- 1State Key Laboratory of Severe Weather, Chinese Academy of Meteorological Sciences, Beijing, China
- 2Collaborative Innovation Center on Forecast and Evaluation of Meteorological Disasters (CIC-FEMD), Nanjing University of Information Science and Technology, Nanjing, China
Heavy rain fell in Kyushu, Japan, in early July 2020, which caused huge life and economic losses. The present work reports that a Tibetan Plateau vortex (TPV) generated over the Tibetan Plateau transformed into a trough at the eastern edge of the Tibetan Plateau, and the trough continued to move eastward and was responsible for the heavy rainfall in Kyushu. Accordingly, the maintenance and eastward movement of the trough and the influencing mechanism of the trough on the rainfall in Kyushu are explored based on the final analysis data (FNL) from NCEP and the JRA-55 reanalysis from JMA. Diagnoses of the potential vorticity (PV) tendency equation indicate that the horizontal PV flux convergence east of the trough is the primary contributor to the eastward movement and maintenance of the trough. Furthermore, the trough is proved to have important effects on the moisture condition and the ascending motion around Kyushu. That is, the trough increases the moisture in Kyushu by intensifying the eastward moisture transportation to Kyushu; the positive vorticity and warm center, as well as the wind perturbation related to the trough, are in favor of stronger ascending motion in Kyushu. The findings in this work provide extended knowledge on the causation of rainfall in Japan, which is beneficial for further precipitation prediction.
1 Introduction
Heavy rainfall hit south Japan in early July 2020, with the precipitation in Kyushu breaking the historic record. The heavy rainfall caused severe floods and resulted in huge life and economic losses. Previous studies have revealed some crucial factors contributing to the heavy rainfall in Japan (shown as follows). Baiu fronts always appear in the Baiu season, and the mesoscale disturbances are frequently observed on Baiu fronts, which are responsible for the heavy rainfall in Japan (Mohri, 1956; Matsumoto et al., 1971; Nguyen-Le et al., 2017). The moisture transported by southerlies is important in triggering rainfall in Japan (Ogura et al., 1985; Kato, 2005; Sekizawa et al., 2019; Shimpo et al., 2019), which can be enhanced by the warm sea surface (Tsuguti and Kato, 2014; Sekizawa et al., 2019). Some rainfall events are related to depressions/tropical cyclone/extratropical cyclone activities (Krishnan and Sugi, 2001; Nguyen-Le et al., 2017), and typhoons in West Pacific always cause heavy rainfall in Japan (Misumi, 1996; Wang et al., 2009; Yoshida and Itoh, 2012; Kanada et al., 2017). In addition, upper-tropospheric troughs and jets are also important rainfall contributors (Shimpo et al., 2019; Tsuji et al., 2020; Yokoyama et al., 2020). In this work, a trough that transformed from a Tibetan Plateau vortex (TPV) at 500 hPa was found to be responsible for the heavy rainfall in Kyushu on 3–4 July 2020. Because both the TPV and trough are low-pressure systems, they are collectively called depression here.
TPVs are generated over the Tibetan Plateau at 500 hPa mainly during May to August, which are local major rainfall producers. Most of them die out in situ, but some can emigrate from the Tibetan Plateau under certain conditions and impose significant effects on the rainfall in east China. Formally, a TPV is defined as a low-pressure system which forms over the Tibetan Plateau with closed contour lines or cyclonic winds at three observation stations at 500 hPa (Lhasa Workgroup for Tibetan Plateau Meteorology Research, 1981). TPVs are shallow when located on the Tibetan Plateau, with the horizontal and vertical scales being 400–800 km and 2–3 km, respectively (Ye and Gao, 1979; Lhasa Workgroup for Tibetan Plateau Meteorology Research, 1981; Luo, 1992; Luo et al., 1994; Feng et al., 2014). After the TPVs emigrate from the Tibetan Plateau, the structures become deeper than those before moving off (Li et al., 2020a). Lots of heavy rainfalls in Southwest and East China are linked to the TPVs emigrating from the Tibetan Plateau (Yang et al., 2001; Zhang et al., 2001; Yu, 2008). Particularly, some eastward-moving TPVs can persist for several days and reach the West Pacific, which affects the rainfall in Japan (Yu and Gao, 2006).
Although our previous work revealed that the mid-upper level trough near Japan is responsible for the heavy rainfall there, the origination and evolution of the trough are not clear yet. In addition, previous studies pointed out that the TPVs emigrating from the Tibetan Plateau can affect the rainfall in Japan, but the ones not emigrating from the Tibetan Plateau are always not concerned. In the present work, a trough at the eastern edge of the Tibetan Plateau was found to be transformed from a TPV, which greatly affected the rainfall in Kyushu on 3–4 July 2020. Accordingly, the maintenance and eastward movement of the trough and its influencing mechanism on the rainfall are investigated. This work attempts to provide extended information about the causation of heavy rainfall in Kyushu, which is helpful for future precipitation prediction.
Data and methods utilized in this work are provided in Section 2. In Section 3, the rainfall which occurred in early July 2020 in Kyushu is introduced, and the maintenance and eastward movement mechanism of the trough transformed from the TPV are explored. In Section 4, the effects of the trough on the rainfall in Kyushu are investigated. Conclusions and discussions are given in Section 5.
2 Data and methods
2.1 Data
The final operational global analysis (FNL) data are used to investigate the large-scale circulations and the evolution of depression originating over the Tibetan Plateau, which is derived from the Global Forecasting System of the National Centers for Environment Prediction (NCEP) (http://rda.ucar.edu/datasets/ds083.2, ds083.2|DOI: 10.5065/D6M043C6). The FNL data are at a 6 h interval and cover globally with a 1°×1° horizontal resolution. The reliability of the FNL data in researching the circulations related to the depressions over the Tibetan Plateau has been verified in our previous work (Li et al., 2014a; Li et al., 2020b). Because the FNL data start from July 1999, the climatic means are taken as the averages between 2000 and 2019. Accordingly, anomalies of the variables (e.g., geopotential height, zonal and meridional winds, moisture flux, etc.) are calculated as differences between the original data and the climatic means. The 6-hourly JRA-55 precipitation data provided by Japan Meteorological Agency (https://jra.kishou.go.jp/JRA-55/) are used in this work, which performs well in displaying extreme rainfall events in East Asia (Kim et al., 2019).
