- Yunnan Key Laboratory for Palaeobiology and MEC International Joint Laboratory for Palaeobiology and Palaeoenvironment, Institute of Palaenotology, Yunnan University, Kunming, China
Genic and genomic data have been reshaping our understanding of the earliest radiation event of metazoans, the well-known Cambrian Evolutionary Radiation, not only from the respects of reshuffling the phylogenetic topologies of some animal phyla but by deciphering the deep homologies of many morphological features. These advances, together with the continuing discoveries of the Ediacaran-Cambrian fossils, are unveiling the cladogenetic process of the early metazoans and the patterns of morphologic evolution during this biological radiation event. In this review, I focus on a small but challenging field, the problematic fossils from the early Cambrian fossil Lagerstätten, such as the Chengjiang biota, mainly on the controversies concerning their interpretation and the consequent impacts on understanding the early evolution of animals. The bizarre body plans of the early Cambrian problematica alone do not account for the difficulties in studying their biology and affinity. Instead, it is the combined action of the taphonomic artifacts and the uncertainty in homologizing the preserved characters that impede generating plausible interpretations. Despite all these issues, a testable and repeatable method for interpreting fossils has emerged and is becoming more practicable. The integration of an evolutionary-grade conceptual frame is beneficial to the interpretation of the Cambrian problematic fossils. Together with the focus on taphonomic alternation and homologic assessment, the Cambrian problematic fossils are becoming more informative nodes in the “parsing tree” of early animal evolution.
1 A sententious background
Ever since Darwin’s time, the early Cambrian has been a contentious geological interval remarkable for the sudden appearance of the fossils of diverse animal crown groups. Nowadays, it has been widely acknowledged that the boost of crown animal fossils during this short geological time interval represents a veritable evolutionary radiation event, i.e., the Cambrian Evolutionary Radiation (more popularly known as the Cambrian Explosion). Nevertheless, animal origin and their early cladogenesis might have a deep root in the pre-Cambrian period (Knoll and Carroll, 1999; Budd and Jensen, 2000; Valentine, 2002; Darroch et al., 2018). Extensive compilations of fossil discoveries and paleoenvironmental proxies, especially after integrating those from the last 2 decades (Sperling and Stockey, 2018; Wood et al., 2019), further confirm the previous claims that animals originated and their early clades diverged as a result of interplays of environmental controls (on life resources), developmental capacity and ecological boosting (Knoll and Carroll, 1999; Marshall, 2006; Rokas, 2008), via a gradual manner (Pontefract and Stone, 2007). In this sense, the diverse crown animal fossils that appeared in the early Cambrian rather than in the Neoproterozoic are not a taphonomic artifact. Rather, it can be logically interpreted as due to the decoupling of the Neoproterozoic evolutionary novelties, both of genetics and morphology, from the ecological successes in the early Cambrian (Seilacher, 2007; Erwin et al., 2011; Erwin, 2021). Such a scenario is intermittently endorsed by comparative studies of gene expression and gene regulatory networks (GRNs) across animal phyla, which keep revealing deep (genic) homologies of many features of crown eumetazoans, such as segmentation, eyes, appendages, muscles, etc. In other words, these features might have their GRNs (at least some elements) evolved in the last common ancestor of bilaterians (LCAB) or even earlier, but the key morphological innovations (the features themselves) that lead to the ecological success evolved later, sometimes in different bilaterian groups by convergence (Davidson et al., 1995; Kimmel, 1996; Arendt and Wittbrodt, 2001; Erwin and Davidson, 2002; Oakley, 2003; Chipman, 2010; Marshall and Valentine, 2010; Chipman, 2018). Another example is the origin and evolution of bilaterality. Growing comparative data reveal that the developmental toolkit of bilateral symmetry in bilaterians might have started to build up back into the time when metazoans began to assemble their multicellular body plan. For instance, Wnt and BMP signaling pathways and Hox genes, the most critical components of bilaterality GRNs, are all present (evolved) in the non-bilaterian taxa (Martindale, 2005; Erwin, 2009; Martindale and Hejnol, 2009), albeit bilaterian-specific genes might mark the appearance of the first true bilaterian (Heger et al., 2020). Intriguingly, further comparisons of gene controls and developmental data with the non-bilaterian animal Nematostella (Cnidarian) show that bilaterality does not necessarily evolve only once during the transition from non-bilaterians to bilaterians (Genikhovich and Technau, 2017).
This framework of the early metazoan evolution inferred from the combined data of genetics and development, together with that from paleontology itself, has profoundly influenced the paleontologic community. For example, it has been accelerating the shift of the community focus from the early Cambrian animals to the records of Ediacaran, Cryogenian, or even Tonian (Knoll and Carroll, 1999; Mills et al., 2018), the periods during which metazoans are predicted to have originated and diverged (Yang et al., 2007; Peterson et al., 2008; Reis et al., 2015; Lozano-Fernandez et al., 2017; Costa-Paiva et al., 2022). Searching for representatives of extant phyla in the Neoproterozoic strata is becoming more promising, as exemplified by recent discoveries of bilaterian fossils (Chen et al., 2019; Evans et al., 2020) and crown cnidarian in Ediacaran (Dunn et al., 2022). So do searching eumetazoan features in Ediacaran fossils and homologizing them across the metazoan tree (Droser and Gehling, 2015; Zhuravlev et al., 2015; Dunn et al., 2017; Dunn and Liu, 2019; Schiffbauer et al., 2020; Evans et al., 2021). This growing body of evidence further validates the long-standing point that eumetazoans and their main subclade bilaterians originated before the onset of the Cambrian. Nonetheless, such a condition does not necessarily eliminate the importance of the Cambrian fossils, as the main burst of animal phyla and the evolution of their stem groups are still hinted to have occurred in the early Cambrian under the framework summed up above. More importantly, the early Cambrian fossils, particularly those with soft-bodied tissues preserved, can provide unparallel evidence about the morphology of early animals on account of the unusual amount of fossil Lagerstätten during this time interval and the exclusive information of the soft-body parts unseen in general preservation (Butterfield, 2003; Briggs and Fortey, 2005; Budd, 2013; Briggs, 2015).
