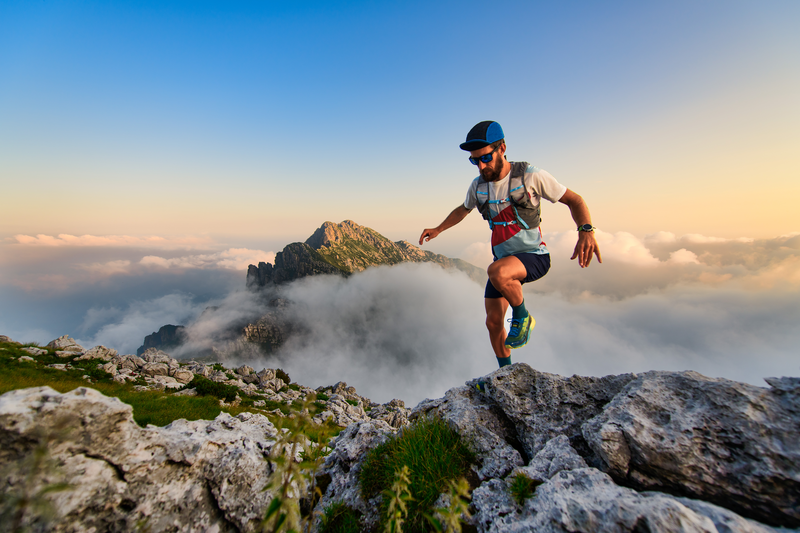
94% of researchers rate our articles as excellent or good
Learn more about the work of our research integrity team to safeguard the quality of each article we publish.
Find out more
ORIGINAL RESEARCH article
Front. Earth Sci. , 19 January 2023
Sec. Economic Geology
Volume 11 - 2023 | https://doi.org/10.3389/feart.2023.1122040
This article is part of the Research Topic Exploration, Exploitation, and Utilization of Coal-Measure Gas into the Future: Volume II View all 17 articles
Coal measure energy production and reservoir space utilization are both important for energy security and can assist in achieving carbon neutrality. Coal measure energy production and reservoir utilization are therefore of scientific significance. This study focuses on the security of coal supply by discussing the possible technical approaches of combining low-carbon mining of coal measure resources and coal reservoir space utilization. Our study found that 1) the integration of underground coal gasification and carbon capture and sequestration (UCG-CCS) is an effective technology to improve energy efficiency and achieve the carbon neutral target in China; 2) CO2-enhanced coalbed methane (CO2-ECBM) recovery is an important way to reduce CO2 emissions during coalbed methane recovery; and 3) the integrated technology of solid waste filling (WF), coal bioconversion (CB), gas production (GP), and CO2 capture and sequestration (CCS) in coal goafs and abandoned coal mines can improve the energy production efficiency and reservoir space utilization and reduce geological disasters due to surface subsidence. These integrated technologies will become increasingly important for coal measure energy production and efficient reservoir space utilization as they will provide scientific theory and technical support to achieve carbon neutrality.
China is richly endowed with coal but has fewer oil and gas resources. Coal production and consumption account for a high proportion of China’s energy production and consumption (Zou et al., 2021a; Chen et al., 2021; Xie et al., 2021). The total primary energy production in China increased from 628 million tons of standard coal in 1980 to 4.08 billion tons of standard coal in 2020, and the total energy consumption increased from 517 million tons of standard coal to 4.98 billion tons of standard coal over the same period (Figure 1). The Chinese gross domestic product (GDP) rapidly increased from 367.87 billion yuan to 101,598.62 billion yuan (National Bureau of Statistics of China, 2021; Zou et al., 2021b). The rapid development of China’s economy has been supported by the increased consumption of energy. The increased energy output also caused a large increase in greenhouse gas emissions, such as CO2 (Dong et al., 2014; Yang et al., 2020). In 2020 alone, China’s energy-related CO2 emissions were about 9.9 billion tons, accounting for 30.9% of the global total carbon emissions and ranking first in the world (Zhang et al., 2021). Against this background, even if the proportions of natural gas and renewable energy could be increased to reduce the carbon-intensive power supply, the development of renewable energy will not outpace the price and security advantages of coal-fired power (Hecking, 2016; Fan & Chen, 2018; Zhang et al., 2018). China’s 14th Five-Year Plan (2021–2025) and the Outline of Long-term Goals for 2035 specifically stated the following: “We will formulate an action plan to peak carbon emissions by 2030, improve the system of controlling both the total amount and intensity of energy consumption, and promote the clean, efficient and safe use of fossil energy such as coal.”
