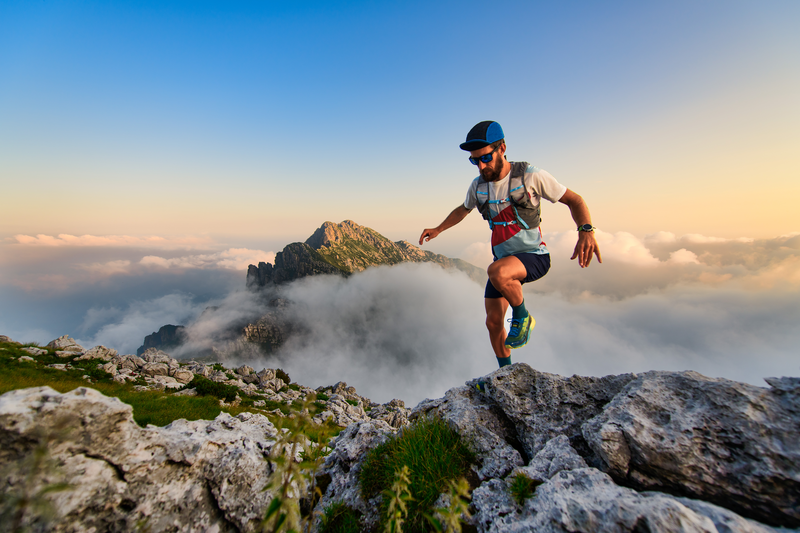
94% of researchers rate our articles as excellent or good
Learn more about the work of our research integrity team to safeguard the quality of each article we publish.
Find out more
ORIGINAL RESEARCH article
Front. Earth Sci. , 28 February 2023
Sec. Economic Geology
Volume 11 - 2023 | https://doi.org/10.3389/feart.2023.1127995
This article is part of the Research Topic Recent Advances in Unconventional Natural Gas View all 10 articles
The buried depth of the main coal beds in SLN Block is more than 1,500 m, and the gas content of the coal beds is high. However, the coal beds are generally in a high geostress environment, the permeability of the coal beds is generally low, and the development effect of conventional fractured straight wells is poor. Fractured horizontal well is an important technical means to improve the development effect of low permeability coalbed methane, but the investment cost is high. To reduce the investment risk of fractured horizontal wells, it is necessary to find out the gas production capacity and main control factors of the fractured horizontal wells, and determine the area suitable for the development of fractured horizontal wells. In the area with permeability higher than 0.28 md and gas content higher than 12.8 m3/t, the daily gas production capacity of fractured horizontal well is 16,450–21500 m3/d, and the development effect is good. The research results are of great significance to quickly evaluate the adaptability of fractured horizontal wells.
China has abundant deep coalbed methane (CBM) resources. The total resources of CBM buried between 1,000 and 2,000 m is approximately 22.4 × 1012 m3 (Luo et al., 2019; Xu et al., 2019). As of 2015, China has been developing CBM for more than 40 years, and 20,000 CBM wells have been drilled (Li et al., 2015; Mu et al., 2015; Yan et al., 2019). Due to the poor permeability of deep coal beds, the development effect of fracturing straight wells is not ideal (Fei et al., 2015; Cao et al., 2016; Ali et al., 2017; Su et al., 2018). How to improve the gas production is a major problem faced by China’s deep CBM development (Song et al., 2015; Sun et al., 2016; Fan et al., 2018). The horizontal well is a very important stimulation technology, which has the advantages of small well pad area, large discharge area, high gas production of single well, and quick capital recovery (Liu et al., 2018). According to whether the horizontal section of horizontal well is fractured, it can be divided into non-fractured horizontal well and fractured horizontal well (Hoang et al., 2008; Gentzis. 2009). Different types of horizontal wells have different geological adaptation conditions. In order to maximize the development benefit, it is necessary to evaluate the geological conditions suitable for fractured horizontal wells. Scholars at home and abroad have conducted a lot of research on coal seam permeability, gas content, coal seam thickness, isothermal adsorption performance, coal structure, structural characteristics and fault development (Laubach and Gale. 2006; Zou et al., 2018). Unfractured horizontal wells are applicable to high permeability and high gas bearing areas with simple structures and undeveloped faults, and fractured horizontal wells are applicable to medium low permeability and medium high gas bearing areas (Thararoop et al., 2012; Zhang et al., 2017). Predecessors’ research results are mainly concentrated in the shallow coal beds with a depth of 1,000 m, the geostress is relatively low, and the physical properties of the coal beds are relatively good (Zhang 2014). The burial depth of the coal beds in SLN Block is generally more than 1,500 m, the ground stress of the coal beds is generally high, and the physical properties are generally poor. Whether the geological adaptability of horizontal wells is the same as that of the middle and shallow parts needs further study. On the basis of previous research results, this paper uses numerical simulation method to study the gas production of fractured horizontal wells under different coal seam physical properties and thicknesses, and determines the geological conditions of deep coal beds suitable for fractured horizontal wells according to the gas production.