2.2 Trough-centered composites
Because the trough transformed from the TPV is a moving system, composites of the variables at different times in geographic coordinates will be greatly smoothed. To exhibit the features of the trough, the trough-centered composites are conducted. That is, at a specific time, the center of the trough (maximum of the positive vertical vorticity at 500 hPa in the trough area) is taken as the origin of the coordinates, and then, the variables at different times are composed. Thus, the composed center of the trough is the origin of the coordinates, and the coordinates on the x-axis (y-axis) are the relative coordinates from the composed center of the trough in the zonal (meridional) direction.
2.3 Potential vorticity (PV) tendency equation and quasi-geostrophic equation
PV budgets analyses can well-interpret the evolution of TPVs (Li et al., 2011; Zheng et al., 2013; Li et al., 2014b), which is conducted in this work to investigate the maintenance and eastward movement mechanism of the trough influencing the rainfall in Kyushu. PV tendency equation without frictional effects is shown in Eq. 1 (Li et al., 2017). Because the trough is accompanied by rainfall during its eastward movement, Q in Eq. 1 is calculated as the condensational latent heating. The formula of the condensational latent heating rate and the meanings of variables and each term can be found in the study by Li et al. (2017).
Additionally, given that the ascending motion is a key factor in triggering the rainfall, the adiabatic quasi-geostrophic
3 Introduction of the rainfall event in Kyushu in early July 2020
3.1 Rainfall
The 6-hourly precipitation averaged between 31°N and 34°N, covering the meridional range of Kyushu, is displayed by the contours in Figure 1. The rainfall centers are observed to propagate eastward between late June and early July, from 100°E to 135°E. The precipitation was weak when falling on and near the Tibetan Plateau and became stronger in central and east China. The rainfall in Kyushu persisted from 12 UTC 02 July to 00 UTC 04 July; however, only the rainfall episode between 00 UTC 03 July and 00 UTC 04 July is related to the rainband stretching from the Tibetan Plateau. Because this work aims to reveal the effect of the eastward-moving depression that originated over the Tibetan Plateau, the rainfall episode from 00 UTC 03 July to 00 UTC 04 July is of primary concern. To further demonstrate the relationship between the rainfall in Kyushu and the depression originating over the Tibetan Plateau, the vertical vorticity at 500 hPa averaged between 28°N and 38°N, which covers the meridional range of the Tibetan Plateau, is represented by shadings in Figure 1. A TPV was generated at 12 UTC 30 June at around 100°E over the Tibetan Plateau, which moved eastward and arrived at 102°E at 18 UTC 30 June. The TPV weakened when reaching the eastern edge of the Tibetan Plateau and then transformed into a trough. The trough emigrated from the Tibetan Plateau and continued to move eastward. The eastward movement of TPV and the related trough corresponds to the eastward extension of the positive vorticity and the eastward-propagating rainfall centers shown in Figure 1, indicating that the depression emigrating from the Tibetan Plateau has a close relationship with the rainfall in Kyushu. Additionally, the rainfall at each time is generally located east of the positive vorticity, which implies that the rainfall usually happens east of the depression.
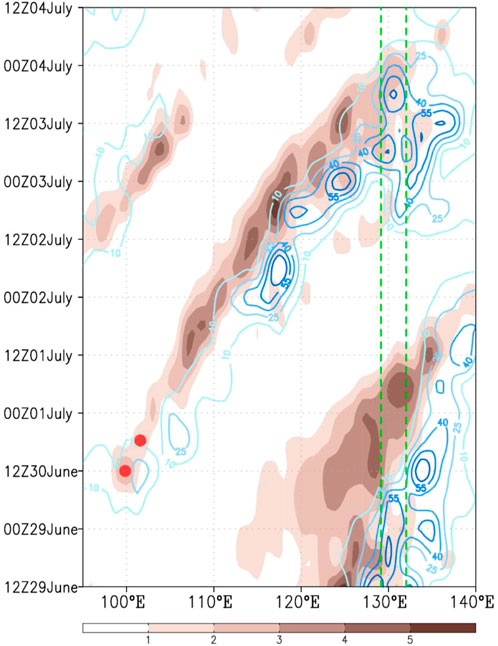
FIGURE 1. Time-longitude sections of 6-hourly precipitation averaged between 31°N and 34°N (contours; unit: mm day−1) and 500 hPa vertical vorticity averaged between 28°N and 38°N (shadings; unit: 10−5 s−1). The green dotted lines indicate the zonal range of Kyushu, and the red dots represent the zonal positions of the TPV.
Temporal variation and spatial distribution of the precipitation in Kyushu are shown in Figure 2. Figure 1 reveals that the rainfall episode related to the depression over the Tibetan Plateau was from 00 UTC 03 July to 00 UTC 04 July. As shown in Figure 2A, the precipitation became stronger after 00 UTC 03 July, with the peaks appearing at 06 UTC 03 July and 18 UTC 03 July; after 00 UTC 04 July, the precipitation weakened sharply. The average 6-hourly precipitation from 00 UTC 03 July to 00 UTC 04 July was distributed from southwest to northeast and covered wide areas of Japan, with the maximum being in south Kyushu.
