Corrigendum: Mantle structure beneath the Macaronesian volcanic islands (Cape Verde, Canaries, Madeira and Azores): a review and future directions
- 1Institut de Ciències del Mar, ICM-CSIC, Barcelona, Spain
- 2Instituto Dom Luiz (IDL), Faculdade de Ciências, Universidade de Lisboa, Lisboa, Portugal
- 3Instituto Superior de Engenharia de Lisboa (ISEL), Instituto Politécnico de Lisboa, Lisboa, Portugal
Ocean island volcanism provides a unique window into the nature of mantle composition, dynamics and evolution. The four Macaronesian archipelagos–Cape Verde, the Canaries, Madeira and the Azores–are the main magmatic systems of the Central-East Atlantic Ocean with volcanic activity that in some islands poses significant risk for the population. The recent development of regional seismic networks in these settings has provided an important step forward in mapping the underlying mantle. However, difficulties in resolving the small-scale structure with geophysical techniques persist leading to discrepancies in the interpretation of the mechanisms responsible for volcanism. Here we review results from a number of studies on the seismic mantle structure beneath the Macaronesian archipelagos including seismic tomography, receiver functions, precursors and shear-wave splitting. Several regional models show low-velocity features in the asthenosphere below the islands, a relatively thinned transition zone and complex anisotropic patterns and attribute the volcanism to mantle plumes. This inference is supported by whole-mantle tomography models, which find broad low-velocity anomalies in the lower mantle below the Central-East Atlantic. Other models call for alternative mechanisms associated with shallower mantle upwellings and purely plate tectonism. Thus, there is still no generally accepted mechanism that explains volcanism in the Macaronesia region. Future research requires improvements in the resolving power of seismic techniques to better illuminate the velocity structure at a much higher resolution than the currently achieved and ultimately define the mechanisms controlling the ocean island volcanism.
1 Introduction
Oceanic island volcanism provides important insights regarding mantle composition, magma formation processes and evolution. Originally, a hot columnar plume rising from the lower mantle has been proposed to support the generation of magma in Hawaii and then applied to other volcanic islands (Morgan, 1972). The surface expression of this type of upwelling, i.e., ‘hotspot volcanism’, can explain many observations such as topographic swells, long-lived linear age-progressive volcanism and distinct radiogenic isotope characteristics of the rocks.
Nevertheless, several cases of ‘non-hotspot volcanism’ exist (e.g., Batiza, 1982; McNutt, 1998; Anderson, 2000). For example, some archipelagos display non-linear age-distance relationships or short-lived volcano chains and call for alternative mechanisms including small-scale convection (Raddick et al., 2002; Ballmer et al., 2007; Manjón-Cabeza Córdoba and Ballmer, 2020), shear-driven upwelling (Conrad et al., 2010; Ballmer et al., 2013), tensional fractures (Anderson, 2000; Stuart et al., 2007) and vertical motion of the lithosphere (e.g., Slater et al., 1998; Bianco et al., 2005).
One key challenge in distinguishing between these mechanisms is to image in high resolution the seismic structure below the volcanic islands. To achieve this, a wide and dense coverage of seismic stations and high-quality recordings (not much contaminated by noise) are needed. However, these requirements are difficult to attain in oceanic environments due to the configuration of the islands, the non-ideal source-receiver geometry and the effect of the water layer on the signal (e.g., Lees, 2007; Spieker et al., 2018; Carvalho et al., 2022).
The Central-East Atlantic Ocean hosts several volcanic islands and seamounts that interrupt the regular seafloor, such as Cape Verde, Canary, Madeira, and Azores archipelagos (Figure 1). These four archipelagos are commonly known as Macaronesian islands, coming from the Greek for ‘islands of the fortunate’. Recent improvements in seismic imaging and computing resources have supported new concepts for the origin of these islands marking significant advances in our understanding of geodynamic mechanisms that sustain such ocean island volcanism. However, many unanswered questions still exist: a) are the lithospheric processes controlling the location of the Central-East Atlantic volcanism or is this volcanism fed by mantle upwellings which are independent of the lithosphere?; b) where do the specific mechanisms that sustain the volcanically active Macaronesian islands lie at depth?; and c) do Cape Verde-Canaries-Madeira-Azores represent a single system, i.e., a plume rising from deep mantle which splits into different branches or are they unrelated to each other?
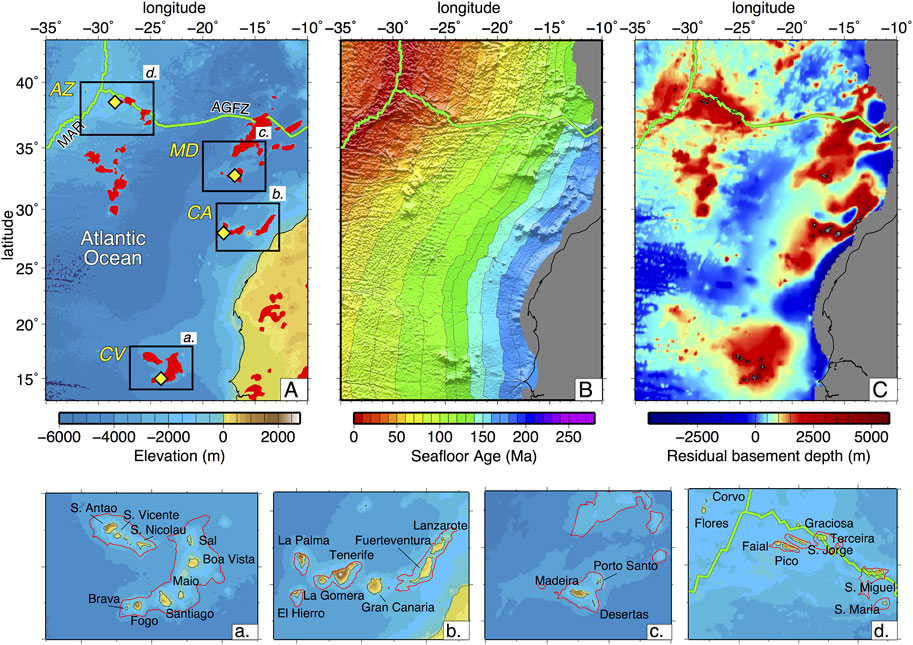
Figure 1. (A) Topographic map with LIP contours (from Johansson et al., 2018) overlaid in red. Macaronesian hotspots are shown as yellow diamonds: CV: Cape Verde; CA: Canaries; MD: Madeira; AZ: Azores. Each LIP province with the names of the islands indicated is shown at the bottom in panels ((A)a–d). AGFZ: Azores-Gibraltar Fracture Zone; MAR: Mid-Atlantic Ridge. Another prominent The New England LIP is located south of the Azores, but it is not discussed in the text. (B) Age of the oceanic lithosphere derived from magnetic and other geophysical data (Müller et al., 2008). (C) Residual ocean basement depth relative to the plate model PS77 (Parsons and Sclater, 1977).
This work describes the state of our knowledge concerning the mantle structure beneath the Macaronesian archipelagos and discusses the challenges and future directions in improving the seismic spatial resolution. Our aim is to summarize for those who do not primarily specialize in seismology the main seismic models of the mantle beneath the Macaronesia region, which provide critical information on the geodynamic mechanisms feeding the ocean island volcanism.