The Ordos Basin is situated in central China and has an area of 25 × 104 km2, which is composed of six structural units (Figure 1): Yimeng uplift, Weibei uplift, Western edge thrusting belt, Jinxi flexural fold belt, Tianhuan depression, and Yishan slope. The SSN Block covers an area of about 975 km2, which is located in the southern area of Ordos Basin. It is adjacent to the Jinxi flexural fold belt in the middle north and Yishan slope in the West. Four main faults are developed in the area: Shilou East fault, Caocun fault, Caocun East fault, and Xixian East fault, forming six structural units, including Shilou anticline, Shilou East syncline, Caocun anticline, Caocun East syncline, Xixian anticline, and Xixian East syncline.
FIGURE 1. The location and geological setting of the SSN Block (revised from Tang et al., 2018), (A) and (B) are the geological structure map; (C) is the stratigraphic column.
The coal-bearing deposits of the basin are composed of Pennsylvanian, Permian, and Triassic. From bottom to top, there are Carboniferous Benxi Formation and Taiyuan Formation, Permian Shanxi Formation, Shihezi Formation and Shiqianfeng Formation. The block mainly develops five layers of coal beds, including No. 4 and No. 5 coal beds in the Shanxi Formation of Permian (P1s), No. 7, No. 8, and No. 9 coal beds in the Taiyuan Formation of upper Carboniferous (C3t). The No. 9 coal seam is the target stratum for the coalbed methane (CBM) development.
The thickness of the No. 9 coal seam ranges from 2.2 to 8.5 m, with a mean value of 5.6 m. The buried depth of No. 9 coal Seam is 1,300–2,000 m, with an average of 1,650 m. The buried depth of the No. 9 coal seam is gradually deepened from the South to the North, while the thickness shows a contradict changes trend. The vitrinite reflectance is 1.70%–2.20%, with an average of 1.93%. The coal rank is dominated by lean coal and lean coal. The coal seam gas content of Shanxi Formation is 14.0–28.9 m3/t, with an average of 16.8 m3/t; The gas saturation is 82.1%–97.6%, with an average of 92.6%. The coal seam gas content of Taiyuan Formation is 14.5–23.2 m3/t, with an average of 18.8 m3/t; Gas saturation is 68.2%–89.8%, with an average of 82.1%. The coal seam porosity is 1.79%–7.24%, with an average of 3.66%. The permeability of coal seams in this area varies greatly from 0.01 to 6.4 mD, which is not only affected by the change of coal seam burial depth, but also related to structural characteristics and coal quality.
The simulation area is 2,315 m × 2,235 m horizontal rectangular area (Figure 2A), the whole simulation area is divided into 49 × 51 grids. The 15th, 20th, 30th, 35th columns and the 25th, 26th, and 27th rows are densified grids, with grid sizes of 5 m × 50 m and 50 m × 5 m, other grid size is 50 m × 50 m. The grid permeability in line 26 is 0.005 mD, simulating the pollution zone around the wellbore.
FIGURE 2. Fractured horizontal well model. (A) fractured horizontal well model, (B) fluid flow model of horizontal wells.