Most Cambrian soft-bodied fossils can be assigned to living animal phyla with varying degrees of confidence based on shared characters, making it less problematic to interpret their morphology and evaluate their evolutionary significance. Examples of this category are various diverse euarthropod fossils that are widely considered to occupy different positions along the stem lineages of arthropods (see Edgecombe, 2020 for a recent review). In addition to these ‘normal’ fossils, however, there are many Cambrian fossils that cannot be readily allied to extant phyla, known as problematic fossils or ‘wired wonders’ (Gould, 1989). They usually possess a body plan incompatible with the living animal phyla or, occasionally, bear features across diverse (supra-)phylum-level clades. The importance of the fossil problematica in understanding the early animal evolution has long been acknowledged (Hoffman & Nitecki, 1986; Simonetta & Conway Morris, 1991). Interpreting their morphology and inferring their affinities, particularly those with preserved soft tissues are proven to be very challenging though. This is not only because of their bizarre body plan, but due to taphonomy, the process of transforming living organisms into fossils exerts much more alternations to their preserved morphology than to fossils only with hard parts (Briggs and Williams, 1981; Allison, 1986; Briggs, 2003). The methodology for studying problematic fossils has been developed and improved with time. Two crucial issues have been identified initially, i.e., 1) the necessity to evaluate multiple hypotheses on the interpretation of morphology, and 2) the employment of computer-based cladistic analysis, which are expected together to bring forth repeatable and testable results (Beall, 1991). With the rapid utilization of computer tools, morphology-based cladistic analysis has become a conventional method in palaeontologic studies. It is worth noting, though, that the validation of cladistic analysis heavily relies on the quality of the matrix it works on, of which the coding of fossils is solely based on the interpretation. That is to say, the first issue, evaluating all alternative interpretations, plays an essential role in studying problematic fossils. This usually comes down to distinguishing taphonomic artifacts, selecting comparative models, assessing homologies, and of course, collecting new fossils (Jenner and Littlewood, 2008; Donoghue and Purnell, 2009; Cong et al., 2015).
Over the last 3 decades since a special international symposium on problematica (Simonetta & Conway Morris, 1991), remarkable advances have been achieved in this small but challenging field. Some Cambrian weird wonders have found their ‘home’ in the animal tree of life, such as conodonts as chordates (Sansom et al., 1992; Aldridge et al., 1994; Pridmore et al., 1996; Donoghue et al., 2000), cambroernids as stem ambulacrarian (Caron et al., 2010; Li et al., 2023), halkieriids as molluscs (Vinther et al., 2017), camenellan tommotiids as stem lophophorate (Guo et al., 2022), radiodonts (anomalocaridids and their relatives) as a lower stem group of euarthropods (Budd and Telford, 2009; Daley et al., 2009; Edgecombe and Legg, 2014). While some other fossil taxa are turned into problematica with increasing, drastic debates concerning their morphology and affinities, such as yunnanozoans (Hou et al., 1991; Chen et al., 1995; Dzik, 1995; Shu et al., 1996; Chen et al., 1999; Mallatt et al., 2003; Shu, 2003; Shu et al., 2003; Cong et al., 2015; Tian et al., 2022), vetulicolians (Hou, 1987; Shu et al., 1999; Shu et al., 2001; Lacalli, 2002; Briggs et al., 2005; Caron, 2005; Shu, 2005; Aldridge et al., 2007; Lieberman, 2008; Ou et al., 2012; García-Bellido et al., 2014) and nectocaridids (Simonetta, 1988; Chen et al., 2005; Smith and Caron, 2010; Mazurek and ZatoŃ, 2011; Smith and Caron, 2011; Smith, 2013) etc. A similar situation also occurred in extant problematic taxa (e.g., placozoans, xenoturbellids, aceolomorphs, chaetognaths), of which the progress has been constantly reviewed in the systematic phylogeny field (e.g., Jenner and Schram, 1999; Edgecombe et al., 2011; Dunn et al., 2014; Telford et al., 2015; Giribet, 2016; King and Rokas, 2017).
In this review, I mainly focus on the controversies in the interpretation of the Cambrian problematic fossils and propose to integrate the concept of evolutionary grade into the interpreting process. Examples, especially those I have worked on during the last couple of years, are used to demonstrate how this developing method impacts our understanding of early animal radiation. This is not to say, however, that other Cambrian problematic fossils are not important. These examples are chosen mainly because they are typical in understanding the evolution of the three main metazoan grades (sponge-, diploblastic, and bilaterian), and in illustrating the impacts of the interpreting method.
2 Integrating the concept of evolutionary grade into interpreting problematic fossils
Like all other evolutionary studies, interpreting problematic fossils requires a phylogenetic frame as robust as possible. However, phylogenic analyses themselves, either morphologic data-based or molecular data-based, are a debate topic. This is particularly emblematic of the relationships among animal phyla and the higher taxonomic levels (Jenner and Schram, 1999; King and Rokas, 2017; Kapli et al., 2021). Such as it is, the profound revolutions in animal phylogeny in the last 3 decades have provided a more accurate phylogenetic topology than ever before, as marked by the recoveries of the three great clades (deuterostomes, ecdysozoans, lophotrochozoans) of bilaterians in the mid-1990s, (e.g., Aguinaldo et al., 1997). Many animal phyla (and sub-phyla) of these three clades have their phylogenetic positions reshuffled from the traditional classification based on morphology, anatomy, and embryogenesis, and many consensuses have been achieved. For example, several crucial phylogenetic relationships among higher taxonomic levels of deuterostome are now consistently embedded in the literature, with echinoderms and hemichordates grouped in a clade named ambulacraria and cephalochordate being the sister group to the olfactories (tunicates and vertebrates), (e.g., Swalla and Smith, 2008; Peterson and Eernisse, 2016; Nanglu et al., 2023). In protostome, we are now more confident about the phylogenetic position not only of the common animals such as arthropods, molluscs, annelids, brachiopods, and bryozoans but of some small cryptic groups such as chaetognaths, gnathifers, tardigrades, xenoturbellids, aceolomorphs (Laumer et al., 2019), albeit final agreements are far from being reached about the inner relationship in either ecdysozoans (e.g., Giribet and Edgecombe, 2017) or lophotrochozoans (e.g., Kocot, 2016; Marlétaz et al., 2019). While in the basal part of the animal tree of life, the phylogenetic position of ctenophores have recently become a bitterly disputed issue on whether they or sponges are the first branch of metazoans (see King and Rokas, 2017 for a recent review on this topic). And several recent genomic phylogenetic analyses began to question the monophyly of deuterostomes (Philippe et al., 2019; Kapli et al., 2021). In sum, these continuously updating metazoan phylogeny would inevitably alter the interpretation of the Cambrian problematic fossils, almost all of which are now considered stem groups of metazoan clades, or at least are interpreted in the conceptual framework of stem group (Budd and Jensen, 2000; Briggs and Fortey, 2005).
