- 1Marine Science Institute, University of the Philippines, Quezon City, Philippines
- 2Chemistry Department, University at Buffalo, The State University of New York, Buffalo, NY, United States
Pharmaceuticals in natural waters are considered emerging pollutants due to their low concentrations and the negative effects they pose to the environment. Common sources of such pollutants include untreated wastewater from hospitals, residential, industrial, and agricultural sources. Many wastewater treatment methods only remove a subset of all pharmaceuticals from the wastewater; remaining pharmaceuticals are discharged into natural waters, and ultimately drain into coastal areas. Regions without proper wastewater treatment are especially susceptible to such contamination. This study deals with the distribution, sources, and seasonal variability of pharmaceuticals in key aquatic systems in the Philippines. Two watershed continuums (Davao Gulf, Davao City; Macajalar Bay, Cagayan de Oro City); two tourist areas (Boracay Island, Aklan; Mabini, Batangas); and one pristine atoll (Tubbataha Reefs, Palawan)—all with varied prevailing human population pressures—were studied. Samples of hospital wastewater as well as groundwater, surface and bottom water samples from rivers and coastal seas collected during dry and wet seasons were analyzed using solid-phase extraction and liquid chromatography-tandem mass spectrometry. Thirty-four target pharmaceutical residues and antibiotics were extracted and quantified. Acetaminophen was detected at concentrations of up to 289.17 ppb in freshwater samples, and at concentrations of up to 253.39 ppb in seawater samples. Ubiquitous to all the sites was caffeine, reaching 1848.57 ppb. Sulfamethazine, a commonly used veterinary antibiotic, was detected at 764.91 ppb in a river site in Cagayan de Oro. Untreated hospital wastewater contained metformin, iopamidol, sulfamethoxazole, acetylsulfamethoxazole, ciprofloxacin, and azithromycin, but these pharmaceuticals were not detected in other river and coastal waters. Samples collected during the dry season exhibited higher concentrations than those from the wet season, which appears to be related to increase in transient populations from tourism activities as well as dilution. The presence of pharmaceutical residues and antibiotics in these areas and the potential impact on the environment indicate the need for stricter wastewater management measures, particularly in communities located near water bodies. As the results of this study show, such measures might be most beneficial and effective if imposed during dry season and in areas open to tourism.
1 Introduction
Pharmaceuticals and personal care products (PPCPs) are emerging pollutants of concern due to their negative effects on the environment, such as bioaccumulation in the food chain, which contributes to shifts in mesocosm communities (Deo, 2014); transcription impacts in microbes (Davies et al., 2006); negative species-specific growth effects (Hagenbuch and Pinckney, 2012); and proliferation of antibiotic-resistant genes (Kummerer, 2009). The United States Environmental Protection Agency (US EPA) and the European Union consider pharmaceuticals and other personal care products as contaminants of emerging concern (CECs): low-concentration pollutants detected in surface waters for which no standard concentration has been established. Though standards have not been established, concerns about their potential impact on aquatic ecosystems have been raised (US EPA, 2008; SCHEER, 2018). Other CECs include persistent organic pollutants, endocrine-disrupting chemicals, nanomaterials, and veterinary medicines (US EPA, 2008).
Technological advancements in the past decades have allowed detection of contaminants to the nanogram level: PPCPs were detected in wastewater, groundwater, submarine groundwater discharge (SGD), river waters, and coastal waters around the globe (e.g., Vulliet et al., 2008; Shimizu et al., 2013; Singh et al., 2019). Further, caffeine and pharmaceutical residues such as carbamazepine, sulfapyridine, sulfamethoxazole, ketoprofen, and diclofenac were detected in the groundwater and SGD of Baltic Sea (Szymczycha, et al., 2020).
General human and animal biotransformation could weaken the life cycle of pharmaceuticals. Microbes also degrade pharmaceuticals further in the wastewater treatment process, but continuous input of pharmaceuticals from multiple sources can potentially overwhelm their capability to degrade increasing pollutant concentrations in the environment (Manzetti and Ghisi, 2014). The impact of emerging pollutants, including endocrine disruption, immunotoxicity, neurological disorders, and cancers in various organisms (Sanchez and Egea, 2018), in aquatic systems is comparatively muted when compared to their impact on humans and animals in terrestrial environments (Kummerer, 2009). This is largely due to relatively low concentrations (≤ lower microgram/liter) because of dilution, aerobic/anaerobic biotransformation, and adsorption by sediment or biofilm (Kummerer, 2009). Nonetheless, the significance of emerging pollutants in aquatic systems should not be minimized, because of the reach and interconnectedness of water systems, and their direct contact with and impact on human and other animal populations. Moreover, their detrimental downstream effects, such as antibiotic resistance, traverse across geopolitical boundaries and thereby constitute a shared global concern.
Wastewater is the major source of pharmaceuticals and their metabolites in highly urbanized environments (Manzetti and Ghisi, 2014). Without proper wastewater treatment, PPCP contamination increases the health risk for humans and other animals through consumption of and contact with contaminated water. Poor treatment of wastewater leads to the release of PPCPs into receiving streams and ultimately into the coastal waters. Many of the clean-up procedures are not designed to specifically remove them from the wastewater completely. Even the most advanced sewage treatment processes are proven ineffective in completely removing pharmaceuticals from the wastewater (Wallace and Aga, 2016; Hurst et al., 2018).
In the Philippines, wastewater treatment is still developing. In urban regions, only 10% of septage is safely treated and disposed of, 4% of the total wastewater is treated, and about 3% of the population openly defecates in nearby water bodies due to the lack of proper facilities (The World Bank, 2012). Previous studies on PPCP contamination were mostly focused on Metro Manila and adjacent urbanized provinces (Shimizu et al., 2013; Singh et al., 2019; Angeles et al., 2021; Van et al., 2021). Studies for highly populated coastal areas are severely lacking despite the archipelagic nature of the Philippines. Coastal sites vary in land-use, geology, population density, coastal tourism, and other anthropogenic factors (Figure 1) each of which determines the nature and intensity of environmental pollution. Therefore, studying PPCP contamination at different sites, for example, coastal tourist areas, mixed-use watersheds, and a pristine atoll, can provide a broader view of the state of PPCP levels in the country’s coastal waters. In this study, samples from various aquatic matrices, such as hospital wastewater, surface and river, and coastal waters were collected for common PPCPs and antibiotics analyses. The main objectives were to (i) identify and quantify PPCP contaminants in key aquatic environments in the Philippines, (ii) examine their distribution in relation to population density and land-use, and (iii) determine how seasonal and physicochemical factors influence the variation in PPCP concentrations within the same sites.
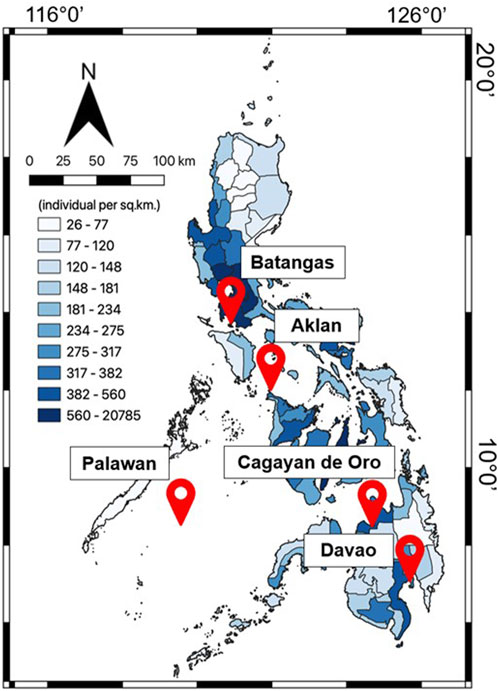
FIGURE 1. Population density map of the Philippines (2015) and localities where sampling campaigns was conducted in this study. Batangas and Aklan represent tourist sites; Cagayan de Oro and Davao Cities are examples of mixed-use populated, urban, and agricultural watersheds; Tubbataha Reefs Natural Park in Palawan serves as the least impacted site.
2 Materials and methods
2.1 Sites
Coastal tourist sites, mixed-use watershed, and pristine atolls were selected as key coastal environments in most Philippine areas and the specific sites are discussed below. Sampling points were chosen with the goal of capturing a holistic view of a sampling site from least to most anthropogenically impacted areas. For the least impacted sampling points, upstream sites, groundwater wells, and coastal areas with little to no infrastructure were chosen. For heavily impacted sampling points, downstream sites, drainage outfalls, and wetlands were chosen. The number of sampling points per site, the basis for selection, and the period of sampling are summarized in Table 1.
Coastal tourist areas represent sites with transient populations influencing the coastal use with economically relevant resources. Mabini, Batangas, also known as the Calumpang Peninsula, is the southwestern tip of Batangas province. The southern peninsula connects to the Verde Island Passage, which is dubbed the “Center of the Center of Marine Shorefish Biodiversity” (Carpenter and Springer, 2005). The 4,296-ha peninsula is subdivided into 34 barangays (local villages), of which Anilao Proper, with only 9.25 ha, is the smallest (Banluta et al., 2013), but heavily touristed because of its well-known dive sites and proximity to Metro Manila. Livestock and poultry farming is a major source of food and income for the people in Mabini, Batangas.