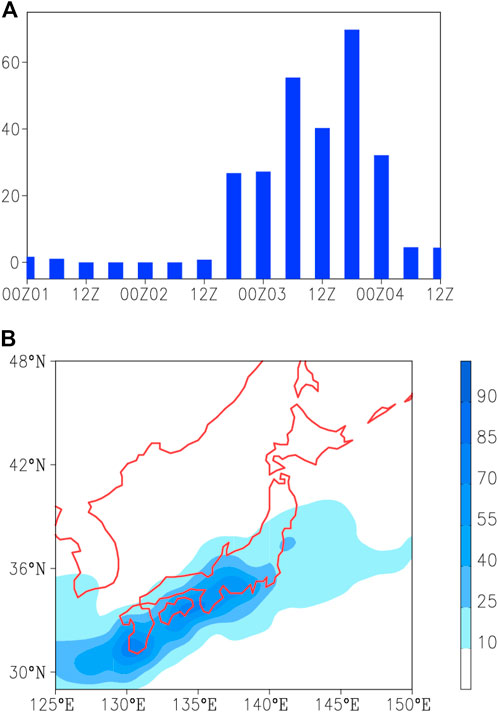
FIGURE 2. (A) Temporal variation of the 6-hourly precipitation averaged in Kyushu (129–132°E, 31–34°N) (unit: mm day−1), and (B) spatial distribution of the average 6-hourly precipitation from 00 UTC 03 July to 00 UTC 04 July (unit: mm day−1).
3.2 Evolution of the depression that originated over the Tibetan Plateau
Geopotential height and wind at 500 hPa are shown in Figure 3. At 06 UTC on 30 June, there were strong westerlies north of the Tibetan Plateau, and no obvious depression was observed at this time. At 12 UTC on 30 June, the westerlies north of the plateau branched into two flows, with one turning into northerlies and the other remaining westerlies. Cyclonic shear is formed by the northerlies and southerlies over the eastern Tibetan Plateau, and accordingly, a TPV was generated at 100°E, 33°N. Similar to the previously revealed genesis mechanism of TPVs (Li et al., 2011, 2014a; Ma et al., 2022; Wu et al., 2022), both the cyclonic wind convergence and the condensational latent heating favor the genesis of the TPV, and the vertically uneven heating plays as a dominant role in the genesis process of this TPV, based on the calculations on PV tendency equation (figure not shown). The surface sensible heating can produce favorable early conditions for the later condensational latent heat release, which also has an important but indirect effect on the genesis of TPVs (Dell’Osso and Chen, 1986; Wang, 1987; Li et al., 2014a; Zhang et al., 2019). At the following time (18 UTC 30 June), the TPV moved eastward and was located at 102°E, 33°N. At 00 UTC 01 July, the westerlies north of the TPV strengthened, which was unfavorable for the maintenance of the TPV’s close cyclonic circulation. Thus, the TPV transformed into a trough at 00 UTC on 01 July, which continues to move eastward. The trough transformed from the TPV was relatively weak when it was near the eastern edge of the Tibetan Plateau (at 00 UTC 01 July and 06 UTC 01 July) and became stronger after 12 UTC 01 July (Figure 1), which is similar to the evolution of a TPV emigrating from the Tibetan Plateau (Li et al., 2014b). At 00 UTC 03 July (Figure 3G), the trough was located at the eastern boundary of China, which started affecting the rainfall in Japan. It is of note that the activities of the trough and the related circulations are not provided here, but are introduced in detail in Section 4.
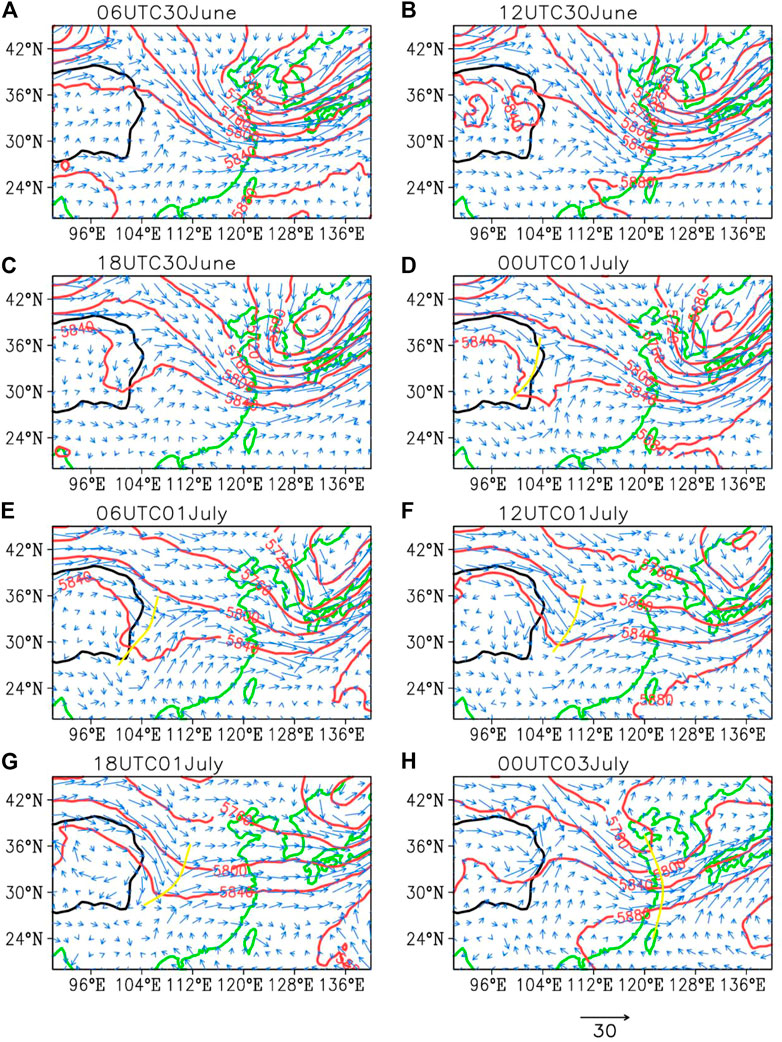
FIGURE 3. Geopotential height (red contours; unit: gpm) and wind (vectors; unit: m s−1) at 500 hPa. (A) 06UTC30June, (B) 12UTC30June, (C) 18UTC30June, (D) 00UTC01July, (E) 06UTC01July, (F) 12UTC01July, (G) 18UTC01July, and (H) 00UTC03July. The yellow curves in (D–G) represent the trough. The thick black lines are the topographic contours of 3,000 m, indicating the border of the Tibetan Plateau.
