- Second Crust Monitoring and Application Center, China Earthquake Administration, Xi’an, China
This paper mainly introduces the application and progress of the time-varying gravity in earthquake research in the Chinese Mainland. Since the Xingtai earthquake in 1966, China has begun mobile gravity monitoring, trying to explore the relationship between gravity changes and seismic activities. The gravity changes before and after the Haicheng MS7.3 earthquake in 1975 and the Tangshan MS7.8 earthquake in 1976 were observed. In 1981, a high-precision metal spring gravimeter was introduced to carry out high-precision mobile gravity observation in the key earthquake monitoring areas in western Yunnan. The gravity anomaly changes near the epicenters of the Lijiang MS7.0 earthquakes in 1996 were observed. In 1998, a high-precision absolute gravity survey was introduced to carry out the overall scale gravity field monitoring in the Chinese Mainland, and the large-scale gravity change information before Wenchuan MS8.0 and Yutian MS7.3 earthquakes in 2008 was obtained, and the effective prediction opinions were given. After the Wenchuan MS8.0 earthquake in 2008, the integration of the national network and the regional network accelerated, forming the whole gravity observation network in the Chinese Mainland, which made a relatively successful medium-term prediction for a series of earthquakes with MS6.0 or above (such as Lushan MS7.0, Menyuan MS6.4, and Jiuzhaigou MS7.0) in recent years and played an important role in the study of the earthquake mechanism and earthquake prediction level in China. Finally, the existing problems in time-varying gravity monitoring in China are pointed out, and the prospect of earthquake research using time-varying gravity monitoring data is put forward.
1 Introduction
The time-varying gravity field is the basic physical field reflecting material migration, which directly reflects the nature and process of tectonic movement and surface mass migration in the Earth’s interior. The relationship between earthquakes and gravity is closely linked to the Earth’s internal tectonic movement and mass (density) change. Therefore, we can make repeated observations of the ground gravity regularly and deeply analyze and study the dynamic changes of its gravity field, which is beneficial to timely capture the precursor information on gravity before some strong earthquakes. This is the basic starting point of taking time-varying gravity as the observation means so as to deeply explore the mechanism of earthquake occurrence and carry out earthquake risk prediction.
The relationship between seismicity and gravity variation has been studied for a long time. Although there were reports of gravity field changes before strong earthquakes in the early 20th century, it was not until the application of high-precision gravimeters that the monitoring and research of seismic gravity changes began in the Soviet Union, the United States, Japan, etc., in the 1960s. At the same time, the study of time-varying gravity was regarded as an important means of earthquake prediction and analysis. Before and after the 1964 Alaska earthquake, the 1965–1967 Songdai earthquake swarm in Japan, the 1974 Izu earthquake in Japan, and the 1976 Gazley earthquake swarm in the Soviet Union (Barnes, 1966; Mao and Zhu, 2018), the time-varying information on gravity before earthquakes was observed. The landmark research achievement is that Cal Barnes, a famous geologist, made it clear in 1966 that before and after the earthquake, the local stress field in the earth’s crust changed due to the fault movement, which led to the change of the density of the underground medium at the fault. At the same time, the deformation of the medium would also produce new cracks, which would increase the cracks of the existing medium. In this way, the fluid medium, such as groundwater or volcanic magma, might directly flow in (or out), causing the density of the fluid medium near the observation point to change, which might directly affect the time change of the regional gravity field (Walsh, 1975; Chen et al., 1979).
Seismology is an observational science. Earthquake research depends not only on the development of relevant basic theories but also on high-precision observation data with complete space-time before and after earthquakes. The research results in recent years show that significant gravity anomaly information may be observed in the regional gravity field before strong earthquakes (Lu et al., 1978; Chen et al., 1979; Zhu et al., 2008a; Zhu et al., 2008c; Shen et al., 2009; Shen et al., 2012; Zhu et al., 2013; Chen et al., 2015; Chen et al., 2016; Zhu et al., 2017). Using high-precision gravity measurement data to analyze the spatial distribution characteristics of gravity anomalies before earthquakes is helpful in determining the high-risk areas of future large earthquakes. Recently, researchers have made accurate medium-term predictions of some large earthquakes by using the data on gravity anomaly and the historical data on earthquakes in the study area (Zhu et al., 2005; Zhu et al., 2008a; Zhu et al., 2008c; Zhu et al., 2013; Zhu et al., 2017; Shen et al., 2020; Zhu et al., 2022). This paper briefly summarizes the application of time-varying gravity in earthquake prediction and the research results obtained.
2 Research on the dynamic change of the regional gravity field
The collision of the Indian–Eurasian plate in the west and the subduction of the Pacific plate in the east have caused frequent strong earthquakes and serious earthquake disasters in China’s Mainland (Zhang et al., 2013; Zhou et al., 2017). Therefore, it is a basic national policy of China to actively carry out earthquake prevention and disaster reduction, including earthquake monitoring and prediction, and minimize earthquake risk. At present, China is the only country in the world with the main purpose of earthquake monitoring and prediction, which has built a large-scale ground gravity monitoring network and actively carried out regular gravity resurvey and earthquake prediction (Jiang et al., 2005; Shen et al., 2020).
2.1 Exploration and development stage
In order to explore the relationship between time-varying gravity and seismic activity, after the Xingtai earthquake in 1966, China began to set up mobile gravity monitoring networks, and all kinds of mobile gravity lines across faults were set up in major fault zones in North China, Sichuan, Yunnan, etc. On the gravity survey line from Beizhen to Zhuanghe, Liaoning Province, about 180 ×10–8 ms−2 of gravity change was observed in a year in the survey section of Gaixian–Donghuangdi before the Haicheng MS7.3 earthquake in 1975 (Lu et al., 1978). The gravity field changes before and after the 1976 Tangshan MS7.8 earthquake were obtained through the long baseline gravity joint survey of Beijing–Tianjin–Tangshan–Shanhaiguan; it was found that there was a 100×10–8 ms−2 gravity anomaly change (Li and Fu, 1983; Li et al., 1997), which proves the existence of gravity anomaly before the earthquake. Chen et al. (1979) pointed out in the analysis of the gravity changes before and after the Haicheng earthquake in 1975 and the Tangshan earthquake in 1976 that the gravity change is closely related to the process of earthquake preparation and occurrence. The gravity change caused by the ground elevation change estimated according to the repeated leveling data is much smaller than the observed gravity change. Therefore, it is speculated that the preparation and occurrence of some large earthquakes may be related to the mass migration in the crust and upper mantle. It is considered that most of the observed gravity changes are caused by mass transfer, and the effects of gravity changes caused by deformation and mass transfer are analyzed theoretically, but the physical process of mass transfer is not completely explained. Li et al. (1997) explained the change process of the regional gravity field before and after the Tangshan earthquake by adopting the expansion model and considered that the epicentral area before and after the Tangshan earthquake experienced the process of gravity increase (stress accumulation and compression), gravity decrease (expansion and expansion), earthquake occurrence, and post-earthquake reverse recovery, and the theoretically calculated gravity change value was in good agreement with the actual observation value (Hu et al., 2021). The gravity measurements before and after the Haicheng and Tangshan earthquakes also showed that there would be significant anomaly changes in gravity before large earthquakes, which provided a typical earthquake example for the study of earthquake prediction by using gravity measurement methods.