Boracay Island, Philippines, a world-renowned tourist destination for its white sand beaches, received at least 2 million tourists in 2019 in its mere 10 km2 land area (Zabal, 2020). In 2018, the island was closed from tourism for rehabilitation due carrying capacity overloading of existing infrastructure, which also hobbled infrastructure development. The island reopened to tourism activities in 2019. Aside from tourists, Boracay Island is also home to around 30,000 residents. Balabag STP (Water Environment Partnership in Asia, 2022) established a sewage treatment plant on the island in 2003. Additional capacity was installed as the Manocmanoc STP in 2018. The two water treatment facilities together can treat 8.7 million liters of wastewater per day servicing both tourist and residential areas of the island (Manila Water, 2018).
Mixed-use watersheds represent river basins with a major stream cascading from a predominantly agricultural upstream area down to a mostly urban downstream area before draining into the sea. The 1,373.83 km2 Cagayan River basin traverses three provinces which consistently and significantly contribute to the total agricultural and livestock products in the region and drains into Macajalar Bay (Department of Environment and Natural Resources (DENR), 2023). More than 70% of the area is utilized for agriculture including pasture for livestock production (Center for Environmental Studies and Management, 2014). Morphometrically, the high stream frequency of Cagayan River basin manifests as steeper slopes and lower rock permeability (Talampas and Cabahug, 2015) readily transport surface runoff down the basin during periods of rainfall. Meanwhile, a sewerage system is non-existent in Cagayan de Oro City as of 2014, and the natural river system serves as the drainage of untreated wastewater (Center for Environmental Studies and Management, 2014).
The 1,623 km2 Davao River basin traverses Bukidnon, Davao del Sur, Davao del Norte, and North Cotabato provinces, and drains eastward into the Davao Gulf (UP TCAGP, 2015). Coffee and cacao are among the priority crops in the area, with 4,977 ha and 2,627 ha of plantation in Davao City as of 2016, respectively (Office of the City Planning and Development Coordinator, 2021). Total poultry and livestock production in 2017 was 7,256,821 heads, which comprises mainly poultry and swine (Office of the City Planning and Development Coordinator, 2021). About 4.8% of the total land area of the Davao River basin is dedicated to annual and perennial crops primarily rice, corn, cassava, and sugarcane with high-value crops mostly fruits, including coffee and cacao (DENR, 2015). Water pollution in Davao City was attributed to domestic sewage due to the inadequate treatment of wastewater discharged from establishments and households (JICA, 2018).
Hospital wastewater samples were also collected in Cagayan de Oro as a possible point source in environmental sites. Treated and untreated wastewater samples were collected from a private and public hospital, respectively. The private hospital wastewater underwent settling and aeration treatment.
Pristine atoll represents least impacted coastal areas that have minimal contact with population. Tubbataha Reef Natural Park (TRNP) is a world-renowned marine protected area. It is in the middle of Sulu Sea, approximately 170 km southeast of Puerto Princesa City, Palawan. The closest human settlement, Cavili Island, Cagayancillo, is over 100 km north of the park (Murray et al., 2019). The number of visitors between 2016 and 2019 was around 2,800, primarily diver tourists visiting during the summer open season (April to early June; 2019 Tubbataha Management Office Annual Report). Tubbataha Reefs play a vital role in sustaining the marine resources and productivity of the greater Sulu Sea, providing it with fish and invertebrate larvae (TEEBcase, 2011). Given the area’s importance in the context of a healthy marine ecosystem and its minimal contact with the population, TRNP is an interest for the occurrence of pharmaceuticals as a “least impacted” site.
2.2 Sample collection and processing
Prior to sample collection, a Hannah multimeter was deployed to the specific depths in the rivers and coastal sites where the samples will be collected. For drainages and wastewater samples, multimeter reading was done in the bucket. Salinity, dissolved oxygen (DO) and pH were recorded. Surface and bottom water samples were collected when possible. Surface samples were collected 0.5 m depth, whereas bottom samples were collected at least 1 m above the riverbed and at 10–15 m depth for coastal sites.
Processing of samples follows the optimized method by Angeles and Aga (2018). Briefly, duplicates of 500 mL water samples and ultrapure water blanks were collected in acid-washed high-density polyethylene (HDPE) bottles. Samples were acidified with 40% phosphoric acid to a pH of 2–3 and stored in ice chests until arrival in the laboratory or basecamp. Then, samples were filtered through 0.7 μm glass microfiber filters to remove particulates. To prevent the chelation of tetracyclines with metal cations, a 2 mL sodium diethyldiamine (Na2EDTA) solution (5% w/v in water) was added before spiking the filtered sample with the surrogate standard mix.
For cleanup and concentration, the samples were passed through 500 mg OASIS HLB cartridges previously conditioned with 4 mL ultrapure water and 4 mL acetonitrile. The samples were loaded into the cartridges at the rate of 3–5 mL/min and dried in the manifold under vacuum. The cartridges were then wrapped in foil and stored in the freezer until it was ready to be transported for further processing.
In the laboratory, the cartridges were eluted with 8 mL of acetonitrile to obtain the extracts, then dried with a turbo evaporator under nitrogen gas at a temperature not exceeding 30°C. The dried extracts were then reconstituted with 900 μL of the mobile phase (A, 0.3% formic acid in water; B, 25% acetonitrile: 75% methanol) and was passed through a 0.45 μm syringe filter for further clean-up. The samples were spiked with 100 ppb of internal standard, d3-diphenhydramine, to account for possible measurement differences.
2.3 Standards and reagents
Five isotopically labelled standards were purchased from Toronto Research Chemicals: Caffeine (CAF), acetylsulfamethoxazole (ASMX), diclofenac (DIC), ciprofloxacin (CIP), and acetaminophen (ACT). LC-MS grade methanol and acetonitrile were obtained from EMD Millipore Corporation (Billerica, MA); and formic acid (88%) from Fisher Chemical (Pittsburgh, PA); and Oasis HLB solid-phase extraction (SPE) cartridges from Waters (Milford, MA). A milligram from each standard was weighed and dissolved in 1 mL of methanol, and the individual solutions were combined and diluted to 1 ppm as surrogate standard mix. Identification of other pharmaceuticals was based on their retention time. For their quantification, the peak area of the standard with the nearest retention time to the target pharmaceutical was used.
2.4 LCMS
A Waters Cortecs C18+ column (Milford, MA) with dimensions 2.1 × 150 mm and 2.7 μm particle size was used for the separation of 34 target analytes: anhydroerythromycin (AERY), azithromycin (AZI), clarithromycin (CLA), erythromycin (ERY), roxithromycin (ROX), spiramycin (SPI), tilmicosin ta (TIL), acetaminophen (ACT), caffeine (CAF), carbamazepine (CBZ), diclofenac (DIC), iopamidol (IOP), metformin (MET), trimethoprim (TMP), ciprofloxacin (CIP), enrofloxacin (ENRO), norfloxacin (NOR), oxolinic acid (OXO), sarafloxacin (SARA), acetylsulfamethoxazole (ASMX), sulfachloropyridazine (SCP), sulfadiazine (SDZ), sulfadimethoxine (S2M), sulfamerazine (SMRZ), sulfamethazine (SMZ), sulfamethizole (SMTH), sulfamethoxazole (SMX), sulfamethoxydiazine (SMD), sulfathiazole (STZ), anhydrotetracycline (ATC), chlortetracycline (CTC), demelocycline (DMC), oxytetracycline (OTC), and tetracycline (TC). Analysis was performed at the University at Buffalo Department of Chemistry using an Agilent 1200 LC system (Palo Alto, CA) and a Thermo Scientific TSQ Quantum Ultra triple quadrupole MS (Waltham, MA) equipped with a heated electrospray ionization (HESI) probe, operated under positive ionization mode.
The total run time for each sample was 45 min, with the mobile phase A and B elute through the column in a gradient starting from 90% A: 10% B (v/v) to 100% B, then switching back to 90% A: 10% B (section 2.2 Sample collection and processing). Instrumental limits of detection for select compounds are listed in Table 2.
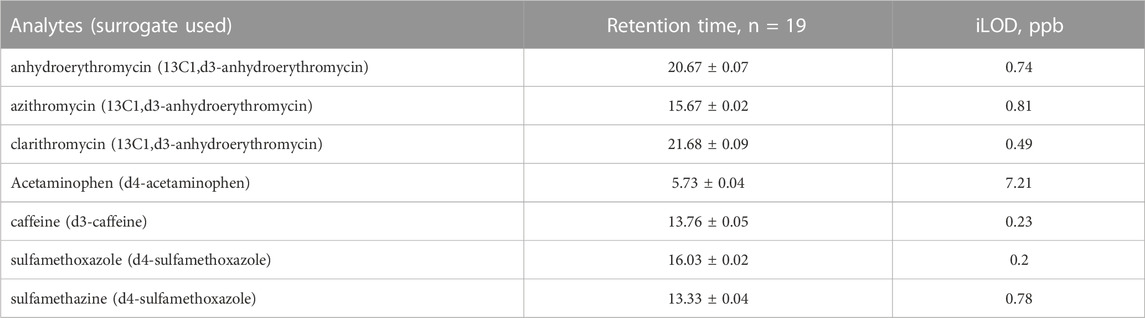
TABLE 2. Selected analytes, their retention times, and instrumental limit of detection (Singh et al., 2019).
