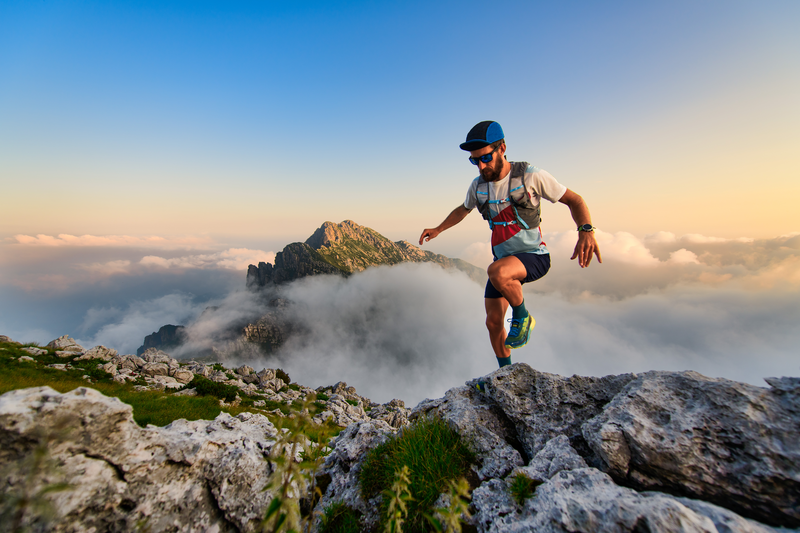
94% of researchers rate our articles as excellent or good
Learn more about the work of our research integrity team to safeguard the quality of each article we publish.
Find out more
EDITORIAL article
Front. Earth Sci. , 19 January 2023
Sec. Cryospheric Sciences
Volume 11 - 2023 | https://doi.org/10.3389/feart.2023.1129795
This article is part of the Research Topic Glaciation and Climate Change in the Andean Cordillera View all 17 articles
Editorial on the Research Topic
Glaciation and climate change in the Andean Cordillera
The Andean Cordillera runs the length of South America (∼10° S to 56° S) and incorporates a wide variety of topographic and climatic zones, and the largest concentration of glacial ice in the southern hemisphere outside of Antarctica. The Andean Cordillera is cryospherically diverse, ranging from high mountain glaciation in the arid north, to large temperate icefields in the humid south. Recent work has indicated that Andean glaciers are among the fastest changing on Earth, posing a threat for regional water security and power generation, ecosystem stability, the prevalence of geohazards, and a potentially substantial contribution to global sea level rise. The region is also subject to changing patterns of large-scale ocean-atmosphere systems, such as the sub-decadal El Niño Southern Oscillation and the Southern Annular Mode, with uncertain future impacts on glaciated basins. Studies on the climate and cryosphere of the Andean Cordillera, both past and present, are thus needed to better understand the sensitivity of these systems to future change.
Despite growing interest, assessments of cryosphere and climate change are currently too limited (both spatially and temporally) to constrain past or future ice mass variability with a high degree of confidence across this diverse mountain range. Data-model comparisons are also rare, which limit the ability to establish the regional drivers (e.g., climatic, topographic) of glacier change. With this Research Topic, we draw together knowledge from the fields of climatology, glaciology, and geomorphology, as well as other disciplines, to build towards an improved understanding of the variability in, and interactions between, the climate-cryosphere system across the Andean Cordillera, and to promote new links between researchers working in this area.
This Research Topic brings together 16 articles from a diverse and multinational authorship that includes researchers from Chile, Argentina, Germany, France, the United States, Ireland, Austria, Switzerland, Canada, Belgium, Germany and Italy, with multiple examples of international collaborations, including between European, North American and South American countries. We are also pleased that at least 50% of the publications in this Research Topic were led by early career researchers. However, only three of the 16 publications were led by female scientists, and several had entirely male co-author lists. We strongly believe that more work needs to be done to improve the gender balance of research in this field, and we encourage authors to reflect on this, and to seek gender balance in future publications.
