is consistent with existing, albeit sparse, paleoenvironmental proxy records in Sri Lanka. Pollen record from the Horton Plains, although distantly located from both Fa-Hien Lena and Kitulgala Beli-lena, indicate forest expansion from around 16,000–8,700 cal years BP (Premathilake and Risberg, 2003; Premathilake, 2012). An alternative explanation for the higher δ13C of fauna from the Holocene layers of Kitulgala Beli-lena could relate to the site’s location and environmental setting. Kitulgala is located closer to higher altitude rainforest and grassland environments as well to intermediate rainforests. The higher δ13C could be brought about by foragers exploiting more diverse ecological niches, including more open environments. Further research, perhaps looking at forager and prey mobility through strontium isotopes, would help shed light on this question.
Focusing on the primate data, the δ13C signatures of cercopithecid monkeys in the assemblage is consistent with previously published values for the three species that occur in Sri Lanka (Roberts et al., 2017b). We initially aimed to describe in detail the difference in the δ 13C signatures among these species. We were able to confidently discriminate isolated langur teeth from macaque teeth based on well documented colobine dental features (e.g., high, pointed molar cusps linked by ridges but separated by deep lingual notches) (Delson, 1973; Kay, 1975; Swindler, 1976). However, our application of a strict identification criteria fails to discriminate the different langur species from each other which resulted to a small sample size. We are currently looking at morphological/geometric morphometric features that could discriminate T. vetulus dental elements from those of S. priam (building on (Kay and Hylander, 1978; Wright and Willis, 2012)). As these species were previously shown to have slightly different δ 13C values (Roberts et al., 2017b), and are known to currently exploit slightly different habitats (Hladik, 1977; Dittus, 1984) differentiating them from each other would be important in reconstructing the different ecological niches exploited by monkeys and if/how they change through time. We observed no significant difference in the δ 13C values between the langurs and the macaques when grouped without consideration of the sites’ occupational layers. This is expected as previous research (Roberts et al., 2017b) has shown that the δ 13C values for S. priam sit between the values for heavily folivorous T. vetulus (Hladik, 1977) and the generalist M. sinica (Dittus, 1984). Interestingly, the δ 13C values for the monkeys track the observed δ 13C difference in the different occupational layers of Fa-Hien Lena, with both langurs and macaques in the Holocene layers of Kitulgala Beli-lena having higher δ 13C values compared to contemporaneous layers in Fa-Hien Lena (see Supplementary Figure S6 and discussion below). This shows that the langurs during the Holocene in Kitulgala were making use of more open habitats, indicating some dietary flexibility, which has also been suggested by colobine δ 13C values from Terminal and Late Pleistocene layers of Batadomba-lena (Roberts et al., 2017b) and studies looking at current langur populations (Dela, 2007; Amato et al., 2020).
Finally, moving to our human data, our study aligns well with previously published isotopic data (Roberts et al., 2017b) in suggesting reliance on rainforest resources by humans that inhabited Fa-Hien Lena from the Terminal Pleistocene (mean = −13.2‰, n = 6) to the Holocene (a single specimen with δ13C of −13.2‰ ± 0.1‰). The human specimen (a fragment of an isolated molar) from the Holocene layers of Kitulgala Beli-lena (δ13C of −12.7‰) also showed a C3 forest signature (Figures 2–4), albeit the δ13C value suggests a reliance on slightly more open forests (i.e. forest-open mosaics) similar to those found currently in Sri Lanka’s Wet and Intermediate Zones and in line with the slightly more open environment suggested by the δ13C from faunal dental enamel (see below). Interestingly, comparison with published data show that the δ13C of Terminal Pleistocene humans in Fa-Hien Lena (mean = −13.5‰, n = 7, df = 29, t = −2.88, p < 0.05) is lower than those from the Late Pleistocene of Batadomba-lena (mean = −12.7‰, n = 23) [(Roberts et al., 2017b) see Supplementary Figure S7], suggesting reliance on resources from more closed forest in Fa-Hien. In terms of δ18O, the human specimens from Fa-Hien Lena had values ranging between −6.5‰ and −4.4‰. Post hoc Tukey pairwise comparison show no significant difference between the δ18O values of the human specimens compared to cercopithecids and other small mammalian taxa (Supplementary Figure S3, Table S10). As humans are obligate drinkers, these values suggest humans mainly sourced their drinking water from with the local forest setting. Comparison with published data indicates that the Terminal Pleistocene specimens from Fa-Hien Lena (mean = −5.11‰, n = 8, df = 29, t = 5.59, p<0.0001) had lower δ18O than those from the Late Pleistocene of Batadomba-lena (mean = −3.11‰, n = 23). This may indicate denser forest conditions, and less evaporative impacts on water sources, in the vicinity of Terminal Pleistocene than at Late Pleistocene Batadomba-lena, something that has also been suggested in previous studies on the basis of faunal isotopes from these different contexts (Roberts et al., 2015b; Roberts et al., 2017a).