2.2 Observation and practice stage
Before 1981, gravimeters were mainly of quartz spring type, and their measuring accuracy was about 30×10–8 ms−2. After 1981, due to the introduction of the LaCoste–Romberg (G) (LCR-G) metal spring gravimeter, its measurement accuracy is within 10×10–8 ms−2, which is greatly improved compared with the quartz spring gravimeter (Zhu et al., 2008b). The China Earthquake Administration has successively carried out repeated observations of seismic gravity (mobile gravity) and laid a number of survey networks or lines throughout the country, taking provincial (municipality directly under the Central Government, autonomous region) territories as units, which are self-contained and independent of each other (Shen et al., 2020).
In 1981, the Institute of Geophysics, China Earthquake Administration, using three LCR-G gravimeters provided by the United States, made 2–3 periods of seismic gravity observations in the Beijing–Tianjin–Tangshan–Zhangjiakou area every year to explore the gravity changes and mechanisms during the preparation of strong earthquakes (Gu et al., 1997). The data on seismic gravity measurement in the Beijing–Tianjin–Tangshan–Zhangjiakou area show that the gravity field in this area has obvious regional variation characteristics, and the most obvious is that there is a large-scale increase in gravity in the south of the survey area. The main reason for this change is that the ground subsidence caused by the extensive use of groundwater resources in the south of the survey area leads to an increase in gravity (Lu et al., 2004). The gravity change in the northern mountainous area of the survey area tends to decrease, and the decreasing amplitude is consistent with the change amount of the absolute gravity point of Xiangshan Mountain. The inherited and synchronous tectonic movement in a large mountainous area is the main reason for the trend reduction of the surface gravity change. The gravity change in the eastern part of the survey area was mainly from June 1990 to June 1994, and the gravity change showed a rapid decline and then a rapid increase, which may be the gravity change related to the preparation of the Luanxian MS5.9 earthquake in October 1995 (Lu et al., 2004).
In 1984, the Institute of Seismology, China Earthquake Administration, in cooperation with the University of Hanover in Germany and others, set up a seismic gravity survey network at the West Yunnan Earthquake Prediction Experimental Site and carried out regular repeated surveys for 2–3 periods every year. It was observed that gravity anomalies of about 70×10–8 ms−2 occurred before the Gengma MS7.6 earthquake in Lancang, Yunnan, in 1988 (Wu et al., 1995), gravity anomalies of about 110×10–8 ms−2 occurred before the MS7.3 earthquake on the border between China and Myanmar in 1995 (Wu et al., 1998), and a gravity anomaly of about 120×10–8 ms−2 occurred near the epicenter before the Lijiang MS7.0 earthquake in 1996 (Wu et al., 1997), and it was recognized that the gravity change related to earthquake preparation is not limited to the fault but presents the characteristics of the “field”. Jia et al. (1995) studied the dynamic images of the gravity field of the earthquake experimental site in western Yunnan during 1985–1994 and its corresponding relationship with nine earthquakes with MS >5.0. During the observation period, nine earthquakes with MS >5.0 occurred in the survey area and its adjacent areas, all of which occurred near the zero line of the transition zone between positive and negative anomaly areas. Before the earthquake, there is always a positive anomaly zone, and the larger the magnitude, the larger the range and amplitude of the anomaly zone. The time scale of the gravity anomaly change before the earthquake is about 3 years, and the complete rule is that the gravity field rises first and then falls; earthquakes occur in the process of falling, and the time from turning to earthquake occurrence is within 1 year. Further comparison and analysis of the gravity field change images before the Yongsheng MS5.1 and MS5.4 earthquakes in December 1992, the Dayao MS5.3 earthquakes in February 1993, and the Lijiang MS7.0 earthquakes in February 1996 show that the positive anomaly areas before the Yongsheng MS5.1 and MS5.4 earthquakes in December 1992 and the Dayao MS5.3 earthquakes in February 1993 are obvious and complete, and the gravity change gradient zones and negative anomaly areas in the southwest and northeast are clearly visible, which are two earthquake-prone areas. Combined with other relevant data, an accurate prediction opinion was put forward before the Yongsheng and Dayao earthquakes (Jia and Zhan, 2000). The magnitude of the gravity positive anomaly area before the Lijiang MS7.0 earthquake in 1996 is seven times that before the Dayao MS5.3 earthquake in 1993, and the anomaly area has a larger range, but the anomaly shape is incomplete, which is related to the relatively small survey network in the experimental site of western Yunnan. Therefore, it is clearly pointed out that to accurately judge the epicenter position before the earthquake of magnitude 7, it is necessary to have a range of 900 km × 900 km in the survey area, while the range of the gravity network in western Yunnan is only 300 km × 300 km. In fact, the gravity researchers of the Yunnan Earthquake Administration have long been aware of the gravity anomaly changes before the 1996 Lijiang earthquake and have made some predictions about this earthquake (Wu et al., 1997; Shen et al., 2003).
Around 1990, China built a regional gravity survey network with independent units of provinces, municipalities, and autonomous regions in North China, the North–South seismic belt, the southeast coast, and other regions. Some of the survey networks are network-like and some are strip-like, with an average range of less than 300 km × 300 km (Shen et al., 2020; Zhu et al., 2020). There are 20 companies carrying out field monitoring of mobile gravity. The gravity anomaly around the epicenter before and after the Zhangbei MS6.2 earthquake in Hebei in 1998 (Zhang and Sun, 2001) and the gravity anomaly near the epicenter before and after the Datong MS5.6 earthquake in Shanxi in 1999 (Li and Qin, 2001) were monitored, and the gravity anomaly at the junction area of Jiangsu, Shandong, and Anhui before the Cangshan MS5.2 earthquake in Shandong in 1995 (Liu, 1997) was also monitored. Before the Yongdeng MS5.8 earthquake in Gansu in 1995 and the Jingtai MS5.9 earthquake in Gansu in 2000, an obvious gravity anomaly change was also observed in the Hexi area of North Qilian, and the medium-term predictions of these two earthquakes were made to some extent (Jiang et al., 1998; Zhu et al., 2001). During the period from 1992 to 1994, significant gravity anomalies occurred in Gulang and Wuwei areas, and the spatial range with gravity variation amplitude greater than 50 ×10–8 ms−2 was more than 100 km in diameter. The Gulang–Tianzhu–Yongdeng area was a gravity variation high-gradient zone connected with the high-value area of gravity variation. The epicenter of the Yongdeng MS5.8 earthquake on 22 July 1995 was located at the edge of this gravity variation high-gradient zone (Jiang et al., 1998). Before the Jingtai MS5.9 earthquake in June 2000, there were two changes in the gravity field from 1998 to 1999, which were similar to those before the Yongdeng earthquake: 1) the regional gravity field showed a wide range of trend anomalies, with a high-gradient zone of gravity change along the main fault tectonic belt of Qilian Mountain, negative gravity changes in mountainous areas, and relatively positive changes in basins; 2) there are many local anomaly areas with uneven spatial changes near the epicenter of the Jingtai MS5.9 earthquake in the east of the survey area (Zhu et al., 2001). Zhu et al. (2004) further expanded the research range and analyzed the dynamic evolution characteristics of the regional gravity field from 1992 to 2001 and its relationship with the preparation and occurrence of the Yongdeng MS5.8 and Jingtai MS5.9 earthquakes by using the gravity observation data on the northeast margin of the Qinghai–Tibet block obtained by the unified starting datum. It is considered that the gravity observation data on the northeast margin of the Qinghai–Tibet block obtained by overall calculation can more completely reflect the complete precursor information on the gravity field changes during the seismogenic process of the Yongdeng MS5.8 and Jingtai MS5.9 earthquakes, and the dynamic change image of the gravity field can clearly reflect the relationship between the orderly evolution process of the regional gravity field and seismic activity (Zhu et al., 2004).