The articles in this Research Topic generally fall into three broad categories; 1) modern day glaciology and climate; 2) palaeoglaciology and glacier reconstruction; and 3) ecological and environmental reconstruction. The articles herein represent a range of disciplines and include state of the art chronological and modelling methods, such as machine learning approaches to understanding glacier behaviour and their potential responses to future climate change. Many articles also make use of traditional and essential approaches, including glacial and geomorphological mapping, geodetic mass balance analyses, chronological methods, and numerical simulations of glacier behaviour. The articles within this Research Topic refine and build on the works of pioneering early researchers who studied past ice sheet fluctuations, including Nordenskjold (1899), Caldenius (1932) and Lliboutry (1956), and expand into new areas where little or no research has been carried out. Geographically, the articles span almost the full extent of the Andean chain, from tropical Peru in the low latitudes to Tierra del Fuego in the high latitudes.
Modern-day glaciological and climate studies are well-represented (approximately one-third of the articles) in this issue. The research encompasses state-of the-art approaches to determining glacier mass balance, glacier dynamics, and sensitivity to climate. The manuscripts focus on areas where data are sparse, and address important problems to understand better Andean cryology. The papers have glaciers playing active (in terms of hydrology, hazards) and passive roles (as a paleoclimate proxy) in Andean (paleo)environments.
In the north of the Andes, Taylor et al. focus on the tropical glaciers of the southern Peruvian Andes, including the Cordilleras Vilcanota, Vilcabamba, and Urubamba. Using Landsat and ASTER archives, they document ice losses of >50% since the 1970s. These glaciated mountains provide the primary water resource for local populations and larger urban areas, such as the Cusco region. Taylor et al. suggest that recent and future changes will lead to increased hazards on destabilizing slopes, in addition to increased pressure on fresh water availability.
Staying in Peru, dos Reis et al. investigate dust concentration in an ice core from the Quelccaya Ice Cap to assess seasonality and climate (wet/dry conditions), including the Pacific Ocean influence and regional dust transport. They observed an association between snow accumulation and Quelccaya dust variability, and suspended sediment load and runoff in the Madeira River system, the largest contributor to the Amazon River. dos Reis et al. infer linkages between regional atmospheric patterns and climates, tropical ice core records and snow accumulation, and ultimately larger Amazon basin rivers.
Further south, in Patagonia (47°S), Martin et al. used the state-of-the-art Parallel Ice Sheet Model to study current and past glacier-climates around the Monte San Lorenzo massif, an ice cap east of the Patagonian Icefields. They quantified present-day physical glacier properties, ice dynamics and surface mass balance. Then, they quantified the paleoclimatic ranges of temperature and precipitation that may have caused glacier advances during late glacial and Holocene times, likely caused by shifts in the westerlies and associated air masses. Martin et al. infer the sensitivity of present-day and past ice masses in Patagonia to climatic and model parameters.
McDonnell et al. use declassified Hexagon imagery and remotely-sensed data to estimate mass balance for subsets of the Northern and Southern Patagonian Icefields, since the 1970s. They found an acceleration of ice loss by a factor of 1.2–2.4 for the icefields since 2000, with lake-terminating glaciers showing the most significant increase in mass loss rate. Spatial heterogeneity in geodetic mass balance trends for glaciers of all terminus environments suggests that sensitivity to climate change is dependent on a multitude of morphological and climatological factors.
Moving to the Southern Patagonian Icefield, Irarrazaval et al. integrate geomorphological and ice-dynamic information to assess proglacial lake development adjacent to Exploradores Glacier. Using field based (including uncrewed aerial vehicles) and satellite imagery to study 20 years of history, they improved understanding of glacier lake dynamics and development. Such efforts are essential to incorporate the influence of lakes in numerical models, which may inform glacier response to future climate scenarios, and understand hazards such as outburst floods.
Taking a broader perspective across the Andean Cordillera, Caro et al. use a machine learning approach to study the role of climatic and morphometric variables (e.g., elevation and aspect, or glacier surface area and slope) on the variance of glacier surface area between 8°S and 55°S. Focusing on the period 1980–2019, they show that spatial variability in climate is the leading driver of spatial variance in glacier changes, and go on to evaluate the relative roles of precipitation and temperature on glacier changes from the outer Tropics and Dry Andes to the wet southern Andes, respectively. They also documented 12 glaciological zones based on clustering analysis, which considers different seasonal climate and the morphometric characteristics of glaciers. Such efforts will be important for regional hydro-glaciological simulations along the Andes.