Figure 10, summarizes all the available dental enamel stable isotopes data for the island of Sri Lanka. Our data adds to the already huge dataset for the island, which overall shows continued human utilization of rainforest resources over the past ca. 48,000 years. Our results show that although humans in Sri Lanka’s Wet Zone region were reliant on forest resources, they had access to grassland and more open environments or had contact with groups exploiting different kinds of ecosystems. More research is required to understand these prehistoric mobility patterns or exchange networks, perhaps by employing strontium stable isotopes. Meanwhile, the sampling more animal taxa, and resolving taxonomy of closely related groups, could further enhance the understanding in the adaptations, not only of humans but also of other animal taxa to different ecological niches at different times. For example, the expansion of rainforests revealed by isotopic data during the Holocene in Fa-Hien Lena coincided with increased frequency of taxa usually associated with more open environments, including the Indian hare (Lepus nigricollis), jackal (Canis aureus) and cervids (Rusa/Axis) (Wedage et al., 2019a). Whether the presence of these taxa represent their adaptation to changing environments (i.e., Amano et al., 2016) or human foraging of different ecological niches remains to be fully explored.
FIGURE 10. Available stable carbon (δ13C) and oxygen (δ18O) isotope data for human and animal tooth enamel samples from Fa-Hien Lena, Kitulgala Beli-lena and Batadomba-lena. Data from (Roberts et al., 2015b; Roberts et al., 2017b) and this study.
The original contributions presented in the study are included in the article/Supplementary Material, further inquiries can be directed to the corresponding authors.
NA and PR designed the study with supervision from NB and MP. NA conducted the zooarchaeological analyses as well as the identification of dental elements. NA, JI, and PR conducted the stable isotopes analyses and analyzed the data. The excavations of Fa-Hien Lena and Kitulgala Beli-lena was conducted by OW with supervision from NB and MP. OW and NA are responsible for the curation and sampling of the finds from the excavations. NA and PR wrote the original draft and all coauthors contributed to the review and editing.
Funding for the study was provided by the Max Planck Society.
We deeply acknowledge Prof. Siran Deraniyagala who recently passed away for his contribution not only to our excavations of sites in the Wet Zone of Sri Lanka but for his work on the prehistory of the island. We thank Prof. S. Dissanayake, Prof. P.B. Mandawala, S.A.T.G. Priyantha, and other members at the Excavations Branch of the Department of Archaeology, Sri Lanka, for assistance and the support of our fieldwork. We also thank L.V.A. De Mel, K. K. Ruwan Pramod, P.G. Gunadasa, Suranga Jayasinha, K.A.S Lakmal, Tiran Ananda and J. Perera for their participation in the fieldwork. For support, we thank Prof. Alexander Kapukotuwa and other faculty members of the Department of History and Archaeology of the University of Sri Jayewardenepura. Mary Lucas and Erin Scott helped us during the stable isotopes analyses. This research was supported by the the research council of the University of Sri Jayewardenepura (RC-USJ) and the Max Planck Society.
The authors declare that the research was conducted in the absence of any commercial or financial relationships that could be construed as a potential conflict of interest.
All claims expressed in this article are solely those of the authors and do not necessarily represent those of their affiliated organizations, or those of the publisher, the editors and the reviewers. Any product that may be evaluated in this article, or claim that may be made by its manufacturer, is not guaranteed or endorsed by the publisher.