The introduction and application of the concept of stem group in paleobiology have facilitated the interpretation of fossils, including problematic ones, by allowing to plot the acquisition sequence of synapomorphic characters of crown groups along their stem lineages in a stepwise manner (Jefferies, 1979; Budd and Jensen, 2000; Briggs and Fortey, 2005; Donoghue, 2005). In the case of problematic fossils, the situation is much more complicated though. This is mainly because identifying the affinities of a problematic fossil taxon needs to determine first which phylum or higher-level taxon it might belong to, which itself is a matter of debate. For example, the proposed affinities of Yunnanozoon lividum Hou et al., 1991 from the Chengjiang biota range from the stem all through to every subclade of bilaterians, such as ecdysozoans, lophotrochozoans, and deuterostome (Cong et al., 2015) (see Section 4 for details), a situation which clearly cannot be accommodated simply by the stem group concept. Similarly, the debates on Conicula striata Luo et Hu, 1990 mainly concern whether it is a diploblastic animal or a triploblastic lophophorate (see Section 3.3 for details), which would require a different conceptual frame, such as evolutionary grade, within which to conduct character identification, morphological comparison, and homological reasoning before its morphology can be further evaluated as stem group of a particular clade.
The term evolutionary grade was first coined by Huxley (1959) and defined as a level of evolutionary advance in a (or part of) given tree of life. In practice, the term grade is usually used as a taxonomic combo as it is not necessarily monophyletic. This attribute has limited its wide usage in modern phylogenetic and systematic works that usually aim to recover monophyletic clades. The evolutionary grade is typically introduced and defined by a key morphological innovation that contributes to advantages in evolutionary and ecological adaptation (Figure 1). For example, all feathered dinosaurs (polyphyletic) can be regarded as an evolutionary grade from which the modern aves arose as feathers might have given them advantages in displaying sexual attraction and keeping body temperature during the early evolution of stem birds and their dinosaur relatives. In modern theory, the morphological innovation that leads to ecological success is regarded as a gradual assembling of evolutionary novelties, either of genetic or functional components and developmental identities (Wagner and Lynch, 2010; Erwin et al., 2011; Erwin, 2015; Erwin, 2021). This means the evolutionary innovation of a morphology feature can be decomposed into a hierarchical scheme, with each hierarchy level representing a sub-grade if they are distinguishable in some sense. For example, the bilaterian grade is marked by the innovation of bilaterality that can be decomposed into two hierarchical levels at least, say the novelty (or innovation) of the anterior-posterior (A-P) axis and the logically later novelty of the dorsal-ventral (D-V) axis. This scenario can be inferred because we know that cnidarians, ctenophores, and some sponges have an oral-aboral axis that is comparable with (but not necessarily homologous to) the A-P axis of bilaterians but lack an actual D-V axis (Martindale, 2005; Martindale and Hejnol, 2009; Kraus et al., 2016; Genikhovich and Technau, 2017). In this sense, the origin of bilaterian clade can be decoupled into a hierarchical scheme with two different levels. And this view of evolutionary grade is argued here to be applicable to the animal tree of life.
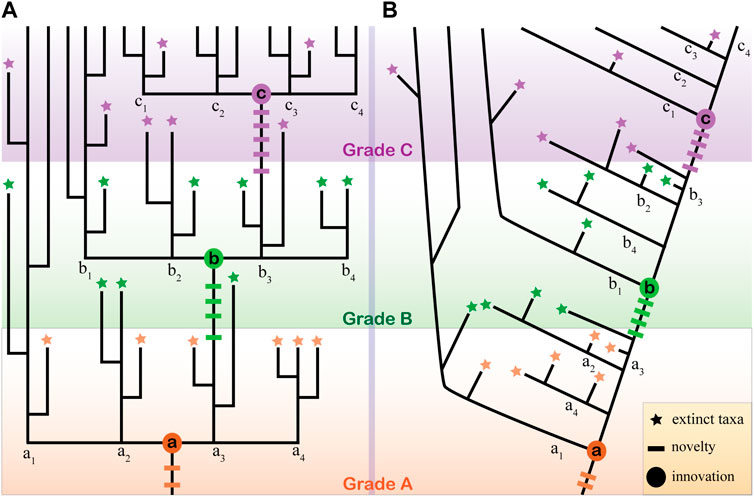
FIGURE 1. Evolutionary grades and the extinct taxa interpretation. (A) An ideal condition illustrating three evolutionary grades (A, B, C) introduced by three evolutionary innovations (a, b, c in circles). Each innovation marks the establishment of a clade that gives rise to four subclades. Each grade, innovation, and the related multiple evolutionary novelties (short transverse line) are distinguished by colors (reddish, green, and purple). Note the earlier grades A and B only have one subclade (a1 and b1) that survived. Extinct fossils (stars) with the same color denote their grade origin or convergent evolution of similar features. (B) In a conventional dichotomous tree, the evolutionary grades are not easy to be depicted, even when the topology is fully resolved.