Six articles in this Research Topic tackle the task of reconstructing former glaciers. A high latitudinal range is represented, ranging from northern Peru (Lee et al.), northern Chile (Aguilar et al.), central Chile (Price et al.), northern and central Patagonia (Leger et al.; Sagredo et al.), through to southern Patagonia (Lira et al.). This group of publications applies remote sensing and field surveys of glacial geomorphology, in some cases together with cosmogenic nuclide dating, to reconstruct glacier behaviour during the Last Glacial Maximum, Late Glacial and Holocene. These papers provide therefore an important contribution to understanding how the timing and climatic drivers of glacial advances and periods of rapid recession vary along this enormous latitudinal gradient, shedding light into changes in large scale atmospheric and oceanic circulation systems, and the likely reach of climatic features such as the Antarctic Cold Reversal, Southern Westerly Winds and the Southern Annular Mode.
Starting farthest north, Lee et al. analyse tropical mountain glaciers in the northern highlands of Peru (5°S), an area presently without glaciers. The authors characterise the glacial geomorphology, mapping cirques, moraines and ice-sculpted bedrock. The authors assume that the moraines were deposited during the local Last Glacial Maximum (21.2–23.5 ka). They reconstructed 29 glaciers using these geomorphological data, and reconstructed ice-surface contours and the equilibrium line altitude (ELA) for these palaeoglaciers, following the method of Pellitero et al. (2015).
In the southern Atacama Desert of Chile, Aguilar et al. mapped glacial landforms in the Encierro River Valley (29.1°S). They used these data and new 10Be exposure ages to reconstruct the extent and timing of palaeoglaciation. The outermost moraines yielded an exposure age of approximately 40 ka. Inboard of this, moraine arcs yielded exposure ages of 25–33 ka (the local Last Glacial Maximum). The glacier had receded from the valley before 18 ka. Higher up-valley, a smaller readvance was recorded at 17–20 ka, coincident with Heinrich Stadial Event 1. A main implication of this 10Be moraine record is that no major late-glacial advance occurred after the c.18 ka deglaciation of this valley that is located in the tropic to extra-tropic geographic transition.
Focusing on the Holocene, Price et al. use novel 36Cl exposure ages on basaltic boulders, dendrochronology, and glacier mass balance modelling to constrain Late Holocene glacial fluctuations at Monte Sierra Nevada (38°S), in the Central-South Chilean Andes. The outermost moraine in the valley yielded an exposure age of 4.2 ka. A series of moraines further up-valley yielded ages from 2.5 to 0.8 ka, suggesting multiple readvances of the glacier during this period. A large readvance resulted in the glacier re-occupying the outermost moraine at 0.7 ka. The glacier then formed a series of nested moraines dating 0.45 to 0.30 ka, with pulsed ice recession during the latest Holocene. Glacier modelling suggests that the conditions were around 1.5°C colder and 20% wetter during the Holocene maximum neoglaciations, relative to modern.
Moving to northeast Patagonia, Leger et al. present geomorphological mapping, terrestrial cosmogenic nuclide exposure dating and glacial numerical modelling to reconstruct the late-Last Glacial Maximum behaviour of two mountain glaciers at 43°S. In both these valleys, Leger et al. find evidence for glacial readvances that occurred after the onset of main deglaciation, with the readvances centred at 18.0 ± 1.15 ka, coincident with readvances observed further north, in the Encierro River Valley (Aguilar et al.). A numerical model, forced to match these Late Glacial moraine extents, suggests that annual temperatures were 1.9°C–2.8°C cooler than today, with precipitation 50%–380% higher. This implicates an equatorward migration of the precipitation-bearing Southern Westerly Winds at this time (19.5–18 ka), bringing increased precipitation and higher humidity to the northern Patagonian Ice Sheet before widespread deglaciation across these mountains.