2 Geodynamic framework
The Macaronesian archipelagos are widely considered to represent hotspots and have many features typically associated with the presence of deep-seated mantle plumes. The Azores are characterized by elevated topography (Adam et al., 2013) and pronounced negative Bouguer anomaly (Thibaud et al., 1998; Gente et al., 2003). Similar, but volumetrically less significant anomalies are observed in Madeira, the Canary Islands, and Cape Verde (e.g., Canales and Dañobeitia, 1998; Grevemeyer, 1999; Carracedo et al., 2015). The Macaronesian rocks show also a common ‘Ocean Island Basalt (OIB)’-type composition, characterized by higher alkalinity at similar SiO2 than standard tholeiitic ‘Mid-Ocean Ridge Basalt (MORB)’-type lavas (Graham, 2002).
However, the geodynamic framework of the four archipelagos is different. The Azores are located close to the Mid-Atlantic Ridge (MAR) and do not show age progression (Supplementary Figure S1). Yet, the seismicity is intense with high-magnitude earthquakes associated primarily with active fracture zones (Storch et al., 2020). In contrast, the Madeira, Canary, and Cape Verde archipelagos are in proximity to the African coast, show a general SW-NE progression of increasing volcanism age consistent with the direction of the African plate and are marked by a generally low-magnitude volcano-related seismicity (Carracedo and Troll, 2021). This has sparked the debate about whether such volcanic archipelagos form by melting of buoyantly upwelling mantle plumes, or simply the result of tectonically-driven melt extraction (Courtillot et al., 2003; Anderson et al., 2005).
3 Large-scale mantle structure of the Central Atlantic Ocean
Many seismic imaging studies illuminated the mantle structure beneath the Central Atlantic Ocean mainly through tomography using different seismic wave types as body waves (Montelli et al., 2004; 2006; Zhao, 2007; Simmons et al., 2012), surface waves (e.g., Mocquet and Romanowicz, 1990; Silveira and Stutzmann, 2002; Pilidou et al., 2004; James et al., 2014; Celli et al., 2020a) or a combination of both (e.g, Honda and Tanimoto, 1987; French et al., 2013; Zhu and Tromp, 2013; French and Romanowicz, 2014).
Using surface waves, Celli et al. (2020a) imaged broad but separated low-velocity anomalies in the upper mantle below the Azores, Cape Verde, Canary and Madeira islands. The finite-frequency tomography images of Montelli et al. (2004, 2006) show that these signatures connect at 1,000–1,400 km depth and reach the bottom of the mantle beneath western Africa.
Figure 2 illustrates four whole-mantle P- and S-wave tomography models (Amaru, 2007; Ritsema et al., 2011; Simmons et al., 2012; French and Romanowicz, 2014), which map isolated upper-mantle low-velocity anomalies merging in a single body at ∼1,200 km depth. This broad feature has been interpreted as a dome-like instability–the so-called Central-East Atlantic Anomaly (CEAA)– connected to the African Large Low Shear-Velocity Province (LLSVP, Davaille and Romanowicz, 2020; Civiero et al., 2021).
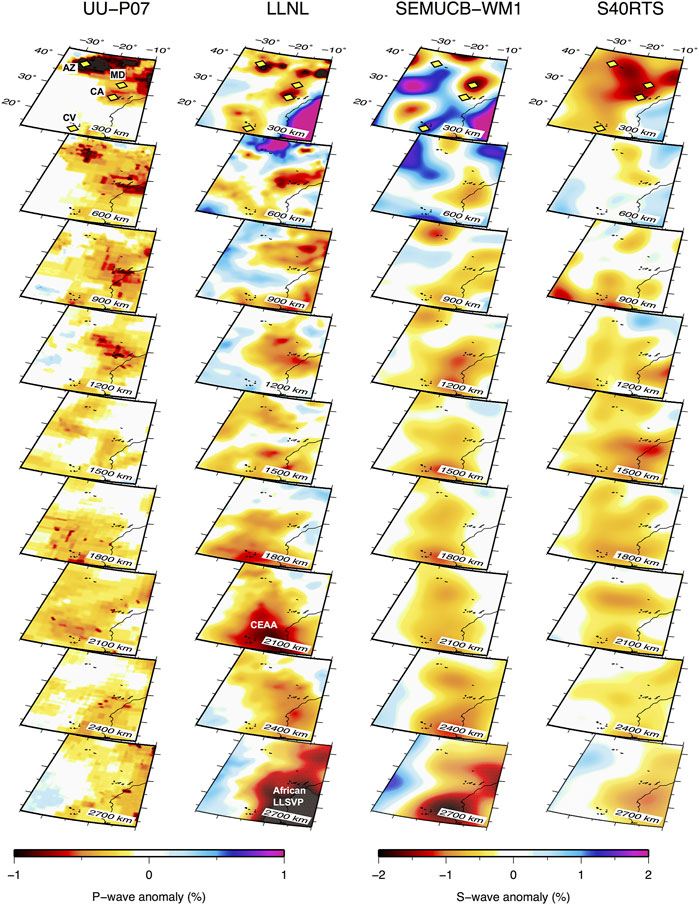
Figure 2. Map views taken from the following whole-mantle tomography models beneath the Central-East Atlantic Ocean: the P-wave model UU-P07 (Amaru, 2007) in the first column; the P-wave model LLNL (Simmons et al., 2012) in the second column; the S-wave model SEMUCB-WM1 (French and Romanowicz, 2014) in the third column; and the S-wave model S40RTS (Ritsema et al., 2011) in the fourth column. CEAA: Central-East Atlantic Anomaly.
PP and SS precursors and receiver functions (RFs) on the mantle transition zone (MTZ) discontinuities at ∼410- and 660-km depth provide independent information on the mantle structure. These discontinuities are caused by pressure-induced mineral phase transformations. The depth at which the phase transformations occur depends mainly on the pressure-temperature conditions at the boundaries. Consequently, the variation of the discontinuities’ topography is commonly seen as a signature of the lateral variation in the thermal structure of the MTZ. Cold downwelling material (e.g., subducting slab) would warp the 410-km discontinuity up and the 660-km discontinuity down, while hot upwelling material (e.g., plume rising from the lower mantle) would result in a depression of the 410-km discontinuity and uplift of the 660-km discontinuity (Bina and Helffrich, 1994). The global SS precursors studies of Flanagan and Shearer (1998) and Houser et al. (2008) find a close-to-normal MTZ thickness in the Central-East Atlantic Ocean. Gu et al. (2009) report a slightly thinned MTZ in the same region, in agreement with other global studies using RFs and sensitivity kernels (Lawrence and Shearer, 2006; 2008). Saki et al. (2015) reveal large depressions of the 410-km discontinuity beneath all the archipelagos and a raised 660-km discontinuity close to the MAR and propose that a large hot plume reaches the base of the MTZ in the central-south Atlantic without penetrating it. This is in line with the tomographic models shown in Figure 2 that image broad low-velocity bodies in the uppermost lower mantle beneath all the archipelagos suggesting plume material accumulation right underneath the MTZ.
