- 1Key Laboratory of Tibetan Plateau Land Surface Processes and Ecological Conservation (Ministry of Education), College of Geographical Science, Qinghai Normal University, Xining, China
- 2Qinghai Province Key Laboratory of Physical Geography and Environmental Process, College of Geographical Science, Qinghai Normal University, Xining, China
- 3School of Finance and Economics, Qinghai University, Xining, China
Human activities strongly influenced the present-day environment of the Tibetan Plateau, especially in the northeastern area. The questions over when and to what extent humans began to utilize the plateau environment have been long investigated, but there are still gaps in our understanding, such as the relationship between forest dynamics and anthropogenic activities. Here, we simulate the potential Holocene geographical distribution of the three dominant coniferous species in the Northeast Tibetan Plateau in response to climate/environment and analyze pollen records and multi-proxies for anthropogenic activities to explore human impact on natural forest dynamics. Simulation results show marked expansion of Picea and Pinus coniferous forests in the Early Holocene (11.5–8.3 ka BP), continuing into the Mid Holocene (8.3–4.0 ka BP). However, there was a slight contraction of forest in the Late Holocene (4.0–2.6 ka BP) in the western part of the region, and near disappearance in the eastern Qaidam Basin, although both Pinus and Picea slightly increased in Hehuang Valley. Pollen analysis confirms the patterns, with an increase in arboreal pollen mainly comprising Pinus and Picea from Early to Early Mid Holocene (11.5–5.3 ka BP), followed by a decrease. Proxies of anthropogenic activities, including carbon concentration, archeological sites, and, synanthropic plants, increased significantly after 5.3 ka BP, with archeological evidence for intensive exploitation of forest and turnover of natural vegetation. We argue that forest retreat at 5.3–4.0 ka BP was due to the expansion of cropland and increasing demand for wood. Significant coniferous forest retreat and degradation from 4.0 to 2.6 ka BP, with forest succession in Hehuang Valley, was driven by the booming population, extensive grazing, and forest exploitation; the drying cooling climate may have aggravated the pace of deforestation at higher elevations. This study presents new insights into the deforestation on the Northeast Tibetan Plateau in the Holocene.
Introduction
A capacity to alter the natural environment is one of the hallmarks of human civilization, and the process of human development and adaptation to the environment is one of modifying the natural environment and rearranging the natural biota (McNeill, 1986; Ellis and Ramankutty, 2008; Harari, 2015; Boivin et al., 2016; Goldewijk et al., 2017; Woodbridge et al., 2020). This mostly has been true since the Holocene, with an increased rate of species turnover (Mottl et al., 2021), rapid extinction of wildlife (Pimm et al., 2014; Teng et al., 2022), and alteration of the terrestrial vegetation (Pongratz et al., 2008; Qin et al., 2010; Yu et al., 2010). Consequently, the composition and carbon sequestration of the biosphere has been profoundly influenced by the legacies of human activities in the past few thousand years (Broecker and Stocker, 2006; Olofsson and Hicker, 2007; Zhang et al., 2012; McMichael, 2021). Natural forests are essential in carbon cycling, biodiversity conservation, and world climate, organic matter production, water conservation, and soil erosion prevention (Foley and Kutzbach, 1994; IGBP, 1994; Whitney, 1994; Vitousek et al., 1997; Wan, 2017; Tuo et al., 2020). Forest degradation was one of the most apparent land cover changes through the Holocene (McNeill, 1986; Ren, 2000; Kaplan et al., 2009; Kaal et al., 2011; Hou et al., 2013a; Cheng et al., 2018), primarily due to cultivation and grazing, and in some areas may attributing to use of fire for hunting (Ren, 2000; Zhou, 2003; Kirch, 2005; Steffen et al., 2007; Hou et al., 2012; Hou et al., 2013a; Leal et al., 2019; Castilla-Beltrán et al., 2021; Miehe et al., 2021). In the mid and lower reaches of the Yangtze River, anthropogenic disturbance induced deforestation started since Mid Holocene (Ren, 2000; Hou et al., 2012). In Longdong Basin, anthropogenic activities, predominantly agriculture production, caused a decrease in the abundance of shrubs and grassland. In river valleys, the original vegetation has turned into coniferous and broadleaf mixed forests (Zhou et al., 2011). Owing to the human disturbance, the coniferous and broadleaf mixed forests cover decreased markedly after 4.6 cal ka BP in the southeast of Gansu (Li et al., 2012). However, the ways humans may have affected the natural forest on the Tibetan Plateau yet remains to be further studied.
Termed the third pole of the Earth, the Tibetan Plateau is one of the least populated regions in the world. The harsh environment of hypoxia, high altitude, and low temperature, as well as scarce resources have largely constrained mass settlement of people in the area even at the present day (Hou, 2016; Liu et al., 2018; Feng and Li, 2020). Thus there is a knowledge gap in understanding the anthropogenic impact on terrestrial vegetation and especially on the role of humans in natural forest dynamics. The ananlysis of fossile pollen records illustrates past vegetation succession, and the Holocene pollen record is generally well-established. However, whether, when, and to what extent human activity influenced spatial and temporal changes in vegetation and forests on the Tibetan Plateau remains unclear. Some studies suggest that substantial anthropogenic modification of Tibetan landscapes began as early as ∼8,000 cal yr BP, with the presence of hunting and possibly grazing and, later, the development of agriculture (Brantingham et al., 2007; Miehe et al., 2014). Supporting evidence includes records of carbon concentration, analysis of wood charcoal, records of grazing related spore pollen and synanthropic plants, expansion of cropland, and human population boom (Miehe et al., 2014; Huang et al., 2017; Miao et al., 2017; Chen F. H et al., 2020; Wei et al., 2020; Wende et al., 2021; Liu et al., 2022). However, other studies argue the plateau, as central Asia’s high-altitude heart, was not occupied by the mass human population until the Late Holocene. Therefore, turnover of terrestrial vegetation, including forest retreat in prehistorical time, was mostly driven by climate change (Herzschuh, 2006; Herzschuh et al., 2010; Kramer et al., 2010; Zhou and Li, 2012). However, charcoal records from soils and archeological evidence illustrated utilization of woods in the areas where they believe to be disappeared. More over, the persistence of isolated trees above the current tree line lead to continued speculation of widespread early human-induced forest clearance.
The human footprint on the Tibetan Plateau has steadily grown since the Epipleistocene and followed by the amelioration of climate after the Late Glacial Maximum (LGM), hunters begin to alter the landscape for food, energy, and other resources (Brantingham et al., 2007; 2013; Rhode et al., 2007; Miehe et al., 2014; Miehe et al., 2021). These alterations were especially pronounced on the Northeast Tibetan Plateau, which was at a relatively lower elevation, and occupied by both herders and farmers. Moreover, the area is one of the regions where there was intensive interaction between plateau people and farming communities from lowland China. In this region, use of fire for wild hunting started as early as 8.0 ka BP, when hunters and gatherers made their first sustained use of the plateau (Brantingham et al., 2007; Brantingham et al., 2013). Low intensity but extensive agriculture began at 5 ka BP during the Majiayao Culture, and intensive agricultural land use expanded around 4.3 ka BP during the late Majiayao Culture (Wende et al., 2021). Evidence shows mass grazing began around 3.6 ka BP and grassland degradation around 3.5 ka BP (Huang et al., 2017). As such, the studies on the human dimensions of environmental change are vitally important to explain the current environmental conditions, and to understand and interpret past human-land interactions.
Here, we investigate changes in the distribution of natural coniferous forests in the Holocene on the Northeast Tibetan Plateau and its relationship with human activities. Our objectives are twofold: 1) to map the potential distribution area of the two dominant families of coniferous forest, Picea and Pinus; and 2) to analyze the anthropogenic role in the dynamic change of forest during the Holocene. We use species distribution modeling (SDM) to simulate the fundamental niche of Picea and Pinus, and to map its potential geographic extent through the Holocene. The modeling results are then compared to evidence from archeological sites, carbon concentration, and fossil pollen records to assess actual and potential forest distribution and the role of human disturbance.
Scope of the study
Environmental setting
This study focuses on the northeastern Tibetan Plateau (Figure 1), including the eastern part of the Qinghai Plateau and the southern part of the Gannan Plateau (He et al., 2005), including geographical units of Hehuang Valley, Qinghai Lake area, and Gonghe Basin, between 99°19′–103°12′E and 35°32′–37°47′N, at an elevation of 1700–5,200 m with a west to east decrease in terrain elevations and bounded by the Qilian Mountains in the north and the Loess Plateau in the east. It is at the intersection of the Tibetan alpine region, the northwest arid region, and the eastern monsoon region, which makes it highly sensitive to climate change due to the interaction between the plateau monsoon, the westerly circulation, and the East Asia summer Monsoon.
Hehuang Valley is situated at the eastern margin of the Qinghai Plateau, with a mean annual temperature range of 2.2°C–9.0°C, a mean annual precipitation range of 252–535 mm, and forest-grassland vegetation (Jia et al., 2019). Forests are mainly coniferous, comprising Picea and Pinus; and mixed coniferous and broadleaf, comprising Poplar, Betula, and Salix. Poplar and Salix are mainly secondary forest species (CFEC, 1997). The region is also the most suitable for agricultural production in the Northeast Tibetan Plateau. The Qinghai Lake-Gonghe Basin has an average elevation of over 3,000 m and a transitional climate between temperate and sub-frigid, characterized by cold and dry winters, cool and humid summers; mean annual temperature ranges from −0.8°C–0.6°C and mean annual precipitation ranges from 324 to 412 mm. The mode of production is semi-pastrolism, and vegetation includes temperate grassland, alpine meadow, and swamp meadow. The surrounding mountains are mostly covered with alpine shrubs and coniferous forests (Chen and Peng, 1993). Drainage areas of the Yellow River at altitudes below 3,600 m, even in the Gonghe Basin, are occupied by forest-shrubs vegetation (Wu and Wu, 2016).
Cultural sequence
This study focuses on the period from 11.5 to 2.6 ka BP, encompassing the Early Holocene (EH) from 11.5 to 8.3 ka BP, the Mid Holocene (MH) from 8.3 to 4.2 ka BP, and the Late Holocene (LH) from 4.2 to 2.6 ka BP. Early hunter-gatherers were active between 8.3 and 5.3 ka BP, which is referred to as Early Mid Holocene (EMH). The Majiayao Culture was formed between 5.3 and 4.0 ka BP, referred to as Late Mid Holocene (LMH), during this period millet agriculture and pottery production were quite common in the Northeast Tibetan Plateau. In fact, the Majiayao Culture was known for its delicacy in pottery. Three different cultures characterized the LH, the Qijia Culture (4.2–3.6 ka BP), Kayue Culture (3.6–2.7 ka BP), and Xindian Culture (3.6–2.6 ka BP). The Qijia Culture was mainly characterized by agriculture and bronze production. The Xindian and Kayue Cultures occupied this region during the same period, while Xindian Culture mostly lived on farming and partially practiced grazing, the Kayue Culture mainly lived on grazing and practiced agriculture as an alternative source of livelihood.
Materials and methods
Species presence data
Due to the high altitude and harsh environment, coniferous forest, comprising Picea crassifolia. Kom. (hereafter referred to as Picea), and Pinus armandii Franch. and Pinus tabulaeformis Carr. (hereafter referred to as Pinus) accounts for 64.2% of the forested areas on the Northeast Tibetan Plateau (CFEC, 1997; Zhang, 2004; Tang et al., 2019). Thus, the geographical distribution of Picea and Pinus should provide a good indicator of forested land in the Northeast Tibetan Plateau. Both species are cold resistant and tolerate to barren soil conditions, with Picea preferring a cool and humid environment, and Pinus a slightly warmer climate.