Although the evolution mechanisms of the TPVs are analyzed in our previous work, and the convergence east of the TPVs is considered the primary factor dominating the evolution of TPVs after they emigrate from the Tibetan Plateau (Li et al., 2019), the maintenance and eastward movement mechanism of the trough originated over the Tibetan Plateau is not clear yet. Therefore, the evolution mechanism of the trough is investigated by diagnosing the PV tendency equation. The 500 hPa PV tendencies east of the trough induced by the horizontal PV flux divergence, the vertical PV flux divergence, and the condensational latent heat, after the trough emigrates from the Tibetan Plateau, are presented in Figure 4A. The horizontal PV convergence and condensational latent heat have positive effects on the increase in PV east of the trough, and the former is obviously larger than the latter. Meanwhile, because of the strong ascending motion around the trough, negative PV tendency induced by the vertical PV divergence is found east of the trough, which is unfavorable for the maintenance and eastward movement of the trough. Thus, the horizontal PV flux convergence contributes the most to the positive PV tendency among these three terms, indicating that the maintenance and eastward movement of the trough is mainly supported by the horizontal PV flux convergence to its east. Actually, the horizontal PV flux convergence contributes to the enhancement of the trough at 12 UTC on 01 July. To further confirm the role of the horizontal PV flux divergence, its spatial distribution and that of the net PV tendency induced by the three terms are shown in Figure 4B. Positive net PV tendency is observed east and northeast of the trough, which corresponds to the general eastward moving direction with a slight northeastward shift. Moreover, the distribution of the horizontal PV flux divergence coincides well with that of the net PV tendency, implying the dominant role of the former. Therefore, horizontal PV flux convergence east of the trough, which is induced by both the PV advection and the wind convergence there, plays a very important role in the maintenance and eastward movement of the trough.
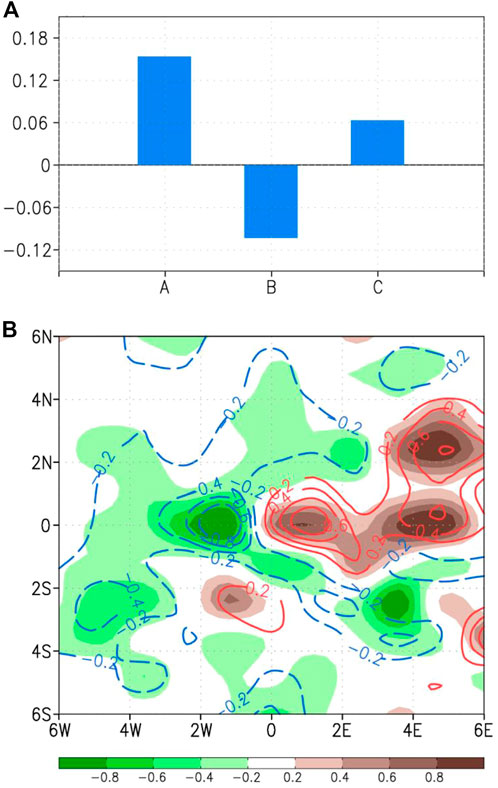
FIGURE 4. (A) PV tendencies (bars; unit: PVU (6 h)−1, 1PVU=10−6 m2 s−1 K kg−1) induced by horizontal PV flux divergence (A), vertical PV flux divergence (B) and condensational latent heat (C), averaged in the region east of the trough (0°–4° in zonal direction, −2°–2° in meridional direction) after the trough emigrates from the Tibetan Plateau (00 UTC 01 July–00 UTC 04 July). (B) Net PV tendency induced by the three terms (shadings; unit: PVU (6 h)−1) and the PV tendency induced by horizontal PV flux divergence (contours; unit: PVU (6 h)−1). Coordinates on the x-axis (y-axis) in (B) are the relative coordinates from the composed center of the trough in the zonal (meridional) direction.
4 Effects of the trough on the rainfall in Kyushu
To highlight the activities of the trough that originated over the Tibetan Plateau when it approached Japan, the anomalies of geopotential height and wind at 500 hPa from 06 UTC 03 July to 00 UTC 04 July are shown in Figure 5. We found that the high north of the trough was stronger than the climatic mean state, whose anomaly easterlies were beneficial for the maintenance of the cyclonic system in the south from 06 UTC 03 July to 00 UTC 04 July. Kyushu was located southeast of the trough before 18 UTC 03 July and south of the trough at 00 UTC 04 July. Accordingly, Kyushu was affected by the southwesterlies associated with the trough in the former period and by the northwesterlies in the latter. Locations of the trough from 06 UTC 03 July to 00 UTC 04 July are 122°E, 35°N, 125°E, 36°N, 128°E, 36°N, and 130°E, 37°N, respectively.
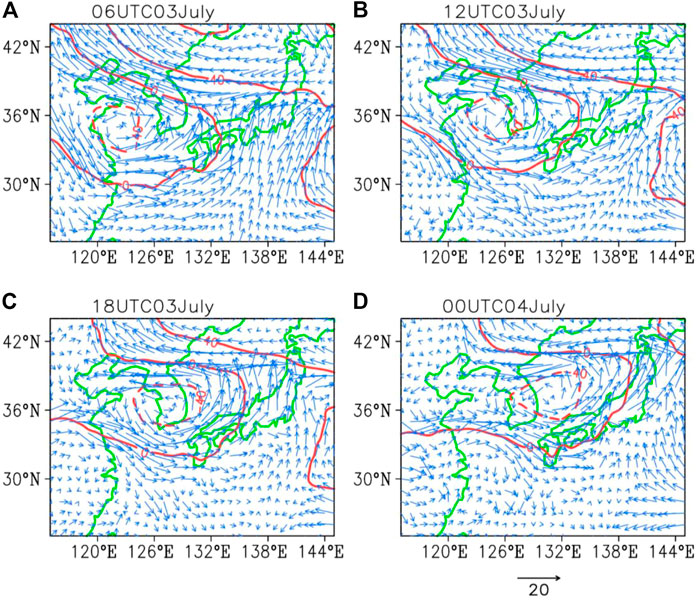
FIGURE 5. Same as Figure 3, but for anomaly geopotential height (red contours; unit: gpm) and wind (vectors; unit: m s−1) at 500 hPa from 06 UTC 03 July to 00 UTC 04 July. (A) 06UTC03July, (B) 12UTC03July, (C) 18UTC03July, and (D) 00UTC04July.