Several studies of shear-wave splitting, travel times and phase velocities detecting large-scale anisotropy features in central-north Atlantic have been published (Kuo et al., 1987; Sheehan and Solomon, 1991; Yang and Fischer, 1994; Tanimoto and Anderson, 2000; Silveira and Stutzmann, 2002; Pilidou et al., 2005; Zhu and Tromp, 2013). The anisotropy tomography of Silveira and Stutzmann (2002) find a striking azimuthal anisotropy signature beneath many Atlantic Ocean archipelagos where fast S-wave velocity direction diverges radially from the hotspots. Pilidou et al. (2004, 2005) show that while the plate motion dominates the upper-mantle flow beneath the North Atlantic, this pattern is disrupted around and below Iceland and they speculate that this could occur when an additional flow type due to rising plumes is introduced.
4 Regional mantle imaging studies on the Macaronesian Islands: State of the art
The seismic coverage in the Macaronesia region has been greatly improved, adding broadband recordings from land-based stations accumulated over the last 20 years (e.g., Lodge and Helffrich, 2006; Silveira et al., 2010; Vinnik et al., 2012; Hannemann et al., 2013; Matos et al., 2015). Despite a few past efforts (e.g., Grevemeyer et al., 2010; Crawford et al., 2017), seismic data from Ocean Bottom Seismometers (OBSs) around the archipelagos are still not available. Below we will provide a short review of the regional seismic studies that help image the mantle structure below the islands and distinguish the geodynamic mechanisms driving ocean island volcanism. A summary of the geophysical observations and the mechanisms proposed for each archipelago is shown in the Supplementary Table S1. Most tomography studies illuminate only the upper mantle and at an insufficiently fine resolution to resolve the small-scale (<100 km) features, especially narrow conduits that would indicate mantle plumes.
4.1 Cape Verde
The Cape Verde archipelago is composed of nine islands and overlies a topographic anomaly known as the Cape Verde Rise (up to ∼2000 m high), considered one of the largest oceanic intraplate bathymetric anomalies (Monnereau and Cazenave, 1990) (Figure 1Aa).
Upper-mantle regional tomography models (Liu and Zhao, 2014; 2021; Lodhia et al., 2018) display low asthenospheric velocities beneath the archipelago, suggesting an anomalously hot upper mantle. Carvalho et al. (2019) using dispersion data image a low-velocity zone from ∼60 to ∼210 km depth. The recent VS model of Liu and Zhao (2021) shows two low-velocity anomalies (100–200 km wide), which merge into a single low-Vs column down to the base of the MTZ, interpreted as the seismic signature of a hot, volatile- and melt-rich plume. This agrees with geochemical data that find HIMU-type and lower-mantle materials in the Cape Verde lava, which are considered to have been carried to the upper mantle by a plume rooted at the base of the mantle (e.g., Hoernle et al., 2002; Mourão et al., 2012; Jackson et al., 2018). Numerical modelling results propose edge-driven flow triggered by the African cratonic root as an alternative mechanism to explain this low-velocity anomaly (King and Ritsema, 2000).
Shear-wave splitting analyses suggest that low-density material produced by upwelling melts spreads from separate melting loci below the islands with a non-radial pattern (Lodge and Helffrich, 2006) confirming the plume hypothesis. The existence of hot mantle upwellings is more debated at deeper depths, especially within the MTZ. Indeed, seismic observations on the MTZ discontinuities found surprisingly different results: a normal MTZ thickness was reported by Deuss (2007) and Helffrich et al. (2010) in contrast with Gu et al. (2009), Vinnik et al. (2012) and Saki et al. (2015) who observe a significant MTZ thinning up to ∼30 km less than average. Evidence of temperature and compositional anomalies in the MTZ remains thus inconclusive, as well as its nature.
4.2 Canaries
The Canary archipelago comprises seven volcanic islands and several seamounts located on the oldest lithosphere in the ocean basins (>170 Ma; Figures 1Ab) with its eastern edge only 100 km from the NW African coast (Geldmacher et al., 2005).
To explain the distribution of the volcanism in the islands, Anguita and Hernán (2000) suggest a model where an upper-mantle residue from a fossil plume interacts with a lithospheric fracture propagating from the Canary Islands to the Atlas Mountains in Morocco. Later, similar unified scenarios invoking a mantle plume interacting with a complex lithospheric architecture have been proposed by Geldmacher et al. (2005); Duggen et al. (2009); Manjón-Cabeza Córdoba and Ballmer, (2022) for this area and applied to other regions on Earth (e.g.; Celli et al., 2020b; Civiero et al., 2022). Currently, the debate is focused on whether the volcanism is due to a deep plume or shallower upper-mantle upwellings (e.g., Hoernle et al., 1995; Piromallo et al., 2008; García-Yeguas et al., 2014; Miller et al., 2015; Saki et al., 2015; Long et al., 2020). Recently, an integrated analysis using tomography and paleo-reconstruction models proposed that upper-mantle plumelets (∼150–200 km wide) at various times and locations since at least 90 Ma may exist below the volcanic chain (Civiero et al., 2021).
Previous RFs and precursors studies (e.g., Deuss, 2007; Martinez-Arevalo et al., 2013; Saki et al., 2015) find an overall thinned MTZ (∼15–20 km less than normal) beneath the Canaries indicating that it is likely crossed by hot mantle material. The presence of a plume beneath the archipelago is also supported by new shear-wave splitting measurements (Schlaphorst et al., 2022), which show a small-scale complexity of the mantle flow pattern in the Canary Islands and suggest that it may be perturbed by a rising upwelling.
4.3 Madeira
Madeira archipelago is located 450 km to the north of the Canary Islands and is considered the current location of the >70 Ma old hotspot which formed a line of seamounts and islands extending from NE to SW (Geldmacher and Hoernle, 2000) (Figures 1Ac).
The upper-mantle structure underneath Madeira is still poorly studied tomographically. The most recent travel-time tomography models of Civiero et al. (2018, 2019) image a low-velocity body below Madeira extending only down to ∼300 km depth, which has been interpreted as a blob-like mantle upwelling (∼100-km wide), apparently disconnected from the MTZ. The upper-mantle structure is partially identified in the previous body-wave tomography of Bonnin et al. (2014) and shows much more complexity than the large-scale low-velocity anomaly detected by global models (e.g.; Hoernle et al., 1995; Montelli et al., 2006; Simmons et al., 2012).
Although Madeira and Canary hotspots are only separated by ∼400–500 km, the geochemistry of their respective magmatic provinces is clearly distinct (Geldmacher et al., 2005); however, their arrays converge to a composition close to the C component (Geldmacher et al., 2011). This common C component has been suggested to reside on a lower-mantle thermochemical source, which may feed all the hotspots in the Central Atlantic Ocean (Hoernle et al., 1995; Mata et al., 1998; Widom et al., 1999).
Shear-wave splitting results (Schlaphorst et al., 2022) find perturbed, small-scale heterogeneous orientation patterns below Madeira ad global RFs whereas precursors studies (e.g., Houser et al., 2008; Lawrence and Shearer, 2008; Deuss, 2009) detect a slightly thinned or close-to-average MTZ thickness, supporting the plume theory. However, more detailed information about the MTZ topography is missing in the literature.