In this study, we collated 131 presence localities for Picea and 235 for Pinus, mostly from field research in 2021–2022, and some from the literature and online search engines including the Global Biodiversity Information Facility (http://www.gbif.cn), the Chinese Vitual Herbarium (http://www.cvh.ac.cn), the Teaching Specimen Resource Net (http://mnh.scu.edu.cn), National Specimen Information Infrastructure (www.nsii.org.cn). Samples with unclear localities and artificially planted records were removed. The coordinates of the geographic position of the sampling places were obtained through Baidu coordinate pickup system and the accuracy of the information was checked by Google satellite map. To minimize overfitting of the modeling result and spatial auto-correlation caused by sampling bias, the occurrence data were trimmed using EMtools software so that only a single presence was retained in each 2.5x2.5 km grid cell. This gave a final total of 97 Picea presence samples and 80 Pinus samples (Figure 2).
Climate data
Climate data for the study were obtained from the World Palaeoclimate Database (www.paleoclim.org), focusing on the periods: the LGM (21 ka BP), EH (11.7–8.3 ka BP), MH (8.3–4.2 ka BP), LH (4.2–0.3 ka BP), and present day (1979–2013 AD). The database uses observations from over 4,000 global sites and Shuttle Radar Topography Mission (STRM) 90–30 topography data. Climate data extracted for each period (excluding the LGM) included 19 biological climatic variables (Bios) that were obtained using the thin plate smooth spline interpolation method integrated with ANUSPLIN software (Hijmans et al., 2005). Climate data for the LGM is based on the implementation of the CHELSA algorithm on PMIP3 data (Karger et al., 2021); this is a well-established ocean-atmosphere coupled climate model that has contributed to the last three Intergovernmental Panel on Climate Change (IPCC) Assessment Reports and has been used to simulate climate for nearly 20 years (Fordham et al., 2017).
Climate data were first limited to the boundary of the Tibetan Plateau (Zhang N. M et al., 2021) and converted into ASC format to obtain the climatic layer for each period. As there is multi-collinearity between the 19 Bios, applying all Bios in the calculations may result in overfitting, causing the simulation result to be limited around the presence localities, affecting model accuracy and predictive ability (Warren and Seifert, 2011). To address this, Pearson’s Correlation was used to analyze the correlation between the 19 Bios; any pairs with R≥0.80 were considered closely correlated (Kumar and Stohlgren, 2009; Lozier and Mills, 2011). Also, a preliminary SDM was performed using the 19 Bios and the importance of each variable to the model was evaluated using the percent contribution and Jacknife result (Ficetola, 2007). For closely correlated variables, the one that provides the most unique information and the highest contribution to the model was selected and the others were excluded. The model was then rerun using the selected set of variables in a stepwise fashion until all the required variables were determined (Table 1; Table 2).
Model calibration
SDMs have been applied in diverse disciplines, yet overfitting or over parameterize is a major concern, that may lead to severe bias in modeling. In this study we used maximum entropy modeling (MaxEnt) of species geographic distribution (Phillips et al., 2006). We selected MaxEnt over other types of SDM due to its high performance (Elith et al., 2006; McPherson and Jetz, 2007) and extensive use in previous studies, which means there is a robust understanding of its strengths and weaknesses (Elith and Graham, 2009; Phillips et al., 2009; VanDerWal et al., 2009; Veloz 2009; Elith et al., 2011). In address to model weaknesses, parameter optimization is essential for model calibration. Feature types and the regularization multiplier are the two crucial parameters that need to be considered. Feature types correspond to environmental variables; these are mathematically transformed so that MaxEnt can use complex mathematical relationships to predict the response of species to various environmental factors. The regularization multiplier is a constraint added to the model based on element parameters that adjusts the response curve of the simulation (Phillips et al., 2009). Here, we used Kuenm, an R package for model calibration. Kuenm selects the best parameters for modeling based on: 1) statistical significance; 2) predictive ability; and 3) model complexity (Cobos et al., 2019). In detail, we used 15 feature types (L, Q, P, H, LQ, LP, LH, QP, QH, PH, LQP, LQH, LPH, QPH, LQPH), 40 regularization multipliers (at intervals of 0.1), giving a total of 600 combinations. Optimal parameters were selected based on omission rates below the statistical significance threshold and delta akaike information criterion (AIC) up to 2 (Warren and Seifert, 2011). AIC is a mathematical method for evaluating how well a model fits the data it was generated from. The best-fit model according to AIC is the one that explains the greatest amount of variation using the fewest possible independent variables. Finally, a combination of feature type and regularization multiplier with AIC of 0 was determined to be the optimal parameter (Milanovich et al., 2012; Zhu et al., 2014).
MaxEnt modeling was performed using the optimized parameters, with 70% of the presence data set as training data for model prediction and 30% used for testing. To reduce the uncertainty of prediction, the process was replicated 10 times, and to avoid model transition pooling, the maximum number of iterations was set to 5,000. The modeling was applied to simulate species distribution for the four periods of interest (LGM, EH, MH, and LH).
Pollen and anthropogenic activity proxies
Nine sites on the Northeast Tibetan Plateau with good palynological data were selected for arboreal pollen analysis, two from Hehuang Valley, namely, Changning site (Dong et al., 2012) and Dashuitang site (Cao et al., 2021), three from Gonghe Basin, namely, Genggahai (Liu, 2016), Dalianhai (Cheng et al., 2010), and Ke section (Miao et al., 2017), three from Qinghai Lake drainage area, namely, Qinghai Lake (Liu et al., 2002), Langgeri (Wei et al., 2020), and YWY(Zhang Y. L et al., 2021), and one along the Qilian Mountains, namely, Luanhaizi (Cao et al., 2021). The data on total amount of arboreal pollen and coniferous trees were extracted from all nine sites to analyze dynamic changes in forest extent and location for comparison with the simulated distributions. The data on carbon concentration was extracted from four sites in Northeast Tibetan Plateau, namely, Nankayan Site (Qi, 2022), Jiangxigou (Jiang et al., 2015) Ke section (Miao et al., 2017), and Langgeri (Wei et al., 2020), along with the synanthropic plants (Chen X. L et al., 2020), number of sites on the Northeast Tibetan Plateau, as indicators of anthropogenic activities in the area. In order to eliminate statistical magnitude variance due to the different units of the collected data, the minimum-maximum normalization method was used in calculations. Finally, archeological evidence for the different periods and cultures on the Northeast Tibetan Plateau was analyzed to further explore the human activities and forest changes.
Results
Geographic distribution of Picea and Pinus since the Late Glacial Maximum
The relative probability of species distribution is expressed on a logistic scale 0–1, with larger values indicating greater species suitability. To derive a map of suitable/unsuitable habitats from the continuous species density distribution, a threshold must be applied. Selection of the optimal threshold is particularly critical because of the binary distribution of species suitability (suitable or unsuitable); small changes in the threshold can result in very different maps (Poirazidis et al., 2019). In previous studies three methods for selecting logistic thresholds for the division of suitable and unsuitable geographical areas that are considered to give greater prediction accuracy have been identified: the minimum training presence (MTP), ten percentile training presence (10P), and equal test sensitivity and specificity (ETSS) (Cao et al., 2013). The MTP method assumes that the least suitable habitat, where the species is known to be present, is the minimum suitability value for that species. The method is prone to exaggerate the geographical distribution range of the species and thus is more suitable for predicting invasive species than native species (Liu et al., 2005). The ETSS method uses a threshold based on where there is the same chance of the habitat being suitable and unsuitable. The 10P method assumes that 10% of the presence localities in the least suitable area are not present in regions that represent the overall habitat of the species and thus should be omitted. This is considered more proper for estimation of native species (Liu et al., 2005; Phillips et al., 2006; Jiménez and Lobo, 2007) and is the approach adopted in this study. The resulting 10P threshold for the binary distribution of Picea is 0.16 and for Pinus is 0.266. As figure two and three illustrates, after the application of the threshold, the area identified as suitable habitat is equally divided into three levels using ArcGIS, namely, low, moderate, and high suitable area. Areas designated moderately and highly suitable are identified as species stable geographical distribution areas.
At the LGM, the coldest period of the Last Glacial, Picea is mostly distributed along the southeast margin of the Tibetan Plateau (Figure 3A); these areas would have provided ecological refugia for Picea during LGM. In the EH, the highly suitable areas for Picea has expanded to the northern part of the Western Sichuan Plateau and southern Gannan Plateau, along the Taohe and Bailong rivers. The moderate and low suitable areas extend to the Huangshui and the Yellow rivers on the Qinghai Plateau and even the eastern margin of Qaidam Basin (Figure 3B). In the MH, the highly suitable areas for Picea have further migrated to the north and enlarged on the northeast Tibetan Plateau, extending along the Yellow and Huangshui rivers and some of their major tributaries. However, most of the highly suitable areas were located on the northern Gannan Plateau, and along the upper reaches of the Dadu River and tributaries on the Western Sichuan Plateau (Figure 3C). By the LH, the highly and moderately suitable areas are concentrated in the northeast Tibetan Plateau, specifically, in the eastern Qinghai Plateau. Low suitable areas include most higher altitude valleys and basins within the northeastern plateau (Figure 3D).
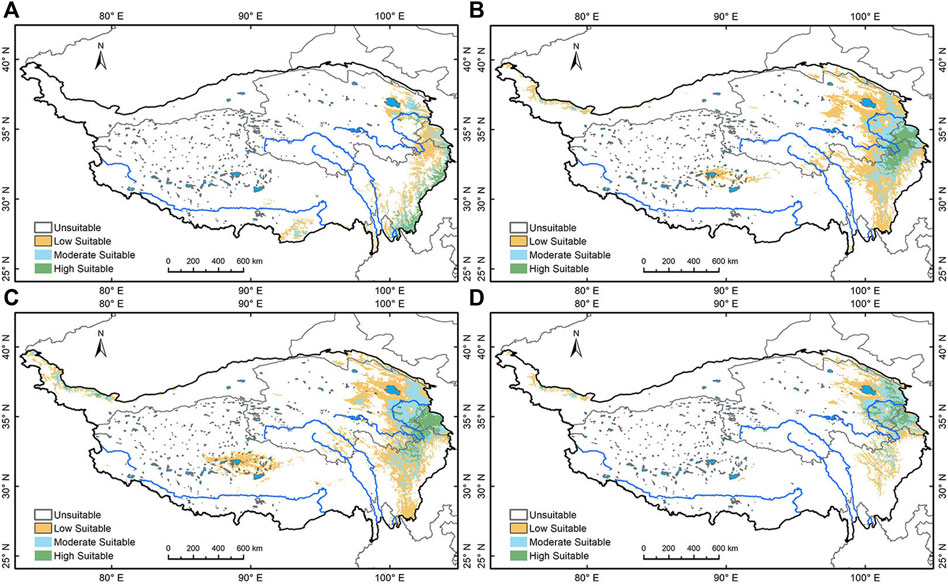
FIGURE 3. Simulation of Picea’s geographical distribution. (A) Picea’s geographical distribution area during LGM; (B) during Early Holocene; (C) during the Mid Holocene; (D) during the Late Holocene.