2.5 Quantification
Due to the low recoveries of the surrogates, concentrations of analytes were obtained through a multipoint calibration curve. As such, values reported in this study are assumed to be underquantified. Unlike in an external one-point calibration with a deuterated standard, there was no correction done for the loss of analytes. A calibration curve was generated from nine concentrations (0.8 ppb, 1 ppb, 3 ppb, 6 ppb, 12 ppb, 25 ppb, 50 ppb, 100 ppb, 200 ppb) of target pharmaceuticals dissolved in freshwater and saltwater (Supplementary Figure S1). Calibration curves for the nine detected compounds have R2 values greater than 0.9959 to 0.9998. The equation of the slope was then used to calculate the concentration of the analyte. The detected analytes were confirmed by checking the signal-to-noise ratio of the peaks and ion ratio (Angeles and Aga, 2018).
For sampling sites with stratification, such as L2 and L3 in the Davao River basin, the freshwater curve was used for the site with freshwater and seawater layers, whereas the seawater curve was used for the site with brackish and seawater layers. For Tubbataha samples, the ultrapure water blank that yielded 0.9 ppb was subtracted from the reported values.
2.6 Statistical analysis
Physicochemical data measured with a Hanna multimeter was extracted using HI141 and HI148 Temperature Datalogger V1.3. Caffeine and acetaminophen concentrations were extracted from Thermo Scientific XCalibur software and further processed through Microsoft Excel. A principal component analysis (PCA) was performed with the PPCP concentration and physicochemical data (temperature, salinity, DO, and pH) using PAST4 with a correlation matrix.
3 Results
Three compounds (ACT, CAF, SMZ) were detected in natural water samples and eight (ACT, CAF, MET, IOP, SMX, ASMX, CIP, AZI) in hospital wastewater samples (Figure 2). Caffeine and acetaminophen were the most abundant compounds (Figure 3). Caffeine was detected in 73% of freshwater (drainages, wetlands, rivers) samples, and 85% of coastal water samples with the highest concentrations in samples collected during dry season, in April, from a drainage in Mabini, Batangas (1,848.57 ppb) and a wetland outlet in Boracay Island (722.315 ppb). The summary of detected PPCPs and their concentrations are listed in Supplementary Tables S1 and S2.
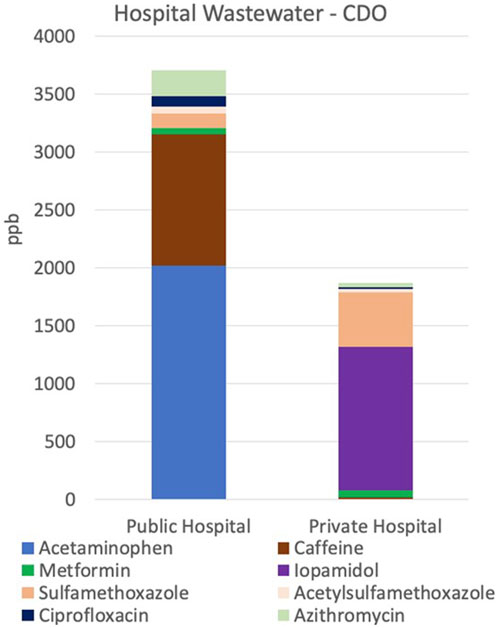
FIGURE 2. Total pharmaceutical and personal care products (PPCP) combination and concentrations in a private and public hospital wastewaters in Cagayan de Oro City. Untreated public hospital wastewater yielded higher total PPCP concentrations, with significantly high concentrations of caffeine and acetaminophen. Wastewater from private hospital go through settling and aeration which resulted to a distinct loss of caffeine and aceataminophen, and persistence of Iodine-contrast media, lopamidol, and Sulfamethoxazole.
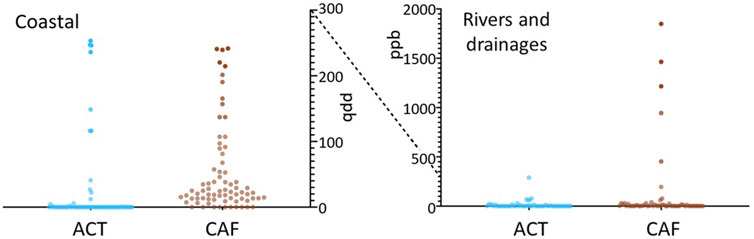
FIGURE 3. Summary of acetaminophen (ACT) and Caffeine (CAF) detections in surface, river, and coastal waters in the select areas in the Philippines.
Acetaminophen was detected in 30% of the freshwater (FW) samples, and only 22% in the coastal water (SW) samples. The highest concentrations were measured from samples in and around Boracay Island (FW=289.17 ppb, SW=253.39 ppb).
In coastal tourist areas, caffeine and acetaminophen concentrations range from levels below detection limits to 10.20 ppb with the Mabini upstream samples and Boracay groundwater samples in the lower concentration range, and inland and drainage water obtained from more populated areas have higher concentrations (Figure 4). Concentrations in samples from the dry season (April 2019) sampling in Mabini were higher compared to those from the wet season (September 2019) sampling (Figure 5).
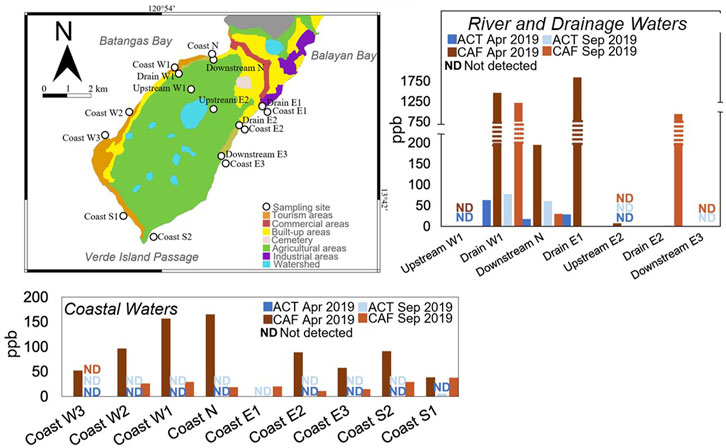
FIGURE 4. Land-use map of Mabini, Batangas, and concentrations of caffeine and acetaminophen in the surface and river waters covering wet (September) and dry (April) seasons. Map adapted from Hijmans and University of California Berkeley Museum of Vertebrate Zoology, 2015 and Banluta et al. (2013).
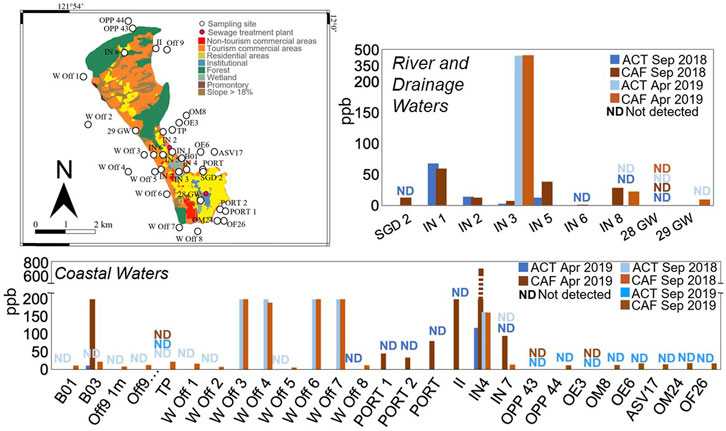
FIGURE 5. Land-use map of Boracay Island and concentrations of caffeine and acetaminophen covering wet (September) and dry (April) seasons during tourist closure (September 2018) and reopening (April 2019) periods. Map adapted from Hijmans and University of California Berkeley Museum of Vertebrate Zoology, 2015 and Department of Tourism (2008).
Additionally, concentrations in samples collected during the period when Boracay Island was open to tourism (April 2019) were higher than when it was closed (September 2018) to tourism. To compare, CAF in sites IN 4 and IN 7 during the September 2018 were 17.35 and 1.62 ppb respectively. The values were 77.11 and 10.31 ppb at the same sampling sites during April 2019. This highlights the link between the presence of transient population and discharge of untreated sewage into the waterways. PPCP detections in Boracay coastal samples collected from suspected sewage discharge zones were tens of magnitudes higher than the other coastal sites in the same study area (Figure 5). Caffeine concentrations in the sites nearest to the coast were also noticeably higher during the period open to tourism.
In Davao, a mixed-use watershed, acetaminophen and caffeine were detected in almost half of the river sites, whereas caffeine was ubiquitous on the coast in samples collected for both dry and wet season (Figure 6). Concentrations in samples from the upper part of the river basin (U1-U5) were generally lower (up to 11.73 ppb at most). On the other hand, downstream sites (L1-L3), where the majority of the city population and industries are located, contain up to 30.96 ppb caffeine in surface waters (site L2).