The Supplementary Material for this article can be found online at: https://www.frontiersin.org/articles/10.3389/feart.2023.1133281/full#supplementary-material
Amano, N., Ingicco, T., Moigne, A. M., Sémah, A. M., Simanjuntak, T., and Sémah, F. (2022). “Monkey hunting in early to mid-holocene eastern java (Indonesia),” in World archaeoprimatology: Interconnections of humans and nonhuman primates in the past. Editors B. Urbani, D. Youlatos, and A. Antczak (Cambridge: Cambridge University Press).
Amano, N., Rivals, F., Moigne, A. M., Ingicco, T., Sémah, F., and Simanjuntak, T. (2016). Paleoenvironment in East Java during the last 25,000 years as inferred from bovid and cervid dental wear analyses. J. Archaeol. Sci. Rep. 10, 155–165. doi:10.1016/j.jasrep.2016.09.012
Amano, N., Wedage, O., Boivin, N., Petraglia, M., and Roberts, P. (In Prep). An in-depth look at the terminal pleistocene/early Holocene faunal remains from archaeological sites of the Sri Lankan wet zone.
Amato, K. R., Kuthyar, S., Ekanayake-Weber, M., Salmi, R., Snyder-Mackler, N., Wijayathunga, L., et al. (2020). Gut microbiome, diet, and conservation of endangered langurs in Sri Lanka. Biotropica 52, 981–990. doi:10.1111/btp.12805
Ashton, P., and Gunatilleke, C. (1987). New light on the plant geography of Ceylon. I. Historical plant geography. J. Biogeogr. 14, 249–285. doi:10.2307/2844895
Bailey, R. C., Head, G., Jenike, M., Owen, B., Rechtman, R., and Zechenter, E. (1989). Hunting and gathering in tropical rain forest: Is it possible? Am. Anthropol. 91, 59–82. doi:10.1525/aa.1989.91.1.02a00040
Balasubramanian, S., Santiapillai, C., and Chambers, M. R. (1980). Seasonal shift in the pattern of habitat utilization by the spotted deer (Axis axis Erxleben 1977) in the Ruhuna National Park, Sri Lanka. Spixiana 3, 157–166.
Buchmann, N., and Ehleringer, J. R. (1998). CO2 concentration profiles, and carbon and oxygen isotopes in C3 and C4 crop canopies. Agric. For. Meteorology 89, 45–58. doi:10.1016/s0168-1923(97)00059-2
Buchmann, N., Guehl, J. M., Barigah, T. S., and Ehleringer, J. R. (1997). Interseasonal comparison of CO2 concentrations, isotopic composition, and carbon dynamics in an Amazonian rainforest (French Guiana). Oecologia 110, 120–131. doi:10.1007/s004420050140
Bulbeck, D. (2007). Where river meets sea: a parsimonious model for Homo sapiens colonization of the Indian Ocean Rim and Sahul. Curr. Anthropol. 48 (2), 315–321.
Carter, M. L., and Bradbury, M. W. (2016). Oxygen isotope ratios in primate bone carbonate reflect amount of leaves and vertical stratification in the diet. Am. J. Primatology 78, 1086–1097. doi:10.1002/ajp.22432
Cerling, T. E., and Harris, J. M. (1999). Carbon isotope fractionation between diet and bioapatite in ungulate mammals and implications for ecological and paleoecological studies. Oecologia 120, 347–363. doi:10.1007/s004420050868
Cerling, T. E., Hart, J. A., and Hart, T. B. (2004). Stable isotope ecology in the ituri forest. Oecologia 138, 5–12. doi:10.1007/s00442-003-1375-4
Dela, J. D. (2007). Seasonal food use strategies of Semnopithecus vetulus nestor, at Panadura and Piliyandala, Sri Lanka. Int. J. Primatology 28, 607–626. doi:10.1007/s10764-007-9150-8
Delson, E. (1973). Fossil colobine monkeys of the circum-Mediterranean region and the evolutionary history of the Cercopithecidae (Primates, Mammalia). Ann Arbor, MI: Columbia University.
Deraniyagala, P. E. P. (1965). Some aspects of the fauna of ceylon. J. Ceylon Branch R. Asiatic Soc. G. B. & Irel. 9, 165–219.