The relatively warm valleys along the eastern and southeastern margins of the plateau may also have provided ecological sanctuary for Pinus during the LGM, according to Hao et al. (2018). Our modeling shows Pinus has very scarcely distributed on the southeastern margin of the Tibetan Plateau at the LGM (Figure 4A). In the EH, the Pinus suitability area expanded significantly; it is sparsely distributed in Hehuang Valley, densely spotted on the Gannan Plateau and the southeastern part of the Western Sichuan Plateau, and extends westward to the eastern margin of the Qaidam Basin (Figure 4B). From EH to MH, Pinus gradually expands to the northern Tibetan Plateau, with a slight expansion in the northeast, and a dwindling of suitable area in the Western Sichuan Plateau (Figure 4C). In the LH, the suitable area contracted in both the Western Sichuan Plateau and Gannan Plateau, expanded in the Northeast Tibetan Plateau, and decreased in Qaidam Basin (Figure 4D).
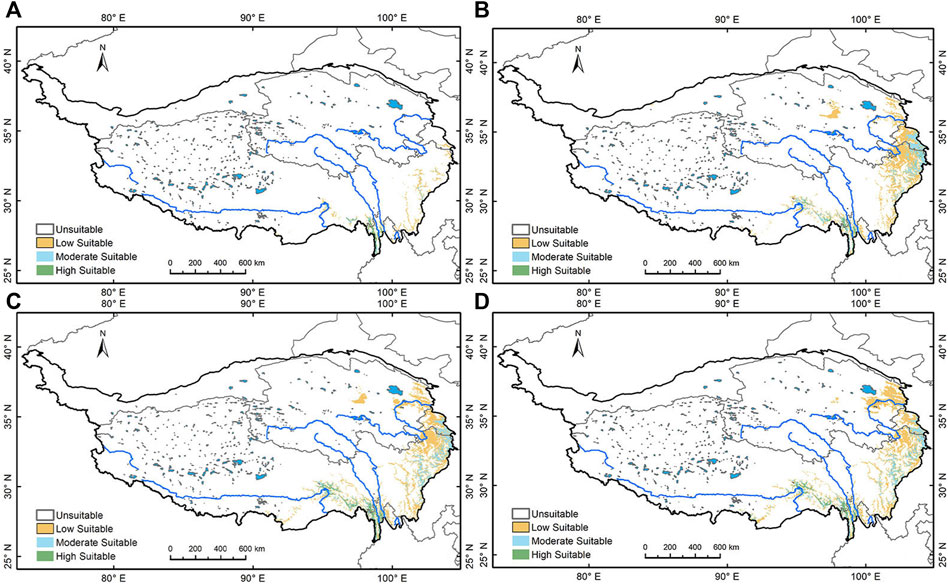
FIGURE 4. Simulation of Pinus’ geographical distribution. (A) Pinus’ geographical distribution area during the LGM; (B) during Early Holocene; (C) during the Mid Holocene; (D) during the Late Holocene.
Overall, both Picea and Pinus show a trend of northward migration and expansion since the LGM. Picea is distributed over a larger area than Pinus on the northeastern Tibetan Plateau, while Hehuang Valley and major drainages of the Yellow River present moderate to high suitability for both families. Therefore, the simulation results suggest that Picea better represents the forested land in the area. Most areas in the Northeast Tibetan Plateau were suitable for coniferous forests in the Holocene. Elevations above 3,000 m could also support sparsely distributed coniferous trees, especially Picea. In the LH, most of the highly suitable areas for Picea are concentrated in the Northeast Tibetan Plateau, indicating the species core distribution area finally reached the Northeast Tibetan Plateau.
Simulation accuracy
Simulation accuracy was quantified by calculating the area under the subject operating characteristic (ROC) curve (AUC). This gives a value range of (0.5–1), and the larger the value, the higher the model prediction accuracy (Hanley and McNeil, 1982). Generally, AUC of <0.6 implies the prediction result has failed, AUC of 0.6≤0.7 implies a poor prediction, AUC of 0.7≤0.8 implies a satisfactory prediction, AUC of 0.8≤0.9 implies a qualified prediction, and 0.9≤1.0 implies an accurate prediction (Swets, 1988). The AUC for Picea is 0.971 (Figure 5A) and the AUC for Pinus is 0.94 (Figure 5B), indicating a high accuracy for the simulation.
Accuracy was also determined by comparing the present day distribution of the Picea and Pinus with the simulation of the contemporary geographical distribution (Figures 6A,B). In terms of present day distribution, the Flora Republiacae Popularis Sinicae of China shows Picea crassifolia Com. is mainly found in Datong, Huangzhong, Huangyuan, Minhe, Ledu, Huzhu, Menyuan, Qilian, Haiyan, Tongren, Zeku, Henan, Xinghai, Maqin, Banma, Wulan (Hehuang Valley of Qinghai Plateau, valleys and basins of the upper Yellow River and around Qinghai Lake) in eastern Qinghai Province (ECFC, 1978). Also, in Xiahe, Zhuoni, and Zhouqu on the Gannan Plateau (Xiahe and its tributaries, Taohe and its tributaries, Bailong River and its tributaries). Pinus armandii Franch. and Pinus tabulaeformis Carr. are mainly found in southern Gansu along the drainage of Taohe and Bailongjiang rivers, Sichuan Province, Yunnan, and the lower reaches of Yalong Zangbu River. Zhang (2004) also indicates that Picea crassifolia is mainly found in the eastern Qilian Mountains and the southern Huangnan Mountains and Pinus in the Hehuang Valley in Qinghai Province. The close match between the modern distribution and the simulation for the Picea and Pinus lends additional weight to the simulation accuracy.
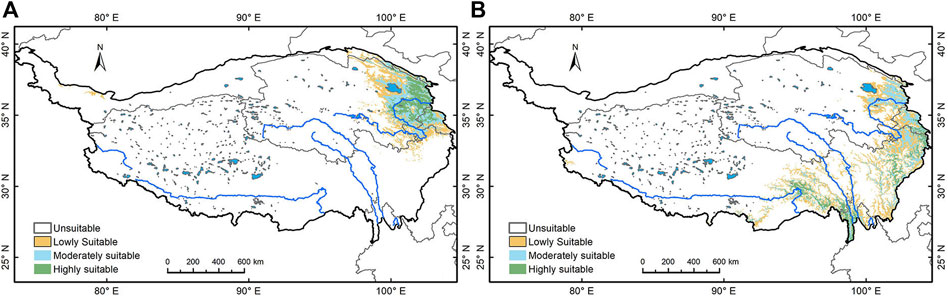
FIGURE 6. Simulation of Picea and Pinus’ current geographical distribution. (A) Picea and (B) Pinus.
In addition, dossil Pollen record indicates that during the LGM, the coniferous forests from north of China retreated to the south (Harrison et al., 2001), and the forests on the plateau mostly retreated to the southeast of the plateau along the Hengduan Mountains and Helan Mountains (Shi et al., 1998; Meng et al., 2007). The molecular phylogeography study of the Picea communities in Qinghai, Gansu and Ningxia showed a recolonization after the LGM from one ecological refugia (Yang et al., 2005; Meng et al., 2007). However, Pinus may had several refugia during the LGM and recolonized to the Tibetan Plateau (Chen, 2008). These findings perfectly matches with the simulation results.
Discussion
Dynamic change of forest and climate from Late Glacial Maximum to Early Mid Holocen (11.7–5.3 ka BP)
During the LGM, both the annual precipitation and annual average temperature in the northeastern Tibetan Plateau were much lower than today, and the region is characterized as dry and cold (Herzschuh, 2006). Following the termination of the LGM, the summer monsoon strengthened and temperature increased, though with some cold events (Anklin et al., 1993; Dykoski et al., 2005) (Figures 7A,B). Pollen concentration in Qinghai Lake Basin gradually increased, and records succession from desert steppe to alpine meadow/sub-alpine shrub at the start of the EH (Shen et al., 2004). At this time, the winter monsoon weakened, but the summer monsoon continued to strengthen (Yang et al., 2019) and total precipitation significantly increased, driving the transition from a cold dry to a relatively warm and humid environment (Chen et al., 2006; Zhao et al., 2006; Chen et al., 2015a; Zhang, 2018). Both the Gonghe Basin and Qinghai Lake areas recorded weakening of aeolian sand activity, increasing vegetation coverage, and enhanced pedogenesis in the EH (Liu et al., 2013). In the MH, solar radiation continued to strengthen and the summer monsoon from the Indian and Pacific oceans gradually increased, with temperatures peaking about 3°C–4°C higher than present around 6 ka BP (Wang et al., 2002), and precipitation peaking around 6.8 ka BP (Shen and Tang, 1996; Tang et al., 2021); this is thought to be the warmest and wettest period of the Holocene (Chen F. H et al., 2020). The warm and wet conditions are recorded by several proxies, including lake levels at Luanhaizi and Qinghai Lake that increased gradually from EH to MH (Mischke et al., 2004), and humidification degree in Hongyuan peat that shows a gradual increase in the percentage of amorphous humus from EH to MH (Wang et al., 2003).
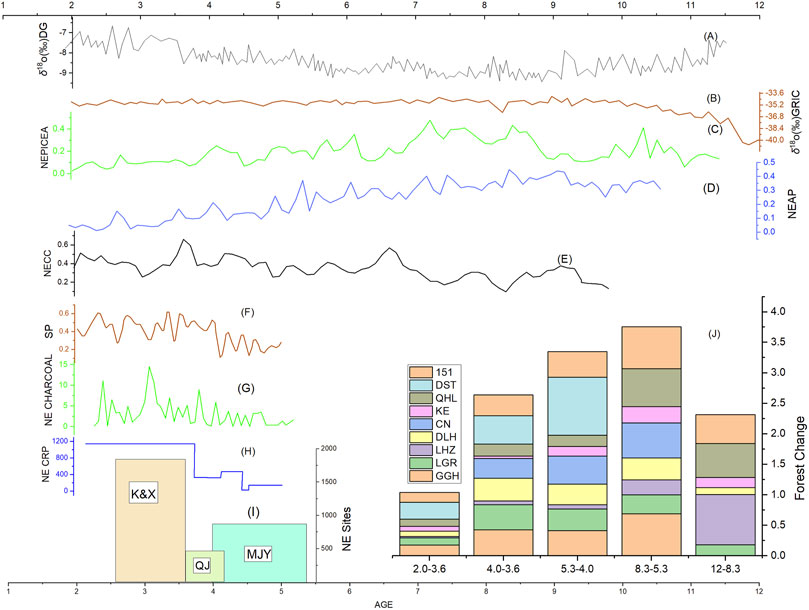
FIGURE 7. Relationships between climate change, forest dynamics, and human activities. (A) Greenland ice core oxygen isotope records representing global temperature changes (Anklin et al., 1993); (B) Oxygen isotope records of stalagmites in Dongge Cave indicating the intensity of Indian monsoon (Dykoski et al., 2005); (C) Normalized Pollen record of Picea from the Northeast Tibetan Plateau; (D) Normalized Pollen record of arboreal pollen from the Northeast Tibetan Plateau; (E) Normalized Carbon concentration record in the Northeast Tibetan Plateau; (F) Record of synanthropic plants through integrative pollen analysis in Northeast Tibetan Plateau during 5.5–2.0 ka BP (Chen X. L et al., 2020); (G) Charcoal concentration in different archeological sites in Northeast Tibetan Plateau during Mid-late Holocene (Liu et al., 2022); (H) Changes in cropland during the mid to late Holocene in the NETP (Wende et al., 2021); (I) Number of archaeological sites in the Northeast Tibetan Plateau. (J) Dynamic changes in arboreal pollen from different sites in Northeast Tibetan Plateau indicating change in forested area.