2.3 Application promotion stage
In 1998, the China Earthquake Administration, together with the State Bureau of Surveying and Mapping, the General Staff Bureau of Surveying and Mapping, the China Academy of Sciences, and four other ministries and commissions, built the “crustal movement observation network of China” project (referred to as the network project). With the implementation of the network project, a unified national seismic gravity network in the Chinese Mainland was built. The gravity joint survey was carried out on 25 reference stations, 56 basic stations, and more than 300 regional stations (or transition points), and the absolute gravity values of the 25 reference stations were also measured. The absolute gravity measurement is made using an FG-5 absolute gravimeter, and the absolute gravity measurement accuracy at each reference station is better than 5×10−8 ms−2. The relative gravity joint measurement is made by LCR-G, Burris, or CG5/6 gravimeter, and the joint measurement accuracy of gravity segment difference is better than 10×10−8 ms−2. Since 1998, the network project has carried out a joint survey of the Chinese Mainland gravity basic network every 2–3 years. In the earthquake-prone areas such as Sichuan–Yunnan and the northeastern edge of the Qinghai–Tibet Plateau, the Seismological Research Institute of the China Earthquake Administration and the Second Crust Monitoring and Application Center of the China Earthquake Administration jointly completed the joint survey of the GNSS stations in the regional gravity survey network. Connect isolated provincial gravity networks into larger regional gravity networks (Zhu et al., 2008b; Shen et al., 2020), and observe and study the dynamic change of regional gravity field and its relationship with strong earthquake preparation.
Li et al. (2009) used the network project gravity observation data to obtain the dynamic change image of the gravity field in the Chinese Mainland at the scale of 2–3 years since 1998, which better reflects the basic contour of the crustal tectonic movement and major strong earthquake activities in the Chinese Mainland. Zhu et al. (2012) obtained the dynamic change images of gravity field in the Chinese Mainland area based on absolute gravity by using the gravity observation data on a network project from 1998 to 2008 and considered that the change of gravity field in the Chinese Mainland not only has the phenomenon of uneven spatial and temporal distribution and zoning of gravity change but also is closely related to active fault structures and earthquake preparation and development. Jiang et al. (2003) analyzed that the gravity difference in the Wudaoliang–Altyn region near the epicenter of the MS8.1 earthquake in the west of the Kunlun Pass in Qinghai in 2001 reached 100 × 10–8 ms−2。. Wang et al. (2004) used repeated gravity observation data to analyze the relationship between co-seismic dislocation and gravity variation of the Lijiang MS7.0 earthquake. Zhang and Zhang (2000) analyzed the gravity change of the Lijiang MS7.0 earthquake based on the mechanics theory, multiplication decomposition theory, and numerical simulation in porous media and proposed that the gravity change experienced the elastic stage of the body, the elastic-plastic stage of the deformation of the body and the hole, and the stress release during the co-seismic dislocation, and then, the hole closed again (Mao and Zhu, 2018). Shen et al. (2011) analyzed the variation characteristics of the gravity field before the Yao’an MS6.0 earthquake in Yunnan in 2009 in combination with the focal mechanism solution, pointed out that the four-quadrant distribution image of the relative gravity change in the epicenter area reflected the existence of shear stress in the seismogenic area before the earthquake (Shen et al., 2020), and proposed the precursor model of “locking shear.” Zhu et al. (2009), Zhu et al. (2012), and Zhu et al. (2015b) have studied that strong earthquakes are prone to occur in the high-gradient zone where the positive and negative gravity change transition areas along the tectonic active fault, and the turning part of the gravity changes the contour line. Due to the strong differential movement and the strongest discontinuity of tectonic deformation, the tectonically active fault zone is prone to produce severe gravity changes, which is conducive to the high accumulation of stress and the preparation of earthquakes. In addition, accurate medium-term predictions have been made for the large earthquakes of MS7.3 in Yutian, Xinjiang, and MS8.0 in Wenchuan, Sichuan, in 2008.
2.4 Overall optimization stage
After the 2008 Wenchuan 8.0 earthquake, the China Earthquake Administration summarized and reflected on the advantages and limitations to the seismic gravity field observation in the Chinese Mainland and believed that it was necessary to connect the regional gravity measurement network into a whole and form a unified observation benchmark. According to the idea of “nationwide and regional networking,” the existing conventional gravity observation tasks were coordinated (Zhu et al., 2020), and the construction of seismic gravity monitoring network and observation technology was developed rapidly. In 2009, the special task of “Strengthening, Monitoring, and Tracking of Strong Earthquakes in North China” was launched to adjust, optimize, and transform the scattered provincial seismic gravity network in North China, effectively connecting the gravity measurement network of the relevant provincial Earthquake Administration and strengthening absolute gravity control to form a new gravity monitoring network in North China as a whole (Zhu et al., 2019). In 2010, the encrypted monitoring network of the gravity field change of “Comprehensive Geophysical Field Observation of China (CGFOOC)” a key scientific research project in the earthquake industry, was launched. This project took the national basic gravity network as the overall framework and optimized and transformed the existing seismic gravity monitoring networks in the eastern margin of the Qinghai–Tibet Plateau, the surrounding areas of Ordos, and the North China by stages, connecting the scattered regional gravity networks (Hao et al., 2015; Hu et al., 2015; Zhu et al., 2019; Wei et al, 2020). In 2010, the Chinese Mainland Tectonic Environment Monitoring Network optimized and upgraded the gravity survey network on the basis of the Crustal Movement Observation Network of China and conducted joint surveys of 100 reference stations (absolute gravity survey points) and more than 600 joint survey points in the Chinese Mainland region (Xing et al., 2016; Shen et al., 2020). Through the Chinese Mainland Tectonic Environment Monitoring Network, Comprehensive Geophysical Field Observation of China, North China’s strong earthquakes, and other large-scale key earthquake monitoring projects, the “scattered distribution” seismic gravity monitoring network has been optimized and transformed many times in its overall structure and gradually built into the Chinese Mainland mobile gravity seismic monitoring network consisting of the relative gravity joint measurement network and the absolute gravity control network in the Chinese Mainland (Figure 1), with regular observations every year. In addition, gravity exploration was carried out for the crustal structure of the North–South seismic belt (Zhu et al., 2019; Shen et al., 2020). According to the national gravity field change image obtained under the unified benchmark, it has played an important role in annual earthquake prediction, especially in location prediction. Many researchers have made an in-depth summary of the relationship between the gravity field change images and earthquakes (Li et al., 2009; Li et al., 2010; Zhu et al., 2010; Zhu et al., 2012; Zhu et al., 2014; Zhu et al., 2015a; Hu et al., 2019; Shen et al., 2020). Figure 1 shows the epicenters of eight earthquakes with magnitude of more than 7 in the Chinese Mainland, including the Wenchuan MS8.0 and Yutian MS7.3 earthquakes in 2008, the Yushu MS7.1 earthquake in 2010, the Lushan MS7.0 earthquake in 2013, the Yutian MS7.3 earthquake in 2014, the Jiuzhaigou MS7.0 earthquake in 2017, and the Maduo MS7.4 earthquake in 2021, since the Kunlun Mountain Pass West MS8.1 earthquake in 2001. It can be seen that there are some gravity measuring points near the epicenter of the seven other major earthquakes, except that there are no gravity measuring points near the epicenter of the Kunlun Mountain Pass west MS8.1 earthquake in 2001. In particular, there are gravity monitoring points around the epicenter of the Wenchuan MS8.0 earthquake in 2008, the Lushan MS7.0 earthquake in 2013, and the Jiuzhaigou MS7.0 earthquake in 2017, which laid a good foundation for extracting precursory information on gravity changes before strong earthquakes. In recent years, significant gravity anomalies have been observed in mobile gravity before many strong earthquakes in the Chinese Mainland. Although the manifestations of the anomalies are different, some of them occur in the high-gradient zone and zero isoline area where the positive and negative gravity anomaly areas undergo transition and some of them occur in the center of the distribution characteristics of the four-quadrants of gravity anomalies. However, according to the range and amplitude of gravity anomaly areas, the magnitude, and characteristics of the gravity anomaly gradient, we can study the location and magnitude of potential strong earthquakes (Zhu et al., 2019). Zhu et al. (2018), Zhu et al. (2019), Zhu et al. (2020), and Zhu et al. (2022), on the basis of an in-depth study on the evolution characteristics and laws of regional gravity fields with different time and space scales in the Chinese Mainland, proposed that regional gravity fields with multiple time and space scales should be used as the basis for analyzing and studying the dynamic images of gravity fields. The prediction method and judgment index of earthquake risk analysis based on the gravity field change trend, gravity anomaly shape, gravity anomaly area range, amplitude, duration, gravity anomaly gradient, and its characteristics in different time and space scales (adjacent two periods of gravity change, year-scale gravity change, cumulative gravity change, etc.) are proposed in Table 1.