The horizontal section of the horizontal well is 1,000 m long, located in the 26th row of the simulation area, and the midpoint of the horizontal section is located in the center of the simulation area. In the horizontal section, there are four pressure fractures with a length of 90 m and a half seam of 90° to the horizontal plane of the shaft, respectively in the 15th, 20th, 30th, and 35th columns. The fracture conductivity is 400 mD, and the fracture conductivity remains unchanged during drainage.
According to the previous test data, the basic geological parameters of CBM in the model are respectively assigned as follows: the initial pressure of the coal bed is 14.85 MPa, the fracture porosity is 0.95%, the initial water saturation of the fracture is 100%, the coal seam density is 1.45 t/m3, and the compressibility coefficient is 4.50 × 10−6/KPa, diffusion coefficient 1.0 × 10-9 cm2/s, Langmuir pressure 2.64 MPa, Langmuir volume 30.2 m3/m3, and coal seam vertical depth 1,650 m (Table 1).
Without considering the influence of engineering factors, the productivity of horizontal wells with gas pressure fractures in coal beds is mainly affected by three factors: single well controlled resources, seepage conditions and coal seam isothermal adsorption performance. As the adsorption performance of coal beds in the study area has little change, only single well control resources and seepage conditions need to be considered. The controlled resources of a single well are jointly affected by the gas content of the coal seam and the thickness of the coal seam. The seepage condition is the cleat fracture permeability of the coal seam.
When the simulated permeability is 0.3, 0.6, 0.9, and 1.2 mD, respectively, the fracturing horizontal well will discharge 3,000 days of gas production, coal seam pressure reduction effect, desorption gas volume and recovery factor. Permeability increased from 0.3mD to 1.2mD, and peak gas production increased from 16,450 m3/d to 21,500 m3/d (Figure 3A). From the shape of gas production curve, the higher the permeability is, the faster the gas production will decline after reaching the peak value. When the permeability is 0.3 mD, the gas production will be basically stable from the third year. When the permeability is>0.6 mD, the gas production will slowly increase from the third year. When the permeability increases from 0.3 to 1.2 mD, the cumulative gas production increases from 2,180 × 104 m3/d increased to 4,240 × 104 m3/d (Figure 3B).
FIGURE 3. Gas production curve under different permeability conditions. (A) Daily gas production curve, (B) Cumulative gas production curve.
There is a linear positive correlation between cumulative gas production and permeability, and the relationship between them is:
where, Q cumulative gas production, 104 m3; K permeability, mD.
Under the same production time, the higher the coal seam permeability is, the larger the desorption radius of coalbed methane is, and the lower the average coal seam pressure is. Taking 8 years of drainage as an example (Figure 4), the permeability increases from 0.3 to 1.2 mD, the desorption radius increases from 225 to 810 m, and the desorption area increases from 0.41 to 2.35 km2. The larger the coal seam desorption area is, the more free CBM is desorbed from the coal seam, which is more conducive to high and stable production of CBM horizontal wells. In the process of drainage and depressurization of coalbed methane horizontal wells, the higher the coal seam permeability is, the higher the pressure conductivity coefficient is, and the faster the pressure transmission is. Under the same pressure difference and production time conditions, the lower the average pressure of the coal seam is, the larger the drainage and depressurization area and desorption area are.
FIGURE 4. Plane distribution of coal seam pressure drop effect under different permeability conditions.
The higher the coal seam gas content, the higher the coal seam gas saturation and desorption pressure, and the more conducive to the high production of coal seam gas wells. Other parameters remain unchanged, and the coal seam gas content is adjusted by adjusting the desorption pressure. The gas content is respectively 20, 16, 14, and 12 m3/t for simulation. The simulation results show that (Figure 5): the gas content increases from 12 to 20 m3/t, the peak gas production increases from 11,700 to 21,500 m3/d, and the total gas production increases from 2,018 × 104 m3 increased to 3,508 × 104 m3. It can be seen that the higher the gas content, the earlier the gas production time, the higher the peak gas production, and the higher the cumulative gas production in the same production time.
FIGURE 5. Variation curve of gas production with gas content. (A) daily gas production curve, (B) cumulative gas production.