4.4 Azores
The Azores mark the triple junction between the North American, Eurasian, and African plates and consist of nine islands volcanically active from 7 Ma to the present (Gente et al., 2003) (Figures 1Ad). Due to the lack of a consistent age progression of the volcanism, the presence of a mantle plume is more debated here compared to the other archipelagos (see Kueppers and Beier, 2018). Besides, many regional fracture systems caused by its vicinity to the MAR lead many geoscientists to prefer tectonic-type or other passive, plate-driven upwellings to explain the nature of Azores volcanism (e.g., Bourdon et al., 1996; King, 2005; Luis and Neves, 2006).
Regional tomography studies in support of the plume theory image a round low-velocity anomaly (200–500 km in diameter) confined in the asthenosphere, which would have formed the Azores Plateau (Montagner and Ritsema, 2001; Silveira and Stutzmann, 2002; Pilidou et al., 2004; Silveira et al., 2006; Yang et al., 2006). To explain the extension of the slow seismic anomalies further to the south they also propose a hotspot-ridge interaction in which the plume conduit is deflected to the southwest in the shallow mantle by asthenospheric flow and plate motion. Rock geochemistry finds incompatible trace element enrichment coupled with high radiogenic isotope ratios and suggests the existence of a common and compositionally heterogeneous mantle source beneath the Azores (França et al., 2006; Beier et al., 2012; Genske et al., 2016).
The RFs study of Silveira et al. (2010) found a normal MTZ thickness in a large region of the Atlantic around the Azores plateau in agreement with the observations of Li et al. (2003), which used one station deployed in São Miguel. These observations are in line with recent active-source seismic results of Beier et al. (2022) and suggest that the low-velocity anomaly is the seismic expression of a mantle plume with no tail, which may be in its dying phase. However, the only two seismic anisotropy studies published did not find any anisotropy signal in the upper mantle beneath the Azores (Kuo and Forsyth, 1992; Silveira et al., 2010).
5 Recent advances and future directions
Recently, Civiero et al. (2021) proposed a unified scenario where several upper-mantle upwellings are connected to the CEAA, which is ponded below the 660-km discontinuity. This new geodynamic framework offers a challenge for the geophysical community to improve the resolution of the mantle in the Central-East Atlantic, namely by collecting new land- and offshore-based data in and around the archipelagos.
To date, the instrumentation gaps put critical limits on the resolving power of seismological imaging but the growing number and quality of seismic data in the target areas will allow for more high-resolution studies revealing the smallest details of the mantle structure. Special focus has been currently placed in the less explored archipelago of Madeira (Bonatto et al., 2022; Schlaphorst et al., 2022), where the topography of the MTZ discontinuities and the anisotropy signature are still poorly known. Significant advancements in the knowledge of mantle structure in other Earth’s hotspots using recently collected high-resolution data have been successfully carried out, for example, in Hawaii (Agius et al., 2017), Réunion (Scholz et al., 2018; Tsekhmistrenko et al., 2021) and Iceland (Celli et al., 2021).
Until now, most of the methods described above have been used independently of one another. Combining the different analysis methods and using them in conjunction with other results will be fundamental to compensate for simplifying assumptions inherent to every single technique and region of study.
To undertake this challenge, the ongoing project UPFLOW (https://upflow-eu.github.io) has recently deployed 50 OBSs on the seafloor of the Central-East Atlantic Ocean. Applying cutting-edge imaging techniques to these data and integrating them with other Earth Science information will open the possibility to image the fine-scale mantle structure beneath the Macaronesia region and thereby address the open questions about the origin of ocean island volcanism.
Author contributions
CC led the conception and design of the paper. CC and JC reviewed the literature and wrote the document with the input of GS. All authors contributed to the development of ideas and the classification of the papers by theme.
Funding
This work acknowledges the grant CEX2019-000928-S funded by AEI 10.13039/501100011033. This study was funded by the Portuguese Fundação para a Ciência e Tecnologia (FCT), I.P./MCTES through national funds PIDDAC—UIDB/50019/2020 (https://doi.org/10.54499/UIDB/50019/2020), UIDP/50019/2020 (https://doi.org/10.54499/UIDP/50019/2020) and LA/P/0068/2020 (https://doi.org/10.54499/LA/P/0068/2020). JC acknowledges the financial support of FCT I.P./MCTES project through national funds project RESTLESS PTDC/CTAGEF/6674/2020 (https://doi.org/10.54499/PTDC/CTA-GEF/6674/2020). This work is a contribution to three projects FCT I.P./MCTES through national funds SIGHT PTDC/CTAGEF/30264/2017, RESTLESS PTDC/CTA-GEF/6674/2020 (https://doi.org/10.54499/PTDC/CTA-GEF/6674/2020) and GEMMA PTDC/CTA-GEO/2083/2021 (https://doi.org/10.54499/PTDC/CTA-GEO/2083/2021). GS is grateful for support from European Research Council (ERC) UPFLOW consolidator grant under the European Union’s Horizon 2020 research and innovation programme (grant agreement No. 10100160).
Conflict of interest
The authors declare that the research was conducted in the absence of any commercial or financial relationships that could be construed as a potential conflict of interest.
Publisher’s note
All claims expressed in this article are solely those of the authors and do not necessarily represent those of their affiliated organizations, or those of the publisher, the editors and the reviewers. Any product that may be evaluated in this article, or claim that may be made by its manufacturer, is not guaranteed or endorsed by the publisher.
Supplementary material
The Supplementary Material for this article can be found online at: https://www.frontiersin.org/articles/10.3389/feart.2023.1126274/full#supplementary-material
References
Adam, C., Madureira, P., Miranda, J. M., Lourenço, N., Yoshida, M., and Fitzenz, D. (2013). Mantle dynamics and characteristics of the Azores plateau. Earth Planet Sci. Lett. 362, 258–271. doi:10.1016/j.epsl.2012.11.014
Agius, M. R., Rychert, C. A., Harmon, N., and Laske, G. (2017). Mapping the mantle transition zone beneath Hawaii from Ps receiver functions: Evidence for a hot plume and cold mantle downwellings. Earth Planet Sci. Lett. 474, 226–236. doi:10.1016/j.epsl.2017.06.033
Amaru, M. L. (2007). Global travel time tomography with 3-D reference models. Geol. Ultraiectina 274, 174.
Anderson, D. L. (2000). The thermal state of the upper mantle: No rule for mantle plumes. Geophys Res. Lett. 27, 3623–3626. doi:10.1029/2000GL011533
Anderson, D. L. (2005). Scoring hotspots: The plume and plate paradigms. in Plates, plumes and paradigms Editors G. R. Foulger and D. M. Jurdy, D. C. Presnall, D. L. Anderson (Geological Society of America). doi:10.1130/0-8137-2388-4.31
Anguita, F., and Hernán, F. (2000). The Canary Islands origin: a unifying model. J. Volcanol. Geotherm. Res. 103 (1–4), 1–26.