To keep the global temperature rise within 1.5°C, as proposed in the Paris Agreement, net-zero global CO2 emissions, where there is a balance between man-made removal and man-made emissions, must be achieved by 2050. This is known as “carbon neutrality” (Chen et al., 2021). Global climate catastrophes are becoming more and more severe. Achieving this goal is regarded as the key to climate security and the survival of humanity (Zhang et al., 2021). By the end of 2020, 127 countries and regions had put forward their carbon neutrality targets, with some having set timetables for restricting or banning the use of fossil energy (Chen et al., 2021). At the General Debate of the 75th Session of the United Nations General Assembly, President Xi Jinping of the People’s Republic of China promised that the CO2 emissions will achieve peak before 2030, and carbon neutrality will be achieved before 2060.
Coal measure energies, which include coal, coalbed methane, coal measure gas, and shale gas, are the main current sources of energy in China. The production of coal measure energies and the utilization of their reservoir spaces are important for achieving the carbon peak and carbon neutrality targets in China. Over the past few decades, significant progress has been made in the exploration and production of coal measure energies.
For a long time, coal mining rates were low in China, being affected by the resource conditions, the state of mining technologies, and resource management. The resource recovery rate of a state-owned coal mine was generally about 50%, while the resource recovery rate of locally administered state and township-individual coal mines with relatively poor conditions was only about 20%–30% (State Administration of Coal Mine Safety, 2002). From 1949 to 2014, the total amount of residual coal in China was 126.644 billion tons. These included state-owned, local state-owned, and township-individual coal mines at 26.93 billion tons, 20.198 billion tons, and 78.87 billion tons, respectively (Huang et al., 2014). The main obstacle was how to improve the coal mining rate.
In contrast, the volumes of coal goafs totaled 13.836 billion m3 in China by the end of 2016. These coal goafs led to frequent mining disasters such as land subsidence, and it is estimated that the volume of coal goafs in China will reach 23.452 billion m3 by 2030 (Xie, 2018). Challenges that must be addressed in coal goaf mining are how to efficiently use the underground space and reduce coal goaf mining disasters.
Coal measure gas in China has the characteristics of “three low and one high” (low pressure, low permeability, low saturation, and high content) due to the complex geological conditions that often complicate coal measure gas production (Lv et al., 2011). Improving the recovery of coal measure gas is a key problem to overcome. Only a few technologies can simultaneously exploit coal measure energy and optimally use coal reservoir space. Coal measure energy production must be increased to reach China’s carbon neutral target, and the reservoir space utilization must be optimized. To meet the demand for clean and green mining against the background of future carbon neutrality, this study considers these two technologies comprehensively by focusing on coal measure energy production and reservoir space utilization from theory and practice.
Underground coal gasification, which involves in situ coal gasification, is the controlled burning of coal under in situ conditions (Figure 2). The thermal and chemical reaction of the solid organic matter in the coal generates mixed gases during controlled burning. These gases can be converted into various fuels or raw materials after ground classification, such as natural gas for power generation, carbon monoxide, and hydrogen for synthetic ethanol. UCG is a relatively new technology for the clean utilization of coal and the production of chemical raw materials (Blinderman, 2017; Perkins, 2018). UCG has a high coal recovery rate (up to 85%) and can be generated at a low economic cost. It also has a limited environmental impact with almost no groundwater pollution risk. Since it is an unstaffed form of underground coal mining, it has a higher worker safety performance, especially for some thin coal seams, deep high gas coal seams, abandoned coal seams, and marginal unminable coal seams. However, its application can be restricted by the coal quality, hydrogeology, and other limitations (Qin et al., 2019).