At Monte San Lorenzo (47°S), Sagredo et al. analyse the Holocene fluctuations of Río Tranquillo Glacier. They apply 24 new 10Be ages, together with published ages, to constrain the dynamics of this glacier. Sagredo et al. find that the glacier approached its maximum Holocene extent, possibly several times, between 9.8 and 6.7 ka. This was followed by smaller readvances at ∼5.4, ∼4.3, ∼3.5, ∼1.4 ka, with Late Holocene readvances at about 670, 430, and 390 years ago. The pattern of multiple moraine-building events, with slightly decreasing extent each time, is mirrored in other parts of the Southern Hemisphere, and is starkly different to the pattern of Holocene glacier behaviour in the Northern Hemisphere. The authors suggest that the difference in Holocene glacier behaviour between the Northern and Southern hemispheres (e.g., the difference between Patagonia and the European Alps) may be due to natural forcing that has now been overwhelmed by anthropogenic greenhouse gas-driven warming. In contrast, now, interhemispheric synchronous retreat seems unprecedented during the Holocene.
In southern Patagonia, Lira et al. attempts to tackle the timing of glacial advances for Seno Skyring Lobe (52°S), one of the few main ice lobes draining the former Patagonian Ice Sheet whose glacial history had remained mostly undocumented until now. Two outer Laguna Blanca moraines yielded mean 10Be exposure ages of 26.3 ± 2.3 ka and 24.3 ± 0.9 ka. Inboard of these, the inner Río Verde moraines yielded mean ages of 18.7 ± 1.5 ka, with deglaciation from this moraine already underway by 16.4 cal yr BP. The authors also used 10Be exposure ages and optically stimulated luminescence dating to constrain lake levels for the palaeolake Laguna Blanca, revealing a drainage event at 22 ± 3 ka. The work by Lira et al. exposes that none of the main southernmost Patagonian ice lobes embrace the complete record of former ice fluctuations, but altogether they uncover full glacial conditions at different times during the last glacial period, including the Marine Isotope Stage (MIS) 4, 3 and 2.
Four contributions in this Research Topic illustrate how palaeoecological methods and associated geochronology can be used to understand better the changes in Late glacial and Holocene glacial environments of the Andean Cordillera.
The northernmost contribution from Mayta and Maldonado describes the climate–vegetation dynamics of the last 4,500 years in the high subtropical Andes of Chile (30°S). Using fossil pollen and macroscopic carbon obtained from sediment cores from two lakes they recognise three contrasting periods in the paleoclimate. From the beginning of the sequences until ∼1,900 cal yr BP, relatively dry climatic conditions are suggested, with a slight trend toward more humid conditions after 2,700 cal yr BP. Pollen records from ∼1,900 to ∼600 cal yr BP suggest wetter conditions than today. Finally, relatively arid conditions have reappeared in the last ∼600 years.
Maldonado et al. present the first pollen and charcoal records from the Lago Cochrane/Pueyrredón valley in Patagonia, spanning the last 11,650 cal yrs BP. Using records from two separate sites at Laguna Maldonado and Laguna Anónima they reconstruct changes in Holocene vegetation dynamics in the period after the drainage of the proglacial lake associated with the Lago Cochrane/Pueyrredón ice lobe. These records show the progression from open forest and scattered patches of forest of variable size in a steppe matrix, through to a closed Nothofagus forest. Their records indicate that after 3,800 cal yrs BP, a highly dynamic open forest or forest patches scattered in a grass/shrub–grass steppe matrix developed around Laguna Anónima. There was a higher fire occurrence synchronous with the presence of hunter-gatherers during the last 3,000 years.
Vásquez et al. also worked at Lago Cochrane/Pueyrredón. They study the spatial and temporal evolution and associated palaeoenvironments of the large ice-dammed lakes that developed along the eastern margin of the Patagonian Ice Sheet during the Last Glacial Termination. Using the distribution and age of shoreline features associated with Glacial Lake Cochrane, they identified five clusters of lake terraces, shorelines, and deltas between elevations ∼600–500 (N5), ∼470–400 (N4), ∼360–300 (N3), ∼230–220 (N2), and ∼180–170 m asl (N1) in the basin. A chronology, based principally on radiocarbon dates from lake sediment cores and new OSL dating, suggests that these phases developed between ∼20.7–19.3 ka (N5), ∼19.3–14.8 ka (N4), ∼14.8–11.3 ka (N3), and shortly thereafter (N2 and N1). They were also able to relate phases of lake stabilization to former temperature and precipitation regimes.