The warmer and wetter climate trend promoted vegetation productivity on the Tibetan Plateau, especially in reforestation. In Hehuang Valley, coniferous forest with steppe vegetation was recorded in warm periods of the Last Ice Age, and coniferous forest gradually increased from the Last Deglaciation into the EH, while shrubs, herbs, and Artemisia decreased (Zhao et al., 2007). In the Qinghai Lake drainage basin, increasing Picea and Pinus was recorded at approximately 16 ka BP, expansion of Picea-dominated coniferous forest began at approximately 10.8 ka BP, and expanded down slope to the lake shore by approximately 6 ka BP (Liu et al., 2002; Zhang N. M et al., 2021). In the upper reaches of the Yellow River, including Gonghe Basin, pollen records at both Dalianhai and Genggahai show increasing Picea levels from EH to MH (Cheng et al., 2010; Liu, 2016). Moreover, both the arboreal and Picea pollen records in the Northeast Tibetan Plateau showed a gradual increase with the warmer and wetter climate (Figures 6C,D). These trends are consistent with the simulation results in this study that show an expanding area of coniferous forest since the LGM.
A number of archeological sites on the Northeast Tibetan Plateau date to the period from the Last Deglaciation to the EH, including Site 151 (Wang et al., 2020), Jiangxigou 1 and 2, Heimahe 1 (Rhode et al., 2007; Gao et al., 2008; Yi, et al., 2011; Hou et al., 2013b), Hudong Zhongyang Chang (Madsen et al., 2006), Bronze Wire Canyon No. 3, and Yandongtai site (Brantingham and Gao, 2006; Sun et al., 2012; Yi, 2012; Brantingham et al., 2013). Evidence from these sites indicates the presence of early microlithic hunters with temporary camps in the Qinghai Lake Basin since 14 ka BP. It could be judged that increasing vegetation productivity provided a suitable habitat for the wild game which attracted nearby hunters. Animal bone finds at the sites are low at the beginning and gradually increase at around 8–7 ka BP (Hou et al., 2013b), suggesting increasing in wild hunting. By the EMH, archeological sites had expanded to the lower reaches of the Yellow River, as evidenced by early culture layers at the Shalongka (8.5–7.3 ka BP) (Yi et al., 2020) and Layihai sites (6.7 ka BP) (Gai and Wang, 1983). In this period, prehistoric humans mainly adopted short-term foraging activities with small groups focused on the procurement and processing of gazelle-sized ungulates (Brantingham et al., 2007; Wang et al., 2020). Due to the relatively small population and high mobility, human activities had no discernable impact on the natural forest.
In summary, forest vegetation began to develop slowly in the northeastern Tibetan Plateau following the LGM, though there was some short-term fluctuation due to cooling events. Humidity and temperature increased significantly Since the LGM, which supported environmental conditions conducive to the growth of forest vegetation. The coniferous forest gradually recovered from the cold climate and expanded from EH to EMH. Relatively, sparse and low-impact human activities in this period did not affect forest growth. Therefore, it could be stated that during the Eh to EMH climate change was essential in the dynamic changes of the forests on the Northeast Tibetan Plateau. It is the warming climate and increasing precipitation that contributed to the regeneration and expansion of the forests in this area.
Forest degradation and human activities in the Late Mid Holocene (5.3–4.2 ka BP)
The East Asia summer Monsoon peaked at around 6 ka BP, and then it declined gradually, but the climate was still warm and humid through the LMH and the Holocene optimum climate lasted until 4 ka BP(Tang et al., 2021). The warm and wet climate conditions encouraged the westward spread of Yangshao culture, bringing millet agriculture to the Northeast Tibetan Plateau and the formation of the Majiayao Culture (Xie, 2002). Both the climate conditions and simulation results show the potential for continuous development of coniferous forests in the study area in the LMH, however, fossil pollen records from archeological sites indicate a gradual decrease in tree pollen: Qinghai Lake (Liu et al., 2002), Genggahai (Liu, 2016), Dalianhai (Cheng et al., 2010), Langgeri (Wei et al., 2020), and Changning (Dong et al., 2012) all show a continuous decrease from 5 ka BP (Figure 7J). At the same time, proxies of anthropogenic activity such as carbon content (Figure 7E), synanthropic plants (Figure 7F), charcoal content (Figure 7G), number of archeological sites (Figure 7I), and area of cultivated land (Figure 7H), increased dramatically, indicating a close relationship between forest degradation and human activities in the LMH.
Evidence from archeological sites indicates the major human activities during this period included millet farming and pottery production. The cultural development of approximately 6.0–5.0 ka BP promoted the spreading of millet crops, and around 5.2 ka BP, millet crops spread from Guanzhong Plain and the eastern Gansu Province to the Hehuang Valley (Jia et al., 2013; Chen et al., 2015b; Dong et al., 2017). Millet grains have been recovered from archeological remains at Lijiatai, Hongya Zhangjia, Shangduoba, Shalongka, and Gayixianggeng (Jia, 2012). Remains of millet grains dating to around 5.2 ka BP were also unearthed from Zonggri site (5.2–4.1 ka BP), in Gonghe Basin in the upper reaches of the Yellow River. As the agricultural economy developed, the area of millet farming in Hehuang Valley rapidly increased (Wende et al., 2021). Many studies have shown that the expansion of farmland strongly impacts the natural vegetation cover (McNeill, 1986; Pongratz et al., 2008; Hou et al.,; Zhou et al., 2011; Fang et al., 2019; Wende et al., 2021); the prevailing slash-and-burn technique is likely to have greatly contributed to the decrease in forest area in Hehuang Valley. Furthermore, the development of agriculture promotes rapid population increase and expansion in settlement areas. In Hehuang Valley, the number of sites of late Majiayao Culture (Machang type) increased sharply in the LMH and the population reached about 39,000 (Wende et al., 2021). The increasing population inevitably required a great supply of wood resources for firewood, construction, production tools, and living utensils. Charcoal remains from archeological dated to this time comprise 100% Pinus and Picea (Liu et al., 2022), indicating coniferous species were the preferred wood resource used by people on the Northeast Tibetan Plateau, and they were sufficiently abundant to be easily accessed in daily life.
High demand for wood resources in the LMH is evident at many archeological settlements and burial sites. Charcoal remains from hearths indicate the daily use of wood for fire, including the firing of pottery. At Minhe Yangshan site, 96.3% of 218 graves were found to possess burial pottery (Qinghai Institute of Cultural Relics and Archaeology, 1990). In Ledu Liuwan cemetery, on the second level terrace of Huangshui River, 53.3% of 257 mid Majiayao Culture graves contained burial pottery, and more than 10,000 pieces of burial pottery were excavated from the 872 late Majiayao Culture graves (Institute of Archaeology CASS, 1984). In Xunhua Suhusa cemetery, 95.3% of 65 graves excavated contained pottery, and all 64 graves excavated at Zongri site contained burial pottery (Li and Xu, 1994). The pervasive usage of pottery indicates high production rates and a great demand for firewood. The pottery firing process requires a temperature above 800°C to be maintained (Papachristodoulou et al., 2006), and early firing kilns used ground pile burning (Qin and Ju, 2021), which consumes much wood to maintain the high temperature. It is important to note that charcoal remains from dead wood contain a large amount of mycelium (Marguerie and Hunot, 2009; Vidal-Matutano et al., 2017), and analysis of charcoal remains from this period shows very little mycelium (Liu, 2019). This suggests that early people obtained most of their firewood supply through deforestation. In addition, many stone axes have been unearthed from archeological sites and graves of this period, which may also provide strong physical evidence for wood cutting. It is reasonable to assume that the rapid increase in archeological settlement sites in the area consumed a great amount of wood for construction. Most of the settlement buildings at Majiayao are semi-subterranean dwellings (Xie, 2002), and dwelling sites and pillar holes have been excavated at Minhe Yangwapo, Hulijia, and Shalongka sites. Finally, most excavated graves contain wooden coffins (Table 3). In Liuwan Cemetery, 84% of graves had wooden coffins made from four slices of complete wood board (no patches), and in Shang Sunjiazhai, on the west bank of Beichuan River, a tributary of Huangshui River, all the graves had wooden coffins. In summary, a wide range of archeological evidence supports the presence of a rich forest resource in Hehuang Valley in the MH that was heavily exploited by humans. The simulation results and climate evidence both support the archeological findings. Forest retreat in the LMH, indicated by the reduction in arboreal pollen content, was most likely caused by heavy exploitation of the forest resource and cropland expansion. Thus, we argue that human activity is the driving force for forest retreat in the LMH.
Forest retreat and community structure evolution in the Late Holocene (4.2–2.3 ka BP)
The East Asia summer Monsoon greatly weakened around 4.0 ka BP in the Northeast Tibetan Plateau, with both temperature and precipitation decreasing in the LH (An et al., 2012; Chen et al., 2015a; Li et al., 2017). The simulation results showed a contraction of the low suitability area for coniferous trees toward the northeast margin of the plateau, while the high suitable area for Picea and moderate suitable area for Pinus increased markedly in Hehuang Valley. This indicates that through several thousand years of recolonization from the LGM refugia, Picea’s core distribution area has finally reached the Northeast Tibetan Plateau, which is also the current core distribution area of the Picea. It also implies that compared to Pinus, Picea is more adapted to the local environment. It is important to note that Picea is a cold and dry-tolerant species. Based on the current distribution, Picea persists with a mean annual temperature of 4.8°C (−4.1–12.3°C) and a mean temperature of the coldest month with −10°C (−17.3–1.1°C), annual precipitation of 351 mm (72–700 mm) and precipitation of the coldest month of 8 mm (1–15 mm) (Fang et al., 2009). While Pinus prefers a warmer climate compared to Picea. Thus, we argue that the LH climate shift alone would not have hindered Picea’s existence/distribution in the area. However, the climate shift may have slowed down the growth speed of the Picea, and modern pollen shows that the maximum Picea pollen appears with temperatures ranging from 0°C–8°C and precipitation ranging from 400–850 mm (Lu et al., 2004).
Charcoal remains from archaeological sites also support the distribution of Picea and a climate suitable for Picea. Specifically, ash pits from Jinchankou site (4.2–3.7 ka BP) contain a large quantity of Pinus (Wang et al., 2016). This implies that the climate in Hehuang Valley during the Qijia Culture period must also have been suitable for Picea since Pinus requires a warmer and more humid environment. In the charcoal remains of the Kayue sites in Hehuang Valley, broadleaf trees far exceeded the coniferous in the LH (Liu et al., 2022). Broadleaf trees mostly grow in environments with higher cumulative annual temperatures, while coniferous trees are the dominant species in areas with lower mean annual temperatures (CFEC, 1997). A large amount of charcoal remains from broadleaf trees excavated in the Hehuang Valley indicates that the LH climate was suitable for forest development. However, fossil pollen records for the area show continuously decreasing Picea and total arboreal pollen content in the Northeast Tibetan Plateau (Figures 7C,D). Both the Changning profile (Dong et al., 2012) and Dashuitang pollen record (Cao et al., 2021) show a significant reduction of arboreal pollen content, and pollen records from Qinghai Lake and Gonghe Basin also show a consistent trend of decreasing arboreal pollen content (Figure 7J). Hence, the pollen records show a very different picture to the results of the simulation and analysis of charcoal remains.