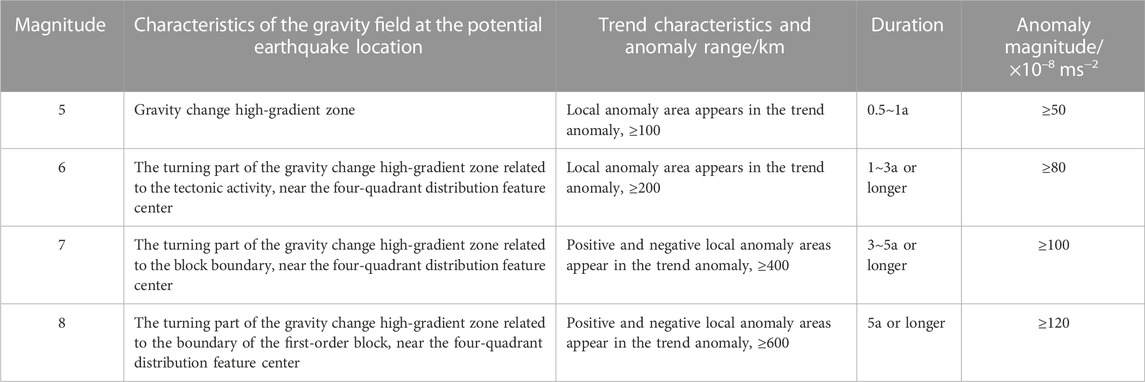
TABLE 1. Relation between the gravity anomaly and earthquake preparation (Zhu et al., 2022).
3 Time-varying gravity and strong earthquake prediction
Earthquakes are a manifestation of deformation and rupture of the medium in the crust, and the observation of gravity field changes during the earthquake preparation period can help in studying the relationship between the accumulation of the elastic strain in the crust medium and the occurrence of earthquakes (Shen et al., 2009; Chen et al., 2015). By analyzing the dynamic changes of the gravity field before and after the earthquake, we can study the source parameters, the rupture process, and the post-earthquake recovery viscoelastic deformation (Fu and Liu, 2017; Fu and Wang, 2020). At present, the gravity field change data obtained based on surface mobile gravity observation is gradually increasing, and with the continuous improvement of instrument performance indicators and the coverage of gravity survey network, much progress has been made in the study of the relationship between the dynamic changes of gravity field obtained through repeated surface gravity observation and tectonic activity (Chen et al., 2015; Shen et al., 2020; Zhu et al., 2020; Zhu et al., 2022). A large number of research results show that the gravity time-varying anomaly is one of the reliable precursor anomalies related to earthquake preparation, especially for the seismogenic process of earthquakes with MS ≥ 6.
1) Prediction of the Wenchuan MS8.0 earthquake in Sichuan in 2008 (Zhu et al., 2008a; Zhu et al., 2010).
Prediction basis: The cumulative gravity change in the Sichuan–Yunnan region during 1998–2005 shows that there is a large range of positive gravity change anomaly area in the Sichuan–Yunnan rhombic block and a large range of negative gravity change anomaly area in the Sichuan region in the north of the Sichuan–Yunnan rhombic block. The difference between the two anomaly areas is more than 100 ×10–8 ms−2, and a high-gradient zone of gravity change is formed in the Maerkang–Wenchuan–Chengdu area (Figure 2).
Prediction opinion: earthquake occurrence time: 2007–2008; location of the earthquake: 103.7°E, 31.6°N as the center, with a radius of 200 km; magnitude: 6–7.
Results: The MS8.0 Wenchuan earthquake (103.4°E, 31.0°N) occurred in the prediction area on 12 May 2008. The predicted epicenter was 72 km away from the MS8.0 Wenchuan earthquake (Figure 2) and between the two extreme earthquake areas of Beichuan and Yingxiu (Figure 3). The time and place were correct, and magnitude 7 was mentioned.
2) Prediction of the Yutian MS7.3 earthquake in Xinjiang in 2008 (Zhu et al., 2008c; Zhu et al., 2012).
Prediction basis: The regional gravity change in a long period from 1998 to 2005 shows that there is a significant gravity anomaly in the border area between Xinjiang and Tibet, with the maximum difference of more than 90 × 10–8 ms−2, and a significant gravity gradient zone has been formed along the boundary zone of tectonic blocks in the Yutian–Hetian area of Xinjiang.
Prediction opinion: earthquake occurrence time: 2007–2008; location of the earthquake: 80.0° E, 36.0° N as the center, with a radius of 200 km; magnitude: six to seven.
Results: The Yutian MS7.3 earthquake (81.6° E, 35.6° N) occurred in the prediction area on 21 March 2008. The predicted epicenter is 149 km away from the Yutian MS7.3 earthquake, which was very accurate in areas with weak earthquake monitoring ability (the distance between the measuring points in the border area between Xinjiang and Tibet is 100–200 km, and the measuring points are sparse).