Ballmer, M. D., Conrad, C. P., Smith, E. I., and Harmon, N. (2013). Non-hotspot volcano chains produced by migration of shear-driven upwelling toward the East Pacific Rise. Geology 41 (4), 479–482. doi:10.1130/G33804.1
Ballmer, M. D., van Hunen, J., Ito, G., Tackley, P. J., and Bianco, T. A. (2007). Non-hotspot volcano chains originating from small-scale sublithospheric convection. Geophys Res. Lett. 34, 1–5. doi:10.1029/2007GL031636
Batiza, R. (1982). Abundances, distribution and sizes of volcanoes in the Pacific Ocean and implications for the origin of non-hotspot volcanoes. Earth Planet Sci. Lett. 60, 195–206. doi:10.1016/0012-821X(82)90003-6
Beier, C., Genske, F., Hübscher, C., Haase, K. M., Bach, W., Nomikou, P., et al. (2022). The submarine Azores Plateau: Evidence for a waning mantle plume. Mar. Geol. 451, 106858. doi:10.1016/j.margeo.2022.106858
Beier, C., Haase, K. M., and Turner, S. P. (2012). Conditions of melting beneath the Azores. Lithos 144 (145), 1–11. doi:10.1016/j.lithos.2012.02.019
Bianco, T. A., Ito, G., Becker, J. M., and Garcia, M. O. (2005). Secondary Hawaiian volcanism formed by flexural arch decompression. Geochem. Geophys. Geosystems 6, 1–24. doi:10.1029/2005GC000945
Bonatto, L., Schlaphorst, D., Silveira, G., Mata, J., Civiero, C., Schimmel, M., et al. (2022). “Unveiling the heterogeneous structure of the upper-mantle beneath the Canary and Madeira volcanic provinces,” in EGU general assembly (Vienna, Austria: EGU). doi:10.5194/egusphere-egu22-10184
Bonnin, M., Nolet, G., Villaseñor, A., Gallart, J., and Thomas, C. (2014). Multiple-frequency tomography of the upper mantle beneath the African/Iberian collision zone. Geophys J. Int. 198, 1458–1473. doi:10.1093/gji/ggu214
Bourdon, B., Langmuir, C. H., and Zindler, A. (1996). Ridge-hotspot interaction along the Mid-Atlantic Ridge between 37°30′ and 40°30′N: The U-Th disequilibrium evidence. Earth Planet Sci. Lett. 142, 175–189. doi:10.1016/0012-821x(96)00092-1
Canales, J. P., and Dañobeitia, J. J. (1998). The canary islands swell: A coherence analysis of bathymetry and gravity. Geophys J. Int. 132, 479–488. doi:10.1046/j.1365-246x.1998.00448.x
Carracedo, J. C., Perez-Torrado, F. J., Rodriguez-Gonzalez, A., Paris, R., Troll, V. R., and Barker, A. K. (2015). Volcanic and structural evolution of Pico do Fogo, Cape Verde. Available at: http://www.
Carracedo, J. C., and Troll, V. R. (2021). North-East atlantic islands: The macaronesian archipelagos. Encycl. Geol. 2021, 674–699. doi:10.1016/b978-0-08-102908-4.00027-8
Carvalho, J., Bonadio, R., Silveira, G., Lebedev, S., Mata, J., Arroucau, P., et al. (2019). Evidence for high temperature in the upper mantle beneath Cape Verde archipelago from Rayleigh-wave phase-velocity measurements. Tectonophysics 770, 228225. doi:10.1016/j.tecto.2019.228225
Carvalho, J., Silveira, G., Kiselev, S., Custódio, S., Ramalho, R. S., Stutzmann, E., et al. (2022). Crustal and uppermost mantle structure of Cape Verde from ambient noise tomography. Geophys J. Int. 231, 1421–1433. doi:10.1093/gji/ggac254
Celli, N. L., Lebedev, S., Schaeffer, A., and Gaina, C. (2020b). African cratonic lithosphere carved by mantle plumes. Nat. Commun. 11, 92. doi:10.1038/s41467-019-13871-2
Celli, N. L., Lebedev, S., Schaeffer, A. J., and Gaina, C. (2021). The tilted Iceland Plume and its effect on the North Atlantic evolution and magmatism. Earth Planet Sci. Lett. 569, 117048. doi:10.1016/j.epsl.2021.117048
Celli, N. L., Lebedev, S., Schaeffer, A. J., Ravenna, M., and Gaina, C. (2020a). The upper mantle beneath the South Atlantic Ocean, South America and Africa from waveform tomography with massive datasets. Geophys J. Int. 221, 178–204. doi:10.1093/gji/ggz574
Civiero, C., Custódio, S., Neres, M., Schlaphorst, D., Mata, J., and Silveira, G. (2021). The role of the seismically slow central-East Atlantic anomaly in the Genesis of the canary and Madeira volcanic provinces. Geophys Res. Lett. 1, 15. doi:10.1029/2021gl092874
Civiero, C., Custódio, S., Rawlinson, N., Strak, V., Silveira, G., Arroucau, P., et al. (2019). Thermal nature of mantle upwellings below the ibero-western maghreb region inferred from teleseismic tomography. J. Geophys Res. Solid Earth 124, 1781–1801. doi:10.1029/2018JB016531
Civiero, C., Lebedev, S., and Celli, N. L. (2022). A complex mantle plume head below east africa-arabia shaped by the lithosphere-asthenosphere boundary topography. Geochem. Geophys. Geosystems 23, e2022GC010610. doi:10.1029/2022GC010610
Civiero, C., Strak, V., Custódio, S., Silveira, G., Rawlinson, N., Arroucau, P., et al. (2018). A common deep source for upper-mantle upwellings below the Ibero-Western Maghreb region from teleseismic P -wave travel-time tomography. Earth Planet Sci. Lett. 499, 157–172. doi:10.1016/j.epsl.2018.07.024
Conrad, C. P., Wu, B., Smith, E. I., Bianco, T. A., and Tibbetts, A. (2010). Shear-driven upwelling induced by lateral viscosity variations and asthenospheric shear: A mechanism for intraplate volcanism. Phys. Earth Planet. Interiors 178, 162–175. doi:10.1016/j.pepi.2009.10.001
Courtillot, V., Davaille, A., Besse, J., and Stock, J. (2003). Three distinct types of hotspots in the Earth’s mantle. Earth Planet Sci. Lett. 205 (3–4), 295–308.
Crawford, W., Cannat, M., Sarradin, P. -M., and Romuald, D. (2017). Array of autonomous short period Ocean Bottom Seismometers from the EMSO-Azores observatory, 2012-2013. SEANOE. doi:10.17882/48330
Davaille, A., and Romanowicz, B. (2020). Deflating the LLSVPs: Bundles of mantle thermochemical plumes rather than thick stagnant “piles. Tectonics 39, 1–21. doi:10.1029/2020tc006265
Deuss, A. (2009). Global observations of mantle discontinuities using SS and PP precursors. Surv. Geophys 30, 301–326. doi:10.1007/s10712-009-9078-y
Deuss, A. (2007). “Seismic observations of transition-zone discontinuities beneath hotspot locations,” in Special paper 430: Plates, plumes and planetary processes. Editors G. R. Foulger, and D. M. Jurdy (Geological Society of America), 121–136. doi:10.1130/2007.2430(07
Duggen, S., Hoernle, K. A., Hauff, F., Klügel, A., Bouabdellah, M., and Thirlwall, M. F. (2009). Flow of Canary mantle plume material through a subcontinental lithospheric corridor beneath Africa to the Mediterranean. Geology 37, 283–286. doi:10.1130/G25426A.1
Flanagan, M. P., and Shearer, P. M. (1998). Global mapping of topography on transition zone velocity discontinuities by stacking SS precursors. J. Geophys Res. Solid Earth 103, 2673–2692. doi:10.1029/97jb03212
França, Z., Lago, M., Nunes, J. C., Galé, C., Forjaz, V. H., Pueyo, O., et al. (2006). Geochemistry of alkaline basalts of corvo island (Azores, Portugal): Preliminary data geoquímica de los basaltos alcalinos de la Isla de Corvo (Azores, Portugal): Datos preliminares. Geogaceta 40, 87–90.