In the LH, there is a significant increase in the proxies indicating human activities (Figures 7E–I). Around 4 ka BP, through cultural exchange barley was introduced to the region from Eurasia (Dodson et al., 2013; Ren and Dong, 2016), which contributed to a shift in subsistence mode from solely millet-based agriculture to a wider range of crops at different altitudes (Ma et al., 2016; Zhang and Dong, 2017). This allowed the expansion of cropland to higher altitudes including Qinghai Lake and Gonghe Basin, and forests were transformed into farmland in the high altitude mountains in Hehuang Valley and middle altitude areas in the upper reaches of the Yellow River (Wende et al., 2021). By the time of the Xindian and Kayue Cultures at approximately 3.6 ka BP, grazing was clearly present in the area. Grazing practices require forest clearance initially, and continued grazing prevents forest regeneration. The Xindian Culture is mainly found in Hehuang Valley, where they practiced farming and livestock herding; the Kayue Culture is mainly at higher elevations, with grazing as their major source of livelihood and cultivation as a secondary source. Grazing seems to have intensified around 3.5 ka BP (Miao et al., 2017), and evidence of overgrazing is recorded in Qinghai Lake and Gonghe Basin (Huang et al., 2017; Wei et al., 2020). Hence, it is likely that the spread of barley and the emergence of grazing resulted in great transformation of native vegetation on a large scale at high altitudes. The new agricultural practices also promoted population development and settlement; by the late LH, there were more than 1,800 settlement sites in the northeastern Tibetan Plateau, with an estimated population of more than 60,000 (Hou et al., 2013a).
Alongside grazing and farming, the production of bronze and pottery was also prevalent in the LH, with the development of a bronze industry seen as the greatest achievement of the Qijia Culture (Xie, 2002). Bronze wares have been excavated from many Qijia sites in the region, including a long bronze spear at Shenna site (Wang et al., 2022), and the earliest bronze mirror at Gamatai site (Qinghai Institute of Cultural Relics and Archaeology and Beijing University, School of Archaeology and Museology, 2015), and the industry continued into the Kayue and Xindian Culture period. Bronze casting requires a temperature of over 1,000°C, especially for producing large wares such as long spears (Mao and Wang, 2019). The pervasive use of bronze wares implies great the consumption of firewood. In addition, pottery production had become a specialized, large scale industry in this period (Xie, 2002), and is likely to have aggravated consumption of the forest resource. Finally, evidence for use of wood for houses at Kayue and Qijia sites, and the dramatic increase in the number of archeological sites, also point to intensive deforestation for infrastructure construction.
Changes in the wood used for coffins over the LH support the persistence of coniferous trees and a declining forest resource. Wooden coffins were used intensively by the Qijia Culture, but their use decreased in most of the sites during the Kayue and Xindian Culture periods (Table 3). Also, most of the Qijia Culture coffins were made from a single log or single plank, but the Kayue and Xindian Culture coffins comprised patchwork boards with a wood diameter of only 10 cm. It is evident that wood utilization was extensive in the early LH and decreased in the late LH, which implies a marked shrinkage in the forest area. In contrast to that, in Suhusa site, located far to the south, the use of wood coffin increased (Xie, 2002). The ample availability of wood at some sites suggests climate was not a limiting factor on forest growth and wood supply. Thus, we argue the high exploitation rate of wood resources in Hehuang Valley and Gonghe Basin since the MH resulted in an extreme shortage of wood by the late LH. However, wood resources were still abundant in less populated areas. In addition, analysis of excavated charcoal shows high rates of broadleaf trees such as Poplar and Salix in the late LH in Hehuang Valley (Liu et al., 2022), which means that primitive coniferous forest in the Northeast Tibetan Plateau was succeeded by secondary forests. Overall, forest retreat in low elevation areas was followed by a secondary succession of the broadleaf forest communities, but intensive grazing and cultivation in higher elevation areas caused forest degradation that, when coupled with the drying and cooling climate, meant the fragile forest ecosystem failed to regenerate.
Conclusion
This study simulated the potential geographical distribution of Picea and Pinus since the LGM to determine the changing area of coniferous forests on the Northeast Tibetan Plateau. Pollen records, carbon concentration, charcoal analysis, archeological evidence, and other proxies for human activities were analyzed to explore human impact on natural forest dynamics. The key results are outlined below.
(1) The simulation shows that climate/environment conditions were suitable for the expansion of coniferous forest on the Northeast Tibetan Plateau from the beginning of the Holocene to the MH. Despite the climate shift in the LH, conditions were still suitable for the pervasive growth of Picea- and Pinus-dominated coniferous forests in Hehuang Valley, as well as the major drainage areas of the Yellow River. Picea showed a strong adaptation to the local environment in the Northeast Tibetan Plateau in the LH.
(2) The pollen record shows rapid forest expansion with optimal climate from EH to EMH, however, this is followed by forest retreat in the LMH, with the formation of the Majiayao Culture, which continued to the LH.
(3) We argue that low population numbers and the low impact of human activities, including short-term encampments for hunting and high mobility wild game, were not sufficient to affect the natural forest in the EH and EMH. However, a demographic boom, extensive cultivation, grazing, and heavy exploitation of the wood resources in the LMH and LH meant that human activities had a stronger influence on forest vegetation than climate on the Northeast Tibetan Plateau.
(4) Our findings also suggest LH climate conditions would not have inhibited forest development at lower elevations, especially coniferous forests, since archeological evidence supports the presence of coniferous and even broadleaf forests in Hehuang Valley. The cold dry climate of the LH may have aggravated the pace of deforestation at high elevations where severe deforestation had already occurred.
Data availability statement
The original contributions presented in the study are included in the article/supplementary material, further inquiries can be directed to the corresponding author.
Author contributions
GH and ZW conceived and designed the paper. GH, ZW, HC, SJ, and ZL participated in the field research. ZW wrote the manuscript and GH, HC, SJ, and ZL revised the manuscript. All authors contributed to the article and approved the submitted version.
Funding
This study wouldn't be possible without the support of National Natural Science Foundation. The grant numbers are 42171165 and 42261030.
Conflict of interest
The authors declare that the research was conducted in the absence of any commercial or financial relationships that could be construed as a potential conflict of interest.
Publisher’s note
All claims expressed in this article are solely those of the authors and do not necessarily represent those of their affiliated organizations, or those of the publisher, the editors and the reviewers. Any product that may be evaluated in this article, or claim that may be made by its manufacturer, is not guaranteed or endorsed by the publisher.
References
An, Z. S., Colman, S. M., Zhou, W. J., Li, X. W., Brown, E. T., Jull, T., et al. (2012). Interplay between the westerlies and asian monsoon recorded in lake Qinghai sediments since 32ka. Sci. Rep. 1, 619. doi:10.1038/srep00619
Anklin, M., Barnola, J. M., and Beer, J. (1993). Climate instability during the last interglacial period recorded in the GRIP ice core. Nature 364 (6434), 203–207. doi:10.1038/364203a0
Boivin, N. L., Zeder, M. A., Fuller, D. Q., Crowther, A., Larson, G., Erlandson, J. M., et al. (2016). Ecological consequences of human niche construction: Examining long-term anthropogenic shaping of global species distributions. Proc. Natl. Acad. Sci. U. S. A. 113, 6388–6396. doi:10.1073/pnas.1525200113
Brantingham, P. J., Gao, X., Madsen, D. B., Rhode, D., Perreault, C., Woerd, J., et al. (2013). Late occupation of the high-elevation northern Tibetan plateau based on cosmogenic, luminescence, and radiocarbon ages. Geoarchaeology 28 (5), 413–431. doi:10.1002/gea.21448
Brantingham, P. J., Gao, X., Olsen, W., Ma, H. Z., Rhode, D., Zhang, H. Y., et al. (2007). A short chronology for the peopling of the Tibetan Plateau. Devs. Quat. Sci. 9, 129–150. doi:10.1016/S15710866(07)090100
Brantingham, P. J., and Gao, X. (2006). Peopling of the northern Tibetan plateau. World Archaeol. 38 (3), 387–414. doi:10.1080/00438240600813301
Broecker, W. S., and Stocker, T. F. (2006). The Holocene CO2 rise: Anthropogenic or natural? EOS 87, 27–29. doi:10.1029/2006EO030002
Cao, X., Tian, F., Li, K., and Ni, J. (2021). Lake surface-sediment pollen dataset for the alpine meadow vegetation type from the eastern Tibetan Plateau and its potential in past climate reconstructions. National Tibetan Plateau Data Center. doi:10.11888/Paleoenv.tpdc.271191
Cao, Y., Dewalt, E., Robinson, J., Tweddale, T., Hinz, L., and Pessino, M. (2013). Using Maxent to model the historic distributions of stonefly species in Illinois streams: The effects of regularization and threshold selections. Ecol. Model. 259, 30–39. doi:10.1016/j.ecolmodel.2013.03.012
Castilla-Beltrán, A., de Nascimento, L., Fernández-Palacios, J. M., Whittaker, R. J., Willis, K. J., Edwards, M., et al. (2021). Anthropogenic transitions from forested to human-dominated landscapes in southern macaronesia. Proc. Natl. Acad. Sci. USA. 118, e2022215118. doi:10.1073/pnas.2022215118
CFEC (China Forest Editorial Committee) (1997). Forests in China. (Volumes I, II and III). Beijing: China Forestry Publishing House.
Chen, F. H., Cheng, B., Zhao, Y., Zhu, Y., and Madsen, D. B. (2006). Holocene environmental change inferred from a high-resolution pollen record, lake zhuyeze, arid China. Holocene 16, 675–684. doi:10.1191/0959683606hl951rp
Chen, F. H., Dong, G. H., Zhang, D. J., Liu, X. Y., Jia, X., An, C. B., et al. (2015b). Agriculture facilitated permanent human occupation of the Tibetan plateau after 3600 BP. Science 347, 6219248–6219250. doi:10.1126/science.1259172
Chen, F. H., Xu, Q. H., Chen, J. H., Birks, H. J., Liu, J. B., Zhang, S. R., et al. (2015a). East asian summer monsoon precipitation variability since the last deglaciation. Sci. Rep. 5, 11186. doi:10.1038/srep11186
Chen, F. H., Zhang, J. F., Liu, J. B., Cao, X. Y., Hou, J. Z., Xu, L. P., et al. (2020). Climate change, vegetation history, and landscape responses on the Tibetan Plateau during the Holocene: A comprehensive review. Quat. Sci. Rev. 243, 106444. doi:10.1016/j.quascirev.2020.106444
Chen, G. C., and Peng, M. (1993). Vegetation and its distribution pattern in Qinghai Lake area. J. Plant Eco. Geobot. 17 (1), 73–78.
Chen, K. M. (2008). “Phylogeography of Pinus tabulaeformis Carr. (Pinaceae), a dominant species of coniferous forest in northern China,”. Thesis (Lanzhou: Lanzhou University).