The gas content and cumulative gas production meet the relationship.
In the formula, the gas content of Gc coal seam, m3/t.
The higher the coal seam gas content is, the earlier the coal seam desorption time is, and the smaller the area of discharge and pressure reduction before desorption. After desorption, the more coalbed methane is desorbed under the same pressure conditions, the higher the gas saturation in the coal seam barrier and the higher the relative permeability of the gas phase, which is not conducive to continuous drainage and depressurization. The lower the gas content, the lower the coal seam desorption pressure and the later the desorption time. After desorption, under the same pressure conditions, the smaller the amount of desorbed coalbed methane is, the lower the gas saturation in the coal seam bedding is, the higher the relative permeability of water phase is, and the greater the pressure conductivity coefficient is, which is more conducive to the pressure reduction of coal seam drainage, and the lower the average coal reservoir pressure. Therefore, although the drainage area of the coal seam is larger and the average pressure of the coal seam is lower under the condition of low gas content, the desorption radius is larger under the condition of high gas content, and the gas saturation of the coal seam partition is higher.
When other geological parameters are the same, the thickness of coal seam is taken as 3, 4, 5, and 6 m, respectively for simulation. The simulation results show that the peak gas production, cumulative gas production and coal seam thickness are positively correlated.
When the thickness of the coal seam changes from 3 to 6 m, the peak gas production of the fractured horizontal well increases from 13,200 to 24,905 m3/d, and the cumulative gas production increases from 2,010 × 104 m3 increased to 4,180 × 104 m3.
The relationship between cumulative gas production and coal seam thickness.
In the formula, h is the coal seam thickness, m.
The greater the thickness of the coal seam, the more CBM can be desorbed from the coal seam under the same pressure drop conditions, and the higher the productivity of the CBM well (Figure 6; Figure 7).
FIGURE 6. Variation curve of gas production with coal thickness. (A) daily gas production curve, (B) cumulative gas production.
Economic benefit evaluation is the core content of horizontal well suitability evaluation. Economic evaluation indicators can be divided into two categories: first, investment value indicators, including net present value, cost limit, etc.; The second is the capital utilization efficiency index, including the rate of return, internal rate of return, etc. This paper mainly evaluates the economic feasibility of fracturing horizontal well development from two indicators: net present value and return on investment. If the net present value and return on investment of the first 8 years are greater than 0, fracturing horizontal well development technology can be adopted.
The calculation formula of NPV is:
CI refers to cash inflow, mainly including CBM sales revenue and financial subsidies; CO is cash outflow, including investment in horizontal well development, daily production and maintenance fees of horizontal well, and various taxes;
(CI − CO) is the net cash flow in year t, i benchmark discount rate; The calculation formula of return on investment is:
NPM is the net rate of return. The higher the NPM, the higher the unit investment income.
According to Formula 4 and Formula 5, respectively calculate the net present value and rate of return of fractured horizontal wells under the conditions of different permeability, gas content and coal seam thickness. When the permeability increases from 0.3 to 1.2 mD, the NPV increases from 11,000 yuan to 97,24,000 yuan. When the gas content increases from 12 m3/t to 20 m3/t, the net present value increases from −3,43,000 yuan to 75,78,000 yuan. When the coal seam thickness increases from 3 to 6 m, the net present value increases from −5,90,000 yuan to 1,03,50,000 yuan.
It can be seen that the geological conditions of CBM suitable for fracturing horizontal well development are: permeability >0.28 mD, gas content >12.8 m3/t, and coal seam thickness >3.1 m.
(1) The burial depth of coal beds in the study area is generally more than 1,500 m, which is characterized by high metamorphic degree, high gas content, high reservoir pressure and low permeability.
(2) The numerical simulation results show that the gas production of fractured horizontal wells is positively correlated with the permeability, gas content and thickness of coal beds: the higher the permeability, the higher the coal seam pressure conductivity coefficient, the better the coal seam pressure reduction effect and the larger the desorption radius after the same production time; The higher the gas content is, the earlier the coal seam desorption time is. After the same production time, the higher the average pressure of the coal seam is, the larger the desorption radius is;
(3) Combined with the economic benefit evaluation model, it is found that the lower limit of geological conditions suitable for the development of fracturing horizontal wells is: permeability >0.28 mD, gas content >12.8 m3/t, and coal seam thickness >3.1 m.