After the Wenchuan earthquake in 2008, the Gravity Discipline Management Department of the China Earthquake Administration strengthened the research on gravity monitoring and prediction and actively explored the use of gravity observation data to predict the annual risk of earthquakes, made full use of increasingly abundant gravity observation data, and deeply and carefully studied the dynamic evolution characteristics and laws of regional gravity field on the basis of fine processing of regional gravity network observation data, searched for tectonic activity and earthquake precursor information from the complex and changeable gravity anomaly phenomena, carried out a retrospective study of earthquake cases, tracked and analyzed the regional gravity field anomaly changes and possible medium and short-term anomaly information, and proposed a variety of gravity field change characteristic models with different space–time scales that have prediction significance before strong earthquakes (Zhu et al., 2013; Chen et al., 2015; Zhu et al., 2016; Shen et al., 2020; Zhu et al., 2020). On the basis of strong earthquake prediction methods and judgment indicators, annual prediction and post-earthquake trend determination of seismic risk in key monitoring areas are carried out using the dynamic change data on the gravity field, which has played an important role in the determination of strong earthquake locations in key seismic risk areas in the Chinese Mainland and has achieved a certain degree of effectiveness in disaster reduction. In recent years, we have predicted many earthquakes, such as the Sichuan Lushan MS7.0 and Gansu Minxian MS6.6 earthquakes in 2013, the Yunnan Ludian MS6.5 and Xinjiang Yutian MS7.3 earthquakes in 2014, the Qinghai Menyuan MS6.4 earthquake in 2016, the Sichuan Jiuzhaigou MS7.0 earthquake in 2017, the Xinjiang Jiashi MS6.4 earthquake in 2020, the Yunnan Yangbi MS6.4 earthquake in 2021, and the Qinghai Menyuan MS6.9 earthquake and Sichuan Luding MS6.8 earthquake in 2022, especially the determination of the location of strong earthquakes. The following table lists the prediction of more than 10 strong earthquakes in the Chinese Mainland since 2013 by gravity observation data in recent years, as shown in Table 2.
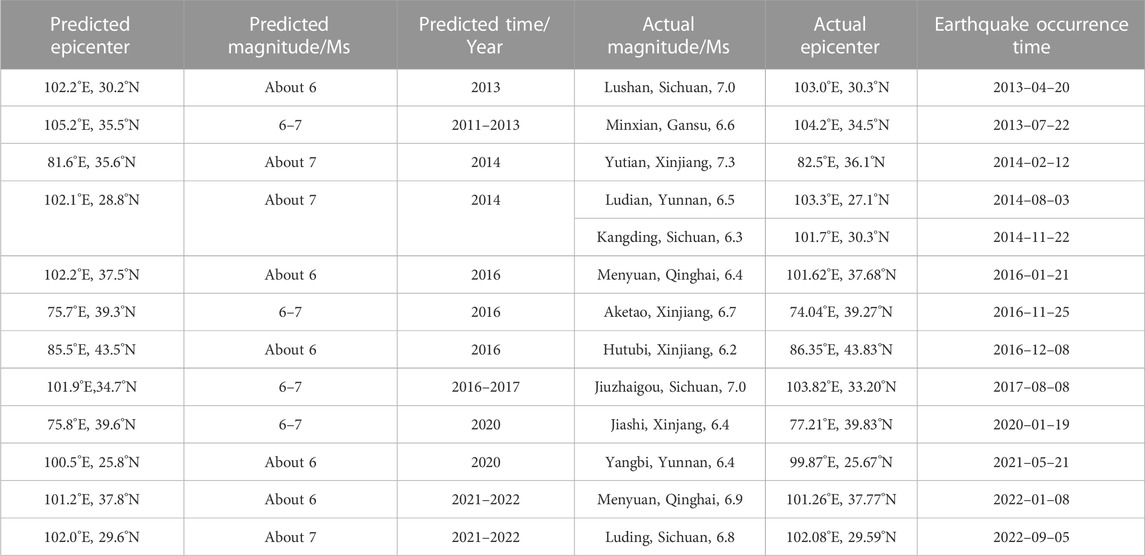
TABLE 2. Midterm prediction information on strong earthquakes with magnitude 6 or above since 2013 (Zhu et al., 2022).
As can be seen from Table 2, in recent years, the medium-term prediction of strong earthquakes/large earthquakes with magnitude 6 or above occurred in areas with monitoring ability in the western Chinese Mainland has been made accurately by using mobile gravity data. However, there are also cases of strong earthquakes which were missed, such as the earthquakes with MS6.9 in Milin, Tibet, on 18 November 2017, and the earthquake with MS6.6 in Nima, Tibet, on 23 July 2020; all occurred in areas with weak gravity monitoring capability on the Qinghai–Tibet Plateau, so they cannot be effectively monitored.
In general, the mobile gravity observation data can better reflect the gravity anomaly changes in the process of earthquake preparation and occurrence, especially for strong earthquakes with a magnitude of 6 or above. Through timely and careful analysis and research of mobile gravity observation data, it is possible to make more accurate medium-term predictions of future strong earthquakes/large earthquakes, especially the determination of strong earthquake locations. For example, in the prediction of the locations of the Menyuan MS6.9 and Luding MS6.8 earthquakes in Qinghai in 2022 (see Table 2), the predicted epicenters of the two earthquakes are less than 10 km away from the epicenter of the earthquake, which is basically the same (Zhu et al., 2022).
4 Problems and prospects
Obtaining reliable earthquake precursor information is the precursor of realizing earthquake prediction. Gravity change measurement is the most precise and difficult work in geodesy at present, and seismic monitoring acquires 10–8 ms−2 level changes. The observation technology is very important, and data processing is also very critical. At present, the relative gravity measurement under the control of absolute gravity is the main method, so data processing is particularly important. The data processing and analysis should be strengthened to ensure the reliability of the first-hand data. In addition, gravity changes caused by different environmental and physical factors should also be considered, such as environmental changes, groundwater, air pressure, and post-glacial rebound.
4.1 Problems
In China, gravimetry, as a means of studying and predicting earthquakes, has gone through a history of more than 50 years. Through constant exploration and practice, it has gradually recognized and captured the typical gravity variation characteristics related to the preparation and occurrence of strong earthquakes, providing an important basis for the mid-year and annual earthquake trend discussion of the China Earthquake Administration, and it has obtained many successful cases of mid-term prediction (Mao and Zhu, 2018; Shen et al., 2020; Zhu et al., 2022). Before the strong earthquake comes, there are four-quadrant of ascending and descending changes or the gravity gradient zone along the main tectonic belts, and the epicenter is often located in the low-value area of gravity isoline, which is also the successful experience of earthquake research and prediction by gravity monitoring in recent 20 years. Shen et al. (2011) and Shen et al. (2020) put forward the basic criterion for judging large earthquakes and the locking shear model from the point of view of energy accumulation and rupture and considered that tectonic earthquakes originated from the deep crust, and when the stress and strain energy of the medium accumulated to a certain extent, it would cause shear rupture, resulting in the four-quadrant distribution characteristics of the surface gravity field along the fault tectonic belt. The four-quadrant distribution image of the relative change in the epicenter area reflected the pre-existing shear stress of the seismogenic body, which provided a theoretical basis for earthquake prediction and mechanism explanation. Many researchers have carried out a lot of mathematical statistics and analysis based on the relationship between the anomaly range, magnitude and duration of gravity changes, and strong earthquakes in previous earthquake cases (Jia and Zhan, 2000; Hu et al., 2019; Zhu et al., 2019; Shen et al., 2020; Zhu et al., 2022), which provides methodological experience for earthquake prediction. Although gravity time-varying monitoring has gained successful experience in earthquake monitoring and research in the Chinese Mainland, it is still insufficient to meet the needs of disaster reduction, such as earthquake prediction and prevention, and there are mainly the following problems:
1) The actual monitoring ability of gravity directly determines the ability to study the evolution of the gravity field and its relationship with seismic activity in key areas of the Chinese Mainland. The current monitoring network in the Chinese Mainland is unevenly distributed, and the gravity monitoring points are mainly distributed in the North China and the North-South seismic belt, while being less distributed in the Qinghai–Tibet Plateau and its surrounding areas, with an insufficient spatial and temporal resolution, and the information obtained is incomplete. Therefore, it is still incapable of monitoring strong earthquakes with magnitude 6, and it is impossible to effectively capture the complete precursor information during the preparation and occurrence of strong earthquakes.