French, S. W., Lekić, V., and Romanowicz, B. (2013). Large-scale mantle convection and the history of subduction. Science 355, 437–440. doi:10.1038/355437a0
French, S. W., and Romanowicz, B. A. (2014). Whole-mantle radially anisotropic shear velocity structure from spectral-element waveform tomography. Geophys J. Int. 199, 1303–1327. doi:10.1093/gji/ggu334
García-Yeguas, A., Ibáñez, J. M., Koulakov, I., Jakovlev, A., Romero-Ruiz, M. C., and Prudencio, J. (2014). Seismic tomography model reveals mantle magma sources of recent volcanic activity at El Hierro Island (Canary Islands, Spain). Geophys J. Int. 199, 1739–1750. doi:10.1093/gji/ggu339
Geldmacher, J., Hoernle, K., Bogaard, P. v. d., Duggen, S., and Werner, R. (2005). New40Ar39Ar age and geochemical data from seamounts in the Canary and Madeira volcanic provinces: Support for the mantle plume hypothesis. Earth Planet Sci. Lett. 237, 85–101. doi:10.1016/j.epsl.2005.04.037
Geldmacher, J., Hoernle, K., Hanan, B. B., Blichert-Toft, J., Hauff, F., Gill, J. B., et al. (2011). Hafnium isotopic variations in East Atlantic intraplate volcanism. Contributions Mineralogy Petrology 162, 21–36. doi:10.1007/s00410-010-0580-5
Geldmacher, J., and Hoernle, K. (2000). The 72 Ma geochemical evolution of the Madeira hotspot (eastern North Atlantic): Recycling of paleozoic (≤500 Ma) oceanic lithosphere. Earth Planet Sci. Lett. 183, 73–92. doi:10.1016/S0012-821X(00)00266-1
Genske, F. S., Beier, C., Stracke, A., Turner, S. P., Pearson, N. J., Hauff, F., et al. (2016). Comparing the nature of the Western and eastern Azores mantle. Geochim. Cosmochim. Acta 172, 76–92. doi:10.1016/j.gca.2015.08.019
Gente, P., Dyment, J., Maia, M., and Goslin, J. (2003). Interaction between the Mid-Atlantic Ridge and the Azores hot spot during the last 85 Myr: Emplacement and rifting of the hot spot-derived plateaus. Geochem. Geophys. Geosystems 4, 527. doi:10.1029/2003GC000527
Graham, D. W. (2002). Noble gas isotope geochemistry of mid-ocean ridge and ocean island basalts: Characterization of mantle source reservoirs. Rev. Mineral. Geochem 47, 247–317. doi:10.2138/rmg.2002.47.8
Grevemeyer, I., Helffrich, G., Faria, B., Booth-Rea, G., Schnabel, M., and Weinrebe, W. (2010). Seismic activity at Cadamosto seamount near Fogo Island, Cape Verdes - formation of a new ocean island? Geophys J. Int. 180, 552–558. doi:10.1111/j.1365-246X.2009.04440.x
Grevemeyer, I. (1999). Isostatic geoid anomalies over mid-plate swells in the Central North Atlantic. J. Geodyn. 28, 41–50. doi:10.1016/S0264-3707(98)00031-3
Gu, Y. J., An, Y., Sacchi, M., Schultz, R., and Ritsema, J. (2009). Mantle reflectivity structure beneath oceanic hotspots. Geophys J. Int. 178, 1456–1472. doi:10.1111/j.1365-246X.2009.04242.x
Hannemann, K., Krüger, F., and Dahm, T. (2013). “Performance comparison of mid-aperture broadband arrays at an ocean island and in the deep ocean,” in EGU general assembly conference abstracts (Germany: Helmholtz Center for Geosciences, Potsdam). Available at: https://ui.adsabs.harvard.edu/abs/2013EGUGA.1510313H.
Helffrich, G., Faria, B., Fonseca, J. F. B. D., Lodge, A., and Kaneshima, S. (2010). Transition zone structure under a stationary hot spot: Cape Verde. Earth Planet Sci. Lett. 289, 156–161. doi:10.1016/j.epsl.2009.11.001
Hoernle, K., Tilton, G., le Bas, M. J., Duggen, S., and Garbe-Schönberg, D. (2002). Geochemistry of oceanic carbonatites compared with continental carbonatites: Mantle recycling of oceanic crustal carbonate. Contributions Mineralogy Petrology 142, 520–542. doi:10.1007/s004100100308
Hoernle, K., Zhang, Y. S., and Graham, D. (1995). Seismic and geochemical evidence for large-scale mantle upwelling beneath the eastern Atlantic and Western and central Europe. Nature 374, 34–39. doi:10.1038/374034a0
Honda, S., and Tanimoto, T. (1987). Regional 3-D heterogeneities by waveform inversion – application to the Atlantic area. Geophys. J. R. Astronomical Soc. 91, 737–753. doi:10.1111/j.1365-246X.1987.tb01667.x
Houser, C., Masters, G., Flanagan, M., and Shearer, P. (2008). Determination and analysis of long-wavelength transition zone structure using SS precursors. Geophys J. Int. 174, 178–194. doi:10.1111/j.1365-246X.2008.03719.x
Jackson, M. G., Becker, T. W., and Konter, J. G. (2018). Evidence for a deep mantle source for EM and HIMU domains from integrated geochemical and geophysical constraints. Earth Planet Sci. Lett. 484, 154–167. doi:10.1016/j.epsl.2017.11.052
James, E. K., Dalton, C. A., and Gaherty, J. B. (2014). R ayleigh wave phase velocities in the A tlantic upper mantle. Geochem. Geophys. Geosystems 15 (11), 4305–4324.