Chen, X. L., Hou, G. L., Jin, S. M., Gao, J. Y., and Duan, R. L. (2020). The pollen records of human activities in qinghai-tibet Plateau during the middle and late Holocene. Earth Envion 6, 643–651. doi:10.14050/j.cnki.1672-9250.2020.48.074
Cheng, B., Chen, F. H., and Zhang, J. F. (2010). Palaeovegetational and palaeoenvironmental changes since the last deglacial in Gonghe Basin, northeast Tibetan Plateau. Acta Geogr. Sin. 65 (11), 136–146. doi:10.1007/s1144201309995
Cheng, Z., Weng, C., Steinke, S., and Mohtadi, M. (2018). Anthropogenic modification of vegetated landscapes in southern China from 6, 000 years ago. Nat. Geosci. 11, 939–943. doi:10.1038/s41561-018-0250-1
Cobos, M. E., Peterson, A. T., Barve, N., and Osorio-Olvera, L. (2019). Kuenm: an R Package for detailed development of ecological niche models using maxent. Peer J. 6, e6281–e6281. doi:10.7717/peerj.6281
Dodson, J. R., Li, X., Zhou, X., Zhao, K., Sun, N., and Atahan, P. (2013). Origin and spread of wheat in China. Quat. Sci. Rev. 72, 108–111. doi:10.1016/j.quascirev.2013.04.021
Dong, G. H., Jia, X., An, C. B., Chen, F. H., Zhao, Y., Tao, S., et al. (2012). Mid-holocene climate change and its effect on prehistoric cultural evolution in eastern Qinghai Province, China. Quat. Res. 1, 23–30. doi:10.1016/j.yqres.2011.10.004
Dong, G. H., Yang, Y. S., Han, J. Y., Wang, H., and Chen, F. H. (2017). Exploring the history of cultural exchange in prehistoric Eurasia from the perspectives of crop diffusion and consumption. Sci. China Earth Sci. 60, 1110–1123. doi:10.1007/s11430-016-9037-x
Dykoski, A. C., Edwards, R. L., Cheng, H., Yuan, D. X., Cai, Y. J., Zhang, M. L., et al. (2005). A high resolution, absolute-dated Holocene and deglacial Asian monsoon record from Dongge Cave, China. Earth Planet. Sci. Letts. 233 (2), 71–86. doi:10.1016/j.epsl.2005.01.036
Elith, J., Graham, C. H., Anderson, R. P., Dudik, M., Ferrier, S., Guisan, A., et al. (2006). Novel methods improve prediction of species’ distributions from occurrence data. Ecography 29, 129–151. doi:10.1111/j.2006.0906-7590.04596.x
Elith, J., and Graham, C. H. (2009). Do they? How do they? WHY do they differ? On finding reasons for differing performances of species distribution models. Ecography 32, 66–77. doi:10.1111/j.1600-0587.2008.05505.x
Elith, J., Phillips, S. J., Hastie, T., Dudik, M., Chee, Y. E., and Yates, C. J. (2011). A statistical explanation of MaxEnt for ecologists. Divers. Distributions 17, 43–57. doi:10.1111/j.1472-4642.2010.00725.x
Ellis, E. C., and Ramankutty, N. (2008). Putting people in the map: Anthropogenic biomes of the world. Fron. Ecol. Environ. 6, 439–447. doi:10.1890/070062
Fang, J. Y., Wang, Z. H., and Tang, Z. Y. (2009). Atlas of woody plants in China: Distribution and climate, volume 1. Beijing: Higher Education Press.
Fang, X. Q., Ye, Y., Zhang, C. P., and Tang, C. C. (2019). Cropland cover change and its environmental impacts in the history of China. J. Paleogeogr. 21 (1), 160–174. doi:10.4135/9781446247501.n891
Feng, Y. X., and Li, G. D. (2020). Interaction between urbanization and eco-environment in Tibetan plateau. J. Geogr. Sci. 31, 298–324. doi:10.1007/s11442-021-1838-8
Ficetola, G. F. (2007). The influence of beach features on nesting of the hawksbill turtle Eretmochelys imbricata in the Arabian Gulf. Oryx 41, 402–405. doi:10.1017/S0030605307000543
Foley, J. A., Kutzbach, J. E., Coe, M. T., and Levis, S. (1994). Feed backs between climate and boreal forests during the Holocene epoch. Nature 371, 52–54. doi:10.1038/371052a0
Fordham, D. A., Saltré, F., Haythorne, S., Wigley, T. M. L., Otto-Bliesner, B. L., Chan, K. C., et al. (2017). PaleoView: A tool for generating continuous climate projections spanning the last 21000 Years at regional and global scales. Ecography 40, 1348–1358. doi:10.1111/ecog.03031
Gai, P., and Wang, G. D. (1983). Excavation report of mesolithic site in laiyihai, upper reaches of Yellow River. Acta. Anthropol. Sin. 1, 49–59. doi:10.16359/j.cnki.cn11-1963/q.1983.01.006
Gao, X., Zhou, Z. Y., and Guan, Y. (2008). Human cultural remains and adaptations strategies in the Tibetan plateau margin region in the late pleistocene. 969–977.
Goldewijk, K. K., Beusen, A., Doelman, J., and Stehfest, E. (2017). Anthropogenic land use estimates for the Holocene - hyde 3.2. Earth Syst. Sci. Data 9, 927–953. doi:10.5194/essd-9-927-2017
Hanley, J. A., and McNeil, B. J. (1982). The meaning and use of the area under a receiver operating characteristic (ROC) curve. Radiology 143, 29–36. doi:10.1148/radiology.143.1.7063747
Hao, Q., de Lafontaine, G., Guo, D., Gu, H., Hu, F. S., Han, Y., et al. (2018). The critical role of local refugia in postglacial colonization of Chinese pine: Joint inferences from DNA analyses, pollen records, and species distribution modeling. Ecography 41, 592–606. doi:10.1111/ecog.03096
Harrison, S. P., Yu, G., Takahara, H., and Prentice, I. C. (2001). Diversity of temperate plants in East Asia. Nature 413 (6852), 129–130. doi:10.1038/35093166
He, F., Li, Y., Xu, Y. N., Zhang, J. H., and Chen, S. B. (2005). Natural environment type, sand environment characteristics in northwest China. Northwest. Geol. 38 (3), 93–99.
Herzschuh, U., Birks, H. J., Ni, J., Zhao, Y., Liu, H., Liu, X., et al. (2010). Holocene land cover changes on the Tibetan plateau. Holocene 20, 91–104. doi:10.1177/0959683609348882
Herzschuh, U. (2006). Palaeo-moisture evolution in monsoonal Central Asia during the last 50, 000 years. Quat. Sci. Revs. 25, 163–178. doi:10.1016/j.quascirev.2005.02.006
Hijmans, R. J., Cameron, S. E., Parra, J. L., Jones, P. G., and Jarvis, A. (2005). Very high resolution interpolated climate surfaces for global land areas. Int. J. Climatol. 25, 1965–1978. doi:10.1002/joc.1276
Hou, G. L., Lai, Z. P., Xiao, J. Y., and E, C. Y. (2012). Reconstruction of cultivated land during mid-holocene in the middle and lower reaches of Yellow River and human impact on vegetations. J. Geogra. Sci. 22, 933–945. doi:10.1007/s11442-012-0974-6
Hou, G. L., E, C. Y., Zhao, X. H., and Wei, H. C. (2013a). Prehistoric population and cultivated land change in the eastern Qinghai Province and its environmental effects. Sci. Geogr. Sin. 03, 299–306. doi:10.13249/j.cnki.sgs.2013.03.010
Hou, G. L. (2016). The prehistoric human activities on the qinghai-tibet plateau. J. Salt Lake Res. 24 (2), 68–74.
Hou, G. L., Wei, H. C., E, C. Y., and Sun, Y. J. (2013b). Human activities and environmental change in Holocene in the northeastern margin of Qinghai-Tibet Plateau: A case study of JXG2 relic site in Qinghai Lake. Acta. Geogr. Sin 3, 380–388. doi:10.11821/xb201303009
Huang, X. Z., Liu, S. S., Dong, G. H., Qing, M. R., Bai, Z. J., Zhao, Y., et al. (2017). Early human impacts on vegetation on the northeastern Qinghai-Tibetan Plateau during the middle to late Holocene. Prog. Phys. Geog. 41, 3286–3301. doi:10.1177/0309133317703035
IGBP (International Geosphere–Biosphere Programme) (1994). IGBP in action: Work plan 1994–1998. Stockholm: IGBP, 38–82.
Jia, X. (2012). Cultural evolution process and plant remains during neolithic-bronze Age in Northeast Qinghai Province. Dissertation. Lanzhou: Lanzhou University.
Jia, X., Dong, G. H., Li, H., Brunson, K., Chen, F. H., Ma, M. M., et al. (2013). The development of agriculture and its impact on cultural expansion during the late neolithic in the western Loess Plateau, China. Holocene 23 (1), 85–92. doi:10.1177/0959683612450203
Jia, X., Lee, H. F., Cui, M. C., Chen, G. Q., Zhao, Y., Ding, H., et al. (2019). Differentiations of geographic distribution and subsistence strategies between Tibetan and other major ethnic groups are determined by the physical environment in Hehuang Valley. Sci. China (Earth Sci. . 62 (2), 412–422. doi:10.1007/s1143001893015
Jiang, Y. Y., E, C. Y., Hou, G. L., Sun, Y. J., Li, F., Zhao, Y. J., et al. (2015). Charcoal concentration reflecting on environmental changes and human activities in Qinghai Lake JXG2 Relics. J. Earth Environ. 2, 98–105.
Jiménez, V. A., and Lobo, J. M. (2007). Threshold criteria for conversion of probability of species presence to either–or presence–absence. Acta. Oecol 31, 3361–3369. doi:10.1016/J.ACTAO.2007.02.001
Kaal, J., Carrión, M. Y., Asouti, E., Martín, S. M., Martínez, C. A., Costa, C. M., et al. (2011). Long-term deforestation in NW Spain: Linking the Holocene fire history to vegetation change and human activities. Quat. Sci. Rev. 30, 161–175. doi:10.1016/j.quascirev.2010.10.006
Kaplan, J. O., Krumhardt, K. M., and Zimmermann, N. (2009). The prehistoric and preindustrial deforestation of europe. Quat. Sci. Rev. 28, 3016–3034. doi:10.1016/j.quascirev.2009.09.028
Karger, D. N., Nobis, M. P., Normand, S., Graham, C. H., and Zimmermann, N. E. (2021). CHELSA-TraCE21k v1. 0. Downscaled transient temperature and precipitation data since the last glacial maximum, doi:10.5194/cp-2021-30
Kirch, P. V. (2005). Archaeology and global change: The Holocene record. Ann. Rev. Environ. Resour. 30 (1), 409–440. doi:10.1146/annurev.energy.29.102403.140700
Kramer, A., Herzschuh, U., Mischke, S., and Zhang, C. (2010). Holocene Treeline Shifts and Monsoon Variability in the Hengduan Mountains (Southeastern Tibetan Plateau): Implications from Palynological Investigations. Palaeogeogr. Palaeocl. 286 (1–2), 23–41. doi:10.1016/j.palaeo.2009.12.001
Kumar, S., and Stohlgren, T. J. (2009). Maxent Modeling for Predicting Suitable Habitat for Threatened and Endangered Tree Canacomyricamonticola in New Caledonia. J. Ecol. Nat. Sci. 1, 94–98. doi:10.5897/JENE.9000071
Leal, A., Gassón, R., Behling, H., and Sánchez, F. (2019). Human-made Fires and Forest Clearance as Evidence for Late Holocene Landscape Domestication in the Orinoco Llanos (Venezuela). Veget. Hist. Archaeobot. 28, 545–557. doi:10.1007/s00334-019-00713-w
Li, J. Y., Dodson, J., Yan, H., Cheng, B., Zhang, X. J., Xu, Q. H., et al. (2017). Quantitative Precipitation Estimates for the Northeastern Qinghai-Tibetan Plateau over the Last 18, 000 Years. J. Geophys. Res. Atmos. 122, 5132–5143. doi:10.1002/2016jd026333
Li, X., Sun, N., Dodson, J., and Zhou, X. (2012). Human Activity and its Impact on the Landscape at the Xishanping Site in the Western Loess Plateau during 4800-4300 cal yr BP Based on the Fossil Charcoal Record. J. Archaeol. Sci. 39, 3141–3147. doi:10.1016/j.jas.2012.04.052
Liu, C., Berry, P. M., Dawson, T. P., and Pearson, R. G. (2005). Selecting Thresholds of Occurrence in the Prediction of Species Distributions. Ecogra 28 (3), 385–393. doi:10.1111/j.0906-7590.2005.03957.x
Liu, F. W. (2019). “Human Wood Utilization Strategy in the Northeast of Qinghai Tibetan Plateau During Neolithic and Bronze Age,”. Dissertation (Lanzhou (China): Lanzhou University).