The ash content of the slag in the oxidation zone is 94.9%, with a small amount of unburned carbon after UCG (Ma, et al., 2019). The volatile content of the residue in the reduction zone is lower than that of the residue in the dry distillation zone, and the fixed carbon content is slightly higher than that in the dry distillation zone. From the temperature and the reaction of the coal seam, the coal seam first undergoes drying and distillation and then undergoes gasification at a higher temperature, making the volatile content of the residual in the reduction zone lower and the fixed carbon content higher. The results show that the residues in the reduction zone and the dry distillation zone contain a small amount of O and S atoms.
Decades ago, researchers found significant differences in the adsorption capacities of gases in coal and shale (Figure 3). Generally, CO2 absorbs better on coal and shale than methane under the same conditions (Liu et al., 2010). Therefore, scientists proposed simultaneous CO2 sequestration and enhanced CBM and shale gas recovery in the same geological formation. Later, due to the demand for greenhouse gas emission reduction, CO2 sequestration in coal measure strata and enhanced coal measure gas recovery were favored.
Biogas is generated by microbial fermentation of organic substances under anerobic conditions. Biogas is generally generated in marshy areas. People often see marshes, sewage ditches, or cesspools emitting biogas bubbles. During biogas generation, human and livestock manure, straw, sewage, and other organic substances are fermented under anerobic (oxygen-free) conditions in a closed biogas tank, where biogas fermentation microorganisms decompose and transform the organic material to biogas. Methanation of the organic substrates under anerobic conditions is thought to occur in four generally accepted stages (Figure 4). The pathways of biogas are acetic acid fermentation and CO2 reduction:
Coal measure gas is an unconventional natural gas. There are two types of coal measure gas: thermal and biological. Biogenic coal measure gas, similar to biogas, is formed through the synergic degradation of organic matter by microbial communities in a coal seam. Due to the theory of biogenesis, coal bioconversion has been receiving more attention as a clean coal utilization technology (Zhang et al., 2017; Zhao et al., 2022).
The energy composition and carbon neutralization target in China requires that coal measure energy should be produced effectively, with low carbon emissions, and that the reservoir space should be utilized effectively. Therefore, several integrated technologies were proposed to exploit coal measure energy and efficiently use the reservoir space.
With continuous economic development, China’s demand for energy is on the rise. Most of the easily exploitable shallow coal seams have already been mined out. Traditional deep coal seam mining methods not only increase energy consumption and pollute the environment but also have a low recovery rate. After mining, it is difficult to use the residual coal reservoir space. Efficient technology is urgently needed to mine underground coal and ensure the rational utilization of the mined reservoir space to reduce disasters caused by surface subsidence. Therefore, we propose a technology that integrates UCG and CCS. In this integrated technology, combustible gas is provided by UCG, after which CO2 is captured and sequestrated in the residual UCG reservoir space.
Previous studies show that the coal reservoir space consists of UCG slag, the cavity, reduction coal, dry distillation coal, and raw coal after UCG, distributed outward along the gasification channel after UCG was implemented (Figure 5). There are significant differences in the material composition of the gasification slag in the three zones (Akbarzadeh & Chalatrunyk, 2014; Bhaskaran et al., 2015; Howaniec, 2016; Liu et al., 2018). As shown in Figure 2, Oxidation zone, reduction zone and dry distillzaiton zone. These factors cause significant differences in the CO2 sequestration paths of the three zones of the UCG space. Slag is mainly composed of alkaline oxides, silica, and a small amount of residue carbon. This means that CO2 sequestration on the slag surface occurs mainly via chemical reactions and/or CO2 adsorption on residual carbon (Figure 6). The chemical reactions that sequestrate CO2 are as follows:
The substances in dry distillation coal are dominated by organic matter and a small amount of morphologically transformed minerals. After high-temperature baking during coal gasification, dry distillation coal has a stronger gas adsorption capacity than raw coal and can, therefore, sequestrate more CO2 (Figure 7). The composition of reduced coal is between that of slag and dry distillation coal. CO2 sequestration in dry distillation coal mainly occurs via adsorption and mineralization.