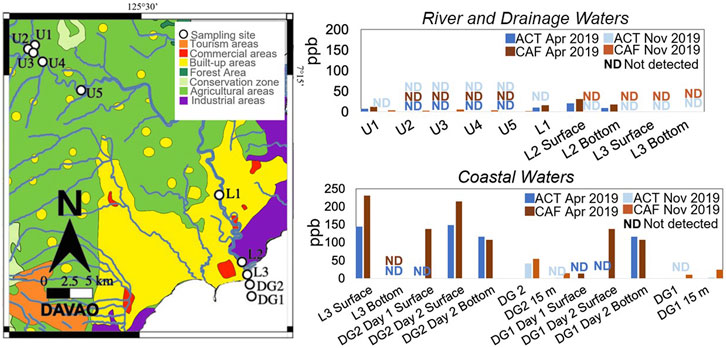
FIGURE 6. Land-use map of Davao River Basin and concentrations of caffeine and acetaminophen in river and coastal waters. Mindanao map adapted from Hijmans and University of California Berkeley Museum of Vertebrate Zoology, 2015 and Davao City Government (2013).
Bottom waters yielded lesser concentrations compared to their surface water counterparts, in both river (L2, L3) and coastal (DG 1 Day 2, DG 2 Day 2) sites (Figure 6). In general, concentrations from the dry season (April 2019) sampling campaign are higher compared to those of the wet season (September 2019).
In the Cagayan de Oro watershed, another example of mixed-use watershed, during the wet season, acetaminophen was not detected in the river sites and was only detected in half of the coastal sites (max = 26.88 ppb) (Figure 7). Caffeine was ubiquitous in the river water samples (max = 5.45 ppb) and surface coastal waters (max = 67.62 ppb) but not in the bottom coastal waters. Interestingly, sulfamethazine, an antibiotic commonly used in veterinary medicine, was detected at the two sampling points along Agusan River, one upstream (SMT = 57.38 ppb) and one at the river mouth (SMT = 764.91 ppb), an order of magnitude higher compared to Cagayan River mouth just a few kilometers westward.
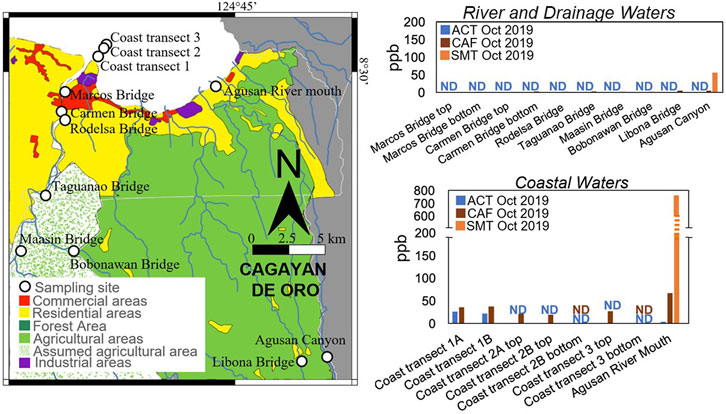
FIGURE 7. Land-use map of Cagayan River Basin, and concentrations of caffeine, acetaminophen, and sulfamethazine concentrations in river and coastal waters. Map adapted from Hijmans and University of California Berkeley Museum of Vertebrate Zoology, 2015 and Cagayan de Oro City Government (2013).
In comparison, in samples taken from the pristine atoll, Tubbataha Reef, caffeine was the only PPCP detected in 50% of the coastal water samples with a maximum concentration of 34.52 ppb at the sampling point where the research vessel was moored (Figure 8).
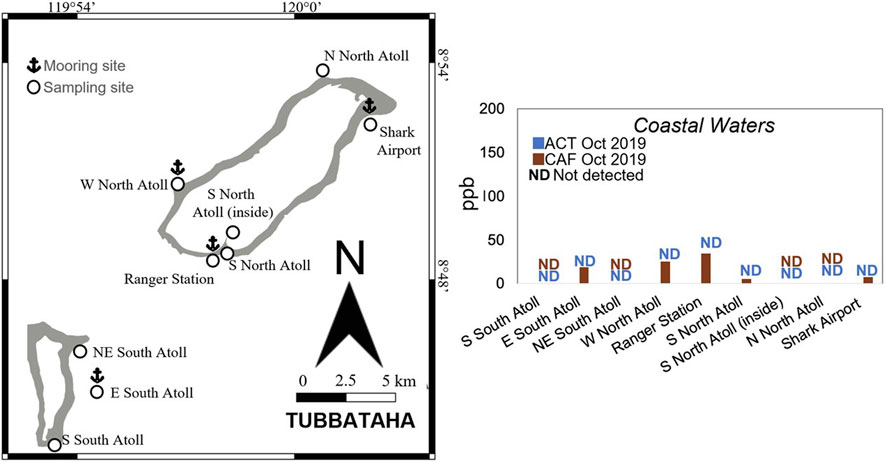
FIGURE 8. North and South Atolls of the Tubbataha Reefs with caffeine concentrations from sampling (circles) and mooring sites (anchor), where the vessel boarded by the research staff and ship crew was anchored for an extended period during the sampling campaign. Acetaminophen was not detected in any site.
In both hospital samples, significant concentrations of metformin (45.93–60.20 ppb), sulfamethoxazole (129.55–470.87 ppb), acetylsulfamethoxazole (30.18–56.94 ppb), ciprofloxacin (9.84–92.24 ppb), and azithromycin (38.84–223.89 ppb) were detected. Caffeine was not detected in the treated private hospital wastewater, and acetaminophen concentration only reached up to 19.95 ppb. This is in contrast with the untreated public hospital wastewater, wherein caffeine (1,132.64 ppb) and acetaminophen (2020.52 ppb) were detected, 50 to 100 times higher than in the private hospital. Iopamidol was also distinctly detected in the private hospital wastewater.
4 Discussion
4.1 Detection of untreated wastewater tracers
Environmental tracers are used to evaluate pollutant source and loading to aquatic systems (Richards et al., 2017). Different kinds of tracers are usually utilized in conjunction with one another to pinpoint sources and assess impacts. For instance, microbiological tracers like Escherichia coli when, detected with a chemical tracer, such as caffeine, points to a sole human sewage contamination (Staley et al., 2016).
Caffeine and acetaminophen were the most detected PPCPs in this study. Despite their high removal in wastewater treatment plants, their ubiquitous presence is usually exclusively related to humans and untreated wastewater (Mizukawa et al., 2019). The human body can metabolize 90%–99.95% of caffeine, hence, its presence in the environment is highly linked to the disposal of caffeine-containing beverages, such as coffee, soda, tea, and from the washing of the containers of these drinks (Seiler et al., 1999). Caffeine has a half-life of only 1.5 days in river water (Lam et al., 2004). Sustained detectable concentrations even in the coastal areas indicate a continuous input of untreated discharge from several non-point sources. Studies have shown that the predicted no-effect concentration (PNEC) for caffeine in rivers is 5.2 ppb (Komori et al., 2013). The PNEC marks the critical concentration at which an ecosystem can be adversely affected (Park and Kim, 2020). Another study determined 0.05 ppb to be the PNEC of caffeine on algae, invertebrates, and fishes (Szymczycha et al., 2020). The 10–30 ppb caffeine concentrations in this study exceed both previously published PNECs, thus raising concern about the long-term impact on the ecosystem if caffeine is continuously introduced unregulated into the aquatic environment.
Acetaminophen, also known as paracetamol, is an over-the-counter pain reliever commonly used due to its accessibility and affordability. Acetaminophen has a half-life of about 16–26 h in river water (Lam et al., 2004), but responds highly to treatment with a removal rate of 96% (Yamamoto et al., 2009). Another experiment on acetaminophen showed that even at concentrations as low as 95 ppb sex hormones in male fishes were affected increasing the fish’s vitellogenesis (Kim et al., 2012). Maximum levels of acetaminophen detected in this study (rivers = 61.25 ppb, coasts = 253.39 ppb) are already approximately 0.6–3 times the experimental concentration reported by Kim et al. (2012), raising concern about the potential risk to non-target species with increasing concentrations of these pharmaceuticals in aquatic systems.
Sulfamethazine, an antibiotic commonly used for poultry farming (Zhanteng et al., 2021), was also detected both in Agusan River and its immediate coastal discharge area in Macajalar Bay. Sulfamethazine concentrations upstream average 58.38 ppb compared to 764.91 ppb in the coastal waters. It is highly possible that accumulated discharge from poultry farms in the Agusan River watershed and Tagoloan River watershed were some of the sources of the detected sulfamethazine. The longshore currents from Tagoloan River indicates southwestward transport that may add into the PPCP concentrations close to Agusan River (Figure 9). It is also possible to have a point source for sulfamethazine given that the ratio of sulfamethazine to caffeine (SMZ/CAF = 10–11) is much higher than expected of a wastewater. Considering that caffeine is the usual tracer of untreated sewage and had the highest concentrations in many of the sampling sites, the expected ratio of ACT/CAF is <1. We take, for example, the untreated wastewater sample from a public hospital from Cagayan de Oro City as a point source, which has ACT/CAF 1.784 and detected antibiotic ratios with CAF ranging from 0.050 (ASMX/CAF) to 0.198 (AZI/CAF) (Supplementary Table S3). Compared to another point source (private hospital with treated wastewaters) where ACT was not detected and CAF decreased by two orders of magnitude, the detected antibiotic ratios to CAF ranged from 0.493 (CIP/CAF) to 23.607 (SMX/CAF). The increase in the ratios of the antibiotics attest to their chemical resilience to settling and aeration treatments. The SMZ/CAF ratio is almost conserved from 10.806 for Agusan Canyon in the upper watershed and 11.312 for Agusan River mouth in Macajalar Bay with CAF increasing from an averagee of 5.31 ppb–67.62 ppb and SMZ increasing from 57.382 ppb to 764.914 ppb respectively. Moreover, ACT/CAF for Agusan River mouth is only 0.065, the increase in ratios of SMZ/CAF does not indicate enrichment through wastewater treatment, but rather through additional input accumulated from its pathways from upper watershed or a point source much closer to the sampling site, such as farm feeds and commercial livestock. Sulfonamides are generally known to be stable and hard to degrade, hence their persistence in environmental matrices even after traditional treatments (Liu et al., 2021a). Though studies on the ecotoxicity of sulfamethazine are scarce, existing studies highlight the high sensitivity of primary producers, such as cyanobacteria, and other microbial communities to this antibiotic (Kergoat et al., 2021).