Johansson, L., Zahirovic, S., and Müller, R. D. (2018). The interplay between the eruption and weathering of large igneous provinces and the deep-time carbon cycle. Geophys Res. Lett. 45, 5380–5389. doi:10.1029/2017GL076691
King, S. D. (2005). North Atlantic topographic and geoid anomalies: The result of a narrow ocean basin and cratonic roots? Special Pap. Geol. Soc. Am. 388, 653–664. doi:10.1130/0-8137-2388-4.653
King, S. D., and Ritsema, J. (2000). African hot spot volcanism: Small-scale convection in the upper mantle beneath cratons. Science 290, 1137–1140. doi:10.1126/science.290.5494.1137
Kueppers. U, and Beier. C (Editors) (2018). in Volcanoes of the Azores revealing the geological secrets of the central northern atlantic islands (Berlin: Springer). doi:10.1007/978-3-642-32226-6
Kuo, B. Y., Forsyth, D., and Wysession, M. (1987). Lateral heterogeneity and azimuthal anisotropy in the North Atlantic determined from SS-S differential travel times. J. Geophys Res. Solid Earth 92, 6421–6436. doi:10.1029/jb092ib07p06421
Kuo, B. -Y., and Forsyth, D. W. (1992). A search for split SKS waveforms in the North Atlantic. Geophys J. Int. 108, 557–574. doi:10.1111/j.1365-246X.1992.tb04636.x
Lawrence, J. F., and Shearer, P. M. (2006). A global study of transition zone thickness using receiver functions. J. Geophys Res. Solid Earth 111, 1–10. doi:10.1029/2005JB003973
Lawrence, J. F., and Shearer, P. M. (2008). Imaging mantle transition zone thickness with SdS - SS finite-frequency sensitivity kernels. Geophys J. Int. 174, 143–158. doi:10.1111/j.1365-246X.2007.03673.x
Lees, J. M. (2007). Seismic tomography of magmatic systems. J. Volcanol. Geotherm. Res. 167, 37–56. doi:10.1016/j.jvolgeores.2007.06.008
Li, X., Kind, R., and Yuan, X. (2003). Seismic study of upper mantle and transition zone beneath hotspots. Phys. Earth Planet. Interiors 136, 79–92. doi:10.1016/S0031-9201(03)00021-9
Liu, X., and Zhao, D. (2014). Seismic evidence for a mantle plume beneath the Cape Verde hotspot. Int. Geol. Rev. 56, 1213–1225. doi:10.1080/00206814.2014.930720
Liu, X., and Zhao, D. (2021). Seismic evidence for a plume-modified oceanic lithosphere-asthenosphere system beneath Cape Verde. Geophys J. Int. 225, 872–886. doi:10.1093/gji/ggab012
Lodge, A., and Helffrich, G. (2006). Depleted swell root beneath the Cape Verde Islands. Geology 34, 449–452. doi:10.1130/G22030.1
Lodhia, B. H., Roberts, G. G., Fraser, A. J., Fishwick, S., Goes, S., and Jarvis, J. (2018). Continental margin subsidence from shallow mantle convection: Example from West Africa. Earth Planet Sci. Lett. 481, 350–361. doi:10.1016/j.epsl.2017.10.024
Long, X., Geldmacher, J., Hoernle, K., Hauff, F., Wartho, J. A., and Garbe-Schönberg, C. D. (2020). Origin of isolated seamounts in the Canary Basin (East Atlantic): The role of plume material in the origin of seamounts not associated with hotspot tracks. Terra nova. 32, 390–398. doi:10.1111/ter.12468
Luis, J. F., and Neves, M. C. (2006). The isostatic compensation of the Azores Plateau: A 3D admittance and coherence analysis. J. Volcanol. Geotherm. Res. 156, 10–22. doi:10.1016/j.jvolgeores.2006.03.010
Manjón-Cabeza Córdoba, A., and Ballmer, M. D. (2022). The role of edge-driven convection in the generation of volcanism – Part 2: Interaction with mantle plumes, applied to the Canary Islands. Solid Earth Discuss. 13, 1585–1605. doi:10.5194/se-13-1585-2022
Manjón-Cabeza Córdoba, A., and Ballmer, M. (2020). The role of edge-driven convection in the generation of volcanism I: A 2D systematic study. Solid Earth Discuss. 2020, 1–25. doi:10.5194/se-2020-120
Martinez-Arevalo, C., Mancilla, F. de L., Helffrich, G., and Garcia, A. (2013). Seismic evidence of a regional sublithospheric low velocity layer beneath the Canary Islands. Tectonophysics 608, 586–599. doi:10.1016/j.tecto.2013.08.021
Mata, J., Kerrich, R., MacRae, N. D., and Wu, T. W. (1998). Elemental and isotopic (Sr, Nd, and Pb) characteristics of Madeira Island basalts: Evidence for a composite HIMU - EM I plume fertilizing lithosphere. Can. J. Earth Sci. 35, 980–997. doi:10.1139/e98-046
Matos, C., Silveira, G., Matias, L., Caldeira, R., Ribeiro, M. L., Dias, N. A., et al. (2015). Upper crustal structure of Madeira Island revealed from ambient noise tomography. J. Volcanol. Geotherm. Res. 298, 136–145. doi:10.1016/j.jvolgeores.2015.03.017
Mocquet, A., and Romanowicz, B. (1990). Three-dimensional structure of the upper mantle beneath the Atlantic Ocean inferred from long-period Rayleigh waves: 2. Inversion. J. Geophys. Res. Solid Earth 95 (B5), 6787–6798.
Miller, M. S., O'Driscoll, L. J., Butcher, A. J., and Thomas, C. (2015). Imaging Canary Island hotspot material beneath the lithosphere of Morocco and southern Spain. Earth Planet Sci. Lett. 431, 186–194.
Monnereau, M., and Cazenave, A. (1990). Depth and geoid anomalies over oceanic hotspot swells: A global survey. J. Geophys Res. 95, 15429. doi:10.1029/jb095ib10p15429
Montagner, J. P., and Ritsema, J. (2001). Interactions BetweenRidges and plumes. Science 294, 1472–1473. doi:10.1126/science.1067486
Montelli, R., Nolet, G., Dahlen, F. A., and Masters, G. (2006). A catalogue of deep mantle plumes: New results from finite frequency tomography. Geochem. Geophys. Geosystems 7, Q11007. doi:10.1029/2006gc001248
Montelli, R., Nolet, G., Dahlen, F. A., Masters, G., Engdahl, E. R., and Hung, S. H. (2004). Finite-frequency tomography reveals a variety of plumes in the mantle. Science 303, 338–343. doi:10.1126/science.1092485
Morgan, W. J. (1972). Plate motions and deep mantle convection. Mem. Geol. Soc. Am. 132, 7–22. doi:10.1130/MEM132-p7
Mourão, C., Mata, J., Doucelance, R., Madeira, J., Millet, M. A., and Moreira, M. (2012). Geochemical temporal evolution of Brava Island magmatism: Constraints on the variability of Cape Verde mantle sources and on carbonatite-silicate magma link. Chem. Geol. 334, 44–61. doi:10.1016/j.chemgeo.2012.09.031
Müller, R. D., Sdrolias, M., Gaina, C., and Roest, W. R. (2008). Age, spreading rates, and spreading asymmetry of the world’s ocean crust. Geochem. Geophys. Geosystems 9, 1–19. doi:10.1029/2007GC001743
Parsons, B., and Sclater, J. G. (1977). An analysis of the variation of ocean floor bathymetry and heat flow with age. J. Geophys Res. 82, 803–827. doi:10.1029/jb082i005p00803
Pilidou, S., Priestley, K., Debayle, E., and Gudmundsson, Ó. (2005). Rayleigh wave tomography in the North Atlantic: High resolution images of the Iceland, Azores and Eifel mantle plumes. Lithos 79, 453–474. doi:10.1016/j.lithos.2004.09.012
Pilidou, S., Priestley, K., Gudmundsson, Ó., and Debayle, E. (2004). Upper mantle S-wave speed heterogeneity and anisotropy beneath the North Atlantic from regional surface wave tomography: The Iceland and Azores plumes. Geophys J. Int. 159, 1057–1076. doi:10.1111/j.1365-246X.2004.02462.x
Piromallo, C., Gasperini, D., Macera, P., and Faccenna, C. (2008). A late Cretaceous contamination episode of the European – mediterranean mantle. Earth Planet. Sci. Lett. 268, 15–27. doi:10.1016/j.epsl.2007.12.019
Raddick, M. J., Parmentier, E. M., and Scheirer, D. S. (2002). Buoyant decompression melting: A possible mechanism for intraplate volcanism. J. Geophys Res. Solid Earth 107, ECV 7-1–ECV 7-14. doi:10.1029/2001jb000617
Ritsema, J., Deuss, A., van Heijst, H. J., and Woodhouse, J. H. (2011). S40RTS: A degree-40 shear-velocity model for the mantle from new Rayleigh wave dispersion, teleseismic traveltime and normal-mode splitting function measurements. Geophys J. Int. 184, 1223–1236. doi:10.1111/j.1365-246X.2010.04884.x
Saki, M., Thomas, C., Nippress, S. E. J., and Lessing, S. (2015). Topography of upper mantle seismic discontinuities beneath the North Atlantic: The Azores, Canary and Cape Verde plumes. Earth Planet Sci. Lett. 409, 193–202. doi:10.1016/j.epsl.2014.10.052
Schlaphorst, D., Silveira, G., Mata, J., Krüger, F., Dahm, T., and Ferreira, A. M. G. (2022). Heterogeneous seismic anisotropy beneath Madeira and Canary archipelagos revealed by local and teleseismic shear wave splitting. Geophys J. Int. Accept 233 (1), 510–528.