Liu, F. W., Zhang, S. J., Zhang, H. C., and Dong, G. H. (2022). Detecting Anthropogenic Impact on Forest Succession from the Perspective of Wood Exploitation on the Northeast Tibetan Plateau During the Late Prehistoric Period. Sci. China Earth Sci. 65, 2068–2082. doi:10.1007/s11430-021-9911-7
Liu, S. S. (2016). “Vegetation and Climate Change since the Last Deglaciation in the Northeast Qinghai–Tibetan Plateau Genggahai Region,”. Dissertation (Lanzhou (China): Lanzhou University).
Liu, X. C., Tang, Q. H., Yin, Y. Y., and Xu, X. C. (2018). Regionalization of Integrated Environmental Risk of China Under Future Climate Change. Sci. Geogr. Sin. 38:4. 636–644. doi:10.13249/j.cnki.sgs.2018.04.018
Liu, X. Q., Shen, J., Wang, S. M., Yang, X. D., Tong, G. B., and Zhang, E. L. (2002). Pollen Record and its Paleo Climate, Paleo Environmental Evolution in Qinghai Lake Since 16ka. Chin. Sics. Bull. 47, 1351–1355.
Liu, X. X., Song, L., Jin, Y. X., and Wang, Q. (2013). History of Aeolian Deposits in Tibetan Plateau and Climate Change over Holocene. J. Arid. land Resours. Environ. 27 (6), 41–47. doi:10.13448/j.cnki.jalre.2013.06.036
Lozier, J. D., and Mills, N. J. (2011). Predicting the potential invasive range of light Brown apple moth (Epiphyas postvittana) using biologically informed and correlative species distribution models. Biol. Invasions 13, 2409–2421. doi:10.1007/s10530-011-0052-5
Lu, H. Y., Wang, S. Y., Shen, C. M., Yang, X. D., Tong, G. B., and Liao, K. B. (2004). Spatial Pattern of Modern Abies ans Picea Pollen in the Qinghai-Xizang Plateau. Chin. Qua. Sci. 1, 39–49.
Ma, M., Dong, G., Jia, X., Wang, H., Cui, Y., and Chen, F. (2016). Dietary Shift after 3600 cal yr bp and its Influencing Factors in Northwestern China: Evidence from Stable Isotopes. Quat. Sci. Revs. 145, 57–70. doi:10.1016/j.quascirev.2016.05.041
Madsen, D. B., Haizhou, M. a., Brantingham, P. J., Xing, G., Rhode, D., Haiying, Z., et al. (2006). The late Upper Paleolithic occupation of the northern Tibetan Plateau margin. J. Archaeol. Sci. 33 (10), 1433–1444. doi:10.1016/j.jas.2006.01.017
Mao, W. M., and Wang, K. P. (2019). Analysis of differences between Chinese and Western Bronze Ages. 4. 12-15
Marguerie, D., and Hunot, J. Y. (2009). Charcoal Analysis and Dendrology: Data from Archaeological Sites in North-Western France. J. Archaeol. Sci. 34 (10), 1417–1433. doi:10.1016/j.jas.2006.10.032
McMichael, C. N. H. (2021). Ecological Legacies of Past Human Activities in Amazonian Forests. New Phytol. 229 (5), 2492–2496. doi:10.1111/nph.16888
McNeill, J. R. (1986). Agriculture, Forests, and Ecological History: Brazil, 1500–1984. Environ. Hist. Rev. 10 (2), 122–133. doi:10.2307/3984562
McPherson, M. J., and Jetz, W. (2007). Effects of Species' Ecology on the Accuracy of Distribution Models. Ecography 30 (1), 135–151. doi:10.1111/j.0906-7590.2007.04823.x
Meng, L. H., Yang, R., Abbott, R. J., Miehe, G., Hu, T. H., and Liu, J. Q.(2007). Mitochondrial and chloroplast phylogeography ofPicea crassifoliaKom. (Pinaceae) in the Qinghai-Tibetan Plateau and adjacent highlands. Mol. Ecol. 16:19. 4128–4137. doi:10.1111/j.1365-294x.2007.03459.x
Miao, Y. F., Zhang, D. J., Cai, X. M., Li, F., Jin, H. L., Wang, Y. P., et al. (2017). Holocene Fire on the Northeast Tibetan Plateau in Relation to Climate Change and Human Activity. Quat. Int. 443, 124–131. doi:10.1016/j.quaint.2016.05.029
Miehe, G., Hasson, S., Glaser, B., Mischke, S., Böhner, J., van der Knaap, W. O., et al. (2021). Föhn, Fire and Grazing in Southern Tibet? A 20, 000-Year MultiProxy Record in an Alpine Ecotonal Ecosystem. Quat. Sci. Rev. 256, 106817. doi:10.1016/J.QUASCIREV.2021.106817
Miehe, G., Miehe, S., Böhner, J., Kaiser, K., Hensen, I., Madsen, F. N. M., et al. (2014). How Old is the Human Footprint in the World’s Largest Alpine Ecosystem? A Review of Multi-proxy Records from the Tibetan Plateau from the Ecologists’ Viewpoint. Quat. Sci. Rev. 86, 190–209. doi:10.1016/j.quascirev.2013.12.004
Milanovich, J. R., Peterman, W. E., Barrett, K., and Matthew, E. H. (2012). Do Species Distribution Models Predict Species Richness in Urban and Natural Green Spaces? A Case Study Using Amphibians. Landsc. Urban Plan. 107 (4), 409–418. doi:10.1016/j.landurbplan.2012.07.010
Mischke, S., Herzschuh, U., Zhang, C., Bloemendal, J., and Riedel, F. (2004). A Late Quaternary lake record from the Qilian Mountains (NW China): lake level and salinity changes inferred from sediment properties and ostracod assemblages. Glob. Planet. Change 46 (1), 337–359. doi:10.1016/j.gloplacha.2004.09.023
Mottl, O. F., Bhatta, K. P., Felde, V. A., Giesecke, T., Goring, S., Grimm, E. C., et al. (2021). Global Acceleration in Rates of Vegetation Change Over the Past 18, 000 Years. Sci 372 (6544), 860–864. doi:10.1126/science.abg1685
Olofsson, J., and Hicker, T. (2007). Effects of Human Land-Use on the Global Carbon Cycle During the Last 6000 years. Veg. Hist. Archaeol. 17 (5), 605–615. doi:10.1007/s00334-007-0126-6
Papachristodoulou, C., Oikonomou, A., Ioannides, K., and Gravani, K. (2006). A Study of Ancient Pottery by Means of X-Ray Fluorescence Spectroscopy, Multivariate Statistics and Mineralogical Analysis. Anal. Chim. Acta 573–574, 347–353. doi:10.1016/j.aca.2006.02.012
Phillips, S. J., Anderson, R. P., and Schapire, R. E. (2006). Maximum Entropy Modeling of Species Geographic Distributions. Ecol. Model. 190, 231–259. doi:10.1016/j.ecolmodel.2005.03.026
Phillips, S. J., Dudik, M., Elith, J., Graham, C. H., Lehmann, A., Leathwick, J., et al. (2009). Sample Selection Bias and Presence-only Distribution Models: Implications for Background and Pseudo-absence Data. Ecol. Appl. 19, 181–197. doi:10.1890/07-2153.1
Pimm, S. L., Jenkins, C. N., Abell, R., Brooks, T. M., Gittleman, J. L., Joppa, L. N., et al. (2014). The Biodiversity of Species and Their Rates of Extinction, Distribution, and Protection. Sci 344, 1246752. doi:10.1126/science.1246752
Poirazidis, K., Bontzorlos, V., Xofis, P., Zakkak, S., Xirouchakis, S., Grigoriadou, E., et al. (2019). Bioclimatic and Environmental Suitability Models for Capercaillie (Tetrao urogallus) Conservation: Identification of Optimal and Marginal Areas in Rodopi Mountain-Range National Park (Northern Greece). Glob. Ecol. Conserv. 17, e00526. doi:10.1016/j.gecco.2019.e00526
Pongratz, J., Reick, C., Raddatz, T., and Claussen, M. (2008). A Reconstruction of Global Agricultural Areas and Land Cover for the Last Millennium. Glob. Biogeochem. Cycles 22 (3), GB3018. doi:10.1029/2007GB003153
Qi, B. Z., (2022). Environmental Evolution and Human Activities in the Gonghe Basin During the Mid-Late Holocene, A Case Study of Nankayan Site. M.
Qin, L., Dorian, Q. F., and Zhang, H. (2010). Modelling Wild Food resource Catchments Amongst Early Farmers: Case Studies From the Lower Yangtze and Central China. Quat. Sci. 30, 2245–2261. doi:10.3969/j.issn.1001-7410.2010.02.02
Qin, X. L., and Ju, X. N. (2021). On The Open Firing Technique and the Neolithic Pottery Firing Technique of the Yangtze River’s Lower Reaches with the Cases of Pishan. Xiaodouli Xindili Sites Zhejiang 6 (109), 44–57. doi:10.16319/j.cnki.0452-7402.2021.06.005
Qinghai Institute of Cultural Relics and Archaeology and Beijing University (2015). School of Archaeology and Museology. Beijing: Science Press.
Qinghai Institute of Cultural Relics and Archaeology (1990). Minghe yangshan. Beijing: Cultural Relics Press.
Ren, G. (2000). Decline of the Mid-to Late Holocene Forests in China: Climatic Change or Human Impact? J. Quat. Sci. 15, 273–281. doi:10.1002/(sici)1099-1417(200003)15:3<273::aid-jqs504>3.0.co;2-2
Ren, L. L., and Dong, G. H. (2016). The history for origin and diffusion of “Six livestock”. Chin. J. Nat. 4, 257–262. doi:10.3969/j.issn.0253-9608.2016.04.00
Rhode, D., Zhang, H. Y., Madsen, D. B., Gao, X., Brantingham, P. J., Ma, H. Z., et al. (2007). Epipaleolithic/Early Neolithic Settlements at Qinghai Lake, Western China. J. Archaeol. Sci. 34 (4), 600–612. doi:10.1016/j.jas.2006.06.016
Shen, C. M., and Tang, L. Y. (1996). Pollen Evidence for Paleo-Monsoon Changes in Holocene over the Tibetan Plateau. Acta Micropalaeontological Sin. 13 (4), 433–436.
Shen, J., Liu, X. Q., Wang, S. M., and Matsumoto, R. (2004). Palaeoclimatic changes in the Qinghai Lake area during the last 18, 000 years. Quat. Int. 136 (1), 131–140. doi:10.1016/j.quaint.2004.11.014
Shi, Y. F., Li, J. J., and Li, B. Y. (1998). Up lift and environmental changes of Qinghai-Tibetan Plateau in the late cenozoic. Guangzhou: Guangdong Science and Technology Press.