Deraniyagala, P. (1938). Some fossil animals from ceylon. Part II. J. Ceylon Branch R. Asiatic Soc. G. B. & Irel. 34, 231–239.
Deraniyagala, S. U. (1992). The prehistory of Sri Lanka: An ecological perspective. Colombo: Department of Archaeological Survey.
Dittus, W. P. (1984). Toque macaque food calls: Semantic communication concerning food distribution in the environment. Anim. Behav. 32, 470–477. doi:10.1016/s0003-3472(84)80283-3
Erdelen, W. (1988). Forest ecosystems and nature conservation in Sri Lanka. Biol. Conserv. 43, 115–135. doi:10.1016/0006-3207(88)90086-9
Gamble, C. (1993). Timewalkers: The prehistory of global colonization. Editor A. Sutton (Cambridge, MA: Harvard University Press).
Geist, V. (1998). Deer of the world: Their evolution, behaviour, and ecology. Mechanicsbarg, PA: Stackpole Books.
Grayson, D. K. (1978). Minimum numbers and sample size in vertebrate faunal analysis. Am. Antiq. 43, 53–65. doi:10.2307/279631
Gunatilleke, I., Gunatilleke, C., and Dilhan, M. (2005). Plant biogeography and conservation of the southwestern hill forests of Sri Lanka. Raffles Bull. Zoology 12, 9–22.
Higham, T. F., Barton, H., Turney, C. S., Barker, G., Ramsey, C. B., and Brock, F. (2009). Radiocarbon dating of charcoal from tropical sequences: Results from the niah great cave, sarawak, and their broader implications. J. Quat. Sci. Publ. Quat. Res. Assoc. 24, 189–197. doi:10.1002/jqs.1197
Hillson, S. (2016). Mammal bones and teeth: An introductory guide to methods of identification. New York, NY: Routledge.
Hladik, C. M. (1977). “A comparative study of two sympatric species of leaf monkeys: Presbytis entellus and Presbytis senex,” in Primate ecology: Studies of feeding and ranging behavior in lemurs, monkeys and apes. Editor T. H. Clutton-Brock (London: Academic Press).
Huffman, M. A., Nahallage, C. A., Ananda, T., Kanthilatha, N., Perera, N., Bardi, M., et al. (2022). “The prehistoric nonhuman primate subfossil remains at sigiriya potana cave, Sri Lanka,” in World archaeoprimatology: Interconnections of humans and nonhuman primates in the past. Editors B. Urbani, D. Youlatos, and A. Antczak (Cambridge: Cambridge University Press).
Ingicco, T., Amano, N., Setiagama, K., Moigne, A. M., Budimansémah, A., Simanjuntak, T., et al. (2020). From food to grave good: Nonhuman primate exploitation in early to mid-holocene eastern java (Indonesia). Curr. Anthropol. 61, 264–277. doi:10.1086/708186
Kay, R. F., and Hylander, W. L. (1978). “The dental structure of mammalian folivores with special reference to Primates and Phalangeroidea (Marsupialia),” in The ecology of arboreal folivores. Editor G. G. Montgomery (Washington D. C: Smithsonian Institution Press).
Kay, R. F. (1975). The functional adaptations of primate molar teeth. Am. J. Phys. Anthropol. 43, 195–215. doi:10.1002/ajpa.1330430207
Kennedy, K. A., and Deraniyagala, S. U. (1989). Fossil remains of 28,000-year-old hominids from Sri Lanka. Curr. Anthropol. 30, 394–399. doi:10.1086/203757
Kennedy, K. A., Deraniyagala, S. U., Roertgen, W. J., Chiment, J., and Disotell, T. (1987). Upper pleistocene fossil hominids from Sri Lanka. Am. J. Phys. Anthropol. 72, 441–461. doi:10.1002/ajpa.1330720405
Kennedy, K. A. (2000). God-apes and fossil men: Paleoanthropology of South Asia. Ann Arbor: University of Michigan Press.
Ki-Kydd, K., and Piper, P. J. (2004). Identification of morphological variation in the humeri of Bornean primates and its application to zooarchaeology. Archaeofauna 13, e95.