The integrated technology of UCG and CCS is efficient for mining coal resources, and the UCG space can also be used effectively while at the same time providing a new pathway for geological CO2 sequestration.
Coal measure gas recovery is limited because the coal measure reservoirs have the “three low” (low pressure, low permeability, and low saturation) characteristics. Many researchers have suggested CO2-enhanced coalbed methane recovery to make up for the defect of the reservoir and to reduce CO2 atmospheric emissions during peak CO2 emissions (Clarkson & Bustin, 2000; Mukherjee & Misra, 2018). The integrated technology that we suggest is simultaneous coal measure gas production and CO2 sequestration. A schematic of this integrated technology is shown in Figure 8.
Thermodynamic calculations indicate that the maximum density of CO2 is 500–700 kg/m3 under the reservoir temperature and pressures characteristic of a depth of 800–1000 m (Orr, 2009). Supercritical carbon dioxide (SC-CO2) has both acidic and supercritical solvent characteristics. Therefore, it is both easy to have a series of complex interactions between SC-CO2 and some inorganic minerals and to extract small organic molecular substances from the coal, which changes its pore structure, adsorption, and permeability.
A weak acidic system is formed after CO2 has been injected into the coal measure strata. Under these conditions, carbonate minerals (such as calcite, siderite, and dolomite) in coal are prone to dissolution, precipitation, and carbonation, while clay minerals may undergo carbonation (Chen &Qin, 2012; Harvey et al., 2013; Du et al., 2018). The chemical reactions for the carbonate mineral dissolution are as follows:
The interaction between the CO2 and the carbonate and clay minerals affects the pore structure, adsorption capacity, and permeability of coal measure reservoirs, which in turn affects the CO2 injectability, sequestration capacity, and stability during the processing of enhanced coal measure gas recovery and CO2 sequestration (Chen & Qin, 2012). The interaction between the CO2 and carbonate minerals and/or clay minerals enlarges the pore size and increases the number of pores, which increases the pores’ special surface and increases the methane adsorption ability (Chen et al., 2017a). Carbonate minerals (calcite, dolomite, etc.) dissolved after the reaction of CO2-H2O increase the permeability of the coal seam (Zhang et al., 2015).
Previous studies have shown that supercritical CO2 extracted small and medium molecular substances that change the physical properties of coal, especially its adsorption and permeability characteristics. The adsorption of coal was enhanced at the low-rank stage and weakened at the high-rank stage, which generally enhanced the recovery of coal measure gas and improved CO2 geological sequestration (Chen et al., 2017b; Chen et al., 2018).
These studies have proved that CO2-ECBM is an important extraction method to improve coal measure gas production, reduce CO2 atmospheric emissions, and effectively utilize the coal measure reservoir space.
Previous studies have identified the presence of large volumes of coal measure gas in coal goafs and abandoned coal mines (Han, 2003; Meng et al., 2016). Coal mining in these strata can easily crack and collapse the overlying strata (Wang et al., 2022). Random stacking of coal gangue and disposal of domestic garbage cause land waste and environmental pollution (Wang, 2006; Jin et al., 2014). An integrated technology of gas production (GP), waste filling (WF), coal bioconversion (CB), and CO2 sequestration, based on the principles of biogas and biogenic coal measure gas, is proposed for the coal goafs and abandoned coal mines.