2) The calibration and control degree of absolute gravity are relatively low, and the absolute gravity and relative gravity are not measured in a quasi-synchronous manner, which cannot eliminate the system error caused by the calibration of the scale value of the relative gravimeter. The fusion degree of continuous gravity and flowing gravity is relatively low, and the number of micro-drift and high stability instruments represented by superconducting gravimeters is scarce.
3) Time-varying gravity measurement is the most precise and difficult work in current seismic monitoring, and what seismic gravity monitoring obtains is the 10–8 ms−2 level change. The observation technology is very important, and the timeliness of data processing is also critical. The Chinese Mainland Basic Gravity Network is jointly completed by several ministries and commissions. As a result of the unsynchronized observation, the fieldwork lasts for 5–6 months, or even longer, so it is impossible to timely and effectively integrate all monitoring data. Therefore, the timeliness of data processing is particularly important to ensure the timeliness and reliability of the calculation results.
4) In the study of time-varying gravity, the influence of structure and water is the biggest factor that causes the change of ground gravity. How to deduct the influence of surface ice and snow, lakes, and groundwater on the change of gravity and effectively extract the signal reflecting the change of structure and dynamics is an urgent task.
5) In terms of in-depth understanding of the characteristics of gravity field changes before earthquakes, some models such as mass migration, density change, fault dislocation and creep, joint expansion, and locking shear force have been put forward successively, and some progress has been made in revealing the physical mechanism of gravity field changes. However, earthquake prediction is an international scientific problem, so we should actively carry out international cooperation and vigorously develop strong earthquake numerical prediction and application research based on various gravity observation data through a large number of anatomical studies of earthquake cases.
4.2 Outlook
Among all kinds of natural disasters, earthquake is the one that has the most serious impact on human life. It is conceivable that it is very important for the benefit of mankind to accurately predict when and where a big earthquake will occur through earthquake monitoring and prediction. However, it is very difficult to predict earthquakes. Earthquake prediction has always been recognized as a scientific problem in the world, and its mechanism is extremely complex, but it is not completely unknown. The occurrence of earthquakes is the deformation process of underground tectonic movement from slow to fast. The question is whether it can be observed and recorded, and even if it is observed, whether it can be identified (Ma, 2016).
In recent years, thanks to the continuous optimization and improvement of the Chinese mainland Seismic Gravity Observation Network, mobile gravity plays an increasingly important role, and many successful cases of medium-term predictions have been obtained. However, at present, there are few absolute gravimeters, and there is a certain zero drift in relative gravimeters, which greatly reduces the efficiency of ground flow observation data. Therefore, in the long run, we should develop high-precision absolute gravity observation technology to replace relative gravity observation technology. Low-altitude satellite gravity observation technology should be developed to make up for the deficiency of ground gravity observation technology (Zhu et al., 2020). In the future, with the progress of observation technology and the enhancement of spatial–temporal monitoring resolution of the gravity field, based on the fusion of multi-source gravity observation data and the further study of the relationship between gravity anomaly and seismic activity, the role of time-varying gravity in earthquake prediction and research will become increasingly significant.
Author contributions
YZ and XY both contributed to topic selection, writing, and editing of the manuscript. FL contributed to the discussion section, and review and editing of the manuscript. YZ and SW contributed to data processing, and review and editing of the manuscript. GZ contributed to drawing, review, and editing of the manuscript.
Funding
This research was funded by the National Natural Science Foundation of China (No.41874092, No.U1939205). The authors express their appreciation to the National Natural Science Foundation of China (No.41874092, No.U1939205) for the financial support of this work.
Conflict of interest
The authors declare that the research was conducted in the absence of any commercial or financial relationships that could be construed as a potential conflict of interest.
Publisher’s note
All claims expressed in this article are solely those of the authors and do not necessarily represent those of their affiliated organizations, or those of the publisher, the editors, and the reviewers. Any product that may be evaluated in this article, or claim that may be made by its manufacturer, is not guaranteed or endorsed by the publisher.
References
Barnes, D. F. (1966). Gravity changes during the Alaska earthquake. J. Geophys. Res. 71 (2), 451–456. doi:10.1029/jz071i002p00451
Chen, S., Liu, M., Xing, L. L., Xu, W., Wang, W., Zhu, Y., et al. (2016). Gravity increase before the 2015 MW7.8 Nepal earthquake[J]. Geophys. Res. Lett. 43, 111–117. doi:10.1002/2015gl066595
Chen, S., Xu, W. M., and Jiang, C. S. (2015). Relationship between gravity variation and seismic hazards in the Western China[J]. Acta Seismol. Sin. 37 (4), 575–587. (in Chinese). doi:10.11939/jass.2015.04.005
Chen, Y. T., Gu, H. D., and Lu, Z. X. (1979). Variations of gravity before and after the Haicheng earthquake, 1975, and the Tangshan earthquake, 1976. Phys. Earth Planet. Interiors 18, 330–338. doi:10.1016/0031-9201(79)90070-0
Fu, G. Y., and Liu, T. (2017). A user-friendly code for Calculating post-seismic displacements and gravity changes on a symmetric viscoelastic spherical Earth[J]. J. Geodesy Geodyn. 37 (7), 661–667. (in Chinese). doi:10.14075/j.jgg.2017.07.001
Fu, G. Y., and Wang, Z. Y. (2020). Crustal structure, isostatic anomaly and flexure mechanism around the Jinghe Ms6.6 earthquake in Xinjiang[J]. J. Geophys. 63 (6), 2221–2229. (in Chinese).
Gu, G. X., Kuo, J. T., and Liu, K. R. (1997). Seismogenesis and occurrence of earthquakes as observed by temporally continuous gravity variations in China[J]. Chin. Sci. Bull. 42 (18), 1919–1930.
Hao, H. T., Li, H., Hu, M. Z., and Zhu, Y. Q. (2015). Gravity variation observed by scientific expedition of Lushan earthquake[J]. J. Geodesy Geodyn. 35 (2), 331–335. (in Chinese). doi:10.14075/j.jgg.2015.02.035
Hu, M. Z., Hao, H. T., and Han, Y. F. (2021). Gravity flexural isostasy background of the 2021 Maduo(Qinghai)Ms7.4 earthquake and gravity change before the earthquake[J]. J. Geophys. 64 (9), 3135–3149. (in Chinese). doi:10.6038/cjg2021O0527
Hu, M. Z., Hao, H. T., and Li, H. (2019). Quantitative analysis of gravity changes for earthquake prediction[J]. Earthq. Res. China 35 (3), 417–430. (in Chinese).