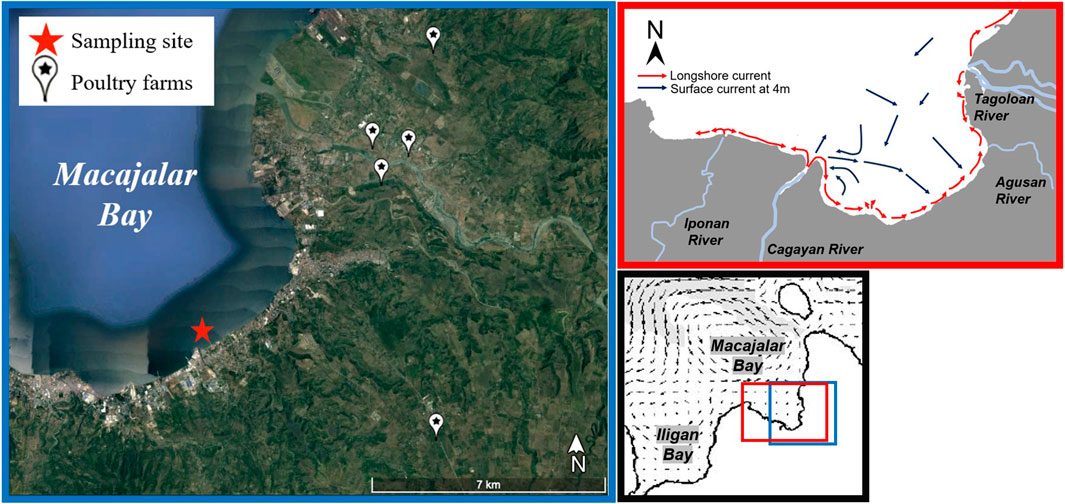
FIGURE 9. (left) Poultry farms within the vicinity of the eastern coast of Macajalar Bay. Agusan River Mouth sampling site is denoted by the red star. Satellite map retrieved from Google Earth (2016) (bottom right) Average annual surface circulation within the Macajalar Bay. (Modified from Bernardo and Villanoy, 2011). (top right) Simplified diagram demonstrating the direction of surface current at 4 m within Macajalar Bay (blue arrows) and the longshore transport along the coast (red arrows) (Modified from Villanoy et al., unpub. and Siringan, et al., 1998).
The private hospital in Cagayan de Oro City also had a relatively lower concentration of caffeine (19.95 ppb), confirming the treatment of wastewater that involved settling and aeration. Iopamidol at 1,237.47 ppb was distinctly detected in the private hospital as it has the facilities for the use of iodinated contrast media. Iopamidol is an iodine contrast media (ICM) for more advanced radiological imaging, such as CT scan, which might not have been available in the public hospital.
Residual concentrations of sulfamethoxazole in the treated wastewater of the private hospital are still relatively high in concentration (470.87 ppb). This antibiotic is also commonly reported to persist with conventional wastewater treatment (Liu et al., 2021b). Considering that the Philippines is second among Southeast Asian countries having high dissolved sulfamethoxazole in natural waters (Shimizu et al., 2013), addressing release from this point source can be beneficial.
Other pharmaceuticals and antibiotics such as azithromycin, ciprofloxacin, and metformin were also detected in both hospital wastewater samples. Though these pharmaceuticals and antibiotics were not detected in the other sampling sites, other PPCP studies of Philippine waters report the presence of these compounds (Shimizu et al., 2013; Van et al., 2021). Hospital wastewaters are known essential sources of antibiotic resistant bacteria (ARBs) and genes (ARGs) (Uluseker et al., 2021). ARGs continue to spread and propagate within the microbial community through horizontal gene transfer even after treatment and discharge (Kümmerer et al., 2018). With the threat of antimicrobial resistance being a major concern for public health, we raise a concern on the eventual disposal the need for advanced wastewater treatment of hospital wastewaters.
4.2 Detections of PPCPs in response to population
In this study, principal components analysis (PCA) was used to characterize the clustering of sampling points based on the detected CAF and ACT, and physicochemical parameters pH, temperature, and DO (Supplementary Table S4). The first two principal components (PCs) explained 32.2% and 26.4% of the total variance for the seawater samples, and 40.2% and 38.1% of the total variance for the freshwater samples (Figure 10). Most of the less populated sites, as well as some densely populated sites sampled during the wet season clustered together at the lower end of the ACT and CAF axes. Tourist coastal areas clustered with watershed coastal areas with high CAF concentrations. Some densely populated sites, which are mostly slow-flowing or stagnant drainages, are isolated, indicating an enclosed system. Generally, surface and river sites of the same characteristics cluster together. However, dilution or enrichment caused by rainfall or enhanced contaminant transport also affect variations in concentration. For example, densely populated sites sampled during wet season cluster with less populated sites. Therefore, PPCP distribution in the sampling sites can be mostly attributed to population and land-use, while variation is also affected by seasonal factors.
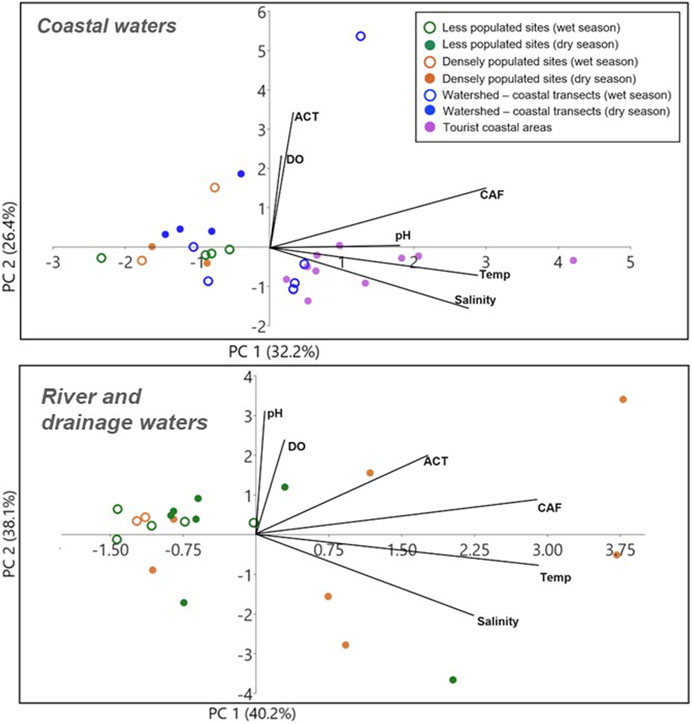
FIGURE 10. PCA plots of river and coastal waters. Data point color are based on the general characteristic of the surrounding sampling area. Variables are the detected caffeine (CAF) and acetaminophen (ACT), and physicochemical parameters pH, temperature (Temp), salinity, and dissolved oxygen (DO). Sites of the same characteristics generally cluster together.
Upstream river sites in Mabini, Cagayan de Oro, and Davao watersheds are mostly freshwater (0.00–0.25 PSU), slightly basic (pH 8.33–8.98) and oxic (5.20–8.47 ppm DO), except one shallow stream Upstream E2 in Mabini (1.41 PSU, pH 6.61, 2.26 ppm DO). Sites with sparse residential structures have notably lower CAF and ACT values than those collected from more populous areas. Though caffeine-bearing plants such as coffee, cacao, and tea may potentially be a source of caffeine contamination in environmental matrices, Cagayan de Oro and Davao watersheds, which harbor cacao plants, do not show preferential increase in the upper watershed where there are plantations, but do so towards the lower watershed, where population concentration is greater.
The impact of population, specifically tourists, on PPCP contamination is also evident from the difference in concentrations obtained from Boracay Island between September 2018 and April 2019. Boracay Island inland sites are freshwater (0.11–0.47 PSU), neutral to basic (pH 7.09–9.63), and mostly oxic (4.26–11.49 ppm DO) with the exception of one suboxic drainage site B01 (1.05 ppm DO). Wetland sites are brackish. Generally, caffeine concentrations from the coastal waters are low. Caffeine and acetaminophen concentrations from the offshore sampling sites suspected to be in the vicinity of wastewater discharge pipes, however, were tens of magnitudes higher in concentration, confirming that the discharge coming from the pipes were most likely untreated sewage during this sampling period.