This paper uses the coal goaf as an example to illustrate the coal measure energy production and its reservoir space utilization. First, pipes are laid in the roof strata of the coal goaf for grouting and gas extraction after mining the coal. Figure 9 shows a schematic diagram of the coal goaf. Second, the coal goaf is infilled with solid wastes such as coal, gangue, and domestic garbage. Third, the coal goaf is closed to inject a solution of microorganisms and nutrients into the goaf. Fourth, CO2 is injected into the coal goaf after a period of gas extraction to sequestrate the CO2.
The feasibility of the integrated technology has been confirmed by research studies. After mining the coal seam, coal measure gas accumulates in the coal goaf through the micro-fissures due to induced pressure differences caused by the mining disturbance (Liu, 2011; Sun, 2014; Yin et al., 2014; Wang et al., 2022). After filling the goaf with domestic garbage and gangue, the coal goaf space is used effectively with WF, and the organic matter in the domestic garbage, the gangue, and the coal is bioconverted to gas by the microorganisms under the anerobic conditions in the goaf (Lin et al., 2006; Rathi et al., 2015; Ritter et al., 2015). Accumulated and bio-converted gases provide sufficient gas resources for extraction. Meanwhile, the mesopore-specific surface area and the pore volume are decreased after coal–gangue–domestic garbage bioconversion, while the micropore-specific surface area increases as the pore volume decreases. After bioconversion, the gas adsorption capacities are increased (Zhang et al., 2017; Wang et al., 2021), which means that the residues can sequestrate more CO2 in the goaf. The changes in the pore structure and gas adsorption enhance both CO2 sequestration in the coal measure strata and coal measure gas recovery. Therefore, the WF-CB-GP-CCS technology is suitable for energy production and reservoir space utilization in coal goafs and abandoned coal mines.
The principles of UCG, CO2-ECBM, biogas generation and CO2 sequestration are used against the background of carbon neutrality that integrates the concepts of coal measure energy production and reservoir space utilization. This study found that 1) the integrated UCG-CCS technology can ensure better energy exploitation and reservoir space utilization in minable coal seams. 2) The integrated CO2-ECBM is especially appropriate for coal measure gas exploitation and reservoir space utilization in unminable coal seams. 3) The integrated WF-CB-GP-CCS technology can be adopted for both energy production and effective reservoir space utilization in coal goafs and abandoned coal mines. These integrated technologies will become an important tool for coal measure energy production and reservoir space utilization and provide scientific theory and technical support for achieving the carbon neutrality target.
The original contributions presented in the study are included in the article/Supplementary Material. Further inquiries can be directed to the corresponding author.
RC and FC designed the facilities; YB, FL, KH, and YZ compiled the data and plotted the graphs; RC and FC writed original draft and reviewed; RC provided feedback to the manuscript.
The authors gratefully acknowledge the financial support from the Fundamental Research Funds for the Central Universities (2019XKQYMS24).
The authors declare that the research was conducted in the absence of any commercial or financial relationships that could be construed as a potential conflict of interest.
All claims expressed in this article are solely those of the authors and do not necessarily represent those of their affiliated organizations, or those of the publisher, the editors, and the reviewers. Any product that may be evaluated in this article, or claim that may be made by its manufacturer, is not guaranteed or endorsed by the publisher.