Each node of higher taxonomic levels in the animal tree of life, usually defined by at least one key morphological feature (synapomorphy), can also be treated as an evolutionary grade. In addition, the animal body plan can be disassembled into hierarchical levels from the perspective of GRNs (Davidson and Erwin, 2006; Erwin and Davidson, 2009; He and Deem, 2010) and thus can potentially be treated as another hierarchical system of evolutionary grades, although linking this GRN hierarchical system with the morphological innovations is still awaiting more works on morphological genesis. Nevertheless, Nielsen’s six steps summarized from morphological and developmental data can be used as another upgradable working template for depicting the evolutionary grades of the early animals (Nielsen, 2008; Nielsen and Parker, 2010; Nielsen, 2019).
This view of evolutionary grades might merely mean a few things in recovering the animal tree of life, as the grades are usually non-monophyletic. However, it can be beneficial to interpret the problematic fossils in several aspects. Firstly, identifying the evolutionary grade can help narrow the range of model selection. In the recently formalized procedure of problematica interpretation, comparing models need to be chosen after the characters of a problematic fossil are identified correctly (Donoghue and Purnell, 2009; Cong et al., 2015). Choosing the comparing models is somewhat arbitrary and lacks instructions in practice. Identifying the potential evolutionary grades of a problematic fossil is a testable and resultful procedure in selecting models, thus facilitating its interpretation. Secondly, evolutionary grades can facilitate the visualization of all potential characters that need to be compared with and all potential phylogenetic positions that need to be considered. Assuming a clade with three evolutionary grades (A, B, C), each with four subgroups (Figure 1A). A problematic fossil with any combination of the novelty characters (colored short transverse lines in Figure 1A) from the three grades can be tentatively grouped within this clade. In this sense, the terminal nodes of extinct taxa represent all possible phylogenetic positions, a situation analogous to the known conditions in interpreting the Cambrian problematic fossils. This large number of possibilities can be narrowed down to a smaller subset if the grade that the fossil belongs to can be confirmed (for example, Grade B). Then the interpretation of the fossil, both about its characters and affinity, would be turned into a question of whether it is an extinct member of clade B or a remote extinct taxon descendent from groups a1 or a2 (Figure 1A). In short, the advantage of integrating this evolutionary grade into the interpretation of problematic fossils is prominent as it not only further decomposes one more testable step but clarifies the specific objectives during the practice of interpreting. For example, during the re-interpretation of the Chengjiang fossil C. striata, it was noticed that at least three sets of features are arranged in a radial manner (tentacles, spine-like structures, and longitudinal lines), which strongly argues a radial symmetry. This higher hierarchical level of body plan fits C. striata well into the diploblastic grade, which would exclude selecting any bilaterians as its comparing models (Zhao et al., 2023).
It is worth noting that in a conventional dichotomous tree that focuses on the stem group concepts, the evolutionary grades are easy to be overlooked (Figure 1B). However, I am not arguing that stem groups are disadvantageous; this just means that integrating the evolutionary grade concept helps bring forth repeatable and testable results in interpreting problematic fossils, two principles set up back to 1990s (Beall, 1991). It should also be noted that the resolution of evolutionary grades heavily relies on our understanding of the origin and evolution of innovations, i.e., the assembling sequence of the novelties. In the practice of metazoan, the uncertainty in the phylogenetic relationships and the polarity of critical features (the morphological innovations) impede the application of the evolutionary grade analysis. A more practical method might be to allow the parallel systems of evolutionary grades to exist in a given tree. For example, chancelloriids have a similar developing mode with sponges and thus can be regarded as sponge-grade animals (Cong et al., 2018). This does not conflict with the claim that chancelloriids are epitheliozoan-grade based on the presence of epidermal-derived integument (Bengtson and Hou, 2001; Yun et al., 2021) (see below).
3 Non-bilaterian problematic fossils and the “eumetazoan” grades
3.1 The body plan of early animals was assembled in a graded manner
The diverse animal body plans are one of the most marvelous evolutionary results in the history of life. Multicellularity is the first and most fundamental step in the origin of metazoans, which would require adhesion, communication, and differentiation among cells decedent from their unicellular ancestors (Richter and King, 2013; Brunet and King, 2017; Sebé-Pedrós et al., 2017). It is on this foundation that the establishment of body axes (and body symmetry), the differentiation of germ layers, and the emergence of peripheral tissues/organs can be unfolded in various temporal and spatial patterns along extinct and living groups. The evolutionary history of metazoans before the appearance of the first urbilaterians is sporadically recorded in the fossils from the later Neoproterozoic to the early Cambrian period and deeply embedded in the genomes of non-bilaterian animals. Unfortunately, both the two sources of information are heavily blurred by the long-time evolution, as reflected by the controversies in interpreting the Ediacaran fossils (e.g., Seilacher et al., 2003; Dunn and Liu, 2019) and the violent debates on the phylogenetic relationships of non-bilaterian groups (see Giribet, 2016; King and Rokas, 2017 for the recent review). Despite all these, a consistent view of early animal evolution, particularly concerning their body plan, is emerging, which is essential in interpreting fossils. This comes to two points: 1) the identity of morphological characters, such as body axes, rooted in their GRNs, and 2) the animal body plan was assembled gradually (although probably rapidly), either of morphology or genetics if we take the body plan of triploblastic bilaterian as a point to polarize the evolution non-bilaterians. The first point allows the interpretation of fossil characters to fit into a framework constructed from the comparative genomic and genetic work after further adequate morphogenetic information is integrated. For example, we are now becoming more confident than any time before to discuss the development of Ediacaran fossils purely based on morphological data and link this with early animal evolution (Dunn et al., 2017; Evans et al., 2021). While the second point enables us to decompose the early evolution of animals into different (sets of) grades, thus making the interpretation of fossils a more testable procedure.