TRNP coastal waters are oxic (5.29–6.17 ppm DO) and mostly slightly basic (pH 8.18–8.49) except one acidic site E South Atoll (pH 4.73). TRNP is about 100 km away from the nearest human settlement, with only a few structures in exposed sections of the atoll during a tidal cycle. Less than a dozen onsite personnel populate the atoll on a rotational basis. Consequently, PPCPs related to untreated sewage were not detected, except for a site with up to 34.52 ppb caffeine. Notably, the detections were mainly in the mooring sites where the research vessel was anchored. Onboard disposal of caffeine-containing foods and beverages and washing of their containers might have been the main source of caffeine during the coastal waters sampling campaign.
For both coastal tourist sites, there are few to no major streams; runoff from land mostly passes through drainages. With the lack of waterways connecting inland waters to the coastal waters, PPCP concentrations from inland point sources, such as drains or wetlands, tend to accumulate and have higher PPCP concentrations compared to the coastal sites. There are exceptions, however, where pipes from establishments extend and drain offshore. Though there is a possibility that the pipe water could have the same or greater concentrations compared with the inland point sources, dilution from entering a bigger body of water could lead to lower detected levels. Another consideration would be the possible presence of submarine groundwater discharge. Carbonate bedrock with porous sediments, and structures like joints, fractures, and faults can act as conduits of groundwater. If the groundwater is contaminated effluents from sewer and latrine systems, then the contaminants can end up in the SGD, thereby contributing to their detection in the coastal site sampling. SGDs were previously reported to contribute significant concentrations of pollutants to the coast (Taniguchi et al., 2019; Knapp et al., 2020).
4.3 Seasonal and physicochemical controls in contaminant distribution
Generally, wet season sampling yielded lower concentrations of PPCPs compared to the dry season sampling, and this can be attributed to dilution from the increase in rainfall and the resulting high runoff (Kozak et al., 2019). However, intense rainfall (>50 mm/h) can paradoxically contribute to increased concentrations of pollutants through flooding, as this phenomenon can overload drains and sewer systems, which will result in pollutants directly entering waterways (US EPA, 2008). Rainfall during the sampling periods, however, was not of heavy intensity. Maximum rainfall measurements for the Cagayan and Davao River basin sampling campaigns were only up to 1.2 mm/h and 0.2 mm/h, respectively (WorldWeatherOnline, 2019). The high stream frequency of Cagayan and Davao River Basins (Pubellier et al., 1994; Talampas and Cabahug, 2015) contribute to the transport of pollutants downstream even with low-intensity rainfall events, but the low concentrations suggest that the additional rainfall and runoff likely diluted the concentrations. Higher PPCP concentrations during the dry season also suggests that future wastewater treatment in these cities should consider implementing more stringent dry season clean-up protocols.
Detected concentrations in the transect of coastal sites in Davao Gulf between the 2-day sampling had a notable difference. It is important to note that between the sampling days, there was significant rainfall during the night. The intrusion of additional freshwater through rainfall and run-off draining through the river likely diluted the concentrations detected during the second day of sampling along the same transect.
Concentrations of contaminants in the aquatic environment are usually governed by dilution, such that upon the absence of an additional point source, they are expected to decrease when entering a larger volume of water. Though underestimated and not comparable to the concentrations from the discharging river, concentrations of samples taken closer to the coast were higher compared to samples farther from the coast for both Davao and Cagayan de Oro. Under the assumption that concentrations from the river become diluted after entering coastal waters, this finding suggests that additional sources of contaminants are directly discharged near the sampling site, or the water circulation within the watershed entrains contaminants along the length of the coast.
In the Davao Gulf circulation model by Villanoy et al. (2009) (Figure 11), the strong outflow of river water to the gulf pushes the water parcel south southwestwards into the gulf. Combined with the high stream frequency of the basin, run-off from terrestrial sources draining through the river and ultimately to the coast could have contributed to the higher concentrations detected during the second day of sampling along the same transect. Another possible source of contamination are leachates from the landfill site within the watershed that could be transported downstream through runoff.
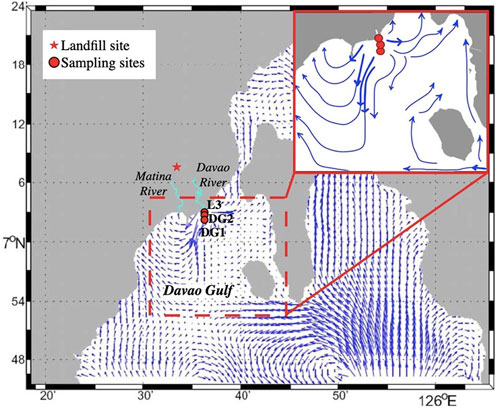
FIGURE 11. Average surface circulation within the Davao Gulf during April. The landfill site upstream might be a possible source of contaminants, wherein leachates may drain into the gulf as runoff through major streams. RM (River Mouth), DG1 (Davao Gulf 1), and DG2 are sampling sites in this study with detections of caffeine and acetaminophen. A gyre-like flow circulates west of the outflow from Davao River, running along the coast, and recirculating within that zone. Simplified Davao River outflow circulation (inset map), showing the westward and eastward flow of water (Modified from Hijmans and University of California Berkeley Museum of Vertebrate Zoology, 2015 and Villanoy et al., 2009). Thicker lines correspond to faster average current.
Notable differences in concentrations between surface and bottom waters were also observed in Davao (L2, L3, DG 1 Day 2, DG 2 Day 2). Surface waters were generally higher in PPCP concentrations compared to the bottom counterpart. Notably, salinity is less on the surface compared to the bottom water parcels, which indicates stratification of the river water due to saltwater intrusion (Table 3). The halocline is known to control vertical mixing within a parcel of water and at times can even restrict vertical transfer of gases and nutrients (Andreev et al., 2002); hence, it could be inferred that the transfer of dissolved pollutants like PPCPs to more saline bottom waters was inhibited by the contiguity of the freshwater stratum. It is also possible that the pH and composition (organic and ionic matter, silt, clay) of the bottom sediment were ideal for the adsorption of PPCP contaminants, as these were reported to influence PPCP uptake of sediments (Maghsodian et al., 2022), thus depleting concentrations within the bottom water. Comparison of pharmaceutical contaminants between sediment and water along the same sites previously showed higher concentrations in sediments (Fairbairn et al., 2015).
5 Conclusion
Detection of common pharmaceutical residues and poultry antibiotics in Philippine waters further highlights widespread occurrence of untreated wastewater discharge. Potential sampling points from critical sites not covered in this paper, such as sites of pollutant transport, can be inferred from the circulation model. One such area is Samal Island in Davao. Known for its high-value aquaculture products, the site must be evaluated for the long-term effects of PPCP exposure on marine life. More intensive wastewater treatment should be considered during the dry season given the generally higher concentration of contaminants detected during this period. Similarly, tourist sites must increase their capacity and capability in wastewater treatment to accommodate peak season population spikes. When necessary, release of untreated wastewater can be timed during quick flushing of the ebb tide, usually midway through an ebb tide for quick dispersal and dilution.
PPCP sources are both from point and non-point sources. Targeted wastewater treatment at point sources might be pursued through public/private partnerships depending on the type of PPCP to clean. For hospital wastewaters, a secondary treatment more targeted to pharmaceutical residues would be beneficial. In the long run, targeted treatment at wastewater locations specific to the type of wastewater influent (agricultural, industrial, domestic, or medical), before the confluence and mixing of these sources, may offer a better opportunity for the elimination of persistent and emerging pollutants than implementation of a large scale, “catch-all” treatment before discharging to the coastal areas. Cities that currently lack substantial wastewater treatment facilities can still configure the best treatment plan for the type of land-use and specific water quality concerns in the city, and address point and non-point source pollutants.
With the threat of stronger typhoons and more intense rainfall due to climate change, institutions must also adapt to the increased influent volumes possibly carrying these contaminants. Overall, further baselining and ecotoxicity studies must be conducted to establish stringent guidelines that could curb the scenario of increasing PPCP contaminants in natural waters.
Data availability statement
The original contributions presented in the study are included in the article/Supplementary Material, further inquiries can be directed to the corresponding author.
Author contributions
SM wrote the first draft of the manuscript and performed most of the sample processing and analysis. CJ contributed to the conception and design of the study, sample collection, processing, revision of the manuscript, and procurement of funding for the projects. DA and LA optimized the methods used for sample processing and analysis. CV contributed to the sampling design of offshore sites and to the interpretation of data. All authors contributed to the article and approved the submitted version.
Funding
Most of the sample analyses and fieldwork to Davao and Cagayan de Oro cities were supported by the USAID Partners for Enhanced Engagement in Research (subaward number 2000009924) “Baselining Persistent and Emerging Organic Pollutant Levels in Environmental and Engineered Systems for Healthy Philippines”. Sample collections for Boracay and Tubbataha were supported by Commission on Higher Education-Philippine-California Advanced Research Institutes research grant IIID (2017-030) “Deploying Unmanned Research Vessels to advance Marine and Environmental Health Monitoring and Data Collection in the Philippines”. Fieldwork and sample collection in Mabini, Batangas were supported by the Marine Science Institute In-house Research Grant for 2019. Additional support from National Science Foundation PIRE-HEARD Grant 1545756 for training and sample analyses. AD also acknowledges funding from the Fulbright U.S. Scholar Program through the Fulbright Global Scholar Award.