Akbarzadeh, H., and Chalaturnyk, R. J. (2014). Structural changes in coal at elevated temperature pertinent to underground coal gasification: A review. Int. J. Coal Geol. 131, 126–146. doi:10.1016/j.coal.2014.06.009
Bhaskaran, S., Samdani, G., Aghalayam, P., Ganesh, A., Singh, R., Sapru, R., et al. (2015). Experimental studies on spalling characteristics of Indian lignite coal in context of underground coal gasification. Fuel 154, 326–337. doi:10.1016/j.fuel.2015.03.066
Blinderman, M. S. (2017). Application of the exergy UCG technology in international UCG projects[C/OL]//2017 institute of physics (IOP) conference series: Proceedings of workshop on challenges and opportunities of underground coal gasification. Inst. Phys. Publ. 76 (1), 012009. doi:10.1088/1755-1315/76/1/012009
Chen, F., Yu, H. C., Bian, Z. F., and Yin, D. Y. (2021). How to handle the crisis of coal industry in China under the vision of carbon neutrality. J. China Coal Soc. 46 (06), 1808–1820. doi:10.31035/cg2021083
Chen, R., and Qin, Y. (2012). Fluid-solid coupling between supercritical CO2 and minerals in coal and geological significances. Coal Sci. Technol. 40 (10), 17–21. doi:10.1021/acs.energyfuels.7b02650
Chen, R., Qin, Y., Wei, C. T., Wang, L., Wang, Y., and Zhang, P. (2017b). Changes in pore structure of coal associated with Sc-CO2 extraction during CO2-ECBM. Appl. Sci. 7 (9), 931. doi:10.3390/app7090931
Chen, R., Wang, Y. Y., Qin, Y., Wang, L., Wei, C., and Zhang, P. (2017a). New discovery on supercritical CO2-H2O treated coal: Pore structure and methane adsorption. Acta Geol. Sin. Engl. Ed. 91 (4), 1509–1510. doi:10.1111/1755-6724.13385
Chen, R., Zhang, P. F., Qin, Y., Wei, C. T., and Wang, L. L. (2018). New discovery on methane adsorption change of coal due to Sc-CO2 extraction during CO2-ECBM. Acta Geol. Sin. Ed. 92 (6), 2438–2439. doi:10.1111/1755-6724.13741
Clarkson, C. R., and Bustin, R. M. (2000). Binary gas adsorption/desorption isotherms: Effect of moisture and coal composition upon carbon dioxide selectivity over methane. Int. J. Coal Geol. 42 (4), 241–271. doi:10.1016/s0166-5162(99)00032-4
Dong, W., and Yang, Y. (2014). Exploitation of mineral resource and its influence on regional development and urban evolution in Xinjiang, China. J. Geogr. Sci. 24 (6), 1131–1146. doi:10.1007/s11442-014-1143-x
Du, Y., Sang, S. X., Wang, W., Liu, S., Wang, T., and Fang, H. (2018). Experimental study of the reactions of supercritical CO2 and minerals in high-rank coal under formation conditions. Energy & Fuels 32 (2), 1115–1125. doi:10.1021/acs.energyfuels.7b02650
Fan, M., and Chen, L. (2018). Spatial characteristics of land uses and ecological compensations based on payment for ecosystem services model from 2000 to 2015 in Sichuan Province, China. Ecol. Inf. 50, 162–183. doi:10.1016/j.ecoinf.2019.01.001
Han, B. (2003). Xi'an. Shaanxi, China: Xi'an Branch of China Coal Institute.Study on evaluation method of CBM resource development potential in abandoned mines
Harvey, O. R., Qafoku, N. P., Cantrell, K. J., Lee, G., Amonette, J. E., and Brown, C. F. (2013). Geochemical implications of gas leakage associated with geologic CO2 storage-a qualitative review. Environ. Sci. Technol. 47 (1), 23–36. doi:10.1021/es3029457
Hecking, H. (2016). Twenty-first century, the century of coal? CO2 prices to curb coal demand. Oxf. Rev. Econ. Policy 32 (2), 260–281. doi:10.1093/oxrep/grw011
Howaniec, N. (2016). The effects of pressure on coal chars porous structure development. Fuel 172, 118–123. doi:10.1016/j.fuel.2016.01.028
Huang, W. G. (2014). Study on comprehensive evaluation and stable production technology for underground gasification of residue coal. Xuzhou, China: China University of Mining and Technology.
Jin, H., Wu, F., and Zhu, M. (2014). Mineral characteristics of coal gangue from liupanshui in guizhou province. Chin. J. Process Eng. 14 (1), 151–156.