The raw data supporting the conclusion of this article will be made available by the authors, without undue reservation.
YF: Conceptualization, methodology, first draft, numerical simulation. WY: Data curation, writing-review and editing. ZX, RZ, and YW: Mapping, data processing. HZ, XW, and XG: Supervision, writing—review and editing. SG and CM: Geological data collection. All authors contributed to the manuscript.
The research was supported by the project of Sanya Yazhou Bay Science and Technology City (Grant No: SCKJ-JYRC-2022-14).
Thanks Shaanxi Tiancheng Petroleum Technology Co., Ltd. for providing geological data.
Author ZX was employed by the company Shiwen Software Co., Ltd., author YW was employed by the company PetroChina Changqing Oilfield Company and authors SG and CM were employed by the company Shaanxi Tiancheng Petroleum Technology Co., Ltd.
The remaining authors declare that the research was conducted in the absence of any commercial or financial relationships that could be construed as a potential conflict of interest.
All claims expressed in this article are solely those of the authors and do not necessarily represent those of their affiliated organizations, or those of the publisher, the editors and the reviewers. Any product that may be evaluated in this article, or claim that may be made by its manufacturer, is not guaranteed or endorsed by the publisher.
Ali, M., Paul, S., and Chatterjee, R. (2017). Cleat orientation from ground mapping and image log studies for in-situ stress analysis: Coal bed methane exploration in South Karanpura Coalfield, India. Energy & Fuels 31, 6812–6824. doi:10.1021/acs.energyfuels.7b00702
Cao, P., Liu, J., and Leong, Y. K. (2016). Combined impact of flow regimes and effective stress on the evolution of shale apparent permeability. J. Unconv. Oil Gas Resour. 14, 32–43. doi:10.1016/j.juogr.2016.01.004
Fan, Y., Deng, C., Zhang, X., Li, F., and Wang, X. (2018). Numerical study of multi-branch horizontal well coalbed methane extraction. Energy Sources, Part A Recovery, Util. Environ. Eff. 40, 1342–1350. doi:10.1080/15567036.2018.1476616
Fei, L., Luo, Z., Wang, Z., and Liu, P. (2015). Gas seepage rule and productivity analysis in designing coalbed methane wells. Chem. Technol. Fuels Oils 50, 516–524. doi:10.1007/s10553-015-0558-0
Gentzis, T. (2009). Stability analysis of a horizontal coalbed methane well in the Rocky Mountain Front Ranges of southeast British Columbia, Canada. Int. J. Coal Geol. 77 (3), 328–337. doi:10.1016/j.coal.2008.05.016
Hoang, S. K., Abousleiman, Y. N., and Al-Tahini, A. M. (2008). Multilaterals drilling and sustainable openhole production from theory to field-case studies. SPE J. 15, 878–892. doi:10.2118/116138-pa
Laubach, S. E., and Gale, J. F. W. (2006). Obtaining fracture information for low permeability (tight) gas sandstones from sidewall cores. J. Pet. Geol. 29 (2), 147–158. doi:10.1111/j.1747-5457.2006.00147.x
Li, T., Wu, C. F., and Liu, Q. (2015). Characteristics of coal fractures and the influence of coal facies on coalbed methane productivity in the South Yanchuan Block, China. J. Nat. Gas. Sci. Eng. 22, 625–632. doi:10.1016/j.jngse.2015.01.014
Liu, S., Tang, S., and Yin, S. (2018). Coalbed methane recovery from multilateral horizontal wells in Southern Qinshui Basin. Adv. Geo-Energy Res. 2, 34–42. doi:10.26804/ager.2018.01.03
Luo, C. J., Zhang, D. F., Lun, Z. M., Zhao, C. P., Wang, H. T., Pan, Z. J., et al. (2019). Displacement behaviors of adsorbed coalbed methane on coals by injection of SO2/CO2 binary mixture. Fuel 247, 356–367. doi:10.1016/j.fuel.2019.03.057