3.2 Interpreting chancelloriids in hierarchical evolutionary grades
Chancelloriids are a group of Cambrian fossils bearing a saccular body covered with hollowed sclerites (Figure 2A). They have a well-constrained apical-abapical axis, like some living sponges (Leininger et al., 2014). Although the sclerites of chancelloriids are not arranged in any sign of a symmetrical pattern, neither those on the main body part nor of the oral tufts, the roughly perfect conical or cylindrical body with one axis indicates that they have a radial symmetry (Bengtson and Collins, 2015). Intriguingly, the comparative study reveals that a growing zone might be in the subapical region, which can developmentally maintain the difference of the sclerites in the tuft and other body regions (Bengtson et al., 1996; Bengtson and Collins, 2015; Cong et al., 2018), a developmental pattern also known in living calcarean sponge Sycon ciliatum (Voigt et al., 2014). This body architecture of chancelloriids, together with their unique skeletonization, epidermis-derived integument (Bengtson and Hou, 2001; Yun et al., 2021), and the inferred muscular contraction (Bengtson and Collins, 2015), confine the phylogenetic position of chancelloriids within the evolutionary grades before the origin of bilaterians, either as sponges (Botting and Muri, 2018), sponge-like (Cong et al., 2018), cnidarian-like (Bengtson and Collins, 2015) or epitheliozoan-grade animals (Yun et al., 2021) that include sponges and cnidarians (see below).
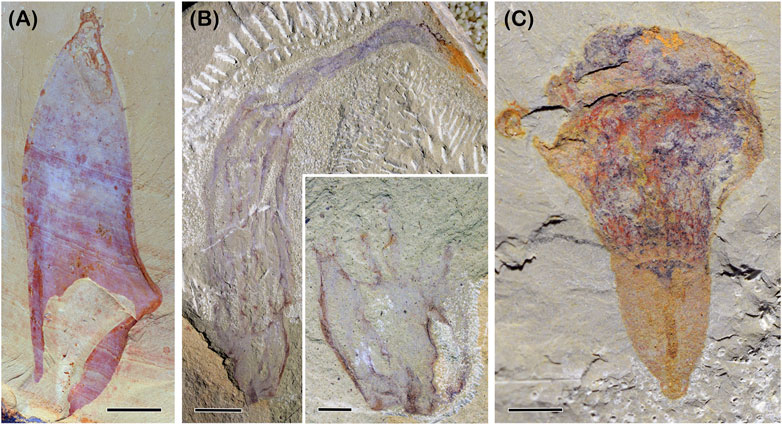
FIGURE 2. Selected non-bilaterian problematica from the early Cambrian. (A) chancelloriid Allonia nuda Cong et al., 2018, YKLP 13501. (B) Mackenziid Paramackenzia canalifera Zhao et al., 2022, YKLP 13348. Inset showing the stalk preserved in the counterpart. (C) Diploblastic probematicum Conicula striata Luo et al., 1999, YKLP 13212. Image courtesy of Zhao, Y. Scale bars: 2 cm (A); 1 cm (B); 5 mm inset of (B), (C).
Among the features of chancelloriids highlighted above, an apical-abapical axis would represent a higher hierarchical level of the evolutionary grades as the tissue/organ-based grades would require this spatial dimension at first, although their origins were not necessarily later than the origin of the axis. In this sense, an evolutionary grade of one main body axis may include many monophyletic taxa, such as the living clades of sponges, ctenophores, cnidarians, bilaterians, and the fossil taxa such as chancelloriids and many Ediacaran fossils. In theory (Figure 1A), chancelloriids can be the stem group of the living clades or represent an independent extinct clade that originated during this grade. Furthermore, in bilaterians, an additional D-V axis and left-right axis are present, which, together with the A-P axis, define the spatial frame of the body architecture. Additional axes are also known in ctenophore (stomodeal axis and tentacular axis) and cnidarians (directive axis). To this extent, chancelloriids are more comparable with sponges in lacking any other symmetrical axis/plane in addition to their apical-abapical one assuming their body plan is not a product of secondary reduction.
Under the hierarchical level of the body axis are those levels of tissues/organs, such as musculature, epithelia, and canal system, etc. In this sense, the inferred presence of contracting muscles in chancelloriids is critical not only in understanding their anatomy but in testing their phylogenetic analysis (Bengtson and Collins, 2015). Sponges are generally thought lacking muscles, while ctenophores, cnidarians, and bilaterians all bear musculatures. However, the controversy concerning the phylogenetic position of ctenophores (Dunn et al., 2015) and the convergent evolution of muscles in cnidarians and bilaterians (Hejnol, 2012; Steinmetz et al., 2012) hamper to evaluate the significance of chancelloriid muscle at the current stage.
The complex integument of chancelloriids indicates that they might bear epithelia (Bengtson and Hou, 2001), a feature previously thought lacking in sponges. This has led to the idea that chancelloriids might represent a higher grade, epitheliazoans that is defined to exclude sponges (Janussen et al., 2002), an idea recently resurgent (Yun et al., 2021). However, it is clear that sponges do have epithelium (Leys et al., 2009; Vernale et al., 2021); thus, the epithelium-bearing grade would be equivalent to the eumetazoans that include sponges (Nielsen, 2019). The argument of chancelloriids being epitheliazoans grade, therefore, does not preclude the potential that they are sponge-related metazoans.
The biomineralization of chancelloriid sclerites is uniquely different in structure and composition from the sponge spicules (Bengtson et al., 1996; but see Botting and Muri, 2018 for a different view), which has led Bengtson and Collins (2015) state that ‘this discrepancy is at the heart of most of the controversy surrounding the nature chancelloriids.’ However, the emerging idea that early sponges might lack spicules (Yin et al., 2015; Andrade et al., 2021; Turner, 2021) and the biomineralization toolkit has a deep origin in pre-metazoans (Murdock, 2020) weakens this claim. In the biomineralization grade, which must have occurred several times in the life history (Knoll, 2003; Pérez-Huerta et al., 2018), differences would have little phylogenetic implications.