Lin, C. M., Li, Y. L., and Qi, B. W. (2006). Research status and exploration potential of biogenic gas of the article. J. Pala. 8, 317–330. doi:10.1016/j.ngib.2019.01.001
Liu, S., Qi, C., Jiang, Z., Zhang, Y., Niu, M., Li, Y., et al. (2018). Mineralogy and geochemistry of ash and slag from coal gasification in China: A review. Int. Geol. Rev. 60 (5), 717–735. doi:10.1080/00206814.2017.1287013
Liu, Y. W., Su, Z. L., and Fang, H. B. (2010). Review on CBM desorption/adsorption mechanism. Well Test. 19 (6), 37–44. doi:10.11885/j.issn.1674-5086
Lv, Y., Tang, D., Xu, H., and Tao, S. (2011). CO2 sequestration enhancing coalbed methane recovery. Environ. Sci. Technol. 34 (5), 95–99.
Ma, A. L., Chen, L. J., and Xu, B. (2019). Study on physicochemical properties of “three zone” residues during underground coal gasification. Coal Sci. Technol. 47 (11), 217–223.
Meng, Z. P., Shi, X. C., Tian, Y. D., and Li, C. (2016). Evaluation model of CBM resources in abandoned coal mine and its application. J. China Coal Soc. 41 (3), 537–544.
Mukherjee, M., and Misra, S. (2018). A review of experimental research on Enhanced Coal Bed Methane (ECBM) recovery via CO2 sequestration. Earth-Science Rev. 179, 392–410. doi:10.1016/j.earscirev.2018.02.018
National Bureau of Statistics of China, (2021). China statistical yearbook 1978-2020. Beijing, China: China Statistics Press.
Orr, F. M. (2009). Onshore geologic storage of CO2. Science 325 (5948), 1656–1658. doi:10.1126/science.1175677
Perkins, G. (2018). Underground coal gasification-part I: Field demonstrations and process performance. Prog. Energy Combust. Sci. 67, 158–187. doi:10.1016/j.pecs.2018.02.004
Qin, Y., Wang, Z. T., and Han, L. (2019). Geological problems in underground coal gasification. J. China Coal Soc. 44 (8), 2516–2530.
Rathi, R., Priya, A., Vohra, M., Lavania, M., Lal, B., and Sarma, P. M. (2015). Development of a microbial process for methane generation from bituminous coal at thermophilic conditions. Int. J. Coal Geol. 147-148, 25–34. doi:10.1016/j.coal.2015.06.004
Ritter, D. J., Vinson, D. S., Barnhart, E. P., Akob, D. M., Fields, M. W., Cunningham, A. B., et al. (2015). Enhanced microbial coalbed methane generation: A review of research, commercial activity, and remaining challenges. Int. J. Coal Geol. 146, 28–41. doi:10.1016/j.coal.2015.04.013
State Administration of Coal Mine Safety, (2020). China coal industry yearbook 2002. Beijing, China: Coal Industry Press.
Sun, H. D. (2014). Study on distribution and evaluation methodology of CBM (coal mine gas) resources in coal resource depleting mine. Xuzhou, China: China University of Mining and Technology.
Sun, Y. N. (2011). The research on the law of methane gas flow and the structure of interspaces in surrounding rock of coal goaf. Fuxin, China: Liaoning Technical University.
Wang, A., Shao, P., and Wang, Q. (2021). Biogenic gas generation effects on anthracite molecular structure and pore structure. Front. Earth Sci. 15 (2), 272–282. doi:10.1007/s11707-021-0925-6
Wang, J. C., Yang, Z. B., and Qin, Y. (2022). Research status and prospects of secondary enrichment and accumulation of residual coalbed methane resources in abandoned mines. Coal Geol. Explor. 50 (4), 35–44.
Wang, X. F. (2006). Study on hydrodynamic law of trace element pollution in coal gangue leaching. Wuhan, China: Huazhong University of Science and Technology.