In Southern Patagonia, Roberts et al. undertook multi-proxy analyses on two sediment cores from Lago Pato in Torres del Paine to provide insights into glacier dynamics and paleolake-level change in the Última Esperanza region over the last ∼30,000 cal a BP (30 ka). The results show that a deep ice-dammed and enlarged paleolake developed ∼30–20 ka after the ice had retreated from local-Last Glacial Maximum limits at c. 48–34 ka and during the build-up to the global-Last Glacial Maximum, ∼26–19 ka. Gaps in the sediment records between ∼20–13.4 ka and ∼20–10 ka suggest hiatuses in sediment accumulation during the global-Last Glacial Maximum and Antarctic Cold Reversal readvances and/or removal by lake lowering or flushing during the Late Glacial–early Holocene. They relate these environmental changes to millennial-scale changes in the precipitation-bearing Southern Hemisphere Westerly Winds.
The 16 articles in this Research Topic provide an exciting insight into how the dynamics of glaciers and climate vary across the diverse Andean Cordillera, both at present and in the past. The articles consider glaciers as taking both active (e.g., hydrology and hazards) and passive roles (e.g., as a paleoclimate proxy) in their Andean environments. These new data are enabling a better understanding of the (a)synchronicity of the timing of glacial advances, and how this can be driven by wind systems (such as the Southern Westerly Winds) or large-scale climate patterns. The research collected in this topic also addresses fundamental problems in the timing of the end of the last glaciation, the structure of the last glacial to interglacial transition, and the patterns of Holocene climate and glacier dynamics. These insights into past climates and past glacier behaviour is critical to help understand how glaciers are behaving today, and how they will behave in the future.
All authors listed have made a substantial, direct, and intellectual contribution to the work and approved it for publication.
The authors declare that the research was conducted in the absence of any commercial or financial relationships that could be construed as a potential conflict of interest.
All claims expressed in this article are solely those of the authors and do not necessarily represent those of their affiliated organizations, or those of the publisher, the editors and the reviewers. Any product that may be evaluated in this article, or claim that may be made by its manufacturer, is not guaranteed or endorsed by the publisher.
Caldenius, C. C. Z. (1932). Las glaciaciones cuaternarias en la Patagonia y Tierra del Fuego: Una investigación regional, estratigráfica y geocronológica.—una comparación con la escala geocronológica sueca. Geografiska Annaler. 14 (1-2), 1–164. doi:10.1080/20014422.1932.11880545
Lliboutry, L. (1956). Nieves y glaciares de Chile Fundamentos de glaciologia. Santiago, Chile: Universidad de Chile, 471.
Nordenskiöld, E. (1899). Om Skifferbitar, som träffats flytande på hafsytan i sydvestra Patagonien. Geologiska Föreningen i Stockholm Förhandlingar. 21 (5), 536–538. doi:10.1080/11035899909442246
Keywords: andes (South America), quaternary, cosmogenic, glaciology, ecology, PALAEO, palaeoclimate
Citation: Davies B, Bendle J, Glasser N, García J-L and Kaplan M (2023) Editorial: Glaciation and climate change in the Andean Cordillera. Front. Earth Sci. 11:1129795. doi: 10.3389/feart.2023.1129795
Received: 22 December 2022; Accepted: 11 January 2023;
Published: 19 January 2023.
Edited and reviewed by:
Michael Lehning, Swiss Federal Institute of Technology Lausanne, SwitzerlandCopyright © 2023 Davies, Bendle, Glasser, García and Kaplan. This is an open-access article distributed under the terms of the Creative Commons Attribution License (CC BY). The use, distribution or reproduction in other forums is permitted, provided the original author(s) and the copyright owner(s) are credited and that the original publication in this journal is cited, in accordance with accepted academic practice. No use, distribution or reproduction is permitted which does not comply with these terms.
*Correspondence: Bethan Davies, YmV0aGFuLmRhdmllc0BuZXdjYXN0bGUuYWMudWs=
Disclaimer: All claims expressed in this article are solely those of the authors and do not necessarily represent those of their affiliated organizations, or those of the publisher, the editors and the reviewers. Any product that may be evaluated in this article or claim that may be made by its manufacturer is not guaranteed or endorsed by the publisher.
Research integrity at Frontiers
Learn more about the work of our research integrity team to safeguard the quality of each article we publish.