Kohn, M. J. (1996). Predicting animal δ18O: Accounting for diet and physiological adaptation. Geochimica cosmochimica acta 60, 4811–4829. doi:10.1016/s0016-7037(96)00240-2
Kourampas, N., Simpson, I. A., Perera, N., Deraniyagala, S. U., and Wijeyapala, W. (2009). Rockshelter sedimentation in a dynamic tropical landscape: Late Pleistocene–Early Holocene archaeological deposits in Kitulgala Beli-lena, southwestern Sri Lanka. Geoarchaeology Int. J. 24, 677–714. doi:10.1002/gea.20287
Krigbaum, J., Berger, M. H., Daegling, D. J., and Mcgraw, W. S. (2013). Stable isotope canopy effects for sympatric monkeys at Taï Forest, Côte d'Ivoire. Biol. Lett. 9, 20130466. doi:10.1098/rsbl.2013.0466
Langley, M. C., Amano, N., Wedage, O., Deraniyagala, S., Pathmalal, M., Perera, N., et al. (2020). Bows and arrows and complex symbolic displays 48,000 years ago in the South Asian tropics. Sci. Adv. 6, eaba3831. doi:10.1126/sciadv.aba3831
Langley, M. C. (2016). Osseous projectile weaponry: Towards an understanding of Pleistocene cultural variability. New York, NY: Springer-Verlag.
Laurie, A. (1982). Behavioural ecology of the greater one-horned rhinoceros (Rhinoceros unicornis). J. Zool. 196 (3), 307–341.
Lee-Thorp, J. A., Sealy, J. C., and Van Der Merwe, N. J. (1989). Stable carbon isotope ratio differences between bone collagen and bone apatite, and their relationship to diet. J. Archaeol. Sci. 16, 585–599. doi:10.1016/0305-4403(89)90024-1
Lee-Thorp, J. A., Sponheimer, M., Passey, B. H., De Ruiter, D. J., and Cerling, T. E. (2010). Stable isotopes in fossil hominin tooth enamel suggest a fundamental dietary shift in the Pliocene. Philosophical Trans. R. Soc. B Biol. Sci. 365, 3389–3396. doi:10.1098/rstb.2010.0059
Lee-Thorp, J., Likius, A., Mackaye, H. T., Vignaud, P., Sponheimer, M., and Brunet, M. (2012). Isotopic evidence for an early shift to C4 resources by Pliocene hominins in Chad. Proc. Natl. Acad. Sci. 109, 20369–20372. doi:10.1073/pnas.1204209109
Mellars, P. (2005). The impossible coincidence. A single-species model for the origins of modern human behavior in Europe. Evol. Anthropol. 14 (1), 12–27.
Mellars, P. (2006). Why did modern human populations disperse from Africa ca. 60,000 years ago? A new model. Proc. Natl. Acad. Sci. U.S.A. 103 (25), 9381–9386.
Moe, S. R., and Wegge, P. (1994). Spacing behaviour and habitat use of axis deer (Axis axis) in lowland Nepal. Can. J. Zoology 72, 1735–1744. doi:10.1139/z94-234
Padmalal, U., Takatsuki, S., and Jayasekara, P. (2003). Food habits of sambar Cervus unicolor at the Horton plains national Park, Sri Lanka. Ecol. Res. 18, 775–782. doi:10.1111/j.1440-1703.2003.00595.x
Pearcy, R., and Ehleringer, J. (1984). Comparative ecophysiology of C3 and C4 plants. Plant, Cell. & Environ. 7, 1–13. doi:10.1111/j.1365-3040.1984.tb01194.x
Pederzani, S., and Britton, K. (2019). Oxygen isotopes in bioarchaeology: Principles and applications, challenges and opportunities. Earth-Science Rev. 188, 77–107. doi:10.1016/j.earscirev.2018.11.005
Perera, N., Kourampas, N., Simpson, I. A., Deraniyagala, S. U., Bulbeck, D., Kamminga, J., et al. (2011). People of the ancient rainforest: Late Pleistocene foragers at the Batadomba-lena rockshelter, Sri Lanka. J. Hum. Evol. 61, 254–269. doi:10.1016/j.jhevol.2011.04.001
Perera, N., Roberts, P., and Petraglia, M. (2016). “Bone technology in South Asia from Late Pleistocene rockshelter deposits in Sri Lanka,” in Osseous Projectile Weaponry. Towards an Understanding of Pleistocene Cultural Variability. Editor M. C. Langley (New York, NY: Springer-Verlag), 173–188.