Acknowledgments
We thank the crew and researchers aboard M/Y Panata expedition PA0421 to Tubbataha in October 2019 and cruise to Boracay December 2019. Our gratitude to Mary Antoinette Limen, Mishel Valery Rañada, Gio Ferson Bautista, and Ernest Guiller Pineda for helping us in the field, and to Lahiruni Halwatura for assisting in the creation of a standard calibration curve for saltwater.
Conflict of interest
The authors declare that the research was conducted in the absence of any commercial or financial relationships that could be construed as a potential conflict of interest.
Publisher’s note
All claims expressed in this article are solely those of the authors and do not necessarily represent those of their affiliated organizations, or those of the publisher, the editors and the reviewers. Any product that may be evaluated in this article, or claim that may be made by its manufacturer, is not guaranteed or endorsed by the publisher.
Supplementary material
The Supplementary Material for this article can be found online at: https://www.frontiersin.org/articles/10.3389/feart.2023.1124313/full#supplementary-material
References
Andreev, A., Kusakabe, M., Honda, M., Murata, A., and Saito, C. (2002). Vertical fluxes of nutrients and carbon through the halocline in the western subarctic Gyre calculated by mass balance. Deep Sea Res. Part II Top. Stud. Oceanogr. 49 (24), 5577–5593. doi:10.1016/S0967-0645(02)00200-X
Angeles, L. F., and Aga, D. S. (2018). Establishing analytical performance criteria for the global reconnaissance of antibiotics and other pharmaceutical residues in the aquatic environment using liquid chromatography-tandem mass spectrometry. J. Anal. Methods Chem. 2018, 1–9. doi:10.1155/2018/7019204
Angeles, L. F., Halwatura, L. M., Antle, J. P., Simpson, S., Jaraula, C. M. B., and Aga, D. S. (2021). In silico supported nontarget analysis of contaminants of emerging concern: increasing confidence in unknown identification in wastewater and surface waters. ACS Est. Water 1 (8), 1765–1775. doi:10.1021/acsestwater.1c00105
Banluta, F., Brion, J., Francisco, L., Magayanes, L., Olaguera, F., Palma, J., et al. (2013). Municipality of Mabini: comprehensive land use plan. https://www.scribd.com/document/289758328/Mabini-SEP-2013-CLUP-2014-2023-Lowres.
Bernardo, L. P., and Villanoy, C. (2011). “Development of a particle dispersal model for bohol sea (Philippines),”. Masters Thesis (Quezon City, Philippines: University of the Philippines Diliman).
Cagayan de Oro City Government (2013). Cagayan de Oro comprehensive land use plan (2013-2022). https://cagayandeoro.gov.ph/phocadownloadpap/CdeO%20CLUP%20Vol%201%20-%20CLUP.pdf.
Carpenter, K. E., and Springer, V. G. (2005). The center of the center of marine shore fish biodiversity: the philippine islands. Environ. Biol. Fishes 72 (4), 467–480. doi:10.1007/s10641-004-3154-4
Center for Environmental Studies and Management (2014). Formulation of an integrated River Basin management and development master plan for cagayan de Oro River Basin. https://faspselib.denr.gov.ph/sites/default/files//DOCUMENTS/cagayan%20de%20oro%20DRAFT%20MASTER%20PLAN.pdf.
Davao City Government (2013). Comprehensive zoning ordinance of Davao city (2013-2022). https://cpdo.davaocity.gov.ph/wp-content/uploads/2016/10/Vol2ZoningOrdinance2013-202220151215090202.pdf.
Davies, J., Spiegelman, G. B., and Yim, G. (2006). The world of subinhibitory antibiotic concentrations. Curr. Opin. Microbiol. 9 (5), 445–453. doi:10.1016/j.mib.2006.08.006
Deo, R. P. (2014). Pharmaceuticals in the surface water of the USA: A review. Curr. Envir Health Rpt 1, 113–122. doi:10.1007/s40572-014-0015-y
Department of Environment and Natural Resources (DENR) (2023). Cagayan de Oro River Basin. Retrieved from https://riverbasin.denr.gov.ph/river/cdo.
Department of Environment and Natural Resources (DENR) (2015). Davao River basin management and development plan. Retrieved from https://riverbasin.denr.gov.ph/masterplans/executivesummary.pdf.
Fairbairn, D. J., Karpuzcu, M. E., Arnold, W. A., Barber, B. L., Kaufenberg, E. F., Koskinen, W. C., et al. (2015). Sediment-water distribution of contaminants of emerging concern in a mixed use watershed. Sci. Total Environ. 505, 896–904. doi:10.1016/j.scitotenv.2014.10.046
Hagenbuch, I. M., and Pinckney, J. L. (2012). Toxic effect of the combined antibiotics ciprofloxacin, lincomycin, and tylosin on two species of marine diatoms. Water Res. 46 (16), 5028–5036. doi:10.1016/j.watres.2012.06.040
Hijmans, R.University of California, Berkeley, Museum of Vertebrate Zoology (2015). Boundary Philippines 2015 UC Berkeley Museum of vertebrate Zoology. Available at: http://purl.stanford.edu/zm113qp3497.
Hurst, J. J., Wallace, J. S., and Aga, D. S. (2018). Method development for the analysis of ionophore antimicrobials in dairy manure to assess removal within a membrane-based treatment system. Chemosphere 197, 271–279. doi:10.1016/j.chemosphere.2018.01.028
Japan International Cooperation Agency (2018). Davao City infrastructure development plan and capacity building project. Retrieved from https://openjicareport.jica.go.jp/pdf/12308706.pdf.
Kergoat, L., Besse-Hoggan, P., Leremboure, M., Beguet, J., Devers, M., Martin-Laurent, F., et al. (2021). Environmental concentrations of sulfonamides can alter bacterial structure and induce diatom deformities in freshwater biofilm communities. Front. Microbiol. 12, 643719. doi:10.3389/fmicb.2021.643719
Kim, P., Park, Y., Ji, K., Seo, J., Lee, S., Choi, K., et al. (2012). Effect of chronic exposure to acetaminophen and lincomycin on Japanese medaka (Oryzias latipes) and freshwater cladocerans Daphnia magna and Moina macrocopa, and potential mechanisms of endocrine disruption. Chemosphere 89 (1), 10–18. doi:10.1016/j.chemosphere.2012.04.006
Knapp, M. A., Geeraert, N., Kim, K., and Knee, K. L. (2020). Submarine groundwater discharge (SGD) to coastal waters of saipan (commonwealth of the Northern Mariana Islands, USA): implications for nitrogen sources, transport and ecological effects. Water 12, 3029. doi:10.3390/w12113029
Komori, K., Suzuki, Y., Minamiyama, M., and Harada, A. (2013). Occurrence of selected pharmaceuticals in river water in Japan and assessment of their environmental risk. Environ. Monit. Assess. 185 (6), 4529–4536. doi:10.1007/s10661-012-2886-4
Kozak, C., Fernandes, C. V. S., Braga, S. M., do Prado, L. L., Froehner, S., and Hilgert, S. (2019). Water quality dynamic during rainfall episodes: integrated approach to assess diffuse pollution using automatic sampling. Environ. Monit. Assess. 191 (6), 402. doi:10.1007/s10661-019-7537-6
Kümmerer, K., Dionysiou, D. D., Olsson, O., and Fatta-Kassinos, D. (2018). A path to clean water. Science 361, 222–224. doi:10.1126/science.aau2405
Kummerer, K. (2009). Antibiotics in the aquatic environment a review Part I. Chemosphere 75 (4), 417–434. doi:10.1016/j.chemosphere.2008.11.086
Lam, M. W., Young, C. J., Brain, R. A., Johnson, D. J., Hanson, M. L., Wilson, C. J., et al. (2004). Aquatic persistence of eight pharmaceuticals in a microcosm study. Environ. Toxicol. Chem. 23, 1431–1440. doi:10.1897/03-421
Liu, X., Su, X., Tian, S., Li, Y., and Yuan, R. (2021a). Mechanisms for simultaneous ozonation of sulfamethoxazole and natural organic matters in secondary effluent from sewage treatment plant. Fontiers Environ. Sci. Eng. 15 (4), 75. doi:10.1007/s11783-020-1368-0
Liu, Z., Hu, W., Zhang, H., Wang, H., and Sun, P. (2021b). Enhanced degradation of sulfonamide antibiotics by UV irradiation combined with persulfate. Processes 9 (2), 226. doi:10.3390/pr9020226
Maghsodian, Z., Sanati, A. M., Mashifana, T., Sillanpää, M., Feng, S., Nhat, T., et al. (2022). Occurrence and distribution of antibiotics in the water, sediment, and biota of freshwater and marine environments: A review. Antibiotics 11 (11), 1461. doi:10.3390/antibiotics11111461
Manila Water (2018). Boracay Water completes sewage treatment plant diversion, connects more establishments to sewer network. Retrieved from https://www.manilawater.com/corporate/boracay-water/agos/2018-05-10/boracay-water-completes-sewage-treatment-plant-diversion--connects-more-establishments-to-sewer-network.