Xie, H. P., Gao, M. Z., and Liu, J. Z. (2018). Research on exploitation and volume estimation of underground space in coal mines. J. China Coal Soc. 43 (6), 1484–1503.
Xie, H. P., Ren, S. H., and Xie, Y. C. (2021). Development opportunities of the coal industry towards the goal of carbon neutrality. J. China Coal Soc. 46 (6), 1808–1820.
Yang, Y., Yu, H. Y., Lu, G., Wang, L. m., Zhao, Y., and Hao, L. s. (2020). Interview on the unprecedented changes of energy geopolitics and national energy security. J. Nat. Resour. 35 (11), 2803–2820. doi:10.31497/zrzyxb.20201119
Yin, Z. S., Sang, S. X., and Zhou, X. Z. (2014). Study on migration and enrichment regularities of CBM in exhausted coal resource. Wells Special Oil Gas Reserv. 21 (5), 48–51.
Zhang, L. L., Long, R. Y., and Chen, H. (2018). Carbon emission reduction potential of urban rail transit in China based on electricity consumption structure. Resour. Conservation Recycl. 142, 113–121. doi:10.1016/j.resconrec.2018.11.019
Zhang, R., Liu, S. M., Bahadur, J., Elsworth, D., Wang, Y., Hu, G., et al. (2017). Changes in pore structure of coal caused by coal-to-gas bioconversion. Sci. Rep. 7, 3840. doi:10.1038/s41598-017-04110-z
Zhang, W. Y., Ni, X. M., and Wang, Y. B. (2015). Variation of coal permeability after injection carbon dioxide reactions with minerals in coal. J. China Coal Soc. 40 (5), 1087–1092.
Zhang, Y. S., Dong, D., Xiao, Y., Wang, T., and Wang, J. (2021). Current status and trends in energy production, consumption, and storage under carbon neutrality conditions in China. China Sci. Bull 66, 4466–4476. doi:10.1360/tb-2021-0797
Zhao, W. Z., Su, X. B., Xia, D. P., Hou, S., Wang, Q., and Zhou, Y. (2022). Enhanced coalbed methane recovery by the modification of coal reservoir under the supercritical CO2 extraction and anaerobic digestion. Energy 259, 124914. doi:10.1016/j.energy.2022.124914
Zou, C. N., He, D. B., and Jia, C. Y. (2021b). Connotation and pathway of world energy transition and its significance for carbon neutral. Acta Pet. Sin. 42 (2), 233–247.
Keywords: carbon neutral target, CO2 sequestration, reservoir space utilization, UCG-CCS, WF-CB-GP-CCS
Citation: Chen R, Bao Y, Lv F, Chen F, Hu K and Zhang Y (2023) Coal measure energy production and the reservoir space utilization in China under carbon neutral target. Front. Earth Sci. 11:1122040. doi: 10.3389/feart.2023.1122040
Received: 12 December 2022; Accepted: 05 January 2023;
Published: 19 January 2023.
Edited by:
Zhenzhi Wang, Henan Polytechnic University, ChinaReviewed by:
Rui Li, Chongqing University, ChinaCopyright © 2023 Chen, Bao, Lv, Chen, Hu and Zhang. This is an open-access article distributed under the terms of the Creative Commons Attribution License (CC BY). The use, distribution or reproduction in other forums is permitted, provided the original author(s) and the copyright owner(s) are credited and that the original publication in this journal is cited, in accordance with accepted academic practice. No use, distribution or reproduction is permitted which does not comply with these terms.
*Correspondence: Run Chen, Y2hlbnJ1bkBjdW10LmVkdS5jbg==
Disclaimer: All claims expressed in this article are solely those of the authors and do not necessarily represent those of their affiliated organizations, or those of the publisher, the editors and the reviewers. Any product that may be evaluated in this article or claim that may be made by its manufacturer is not guaranteed or endorsed by the publisher.
Research integrity at Frontiers
Learn more about the work of our research integrity team to safeguard the quality of each article we publish.