Hu, M. Z., Li, H., and Liu, Z. X. (2015). The gravity change over Sichuan-Yunnan region in 2010-2013 and the earthquake monitoring ability of the gravimetric network[J]. J. Geodesy Geodyn. 35 (4), 616–620. (in Chinese). doi:10.14075/j.jgg.2015.04.016
Jia, M. Y., Xing, C. F., and Sun, S. A. (1995). Two dimensional image of gravity variation in Western Yunnan and its relationship with earthquakes above magnitude 5 (MS)[J]. Crustal Deformation Earthquakes 15 (3), 9–19. (in Chinese).
Jia, M. Y., and Zhan, J. H. (2000). The structure and ability of the China seismological gravity monitoring system[J]. Acta Seismol. Sin. 22 (4), 360–367. (in Chinese). doi:10.3321/j.issn:0253-3782.2000.04.004
Jiang, Z. S., Ren, J. W., and Li, Z. X. (2005). Strategic countermeasures for promoting earthquake prediction research[J]. Recent Dev. World Seismol. 35 (5), 168–173. (in Chinese).
Jiang, Z. S., Zhang, X., and Zhu, Y. Q. (2003). Regional tectonic deformation background before the MS8.1 earthquake in the west of the Kunlun Mountains Pass[J]. Sci. China (Series D) 33 (S1), 163–172. (in Chinese). doi:10.3969/j.issn.1674-7240.2003.z1.018
Jiang, Z. S., Zhu, Y. Q., and Wang, Q. L. (1998). On fault deformation and geodynamic characteristics during seismogenic progress of Yongdeng MW5.8 earthquake[J]. Acta Seismol. Sin. 20, 264–271. doi:10.1088/0256-307X/16/9/027
Li, H., Shen, C. Y., and Sun, S. A. (2009). Dynamic gravity change in recent years in China continent[J]. J. Geodesy Geodyn. 29 (3), 1–10. (in Chinese). doi:10.3969/j.issn.1671-5942.2009.03.001
Li, H., Xu, R. G., and Shen, C. Y. (2010). Fractal characteristics of seismological dynamic gravity network in northern China. J] J. Geodesy Geodyn. 30 (5), 15–18. (in Chinese). doi:10.3969/j.issn.1671-5942.2010.05.003
Li, Q. L., and Qin, J. Z. (2001). Variation of gravity field and deep dynamic process before and after Datong MS5.6 earthquake [J]. Crustal Deformation Earthq. 21 (4), 43–51. (in Chinese). doi:10.3969/j.issn.1671-5942.2001.04.006
Li, R. H., and Fu, Z. (1983). The gravity changes before and after the Tangshan earthquake (M=7.8) and dilatation process[J]. Tectonophysics 97, 159–169. doi:10.1016/0040-1951(83)90143-9
Li, R. H., Huang, J. L., and Li, H. (1997). The mechanism of gravity changes before and after the Tangshan earthquake[J]. Acta Seismol. Sin. 19, 399–407. (in Chinese).
Liu, C. H. (1997). Temporal variation of gravity field in northeast Anhui and the junction of Anhui and Jiangsu before cangshan MS5.2 earthquake[J]. Crustal Deformation Earthq. 17 (1), 109–111. (in Chinese).
Lu, H. Y., Zheng, J. H., and Liu, D. F. (2004). Gravity variation in beijing Tianjin tangzhang area from 1985 to 2003[J]. Prog. Geophys. 19 (4), 887–892. (in Chinese).
Lu, Z. X., Fang, C. L., and Shi, Z. T. (1978). Variation of the gravity field and the Haicheng earthquake[J]. Chin. J. Geophys. 21 (1), 1–8. (in Chinese).
Ma, J. (2016). On “whether earthquake precursors help for prediction do exist”. Chin. Sci. Bull. 61 (Z1), 409–414. (in Chinese). doi:10.1360/n972015-01239
Mao, J. L., and Zhu, Y. Q. (2018). Progress in the application of ground gravity observation data in earthquake prediction[J]. Adv. Earth Sci. 33 (3), 236–247. (in Chinese).
Shen, C. Y., Li, H., and Fu, G. Y. (2003). Study on a gravity precursor sormode of Lijiang earthquake with MS=7.0[J]. Acta Seismol. Ainica 25 (2), 163–171. (in Chinese).
Shen, C. Y., Li, H., and Sun, S. A. (2009). Dynamic variations of gravity and the preparation process of the Wenchuan MS8.0 earthquake[J]. Chin. J. Geophys. 52 (10), 2547–2557. (in Chinese). doi:10.3969/j.issn.0001-5733.2009.10.013
Shen, C. Y., Tan, H. B., and Hao, H. T. (2011). Mechanism of precursory gravity change before Yaoan MS6.0 earthquake in 2009[J]. J. Geodesy Geodyn. 31 (2), 17–47. (in Chinese). doi:10.3969/j.issn.1671-5942.2011.02.005
Shen, C. Y., Xing, L. L., and Tan, H. B. (2012). The absolute gravity change in the eastern margin of the Qinghai-Tibetan Plateau before and after Yushu MS7.1 earthquake in 2010[J]. Prog. Geophys. 27 (6), 2348–2357. (in Chinese). doi:10.6038/j.issn.1004-2903.2012.06.010
Shen, C. Y., Zhu, Y. Q., and Hu, M. Z. (2020). Monitoring of gravity field time variation and prediction of strong earthquakes in Chinese mainland[J]. Earthq. Res. China 36 (4), 729–743. (in Chinese).
Walsh, J. B. (1975). An analysis of local changes in gravity due to deformation. Pure Appl. Geophys. 113, 97–106. doi:10.1007/bf01592902
Wang, Y., Zhang, W. M., and Zhan, J. G. (2004). Gravity change detected by repeated absolute gravity measurements in the Western Yunnan and Lhasa China and its implication[J]. Chin. J. Geophys. 47 (1), 95–100. (in Chinese). doi:10.3321/j.issn:0001-5733.2004.01.014
Wei, S. C., Zhu, Y. Q., and Zhao, Y. F. (2020). Study on characteristics of gravity variation before and after Hutubi MS6.2 earthquake[J]. Seismol. Geol. 42 (4), 923–935. (in Chinese). doi:10.3969/j.issn.0253-4967.2020.04.010
Wu, G. H., Luo, Z. X., and Lai, Q. (1998). Gravity variation of West Yunnan test site before the MS7.3 earthquake on the China Myanmar border in Menglian, Yunnan[J]. Earthquake 18 (2), 146–154. (in Chinese).
Wu, G. H., Luo, Z. X., and Lai, Q. (1995). Lancang Gengma earthquake in 1988 and gravity variation at the test site in Western Yunnan[J]. Crustal Deformation Earthquakes 15 (2), 66–73. (in Chinese).
Wu, G. H., Luo, Z. X., and Lai, Q. (1997). Variation characteristics of gravity anomaly in West Yunnan test site before and after Lijiang MS7.0 earthquake[J]. Earthq. Res. 20 (1), 101–107. (in Chinese).
Xing, L. L., Li, H., and Li, J. G. (2016). Establishment of absolute gravity datum in CMONOC and its application[J]. Acta Geod. Cartogr. Sinica 45 (5), 538–543.