Picin, A., Wedage, O., Blinkhorn, J., Amano, N., Deraniyagala, S., Boivin, N., et al. (2022). Homo sapiens lithic technology and microlithization in the South Asian rainforest at Kitulgala Beli-lena (c. 45–8,000 years ago). Plos one 17, e0273450. doi:10.1371/journal.pone.0273450
Piper, P., Rabett, R., and Kurui, E. B. (2008). Using community, composition and structural variation in Terminal Pleistocene vertebrate assemblages to identify human hunting behaviour at the Niah Caves, Borneo. Bull. Indo-Pacific Prehistory Assoc. 28, 88–98.
Premathilake, R. (2012). Human used upper montane ecosystem in the Horton Plains, central Sri Lanka–a link to Lateglacial and early Holocene climate and environmental changes. Quat. Sci. Rev. 50, 23–42. doi:10.1016/j.quascirev.2012.07.002
Premathilake, R., and Risberg, J. (2003). Late Quaternary climate history of the Horton plains, central Sri Lanka. Quat. Sci. Rev. 22, 1525–1541. doi:10.1016/s0277-3791(03)00128-8
R Core Team (2016). R: A Language and Environment for Statistical Computing, Vienna, Austria. Available at: https://www.R-project.org/.
Roberts, P., Blumenthal, S. A., Dittus, W., Wedage, O., and Lee-Thorp, J. A. (2017a). Stable carbon, oxygen, and nitrogen, isotope analysis of plants from a South Asian tropical forest: Implications for primatology. Am. J. primatology 79, e22656. doi:10.1002/ajp.22656
Roberts, P., Boivin, N., and Petraglia, M. (2015a). The Sri Lankan ‘microlithic’tradition c. 38,000 to 3,000 years ago: Tropical technologies and adaptations of Homo sapiens at the southern edge of Asia. J. World Prehistory 28, 69–112. doi:10.1007/s10963-015-9085-5
Roberts, P., Fernandes, R., Craig, O. E., Larsen, T., Lucquin, A., Swift, J., et al. (2018). Calling all archaeologists: Guidelines for terminology, methodology, data handling, and reporting when undertaking and reviewing stable isotope applications in archaeology. Rapid Commun. Mass Spectrom. 32, 361–372. doi:10.1002/rcm.8044
Roberts, P., Perera, N., Wedage, O., Deraniyagala, S., Perera, J., Eregama, S., et al. (2015b). Direct evidence for human reliance on rainforest resources in late Pleistocene Sri Lanka. Science 347, 1246–1249. doi:10.1126/science.aaa1230
Roberts, P., Perera, N., Wedage, O., Deraniyagala, S., Perera, J., Eregama, S., et al. (2017b). Fruits of the forest: Human stable isotope ecology and rainforest adaptations in late pleistocene and Holocene (∼ 36 to 3 ka) Sri Lanka. J. Hum. Evol. 106, 102–118. doi:10.1016/j.jhevol.2017.01.015
Sponheimer, M., Lee-Thorp, J., De Ruiter, D., Codron, D., Codron, J., Baugh, A. T., et al. (2005). Hominins, sedges, and termites: New carbon isotope data from the sterkfontein valley and kruger national Park. J. Hum. Evol. 48, 301–312. doi:10.1016/j.jhevol.2004.11.008
Suraprasit, K., Bocherens, H., Chaimanee, Y., Panha, S., and Jaeger, J. J. (2018). Late Middle Pleistocene ecology and climate in Northeastern Thailand inferred from the stable isotope analysis of Khok Sung herbivore tooth enamel and the land mammal cenogram. Quat. Sci. Rev. 193, 24–42. doi:10.1016/j.quascirev.2018.06.004
Swindler, D. R. (2022). Primate dentition: An introduction to the teeth of non-human primates. Cambridge: Cambridge University Press.