Steffen, W., Crutzen, P. J., and McNeill, J. R. (2007). The Anthropocene: Are Humans Now Overwhelming the Great Forces of Nature. AMBIO 36 (8), 614–621. doi:10.1579/0044-7447(2007)36[614:taahno]2.0.co;2
Sun, Y. J., Lai, Z. P., Madsen, D., and Hou, G. L. (2012). Luminescence Dating of a Hearth from the Archaeological site of Jiangxigou in the Qinghai Lake area of the northeastern Qinghai-Tibetan Plateau. Quat. Geochronol. 12, 107–110. doi:10.1016/j.quageo.2012.01.010
Swets, J. A. (1988). Measuring the Accuracy of Diagnostic Systems. Science 240, 48571285–48571293. doi:10.1126/science.3287615
Tang, L. T., Liu, D., Luo, X. P., Hu, L., and Wang, C. T. (2019). Forest Soil Phosphorus Stocks and Distribution Patterns in Qinghai, China. Plant Eco 43 (12), 1091–1103. doi:10.17521/cjpe.2019.0194
Tang, L. Y., Shen, C. M., Lu, H. Y., Li, C. H., and Ma, Q. F. (2021). Fifty Years of Palynology in the Tibetan Plateau. China. Sci. earth. Sci. 64, 1825–1843. doi:10.1007/s11430-020-9809-5
Teng, S. Q., Xu, Z. W., Lu, H. Y., and Xu, C. (2022). Megafauna declines and extinctions over the past 40, 000 years in eastern monsoonal China: causes, consequences and implications. Sci. Sin. Vitae. 52, 418–431. doi:10.1360/SSV-2021-0212
Tuo, F., Liu, X. D., Liu, R. H., Zhao, W. J., Jin, W. M., Ma, J., et al. (2020). Spatial Pattern and Correlation of Qinghai Spruce Population in Dayekou stream area of Qilian Mountains. Plant Eco 11, 1172–1183. doi:10.17521/cjpe.2020.0177
VanDerWal, J., Shoo, L. P., Graham, C., and William, S. E. (2009). Selecting Pseudo-absence Data for Presence-only Distribution Modeling: How Far Should You Stray From What You Know? Ecol. Model. 220, 589–594. doi:10.1016/j.ecolmodel.2008.11.010
Veloz, S. D. (2009). Spatially Autocorrelated Sampling Falsely Inflates Measures of Accuracy for Presence-only Niche Models. J. Biogeogr. 36, 2290–2299. doi:10.1111/j.1365-2699.2009.02174.x
Vidal-Matutano, P., Henry, A., and Théry-Parisot, I. (2017). Dead Wood Gathering Among Neanderthal Groups: Charcoal evidence from Abric del Pastor and El Salt (Eastern Iberia). J. Archaeol. Sci. 80, 109–121. doi:10.1016/j.jas.2017.03.001
Vitousek, P. M., Mooney, H. A., Lubchenco, J., and Melillo, J. M. (1997). Human Domination of Earth’s Ecosystems. Sci 277, 494–499. doi:10.1126/science.277.5325.494
Wan, Y. F. (2017). Analysis on transpiration characteristics and influencing factors of Qinghai spruce forest in qilian mountains. [M]. Lanzhou: Gansu Agricultural University.
Wang, H., Hong, Y. T., Zhu, Y. X., Lin, Q. H., Len, X. T., and Mao, X. M. (2003). The Peat Humification Recors of Holocene Climate Change in Hongyuan Region.
Wang, Y., Li, G. H., Qiao, H., Zhang, Q. M., and Feng, X. (2022). Shenna site, Chengbei district, Xining city, 1992-1993 excavation brief. Archaeology 05, 3–23+2.
Wang, J., Xia, H., Yao, J. T., Shen, X. K., Cheng, T., Wang, Q. Q., et al. (2020). Subsistence Strategies of Prehistoric Hunter-Gatherers on the Tibetan Plateau during the Last Deglaciation. Sci. China Earth Sci. 63 (3), 395–404. doi:10.1007/s11430-019-9519-8
Wang, S. W., Cai, J. N., Zhu, J. H., and Gong, D. Y. (2002). Studies on Climate Change in China. Clim. Environ. Res. 7 (2), 137–145.
Wang, S. Z., Wang, Q. Q., Wang, Z. X., Liang, G. J., Qi, W. Y., and Ren, X. Y. (2016). Utilization and Ecological Environment Indicated by Charcoal Remains of Middle and Late Qijia Culture in Jinchankou Site. Agri. Archaeo. 1, 9–15.
Warren, D. L., and Seifert, S. N. (2011). Ecological niche modeling in Maxent: the importance of model complexity and the performance of model selection criteria. Ecol. Appl. 21, 335–342. doi:10.1890/10-1171.1
Wei, H. C., E, C. Y., Zhang, J., Sun, Y. J., Li, Q. K., Hou, G. L., et al. (2020). Climate Change and Anthropogenic Activities in Qinghai Lake Basin Over the Last 8500 Years Derived From Pollen and Charcoal Records in an Aeolian Section. Catena 193, 104616. doi:10.1016/j.catena.2020.104616
Wende, Z., Hou, G., Gao, J., Chen, X., Jin, S., and Lancuo, Z. (2021). Reconstruction of Cultivated Land in the Northeast Margin of Qinghai–Tibetan Plateau and Anthropogenic Impacts on PalaeoEnvironment During the Mid-Holocene. Front. Earth Sci. 9, 681995. doi:10.3389/feart.2021.681995
Whitney, G. G. (1994). From coastal wilderness to fruited plain: A history of environmental change in temperate North America from 1500 to the present. Cambridge: Cambridge University Press.
Woodbridge, J., Fyfe, R., Smith, D., Pelling, R., Vareilles, A., Batchelor, R., et al. (2020). What Drives Biodiversity Patterns? Using Long-term Multidisciplinary Data to Discern Centennial-Scale Change. J. Ecol. 109 (3), 1396–1410. doi:10.1111/1365-2745.13565,
Wu, Y. H., and Wu, R. H. (2016). Spermatophytes of upper Yellow River Valley in eastern Qinghai. Acta Bot. Boreali-Occidentalia Sin. 28 (1), 1–12.
Yang, R., Meng, L. H., Zhang, X., and Liu, J. Q. (2005). The Mitochondrial DNA nad1Sequence Variation of Picea crassifolia in the Qinghai-Tibetan Plateau Platform and Adjacent Populations. Acta ecolo. Sin. 12, 3307–3313.
Yang, S. L., Dong, X. X., and Xiao, J. L. (2019). The East Asian Monsoon since the Last Glacial Maximum: Evidence from Geological Records in Northern China. Sci. China Earth Sci. 49 (8), 1169–1181. doi:10.1360/N072018-00056
Yi, H. L., Song, Y. B., and Xiao, Y. M. (2020). Study of Shalongka Site Animal Relics, Qinghai Huanlong County. Northern Cul. Relics 5, 66–77. doi:10.16422/j.cnki.1001-0483.2020.05.007
Yi, M. J., Gao, X., Zhang, X. L., Sun, Y. J., Brantingham, P. J., Madsen, D. B., et al. (2011). A Preliminary Report on Investigations in 2009 of Some Prehistoric Sites in the Tibetan Plateau Marginal Region. 2:124-136.
Yi, M. J. (2012). “The Discovery and Study of Paleolithic in Qinghai Province,” in Proceedings of the 13th annual conference of Chinese vertebrate paleontology (Beijing: China Ocean Press), 195–202.
Yu, Y. Y., Wu, H., and Guo, Z. T. (2010). A New Simulation Model for Prehistoric Land Use and Carbon Storage(PLCM)- An Application in Yiluo Valleys. Quat. Sci. 30 (3), 540–549. doi:10.3969/j.issn.10017410.2010.03.12
Zhang, N. M., Cao, X. Y., Xu, Q. H., Huang, X. Z., Herzschuh, U., Shen, Z. W., et al. (2021). Vegetation Change and Human-Environment Interactions in the Qinghai Lake Basin, Northeastern Tibetan Plateau, since the Last Deglaciation. Catena 210, 105892. doi:10.1016/j.catena.2021.105892
Zhang, S. J., and Dong, G. H. (2017). Human Adaptation Strategies to Different Altitude Environment During Mid-Late Bronze Age in Northeast Tibetan Plateau. Quat. Sci. 37, 4696–4708. doi:10.11928/j.issn.1001-7410.2017.04.03
Zhang, X. L., Ha, B. B., Wang, S. J., Chen, Z. J., Ge, J. Y., Long, H., et al. (2018). The Earliest Human Occupation of the High-Altitude Tibetan Plateau 40 Thousand to 30 Thousand Years Ago. Sci 362, 1049–1051. doi:10.1126/science.aat8824
Zhang, X., Wang, W. C., Fang, X., Ye, Y., and Zheng, J. (2012). Agriculture Development-Induced Surface Albedo Changes and Climatic Implications Across Northeastern China. Chin. Geogr. Sci. 22 (3), 264–277. doi:10.1007/s11769-012-0535-z
Zhang, Y. L., Li, B. Y., Liu, L. S., and Zhen, D. (2021). Rediscussion on the extent of the Tibetan Plateau. Geogr. Res. 40 (6), 1543–1553. doi:10.11821/dlyj020210138
Zhao, Y., Yu, Z. C., Chen, F. H., and Zhao, C. (2006). Holocene Vegetation and Climate History at Hurleg Lake in the Qaidam Basin, Northwest China. Rev. Palaeobot. 145, 275–288. doi:10.1016/j.revpalbo.2006.12.002
Zhao, Y., Yu, Z., Chen, F. H., and An, C. (2007). “Holocene Vegetation and Climate Changes from Fossil Pollen Records in Arid and Semiarid China Late Quat. Climate Change and Human Adaptation in Arid China,” in Developments in quaternary science (Netherlands: Elsevier), 51–65.
Zhou, Q. Y. (2003). High-resolution study on the Holocene pedogenic environment evolution and human influence in the weihe river basin. D. Xi'an: Shanxi Normal University.
Zhou, X., and Li, X. (2012). Variations in Spruce (Picea sp.) Distribution in the Chinese Loess Plateau and Surrounding Areas during the Holocene. Holocene 22, 687–696. doi:10.1177/0959683611400195
Zhou, X. Y., Li, X. Q., Zhao, K. L., Dodson, J., Sun, N., and Yang, Q. (2011). Early agricultural development and environmental effects in the Neolithic Longdong basin (eastern Gansu). Sci. Bull. 56, 762–771. doi:10.1007/s11434-010-4286-x
Keywords: coniferous forest, simulation, pollen, human activities, Tibetan plateau
Citation: Wende Z, Hou G, Chen H, Jin S and Zhuoma L (2023) Dynamic changes in forest cover and human activities during the Holocene on the northeast Tibetan plateau. Front. Earth Sci. 11:1128824. doi: 10.3389/feart.2023.1128824
Received: 21 December 2022; Accepted: 17 January 2023;
Published: 30 January 2023.
Edited by:
Ren Lele, Lanzhou University, ChinaCopyright © 2023 Wende, Hou, Chen, Jin and Zhuoma. This is an open-access article distributed under the terms of the Creative Commons Attribution License (CC BY). The use, distribution or reproduction in other forums is permitted, provided the original author(s) and the copyright owner(s) are credited and that the original publication in this journal is cited, in accordance with accepted academic practice. No use, distribution or reproduction is permitted which does not comply with these terms.
*Correspondence: Guangliang Hou, hgl20@163.com