Given that the moisture condition and vertical motion are crucial to trigger rainfall, the effects of the trough on these two factors are investigated in the following text. The moisture condition is analyzed first.
The anomalies of vertically integrated (from surface to 100 hPa) moisture flux and its divergence in the rainfall episode from 00 UTC 03 July to 00 UTC 04 July are presented in Figure 6. The moisture converged in Kyushu, which is greatly attributed to the moisture transported by the westerlies west of Kyushu. According to the circulations at 500 hPa shown in Figure 5, strong westerly anomalies were observed south of the trough, which greatly contributed to the eastward transportation of the moisture. To further confirm the role of anomaly westerlies associated with the trough in transporting the moisture, time series of the anomaly vertically integrated zonal moisture flux and speed of westerlies at the western boundary of Kyushu (approximately 129°E) averaged between 30°N and 35°N are shown in Figure 7. It is found that the moisture transported through the western boundary of Kyushu increased sharply at 06 UTC 03 July, corresponding to the robust increase in westerly speed at this time. Actually, the westerlies associated with the trough in Kyushu were apparently stronger at 06 UTC 03 July than those at 00 UTC 03 July because the trough was located farther from Kyushu at the latter time. The eastward transported moisture increased during the following times, reached the peak at 18 UTC 03 July, and then decreased at 00 UTC 04 July. Variation of the moisture transported by the westerlies generally coincides with that of the westerly speed and the precipitation in Kyushu, indicating that the trough that originated over the Tibetan Plateau contributed to the favorable moisture condition for the rainfall in Kyushu.
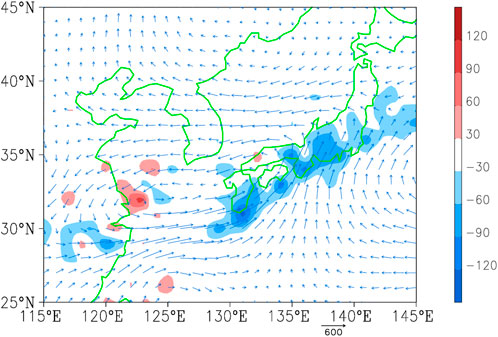
FIGURE 6. Vertical integrated anomaly moisture flux (vectors; unit: kg m−1 s−1) and moisture flux divergence (shadings; unit: 10−5 kg m−2 s−1), averaged between 00 UTC 03 July and 00 UTC 04 July.
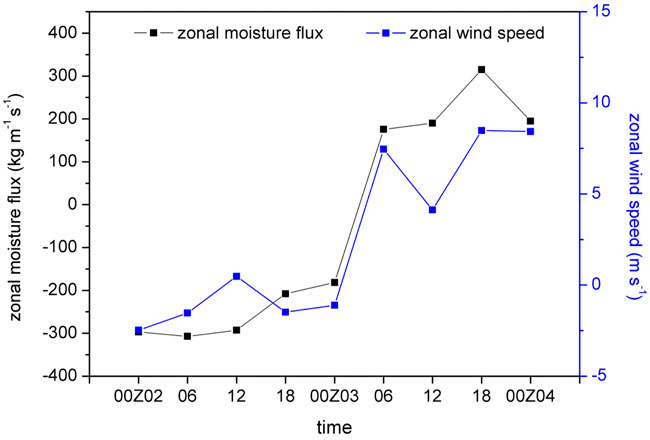
FIGURE 7. Average vertically integrated zonal moisture flux (black line; unit: kg m−1 s−1) and 500-hPa zonal wind speed (blue line; unit: m s-1) anomalies at 129°E (approximately the most west longitude of Kyushu) between 30°N and 35°N.
Second, the effect of the trough on the vertical motion in the region around Kyushu is explored. The anomaly vertical velocity at 500 hPa averaged in 127°E−135°E, 30°N−35°N, which includes Kyushu, is shown in Figure 8A to demonstrate the variation of the vertical motion there under the influence of the eastward-moving trough. The ascending motion strengthened during the eastward movement of the trough, was the strongest at 18 UTC 03 July, and then weakened sharply at 00 UTC 04 July. Actually, the ascending motion associated with the trough appeared on its east and southeast sides, and the descending motion on its west side (Figure 8B). Because Kyushu was located south of the trough at 00 UTC 04 July and southeast at the previous times, the ascending motion in Kyushu at 00 UTC 04 July was much weaker than that at the previous times. According to the aforementioned analyses, the ascending motion in the region around Kyushu is closely related to the eastward movement of the trough. Furthermore, the variation of the vertical motion coincides well with the precipitation variation from 00 UTC 03 July to 00 UTC 04 July, which further verifies the significant impact of the trough on the rainfall in Kyushu by changing the vertical motion there.