Scholz, J. R., Barruol, G., Fontaine, F. R., Mazzullo, A., Montagner, J. P., Stutzmann, E., et al. (2018). SKS splitting in the Western Indian Ocean from land and seafloor seismometers: Plume, plate and ridge signatures. Earth Planet Sci. Lett. 498, 169–184. doi:10.1016/j.epsl.2018.06.033
Sheehan, A. F., and Solomon, S. C. (1991). Joint inversion of shear wave travel time residuals and geoid and depth anomalies for long-wavelength variations in upper mantle temperature and composition along the Mid-Atlantic Ridge. J. Geophys Res. 96, 19981–20009. doi:10.1029/91jb01988
Silveira, G., and Stutzmann, E. (2002). Anisotropic tomography of the Atlantic Ocean. Phys. Earth Planet. Interiors 132, 237–248. doi:10.1016/S0031-9201(02)00076-6
Silveira, G., Stutzmann, E., Davaille, A., Montagner, J., Mendes-Victor, L., and Sebai, A. (2006). Azores hotspot signature in the upper mantle. J. Volcanol. Geotherm. Res. 156, 23–34. doi:10.1016/j.jvolgeores.2006.03.022
Silveira, G., Vinnik, L., Stutzmann, E., Farra, V., Kiselev, S., and Morais, I. (2010). Stratification of the Earth beneath the Azores from P and S receiver functions. Earth Planet Sci. Lett. 299, 91–103. doi:10.1016/j.epsl.2010.08.021
Simmons, N. A., Myers, S. C., Johannesson, G., and Matzel, E. (2012). LLNL-G3Dv3: Global P wave tomography model for improved regional and teleseismic travel time prediction. J. Geophys Res. Solid Earth 117, B10302. doi:10.1029/2012jb009525
Slater, L., Jull, M., McKenzie, D., and Gronvöld, K. (1998). Deglaciation effects on mantle melting under Iceland: Results from the northern volcanic zone. Earth Planet Sci. Lett. 164, 151–164. doi:10.1016/S0012-821X(98)00200-3
Spieker, K., Rondenay, S., Ramalho, R., Thomas, C., and Helffrich, G. (2018). Constraints on the structure of the crust and lithosphere beneath the Azores Islands from teleseismic receiver functions. Geophys J. Int. 213, 824–835. doi:10.1093/gji/ggy022
Storch, B., Haase, K. M., Romer, R. H. W., Beier, C., and Koppers, A. A. P. (2020). Rifting of the oceanic Azores Plateau with episodic volcanic activity. Sci. Rep. 10, 19718–19812. doi:10.1038/s41598-020-76691-1
Stuart, W. D., Foulger, G. R., and Barall, M. (2007). Propagation of the Hawaiian-Emperor volcano chain by Pacific plate cooling stress.
Tanimoto, T., and Anderson, D. L. (2000). Lateral heterogeneity and azimuthal anisotropy of the upper mantle’ love and Rayleigh waves. J. Geophys. Res. Atmos. 90, 1842–1858. doi:10.1029/JB090iB02p01842
Thibaud, R., Gente, P., and Maia, M. (1998). A systematic analysis of the Mid-Atlantic Ridge morphology and gravity between 15°N and 40°N: Constraints of the thermal structure. J. Geophys Res. Solid Earth 103, 24223–24243. doi:10.1029/97jb02934
Tsekhmistrenko, M., Sigloch, K., Hosseini, K., and Barruol, G. (2021). A tree of Indo-African mantle plumes imaged by seismic tomography. Nat. Geosci. 14, 612–619. doi:10.1038/s41561-021-00762-9
Vinnik, L., Silveira, G., Kiselev, S., Farra, V., Weber, M., and Stutzmann, E. (2012). Cape Verde hotspot from the upper crust to the top of the lower mantle. Earth Planet Sci. Lett. 319–320, 259–268. doi:10.1016/j.epsl.2011.12.017
Widom, E., Hoernle, K. A., Shirey, S. B., and Schmincke, H. U. (1999). Os isotope systematics in the Canary Islands and Madeira: Lithospheric contamination and mantle plume signatures. J. Petrology 40, 279–296. doi:10.1093/petroj/40.2.279
Yang, T., Shen, Y., van der Lee, S., Solomon, S. C., and Hung, S. H. (2006). Upper mantle structure beneath the Azores hotspot from finite-frequency seismic tomography. Earth Planet Sci. Lett. 250, 11–26. doi:10.1016/j.epsl.2006.07.031
Yang, X., and Fischer, K. M. (1994). Constraints on North Atlantic upper mantle anisotropy from S and SS phases. Geophys Res. Lett. 21, 309–312. doi:10.1029/93gl03261
Zhao, D. (2007). Seismic images under 60 hotspots: Search for mantle plumes. Gondwana Res. 12, 335–355. doi:10.1016/j.gr.2007.03.001
Keywords: Macaronesia region, mantle structure and dynamics, seismic imaging, ocean island volcanism, Cape Verde islands, Canary and Madeira islands, Azores islands
Citation: Civiero C, Carvalho J and Silveira G (2023) Mantle structure beneath the Macaronesian volcanic islands (Cape Verde, Canaries, Madeira and Azores): A review and future directions. Front. Earth Sci. 11:1126274. doi: 10.3389/feart.2023.1126274
Received: 17 December 2022; Accepted: 27 March 2023;
Published: 06 April 2023.
Edited by:
Dhananjay Ravat, University of Kentucky, United StatesReviewed by:
Isabel Blanco-Montenegro, University of Burgos, SpainCopyright © 2023 Civiero, Carvalho and Silveira. This is an open-access article distributed under the terms of the Creative Commons Attribution License (CC BY). The use, distribution or reproduction in other forums is permitted, provided the original author(s) and the copyright owner(s) are credited and that the original publication in this journal is cited, in accordance with accepted academic practice. No use, distribution or reproduction is permitted which does not comply with these terms.
*Correspondence: Chiara Civiero, cciviero@icm.csic.es