Mu, F. Y., Zhong, W. Z., Zhao, X. L., Che, C. B., Chen, Y. P., Zhu, J., et al. (2015). Strategies for the development of CBM gas industry in China. Nat. Gas. Ind. B 2 (4), 383–389. doi:10.1016/j.ngib.2015.09.013
Song, H., Cao, Y., Yu, M. X., Wang, Y., Killough, J. E., and Leung, J. (2015). Impact of permeability heterogeneity on production characteristics in water-bearing tight gas reservoirs with threshold pressure gradient. J. Nat. Gas Sci. Eng. 22, 172–181. doi:10.1016/j.jngse.2014.11.028
Su, X., Zhou, F., and Tyson, S. (2018). Numerical investigation of the potential contamination of a shallow aquifer in producing coalbed methane. Energy Explor. Exploitation 36 (2), 282–296. doi:10.1177/0144598717736629
Sun, X., Zhang, Y., Li, K., and Gai, Z. (2016). A new mathematical simulation model for gas injection enhanced coalbed methane recovery. Fuel 183, 478–488. doi:10.1016/j.fuel.2016.06.082
Thararoop, P., Karpyn, Z. T., and Ertekin, T. (2012). Development of a multi-mechanistic, dual-porosity, dual-permeability, numerical flow model for coalbed methane reservoirs. J. Nat. Gas Sci. Eng. 8, 121–131. doi:10.1016/j.jngse.2012.01.004
Xu, J. Z., Zhai, C., Ranjith, P. G., Sun, Y., and Qin, L. (2019). Petrological and ultra sonic velocity changes of coals caused by thermal cycling of liquid carbon dioxide in coalbed methane recovery. Fuel 249, 15–26. doi:10.1016/j.fuel.2019.03.089
Yan, J., Lu, Y. Y., Zhong, D. L., Zou, Z. L., and Li, J. B. (2019). Enhanced methane recovery from low-concentration coalbed methane by gas hydrate formation in graphite nanofluids. Energy 180, 728–736. doi:10.1016/j.energy.2019.05.117
Zhang, J. (2014). Numerical simulation of hydraulic fracturing coalbed methane reservoir. Fuel 136, 57–61. doi:10.1016/j.fuel.2014.07.013
Zhang, L. H., Shan, B. C., and Zhao, Y. L. (2017). Production performance laws of vertical wells by volume fracturing in CBM gas reservoirs. J. Nat. Gas. Ind. 36 (2), 36–44.
Keywords: deep CBM, fractured horizontal well, numerical simulation, geological suitability, sln block
Citation: Fu Y, Yuan W, Xu Z, Zhao R, Wu Y, Zheng H, Wang X, Guo X, Gou S and Ma C (2023) Geological adaptability of deep CBM fractured horizontal well in SLN block. Front. Earth Sci. 11:1127995. doi: 10.3389/feart.2023.1127995
Received: 20 December 2022; Accepted: 23 January 2023;
Published: 28 February 2023.
Edited by:
Yu Song, China University of Mining and Technology, ChinaReviewed by:
Shiqi Liu, China University of Mining and Technology, ChinaCopyright © 2023 Fu, Yuan, Xu, Zhao, Wu, Zheng, Wang, Guo, Gou and Ma. This is an open-access article distributed under the terms of the Creative Commons Attribution License (CC BY). The use, distribution or reproduction in other forums is permitted, provided the original author(s) and the copyright owner(s) are credited and that the original publication in this journal is cited, in accordance with accepted academic practice. No use, distribution or reproduction is permitted which does not comply with these terms.
*Correspondence: Yutong Fu, Znl0eHV6aGlkYW5AMTYzLmNvbQ==
Disclaimer: All claims expressed in this article are solely those of the authors and do not necessarily represent those of their affiliated organizations, or those of the publisher, the editors and the reviewers. Any product that may be evaluated in this article or claim that may be made by its manufacturer is not guaranteed or endorsed by the publisher.
Research integrity at Frontiers
Learn more about the work of our research integrity team to safeguard the quality of each article we publish.