Tejada, J. V., Flynn, J. J., Antoine, P. O., Pacheco, V., Salas-Gismondi, R., and Cerling, T. E. (2020). Comparative isotope ecology of Western Amazonian rainforest mammals. Proc. Natl. Acad. Sci. 117, 26263–26272. doi:10.1073/pnas.2007440117
Thomas, D. H. (1969). Great Basin hunting patterns: A quantitative method for treating faunal remains. Am. Antiq. 34, 392–401. doi:10.2307/277736
Timmins, R., Kawanishi, K., Giman, B., Lynam, A., Chan, B., Steinmetz, R., et al. (2015). “Rusa unicolor,” in The IUCN Red List of Threatened Species 2015: e.T41790A85628124 (IUCN). Available at: http://dx.doi.org/10.2305/IUCN.UK.2015-2.RLTS.T41790A22156247.en.
Ventresca Miller, A., Fernandes, R., Janzen, A., Nayak, A., Swift, J., Zech, J., et al. (2018). Sampling and pretreatment of tooth enamel carbonate for stable carbon and oxygen isotope analysis. JoVE J. Vis. Exp. 138, e58002. doi:10.3791/58002
Vishvanath, N., Nanayakara, R. P., and Herath, H. (2014). Current status, distribution and conservation of the Sri Lankan hog deer Hyelaphus porcinus (Zimmermann, 1780)(Cetartiodactyla: Cervidae). J. Threat. Taxa 6, 6515–6522. doi:10.11609/jott.o3263.6515-22
Wedage, O., Amano, N., Langley, M. C., Douka, K., Blinkhorn, J., Crowther, A., et al. (2019a). Specialized rainforest hunting by Homo sapiens∼ 45,000 years ago. Nat. Commun. 10, 739–748. doi:10.1038/s41467-019-08623-1
Wedage, O., Picin, A., Blinkhorn, J., Douka, K., Deraniyagala, S., Kourampas, N., et al. (2019b). Microliths in the South asian rainforest∼ 45-4 ka: New insights from fa-hien lena cave, Sri Lanka. Plos One 14, e0222606. doi:10.1371/journal.pone.0222606
Wedage, O., Roberts, P., Faulkner, P., Crowther, A., Douka, K., Picin, A., et al. (2020). Late pleistocene to early-holocene rainforest foraging in Sri Lanka: Multidisciplinary analysis at Kitulgala beli-lena. Quat. Sci. Rev. 231, 106200. doi:10.1016/j.quascirev.2020.106200
Wijeyapala, W. (1997). New light on the prehistory of Sri Lanka in the context of recent investigations of cave sites. Peradeniya: University of Peradeniya.
Wright, B. W., and Willis, M. S. (2012). Relationships between the diet and dentition of Asian leaf monkeys. Am. J. Phys. Anthropol. 148, 262–275. doi:10.1002/ajpa.22081
Keywords: South Asia, Sri Lanka, stable isotope analysis, rainforest, human adaptation, environmental change
Citation: Amano N, Wedage O, Ilgner J, Boivin N, Petraglia M and Roberts P (2023) Of forests and grasslands: human, primate, and ungulate palaeoecology in Late Pleistocene-Holocene Sri Lanka. Front. Earth Sci. 11:1133281. doi: 10.3389/feart.2023.1133281
Received: 28 December 2022; Accepted: 17 April 2023;
Published: 05 May 2023.
Edited by:
Hao Li, Institute of Tibetan Plateau Research (CAS), ChinaCopyright © 2023 Amano, Wedage, Ilgner, Boivin, Petraglia and Roberts. This is an open-access article distributed under the terms of the Creative Commons Attribution License (CC BY). The use, distribution or reproduction in other forums is permitted, provided the original author(s) and the copyright owner(s) are credited and that the original publication in this journal is cited, in accordance with accepted academic practice. No use, distribution or reproduction is permitted which does not comply with these terms.
*Correspondence: Noel Amano, YW1hbm9Ac2hoLm1wZy5kZQ==; Patrick Roberts, cm9iZXJ0c0BzaGgubXBnLmRl
Disclaimer: All claims expressed in this article are solely those of the authors and do not necessarily represent those of their affiliated organizations, or those of the publisher, the editors and the reviewers. Any product that may be evaluated in this article or claim that may be made by its manufacturer is not guaranteed or endorsed by the publisher.
Research integrity at Frontiers
Learn more about the work of our research integrity team to safeguard the quality of each article we publish.