3.3 Some problematic fossils of diploblastic grade
Traditional views from embryology, development, and morphology treat the diploblastic animals, i.e., ctenophores and cnidarians, as an intermediate grade between sponges and bilaterians, which is supposed to have documented the transition from a radially organized ancestor to the LCAB. While the general principles remain standing, the detailed scenarios within this view have been fundamentally altered by new data from genomic phylogeny, development, and genetic controls on the evolution of body axes and peripheral tissues/organs. The uncertainty about the phylogenetic position of ctenophores poses the possibility that their complex features, such as striated muscle, and interconnected nerve nets, are probably produced by convergent evolution (Dunn et al., 2015), although these confront the parsimony criteria from the perspective of morphology (Nielsen, 2019). Intriguingly, updated comparative studies of embryology, including cell fate mapping and gene regulation, reveal that the gastrulation sites in cnidarian and bilaterians are different, thus negating the assumed homology between the oral-aboral axis of cnidarians and the A-P axis of bilaterians, and the homology of the mouth opening in these two groups (Martindale and Hejnol, 2009). This result is further reinforced by the expression pattern of Hox genes in cnidarian N. vectensis, which reveal that the staggered expression of Hox genes is unfolded along the directive axis rather than the oral-aboral axis of Nematostella vectensis (He et al., 2018; Technau and Genikhovich, 2018). In addition, there is evidence rejecting the homologies of the musculature (Hejnol, 2012; Steinmetz et al., 2012) and the condensed nervous system between cnidarian and bilaterians (Martindale and Hejnol, 2009). All these new data hints at a complicated transition from a radially organized ancestor to the LCAB.
Despite all these, something consensus can still be reached from this updated view of evo-devo for guiding the interpretation of problematic fossils. For example, the staggered expression of Hox genes in cnidarians indicates that their radially arranged tentacles, probably all other tissues/organs with the same circular repeating pattern, might represent the ancestral state before the body axis is reorganized in the stem groups towards the urbilaterian. And it is known that this repetitive pattern regulated by Hox can generate varying numbers, as seen in the segmentation number of arthropods, annelids, and chordates. With this new light, it would not be surprising to see a varying number of faces (6, 10, 14, etc.) in hexangulaconulariids (Guo et al., 2019; Guo et al., 2021), an extinct group of cnidarians. Other radially arranged tissues/organs of diploblastic animals might also be controlled by the same gene-regulating mechanism, such as the tentacles, canal systems, and mesenteries, etc. In other words, the genetic control of Hox genes on ‘radially’ arranged tissues/organs represents an innovation/grade in the evolution of non-bilaterian animals. This indicates that any problematic fossil with one axis and some radially arranged peripheral tissue/organs should be a member of this evolutionary grade.
Mackenziids are a rarely noticed problematic fossils with only two genera known by now, Mackenzia from the Burgess Shale (Walcott, 1911) and Paramakenzia (Figure 2B) from the Chengjiang biota (Zhao et al., 2022). They are atentaculate animals with an elongated cylindrical body and only an apical-abapical axis. In addition, mackenziids bear longitudinal strands that are radially arranged, along which small transverse tubular structures evenly occur (Zhao et al., 2022). These strands are interpreted as septal lamellae that bear hollow tubes connecting the internal cavity to the exterior, a canal system. Between the septal lamellae are tubular modules comparable with some Edicarian fossils, such as Ernietta. Interestingly, the septal lamellae seem to be able to extend into the stalk and merge into a fewer number (inset in Figure 2B). These radially arranged lamellae with a canal system represent a peripheral organ added to the apical-abapical axis. In this sense, makenziids can be confidently interpreted as diploblastic-grade animals with a more complex body plan than chancelloriids. While the differentiation of the main body and the stalk, particularly their different number of septal lamellae, represent an independently evolved body regionalization.
Another rarely mentioned example is C. striata from the Chengjiang biota (Figure 2C). The early description was only based on one poorly preserved specimen. A very recent description of 51 specimens reveals that Coniclua has a conical shell, a columnar body, and circumoral tentacles, all of which show perfect radial symmetry (Zhao et al., 2023). These radially arranged structures put a constrain on the phylogenetic position of Conicula as being a diploblastic animal. While any other alternative hypotheses on the affinity of Conicula, such as lophophorate (Luo et al., 1999) and deuterostomes (Caron et al., 2010), would require at least one bilateral feature to be confidently identified. Based on this step of determining the evolutionary grade, further character coding in the model of cnidarians is integrated into a phylogenetic analysis, which reveal that Conicula might potentially be a polyp form of ancestral medusozoans (Zhao et al., 2023). Through the procedure highlighted above, every interpretation step of Conicula becomes testable and repeatable.
Perhaps the most surprising outcome in the recent interpretation of the problematic Cambrian fossils is grouping Xianguangia with Dinomischus into a group of dinomischiids and recovering them in the stem group of ctenophores (Zhao et al., 2019). Xianguangia has long been viewed as an icon fossil of the Cambrian sea anemone (Hou et al., 2017) or stem cnidarian (Ou et al., 2017). While Dinomichus was pervasively conceived as a bilaterian (Conway Morris, 1977; Hou et al., 2017). Extensive investigation of dozens of specimens from the Chengjiang biota reveals that these two taxa, together with a newly erected Daihua sanqiong, share the same body plan. They all possess 18 radially arranged tentacles with pinnules and a calyx with radial septa (Zhao et al., 2019). Again, these two sets of radially arranged body structures constrain the affinity of dinomischiids within diploblastic grades. Further phylogenetic analysis supports dinomichiids lying in the stem towards the crown ctenophores (Zhao et al., 2019).