Manzetti, S., and Ghisi, R. (2014). The environmental release and fate of antibiotics. Mar. Pollut. Bull. 79, 7–15. doi:10.1016/j.marpolbul.2014.01.005
Mizukawa, A., Filippe, T. C., Peixoto, L. O. M., Scipioni, B., Leonardi, I. R., and Azevedo, J. C. R. D. (2019). Caffeine as a chemical tracer for contamination of urban rivers. RBRH 24, e29. doi:10.1590/2318-0331.241920180184
Murray, R., Conales, S., Araujo, G., Labaja, J., Snow, S. J., Pierce, S. J., et al. (2019). Tubbataha Reefs Natural Park: the first comprehensive elasmobranch assessment reveals global hotspot for reef sharks. J. Asia-Pacific Biodivers. 12 (1), 49–56. doi:10.1016/j.japb.2018.09.009
Office of the City Planning and Development Coordinator (2021). Socio-economic indicators 2016-2020. Retrieved from https://cpdo.davaocity.gov.ph/wp-content/uploads/2022/03/2021-SEI.pdf.
Park, J., and Kim, S. D. (2020). Derivation of predicted No effect concentrations (PNECs) for heavy metals in freshwater organisms in korea using species sensitivity distributions (SSDs). Minerals 10 (8), 697. doi:10.3390/min10080697
Pubellier, M., Deffontaines, B., Quebral, R., and Rangin, C. (1994). Drainage network analysis and tectonics of Mindanao, southern Philippines. Geomorphology 9 (4), 325–342. doi:10.1016/0169-555X(94)90053-1
Richards, S., Withers, P. J. A., Paterson, E., McRoberts, C. W., and Stutter, M. (2017). Potential tracers for tracking septic tank effluent discharges in watercourses. Environ. Pollut. 228, 245–255. doi:10.1016/j.envpol.2017.05.044
Sanchez, W., and Egea, E. (2018). Health and environmental risks associated with emerging pollutants and novel green processes. Environ. Sci. Pollut. Res. 25 (7), 6085–6086. doi:10.1007/s11356-018-1372-0
SCHEER (2018). Statement on emerging health and environmental issues. Brussels, Belgium: European Commission.
Seiler, R. L., Steven, Z. D., Thomas, J. M., and Howcroft, D. L. (1999). Caffeine and pharmaceuticals as indicators of waste water contamination in wells. Ground Water 37 (3), 405–410. doi:10.1111/j.1745-6584.1999.tb01118.x
Shimizu, A., Takada, H., Koike, T., Takeshita, A., Saha, M., Rinawati, N., et al. (2013). Ubiquitous occurrence of sulfonamides in tropical Asian waters. Sci. Total Environ. 452–453, 108–115. doi:10.1016/j.scitotenv.2013.02.027
Singh, R. R., Angeles, L. F., Butryn, D. M., Metch, J. W., Garner, E., Vikesland, P. J., et al. (2019). Towards a harmonized method for the global reconnaissance of multi-class antimicrobials and other pharmaceuticals in wastewater and receiving surface waters. Environ. Int. 124, 361–369. doi:10.1016/j.envint.2019.01.025
Siringan, F. P., Berdin, R. D., Doruelo, J. S., Jaraula, C. M. B., Mateo, Z. R. P., Ong, J. B., et al. (1998). Sedimentation patterns and sediment dispersal along the coast of macajalar Bay: Establishing environmental baselines and predictive tools for coastal resource management using the sediment record. Diliman, Quezon City: National Institute of Geological Sciences (NIGS)-University of the Philippines.
Staley, Z. R., Grabuski, J., Sverko, E., and Edge, T. A. (2016). Comparison of microbial and chemical source tracking markers to identify fecal contamination sources in the humber river (Toronto, ontario, Canada) and associated storm water outfalls. Appl. Environ. Microbiol. 82 (21), 6357–6366. doi:10.1128/AEM.01675-16
Szymczycha, B., Borecka, M., Białk-Bielińska, A., Siedlewicz, G., and Ksenia, P. (2020). Submarine groundwater discharge as a source of pharmaceutical and caffeine residues in coastal ecosystem: bay of puck, southern baltic sea case study. Sci. Total Environ. 713, 136522. doi:10.1016/j.scitotenv.2020.136522
Talampas, W. D., and Cabahug, R. R. (2015). Catchment characterization to understand flooding in cagayan de Oro River Basin in northern mindanao, Philippines. Mindanao J. Sci. Technol. 13.
Taniguchi, M., Dulai, H., Burnett, K. M., Santos, I. R., Sugimoto, R., Stieglitz, T., et al. (2019). Submarine groundwater discharge: updates on its measurement techniques, geophysical drivers, magnitudes, and effects. Front. Environ. Sci. 7. doi:10.3389/fenvs.2019.00141
TEEBcase (2011). Benefit sharing as key to conserving the Tubbataha Reefs, Philippines. Available at: https://TEEBweb.org.
The World Bank (2012). Implementation completion and results report IBRD 81620-PH on a loan in the amount of US$ 275 million to the land bank of the Philippines with the guarantee of the republic of the Philippines for the Metro Manila wastewater management project [data file]. Retrieved from https://documents1.worldbank.org/curated/en/265781610382943823/pdf/Philippines-Metro-Manila-Wastewater-Management-Project.pdf.
Uluseker, C., Kaster, K. M., Thorsen, K., Basiry, D., Shobana, S., Jain, M., et al. (2021). A review on occurrence and spread of antibiotic resistance in wastewaters and in wastewater treatment plants: mechanisms and perspectives. Front. Microbiol. 12, 717809. doi:10.3389/fmicb.2021.717809
UP TCAGP (2015). Flood forecasting and flood hazard mapping for Davao River Basin, disaster risk and exposure assessment for mitigation (DREAM) Program DOST-grants-in-aid Program. Quezon City, Philippines: University of the Philippines Diliman.
US Environmental Protection Agency (2008). Aquatic life criteria for contaminants of emerging concern. https://www.epa.gov/sites/default/files/2015-08/documents/white_paper_aquatic_life_criteria_for_contaminants_of_emerging_concern_part_i_general_challenges_and_recommendations_1.pdf.
Van, D. A., Ngo, T. H., Huynh, T. H., Nakada, N., Ballesteros, F., and Tanaka, H. (2021). Distribution of pharmaceutical and personal care products (PPCPs) in aquatic environment in Hanoi and Metro Manila. Environ. Monit. Assess. 193 (12), 847. doi:10.1007/s10661-021-09622-w
Villanoy, C., Cabrera, O., Bernardo, L. P., and Roa-Quiaoit, H. A. (2009). Oceanography and larval connectivity of macajalar Bay. Cagayan de Oro City, Phillippines: Macajalar Bay Development Alliance.
Vulliet, E., Wiest, L., Baudot, R., and Grenier-Loustalot, M. F. (2008). Multi-residue analysis of steroids at sub ng/L levels in surface and groundwaters using liquid chromatography coupled to tandem mass spectrometry. J. Chromatogr. A 1210, 84–91. doi:10.1016/j.chroma.2008.09.034
Wallace, J. S., and Aga, D. S. (2016). Enhancing extraction and detection of veterinary antibiotics in solid and liquid fractions of manure. J. Environ. Qual. 45 (2), 471–479. doi:10.2134/jeq2015.05.0246
Water Environment Partnership in Asia (2022). Municipal sewage treatment no.3. Retrieved from http://www.wepa-db.net/technologies/individual/datasheet/phi/06_municipal_no3.htm.
WorldWeatherOnline (2019). Weather data provided. https://www.worldweatheronline.com/hwd/.
Yamamoto, H., Nakamura, Y., Moriguchi, S., Nakamura, Y., Honda, Y., Tamura, I., et al. (2009). Persistence and partitioning of eight selected pharmaceuticals in the aquatic environment: laboratory photolysis, biodegradation, and sorption experiments. Water Res. 43 (2), 351–362. doi:10.1016/j.watres.2008.10.039
Zabal, B. R. (2020). Boracay draws 2 million tourists in 2019. https://www.panaynews.net/boracay-draws-2-million-tourists-in-2019/.
Keywords: pharmaceuticals, marine pollution, emerging contaminants, PPCP contamination, antibiotics
Citation: Mariano SMF, Angeles LF, Aga DS, Villanoy CL and Jaraula CMB (2023) Emerging pharmaceutical contaminants in key aquatic environments of the Philippines. Front. Earth Sci. 11:1124313. doi: 10.3389/feart.2023.1124313
Received: 15 December 2022; Accepted: 16 August 2023;
Published: 13 September 2023.
Edited by:
Venugopalan Ittekkot, University of Bremen, GermanyReviewed by:
Chui Wei Bong, University of Malaya, MalaysiaMorgane Derrien, Universidad de O’Higgins, Chile
Copyright © 2023 Mariano, Angeles, Aga, Villanoy and Jaraula. This is an open-access article distributed under the terms of the Creative Commons Attribution License (CC BY). The use, distribution or reproduction in other forums is permitted, provided the original author(s) and the copyright owner(s) are credited and that the original publication in this journal is cited, in accordance with accepted academic practice. No use, distribution or reproduction is permitted which does not comply with these terms.
*Correspondence: Caroline Marie B. Jaraula, cjaraula@msi.upd.edu.ph