Zhang, J., and Sun, B. C. (2001). Discussion on gravity anomaly and anomaly mechanism before Zhangbei MS6.2 earthquake[J]. Earthquake 21 (2), 75–78. (in Chinese). doi:10.3969/j.issn.1000-3274.2001.02.012
Zhang, P. Z., Deng, Q. D., and Zhang, Z. Q. (2013). Active fault, earthquake hazards and associated geodynamic processes in continental China [J]. Scientia Sinica Terrae 43 (10), 1607–1620. (in Chinese).
Zhang, Y. Z., and Zhang, K. S. (2000). The gravity variation during the breaking process in Earth crust[J]. Crustal Deformation Earthq. 20 (1), 8–16. (in Chinese). doi:10.3969/j.issn.1671-5942.2000.01.002
Zhou, S. Y., Wu, Y., and Jiang, Z. S. (2017). Earthquake geodesy and earthquake prediction: Progress, Innovations and problems over fifty years[J]. J. Geodesy Geodyn. 37 (6), 551–562. (in Chinese). doi:10.14075/j.jgg.2017.06.001
Zhu, Y. Q., Chen, B., and Zhang, X. (2001). Study of the gravity changes before and after the Jingtai MS5.9 earthquake[J]. Earthq. Res. China 17 (4), 356–363. (in Chinese). doi:10.3969/j.issn.1001-4683.2001.04.004
Zhu, Y. Q., Fu, G. Y., and Liang, W. F. (2015b). Spatial-temporal gravity changes before the ludian MS6.5, lushan MS7.0 and wenchuan MS8.0 earthquakes[J]. Seismol. Geol. 37 (1), 319–330. (in Chinese). doi:10.3969/j.issn.0253-4967.2015.25
Zhu, Y. Q., Hu, B., and Zhu, G. Z. (2005). Research on the variation of gravity field before Minle MS6.1 and Minxian MS5.2 earthquakes[J]. J. Geodesy Geodyn. 25 (1), 24–29. (in Chinese). doi:10.3969/j.issn.1671-5942.2005.01.005
Zhu, Y. Q., Li, H., and Zhu, G. Z. (2004). Gravity evolution and earthquake actives of the northeastern edge of Qinghai-Xizang block[J]. Acta Seismol. Sin. 26 (S1), 71–78. (in Chinese).
Zhu, Y. Q., Li, T., and Hao, M. (2016). Gravity changes before the menyuan, Qinghai MS6.4 earthquake of 2016[J]. Chin. J. Geophys. 59 (10), 3744–3752. (in Chinese).
Zhu, Y. Q., Liang, W. F., and Xu, Y. M. (2008a). Medium-term prediction of MS8.0 earthquake in Wenchuan, Sichuan by mobile gravity[J]. Recent Dev. World Seismol. 38 (7), 36–39. (in Chinese).
Zhu, Y. Q., Liang, W. F., and Zhan, F. B. (2012). Study on dynamic change of gravity field in China continent[J]. Chin. J. Geophys. 55 (3), 804–813. (in Chinese).
Zhu, Y. Q., Liang, W. F., and Zhang, S. (2018). Earthquake precursors: Spatial-temporal gravity changes before the great earthquakes in the sichuan-yunnan area. J. Seismol. 22 (1), 217–227. doi:10.1007/s10950-017-9700-2
Zhu, Y. Q., Liang, W. F., and Zhao, Y. F. (2017). Gravity changes before the Jiuzhaigou, sichuan, MS7.0 earthquake of 2017[J]. Chin. J. Geophys. 60, 4124–4131. (in Chinese). doi:10.ssss/j.issn.0001-5733.2017.10.036
Zhu, Y. Q., Liu, F., and Li, T. M. (2015a). Dynamic variation of the gravity field in the Sichuan-Yunnan region and its implication for seismic risk[J]. Chin. J. Geophys. 58 (11), 4187–4196. (in Chinese). doi:10.6038/cjg20151125
Zhu, Y. Q., Liu, F., and Zhang, G. Q. (2022). Mobile gravity monitoring and earthquake prediction in China[J]. Geomatics Inf. Sci. Wuhan Univ. 47 (6), 820–829. (in Chinese). doi:10.13203/j.whugis20220127
Zhu, Y. Q., Liu, F., Zhang, G. Q., and Xu, Y. M. (2019). Development and prospect of mobile gravity monitoring and earthquake forecasting in recent ten years in China. Geodesy Geodyn. 10 (6), 485–491. doi:10.1016/j.geog.2019.05.006
Zhu, Y. Q., Wang, Q. L., and Xu, Y. M. (2008b). Thoughts on the development of earthquake monitoring and prediction in mobile gravity[J]. Recent Dev. World Seismol. 38 (9), 19–25. (in Chinese). doi:10.3969/j.issn.0253-4975.2008.09.004
Zhu, Y. Q., Wen, X. Z., and Sun, H. P. (2013). Gravity changes before the lushan, sichuan, MS=7.0 earthquake of 2013[J]. Chin. J. Geophys. 56 (6), 1887–1894. (in Chinese). doi:10.6038/cjg20130611
Zhu, Y. Q., Xu, Y. M., and Liang, W. F. (2008c). Medium-term prediction of yutian, Xinjiang MS7.3 earthquake in 2008[J]. J. Geodesy Geodyn. 28 (5), 13–15. (in Chinese). doi:10.3969/j.issn.1671-5942.2008.05.003
Zhu, Y. Q., Xu, Y. M., and Lü, Y. P. (2009). Relations between gravity variation of Longmenshan fault zone and Wenchuan MS8.0 earthquake[J]. Chin. J. Geophys. 2 (10), 2538–2546. (in Chinese). doi:10.3969/j.issn.0001-5733.2009.10.012
Zhu, Y. Q., Zhan, F. B., Zhou, J. C., Liang, W., and Xu, Y. (2010). Gravity measurements and their variations before the 2008 wenchuan earthquake. Bull. Seismol. Soc. Am. 100, 2815–2824. doi:10.1785/0120100081
Zhu, Y. Q., Zhang, Y., Zhang, G. Q., Liu, F., and Zhao, Y. (2020). Gravity variations preceding the large earthquakes in the Qinghai-Tibet Plateau from 21st century. Chin. Sci. Bull. 65 (7), 622–632. (in Chinese). doi:10.1360/tb-2019-0153
Keywords: gravity observation, time-varying gravity, tectonic activity, earthquake precursor, earthquake prediction
Citation: Zhu Y, Yang X, Liu F, Zhao Y, Wei S and Zhang G (2023) Progress and prospect of the time-varying gravity in earthquake prediction in the Chinese Mainland. Front. Earth Sci. 11:1124573. doi: 10.3389/feart.2023.1124573
Received: 15 December 2022; Accepted: 09 January 2023;
Published: 24 January 2023.
Edited by:
Henglei Zhang, China University of Geosciences Wuhan, ChinaReviewed by:
Lianghui Guo, China University of Geosciences, ChinaYunlong Wu, China University of Geosciences Wuhan, China
Copyright © 2023 Zhu, Yang, Liu, Zhao, Wei and Zhang. This is an open-access article distributed under the terms of the Creative Commons Attribution License (CC BY). The use, distribution or reproduction in other forums is permitted, provided the original author(s) and the copyright owner(s) are credited and that the original publication in this journal is cited, in accordance with accepted academic practice. No use, distribution or reproduction is permitted which does not comply with these terms.
*Correspondence: Xiong Yang, MTA3Njc3MjkyMUBxcS5jb20=