4 Problematic fossils of bilaterian grade–an example of Yunnanozoon
The establishment of bilateral symmetry, together with the differentiation of the mesoderm layer, lays the foundation for the diverse body plan in the bilaterian grades. It is within the three-dimensional space of the A-P, D-V, and left-right axes that manifold tissues/organs are organized in various patterns. In this sense, the bilateral symmetry, usually illustrated by the paired structures, represents the highest level of hierarchical grade in the diversification of bilaterians. While those bilaterian-specific organs and structures, such as eyes, various appendages, and segments, are at the lower level of hierarchical innovation but usually have deep (genetic) homologies back into the diploblastic grade or earlier (Davidson et al., 1995; Kimmel, 1996; Arendt and Wittbrodt, 2001; Erwin and Davidson, 2002; Oakley, 2003; Chipman, 2010; Marshall and Valentine, 2010; Chipman, 2018), with the latter scenario responsible for most convergent evolutions known in bilaterian groups. The diverse body plans within each of the three great clades of bilaterians (deuterostomes, spiralians, and ecdysozoans) are indeed produced partially by these lower-level hierarchical innovations that occurred in various combined ways. For example, there are no credible morphological features to define deuterostomes, spiralians, or ecdysozoans, thus it is quite difficult to structure evolutionary grades along their evolutionary path. The lack of recognizable evolutionary grades in the three great clades strongly impacts interpreting the problematic bilaterian fossils. For example, vetulicolians has been interpreted either as protostomes (Hou, 1987; Briggs et al., 2005; Caron, 2005; Aldridge et al., 2007; Lieberman, 2008) or deuterostomes (Shu et al., 1999; Shu et al., 2001; Lacalli, 2002; Shu, 2005; Ou et al., 2012; García-Bellido et al., 2014). Characters used to support these two hypotheses is as equivocal to each other, making it impossible to constrain vetulicolians either within protostome grade or deuterostome grade with current evidence.
Another pertinent example is the debates about yunnanozoans that are only known from the Chengjiang biota, south China. Historically, three species allied to two genera have been described. Recent work synonymized the three species into one taxon, Yunnanozoon. lividum (Cong et al., 2015). Yunnanozoon is a fusiform animal with seven anterior metameric filamentous arches covered by membrane sacs, a series of dorsal imbricating segments, an axial void region with stripes, a ventral coiled tube, and four pairs of dark circles located right before the coiled tube (Figures 3A, B). Over the last 30 years, debates on Yunnanozoon have been unimaginably divergent, with about ten proposed affinities have been proposed (Figure 3C), partially reflecting the difficulties in interpreting this fascinating animal. These include stem bilaterian (Dewel, 2000), protostome with uncertainty (Hou et al., 1991), gnathifera spiralian (Cong et al., 2015), ecdysozoans (Bergström, 2010), stem deuterostome (Shu et al., 2003), stem ambulacraria (Shu and Morris, 2003), hemichordate (Shu et al., 1996), stem chordate (Dzik, 1995), cephalochordate (Chen et al., 1995), and stem vertebrate (Chen et al., 1999).
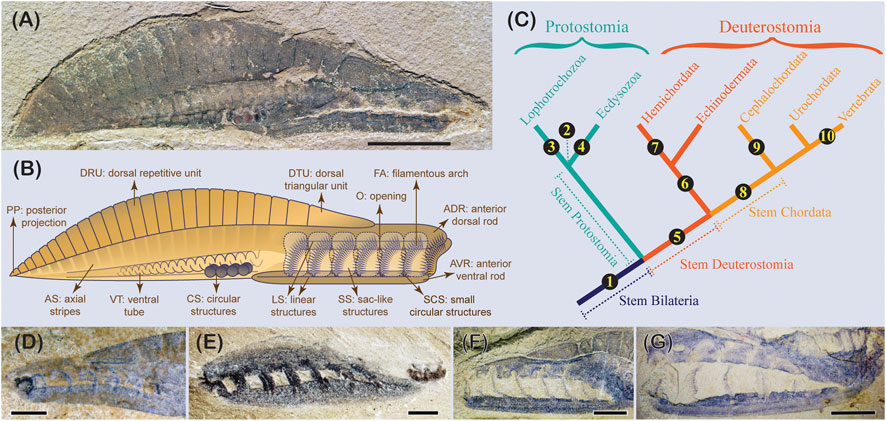
FIGURE 3. The early Cambrian problematic bilaterian Yunnanozoon lividum Hou et al., 1991. (A) One of the most complete specimens (YKLP 13005) with the sac membranes preserved exterior to the filamentous arches (invisible). (B) Lines and shapes of the unanimous morphological features of Y. lividum, with each feature given a neutral describing term. (C) Proposed affinities of Y. lividum across the animal tree. ① stem bilaterian, Dewel, 2000; ② protostome uncertain; Hou et al.,1991; ③ spiralians Cong et al., 2015; ④ ecdysozoans; Bergström 2010; ⑤ stem deuterostome Shu et al., 2003; ⑥ stem ambulacraria; Shu 2003; ⑦ hemichordate; Shu et al., 1996. ⑧ stem chordate; Dzik 1995; ⑨ cephalochordate; Chen et al., 1995; ⑩ stem vertebrate (craniate), Chen et al., 1999. (D)–(G) feeding structure of Y. lividum, showing the relative position of the ADR and AVR. (D) RCCBYU 10339, (E) YKLP 13058, (F) YKLP 13024, image flipped horizontally, (G) YKLP 13065. Scale bars: 1 cm (A); 2 mm (D), (E); 5 mm (F), (G).
The early dispute concerning Yunnanozoon was mainly caused by incongruence in identifying the preserved features. For example, the dorsal cuticularized repetitive units have been interpreted as myomeres in chordate hypotheses (Chen et al., 1995; Dzik, 1995; Chen et al., 1999) but rejected by others (Hou et al., 1991; Shu et al., 1996; Shu et al., 2003; Shu et al., 2010; Cong et al., 2015). Such inconsistencies in identifying characters are not rare in studying problematic fossils. Testing a reliable character identification would require eliminating taphonomic artifacts and employing neutral terms to describe the preserved features before identifying them as morphologic characters (Donoghue and Purnell, 2009; Cong et al., 2015). Indeed, a more radically testable manner has been applied to interpreting Yunnanozoon, which not only neutrally describes and tests all preserved features via comparatively scrutinizing all specimens available but even puts the interpretation of body orientation (such as anterior, posterior, dorsal, and ventral) in a testable model (Cong et al., 2015). This method has produced a number of unanimous morphologic characters present in Yunnanozoon and laid a testable foundation upon which to interpret the homologies of these characters and, ultimately, the affinity of this problematic fossil (Figure 3B).