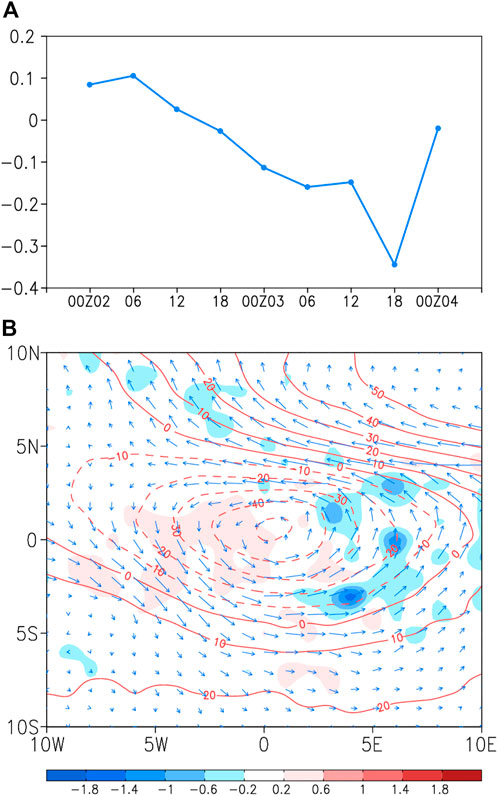
FIGURE 8. (A) Time series of anomaly vertical velocity at 500 hPa averaged in 127–132°E, 30–35°N (unit: Pa s−1). (B) Trough-centered composites of anomaly geopotential height (contours; unit: gpm), vertical velocity (shadings; unit: s−1), and wind (vectors; unit: m s−1) at 500 hPa from 00 UTC 03 July to 00 UTC 04 July. Coordinates on the x-axis (y-axis) in (B) are the relative coordinates from the composed center of the trough in the zonal (meridional) direction.
The quasi-geostrophic
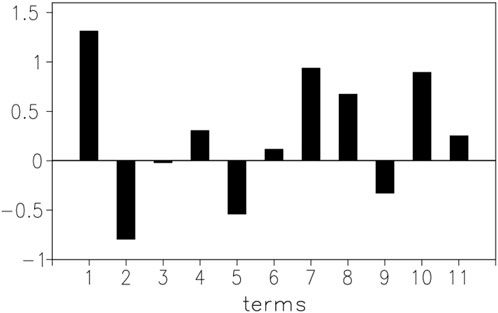
FIGURE 9. Terms 1–11 in Eq. 2 averaged in 127–132°E, 30–35°N from 00 UTC 03 July to 00 UTC 04 July (unit: 10−18 m s−1 kg−1).
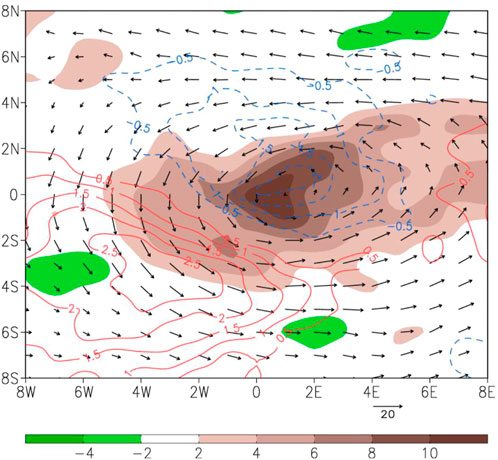
FIGURE 10. Trough-centered composites of vorticity perturbation (shadings; unit: 10−5 s−1), temperature perturbation (contours; unit: K), and wind perturbation (vectors; unit: m s−1) at 500 hPa. Coordinates on the x-axis (y-axis) are the relative coordinates from the composed center of the trough in the zonal (meridional) direction.
In a word, the trough that originated over the Tibetan Plateau enhanced the rainfall in Kyushu on 3–4 July 2020 by intensifying the moisture transportation to Kyushu and the ascending motion there.
5 Conclusion
Heavy rainfall affected Kyushu in early July 2020, causing huge damage and life and economic losses. The rainfall episode on 3–4 July 2020 is found to be greatly related to the Tibetan Plateau vortex (TPV) over the Tibetan Plateau, which transformed into a trough near the eastern edge of the Tibetan Plateau. This work analyzes the maintenance and eastward movement mechanism of the trough transformed from the TPV and further investigates the effects of the trough on the rainfall in Kyushu. The results are beneficial for understanding the causation of the rainfall in Kyushu and helpful for future precipitation prediction in this region. The results are summarized as follows:
A TPV is generated over the eastern Tibetan Plateau and transformed into a trough at the eastern edge of the Tibetan Plateau. The trough transformed from the TPV continues to move eastward and affects the rainfall in Kyushu when it approaches Japan in the zonal direction. The eastward movement and maintenance mechanism of the trough emigrating from the Tibetan Plateau are subsequently investigated. It is found that the horizontal PV flux convergence and condensational latent heat east of the trough are beneficial for its eastward movement and maintenance, and the contribution of the former is apparently larger than that of the latter.
The trough that originated over the Tibetan Plateau enhances the rainfall by increasing the moisture transportation and intensifying the ascending motion in Kyushu. First, there are anomaly westerlies south of the trough, which enhances the zonal moisture transportation to Kyushu. Generally, variation of the zonal moisture flux at the western boundary of Kyushu coincides well with that of the zonal wind speed, indicating the important role of the trough in producing favorable moisture condition for the rainfall in Kyushu. Second, the ascending motion in Kyushu becomes stronger when the trough is zonally approaching Kyushu, indicating that the vertical motion in Kyushu is closely related to the eastward-moving trough. The influencing mechanism of the trough on the vertical motion in Kyushu is further explored by diagnosing the quasi-geostrophic
It should be noted that there were several rainfall episodes in Kyushu in early July 2020, and we only selected the one during 3–4 July 2020 that was affected by the trough that originated over the Tibetan Plateau. Additionally, the rainfall in Kyushu is the result of the coaction of multi-scale circulations. Although the trough emigrating from the Tibetan Plateau plays an important role in the rainfall in Kyushu, it is just part of the causations. This work aims to reveal the effect of the trough that originated over the Tibetan Plateau; thus, the roles of the other weather systems are not discussed here. Moreover, understanding the quantitative contribution of the trough to the record-breaking rainfall is important to understand the effect of the trough, which is not involved in the present work but is worthy of further investigation by conducting numerical experiments in future works.
Data availability statement
The original contributions presented in the study are included in the article/Supplementary Material; further inquiries can be directed to the corresponding author.
Author contributions
LL and CZ designed the manuscript, and LL and HH wrote the draft.
Funding
This work was supported by the Second Tibetan Plateau Scientific Expedition and Research (STEP) program (Grant No. 2019QZKK0105), Heavy Rain and Drought–Flood Disasters in Plateau and Basin Key Laboratory of Sichuan Province (Grant No. SZKT202202), and Basic Scientific Research and Operation Foundation of CAMS (Grant No. 2021Z007).
Acknowledgments
FNL data can be obtained at http://rda.ucar.edu/datasets/ds083.2. 6-hourly precipitation data are provided by the Japan Meteorological Agency at https://jra.kishou.go.jp/JRA-55/.
Conflict of interest
The authors declare that the research was conducted in the absence of any commercial or financial relationships that could be construed as a potential conflict of interest.
Publisher’s note
All claims expressed in this article are solely those of the authors and do not necessarily represent those of their affiliated organizations, or those of the publisher, the editors, and the reviewers. Any product that may be evaluated in this article, or claim that may be made by its manufacturer, is not guaranteed or endorsed by the publisher.
References
Dell’Osso, L., and Chen, S. J. (1986). Numerical experiments on the Genesis of vortices over the qinghai-xizang plateau. Tellus 38 (A), 235–250.
Feng, X. Y., Liu, H. C., Rusmussen, R., and Fan, G. Z. (2014). A 10-yr climatology of Tibetan plateau vortices with NCEP climate forecast system reanalysis. J. Appl. Meteor. Climatol. 53, 34. doi:10.1175/JAMC-D-13-014.1
Kanada, S., Tsuboki, K., Aiki, H., Tsujino, S., and Takayabu, I. (2017). Future enhancement of heavy rainfall events associated with a typhoon in the midlatitude regions. SOLA 13, 246–251. doi:10.2151/sola.2017-045
Kato, T. (2005). Statistical study of band-shaped rainfall systems, the Koshikijima and Nagasaki lines, observed around Kyushu island, Japan. J. Meteor. Soc. Jpn. 83, 943–957. doi:10.2151/jmsj.83.943
Kim, I., Oh, J., Woo, S., and Kripalani, R. H. (2019). Evaluation of precipitation extremes over the asian domain: Observation and modelling studies. Clim. Dyn. 52, 1317–1342. doi:10.1007/s00382-018-4193-4
Kosaka, Y., Xie, S., and Nakamura, H. (2011). Dynamics of interannual variability in summer precipitation over East Asia. J. Clim. 24, 5435–5453. doi:10.1175/2011JCLI4099.1
Krishnan, R., and Sugi, M. (2001). Baiu rainfall variability and associated monsoon teleconnections. J. Meteor. Soc. Jpn. 79, 851–860. doi:10.2151/jmsj.79.851
Lhasa group for Tibetan Plateau meteorology research (1981). Research of 500 hPa vortices and shear lines over the Tibetan plateau in summer. Beijing: Science Press. (in Chinese).
Li, L., Zhang, R. H., and Wen, M. (2011). Diagnostic analysis of the evolution mechanism for a vortex over the Tibetan Plateau in June 2008. Adv. Atmos. Sci. 28, 797–808. doi:10.1007/s00376-010-0027-y
Li, L., Zhang, R. H., and Wen, M. (2014a). Diurnal variation in the occurrence frequency of the Tibetan Plateau vortices. Meteorology Atmos. Phys. 125, 135–144. doi:10.1007/s00703-014-0325-5
Li, L., Zhang, R. H., Wen, M., and Duan, J. (2019). Development and eastward movement mechanisms of the Tibetan Plateau vortices moving off the Tibetan Plateau. Clim. Dyn. 52, 4849–4859. doi:10.1007/s00382-018-4420-z
Li, L., Zhang, R. H., and Wen, M. (2017). Genesis of southwest vortices and its relation to Tibetan Plateau vortices. Q. J. R. Meteor. Soc. 143, 2556–2566. doi:10.1002/qj.3106
Li, L., Zhang, R. H., Wen, M., and Liu, L. (2014b). Effect of the atmospheric heat source on the development and eastward movement of the Tibetan plateau vortices. Tellus A 66, 24451. doi:10.3402/tellusa.v66.24451
Li, L., Zhang, R. H., and Wen, M. (2020a). Structure characteristics of the vortices moving off the Tibetan Plateau. Meteorol. Atmos. Phys. 132, 19–34. doi:10.1007/s00703-019-00670-z
Li, L., Zhang, R. H., and Wu, P. L. (2020b). Evaluation of NCEP-FNL and ERA-interim data sets in detecting Tibetan plateau vortices in may–august of 2000–2015. Earth Space Sci. 7, e2019EA000907. doi:10.1029/2019EA000907
Luo, S. W., He, M. L., and Liu, X. D. (1994). Study on the vortex of the qinghai-xizang (tibet) plateau in summer. Sci. China Ser B 37, 601–612. (in Chinese). doi:10.1007/BF00717371
Luo, S. W. (1992). Study on some kinds of weather systems over and around the qinghai-xizang plateau. Beijing: China Meteorological Press. (in Chinese).
Ma, T. T., Wu, G. X., Liu, Y. M., and Mao, J. Y. (2022). Abnormal warm sea-surface temperature in the Indian Ocean, active potential vorticity over the Tibetan Plateau, and severe flooding along the Yangtze River in summer 2020. Q. J. R. Meteorological Soc. 148, 1001–1019. doi:10.1002/qj.4243
Matsumoto, S., Ninomiya, K., and Yoshizumi, S. (1971). Characteristic features of “Baiu” front associated with heavy rainfall. J. Meteor. Soc. Jpn. 49, 267–281. doi:10.2151/jmsj1965.49.4_267
Misumi, R. (1996). A study of the heavy rainfall over the ohsumi peninsula (Japan) caused by typhoon 9307. J. Meteor. Soc. Jpn. 74, 101–113. doi:10.2151/jmsj1965.74.1_101
Mohri, K. (1956). An aerological study of heavy rainfall over west and central Japan during early summer 1953. J. Meteor. Soc. Jpn. 34, 244–253. doi:10.2151/jmsj1923.34.5_244
Nguyen-Le, D., Yamada, T. J., and Tran-Anh, D. (2017). Classification and forecast of heavy rainfall in northern Kyushu during Baiu season using weather pattern recognition. Atmos. Sci. Lett. 18, 324–329. doi:10.1002/asl.759
Ogura, Y., Asai, T., and Dohi, K. (1985). A case study of a heavy precipitation event along the Baiu front in northern Kyushu, 23 july 1982: Nagasaki heavy rainfall. J. Meteor. Soc. Jpn. 63, 883–900. doi:10.2151/jmsj1965.63.5_883
Sekizawa, S., Miyasaka, T., Nakamura, H., Shimpo, A., Takemura, K., and Maeda, S. (2019). Anomalous moisture transport and oceanic evaporation during a torrential rainfall event over western Japan in early july 2018. SOLA 15A, 25–30. doi:10.2151/sola.15A-005
Shimpo, A., Takemura, K., Wakamatsu, S., Togawa, H., Mochizuki, Y., Takekawa, M., et al. (2019). Primary factors behind the heavy Rain event of july 2018 and the subsequent heat wave in Japan. SOLA 15A, 13–18. doi:10.2151/sola.15A-003
Tam, C. Y., and Li, T. (2006). The origin and dispersion characteristics of the observed tropical summertime synoptic-scale waves over the western pacific*. Mon. Wea. Rev. 134, 1630–1646. doi:10.1175/MWR3147.1
Tsuguti, H., and Kato, T. (2014). Contributing factors of the heavy rainfall event at amami-oshima island, Japan, on 20 october 2010. J. Meteor. Soc. Jpn. 92, 163–183. doi:10.2151/jmsj.2014-202
Tsuji, H., Yokoyama, C., and Takayabu, Y. N. (2020). Contrasting features of the july 2018 heavy rainfall event and the 2017 northern Kyushu rainfall event in Japan. J. Meteor. Soc. Jpn. 98, 859–876. doi:10.2151/jmsj.2020-045
Wang, B. (1987). The development mechanism for Tibetan Plateau warm vortices. J. Atmos. Sci. 44, 2978–2994. doi:10.1175/1520-0469(1987)044<2978:tdmftp>2.0.co;2
Wang, Y. Q., Wang, Y. Q., and Fudeyasu, H. (2009). The role of typhoon songda (2004) in producing distantly located heavy rainfall in Japan. Mon. Weather Rev. 137, 3699–3716. doi:10.1175/2009MWR2933.1
Wu, G. X., Tang, Y. Q., He, B., Liu, Y. M., Mao, J. Y., Ma, T. T., et al. (2022). Potential vorticity perspective of the Genesis of a Tibetan Plateau vortex in June 2016. Clim. Dyn. 58, 3351–3367. doi:10.1007/s00382-021-06102-2
Yang, K. M., Bi, B. G., Li, Y. A., and Dong, L. Q. (2001). On flood-causing torrential rainfall in the upstream distrct of Changjiang River in 1998. Meteorol. Mon. 27, 9–14. (in Chinese).
Ye, D. Z., and Gao, Y. X. (1979). The Tibetan Plateau Meteorology. Beijing: Science Press. (in Chinese).
Yokoyama, C., Tsuji, H., and Takayabu, Y. N. (2020). The effects of an upper-tropospheric trough on the heavy rainfall event in july 2018 over Japan. J. Meteor. Soc. Jpn. 98, 235–255. doi:10.2151/jmsj.2020-013
Yoshida, K., and Itoh, H. (2012). Indirect effects of tropical cyclones on heavy rainfall events in Kyushu, Japan, during the Baiu season. J. Meteor. Soc. Jpn. 90, 377–401. doi:10.2151/jmsj.2012-303
Yu, S. H., and Gao, W. L. (2006). Observational analysis on the movement of vortices before/after moving out the Tibetan Plateau. Acta Meteor. Sin. 64, 392–399. (in Chinese). doi:10.3321/j.issn:0577-6619.2006.03.014
Yu, S. H. (2008). New research advances of the Tibetan Plateau vortex in summer. Torrential Rain Disasters 27, 367–372. (in Chinese).
Zhang, F. M., Wang, C. H., and Pu, Z. X. (2019). Genesis of Tibetan Plateau vortex: Roles of surface diabatic and atmospheric condensational latent heating. J. Appl. Meteorology Climatol. 58, 2633–2651. doi:10.1175/jamc-d-19-0103.1
Keywords: Tibetan Plateau vortex, trough, eastward movement, moisture, ascending motion
Citation: Li L, Huang H and Zhu C (2023) Causation of the heavy rainfall in Kyushu in early July 2020: A perspective of the depression that originated over the Tibetan Plateau. Front. Earth Sci. 11:1127299. doi: 10.3389/feart.2023.1127299
Received: 19 December 2022; Accepted: 26 January 2023;
Published: 15 February 2023.
Edited by:
Chenghai Wang, Lanzhou University, ChinaCopyright © 2023 Li, Huang and Zhu. This is an open-access article distributed under the terms of the Creative Commons Attribution License (CC BY). The use, distribution or reproduction in other forums is permitted, provided the original author(s) and the copyright owner(s) are credited and that the original publication in this journal is cited, in accordance with accepted academic practice. No use, distribution or reproduction is permitted which does not comply with these terms.
*Correspondence: Lun Li, bGlsdW5AY21hLmdvdi5jbg==