Yunnanozoon is a bilaterian animal as manifested by the paired ventral circular structures and the paired filamentous arches, thus fitting well in the bilaterian grade (Cong et al., 2015). However, other features, such as the anterior filamentous arches, the dorsal repetitive units, and the axial stripes, are a matter of debate concerning their biological nature. In this sense, interpreting Yunanozoon is turned into a series of questions on homologizing these features within the bilaterian grade. It is worth noting that the homology of one character needs to be reconciled with that of all others. A primary comparison of the preserved features of Yunnanozoon reveals this is not an easy task (Cong et al., 2015). The cuticularized dorsal segments allude to an affinity within the protostomes, while the filamentous arches superficially resemble the gill arches of chordates although prominent differences exist in the sub-structural composition of these two arch systems. These difficulties in reconciling the potential homology of one character with another in Yunnanozoon might be a reflection that early bilaterians exploit the peripheral tissue/organs in more diverse pathways than those seen in the living clades.
The metameric arranged filamentous arches, together with the dorsal and ventral sclerotized rods they attached with, are the most contentious structural complex of Yunnanozoon. The inferred anterior location and the connection with the interpreted gut indicate this complex has a function of feeding. Confusingly, however, this structural complex is more commonly known to be easily detached from other body parts (Figure 3E) and preserved in aggregation. In addition, when preserved with their outer coverings, the membrane sacs, in articulated specimens, the filamentous arches seem more prone to decay (Figures 3F, G). All proposed affinities as chordates or other deuterostomes are based on the speculation that these filamentous arches, and the implied gill slits, are homologous with those of chordates or are the ancestral form of the early deuterostomes. There is no attempt to reconcile their difference (such as the presence of the dorsal and ventral sclerotized rods) with the gill arch/slit system of various deuterostomes. Not to mention to interpret the difference in the body plan between Yunnanozoon and deuterostomes.
A recent effort to depict the ultrastructure of the carbonaceous remains reveals, as claimed by the authors, the arches of Yunnanozoon ‘consist of cellular cartilage with an extracellular matrix dominated by microfibrils’ (Tian et al., 2022). While cheering on this nanometer-level of triumph, cautious tests are needed to validate this high-tech achievement in 518 Ma years of fossils and to appraise how this finding can overcome the difficulties in reconciling the homologies of various characters of Yunnanozoon as highlighted above.
5 Revisiting critical issues in interpreting problematic fossil–a case of Yunnanozoon
Before coming to the inquiries on the new findings in Yunnanozoon, it would be worth looking over the steps required in interpreting the problematic fossils. At first, taphonomic consideration needs to be conducted as soft-bodied fossils are more prone to decay. During the process, phylogenetic characters can be lost, or taphonomic artifacts can be introduced. Indeed, most early controversies about Yunnanozoon were caused by the taphonomic process, such as the identification of notochord, neural cord, myomeres, dorsal and ventral fins, heard, atrio/atriopore, and pharyngeal teeth in the chordate hypothesis (Chen et al., 1999), and the proboscis and collar in hemichordate hypothesis (Shu et al., 1996). Secondly, the criteria for choosing a comparing model need to be clarified. And lastly, the homological assessment of all available characters needs to be conducted to meet the three criteria, conjunction, similarity, and congruence, proposed by Patterson (1982) about 40 years ago.
The main finding of the high-tech application is the identified microfibrils that are supposed to occur in the extracellular matrix of the arch cartilage (Tian et al., 2022). Two questions need to be addressed in reading the data supporting this claim, the robustness of the data and the potential of nano-meter biomolecules to be preserved in their original arrangement. While the second question might be beyond the scope of interpretation, here I just address the first one. The morphological evidence corroborating the identification of carbonaceous microfibrils mainly comes from two types of images, those collected with the Scanning Electronic Microscope (SEM) and those with the Transmission Electronic Microscope (TEM). It is not clarified in the methodology section whether the specimens were coated before scanning (Material and Methods in Tian et al., 2022). The common appearance of smooth patches, though, indicates that coating has been applied (Figures 3D, F; fig. s3c-f, i, l, m therein). The grayscale in these images shows that the bundled microfibers can be very bright, similar to the inferred covering coating or to the fabric iron minerals at their broken profile (Figure 3F therein). Such a high grayscale is contradicted by the interpretation that these bundled microfibers are carbonaceous microfibrils, which would be expected with a relatively lower grayscale. Future work should focus on testing the carbonaceous nature of these microfibers and whether they are present in other parts of the fossils or even in the rock matrix. A point analysis of EDX is not adequate to argue that the whole structure is carbonaceous, as the signals collected by EDX at one point is normally expanded in the order of magnitude to its surrounding region.
The parameters of TEM images also need to be clarified. It is not even known whether the illustrated images (Figures 3G, H in Tian et al., 2022) are produced in the darkfield or the brightfield mode. This is important because the brightness in these two different imaging modes means different things. In the brightfield mode, the brightness, or the greyscale, of a TEM image indicates the degree of crystallinity, while in the darkfield mode, the higher greyscale normally marks heavier molecular/atom weight, such as metals. It is worth noting that, during the preparation of TEM samples and the ion cleaning before taking images, contamination by the metal ions is common. These contaminated ions normally have brighter grayscale and are enriched in the topographically higher region. Future work should aim to test whether the brighter spots illustrated in the TEM images of Tian et al. (2022) are carbonaceous remains. Furthermore, in situ preparation of TEM samples are also a plausible practice to check the original composition of the carbonaceous remains in the filamentous arches of Yunnanozoon.
Even if the interpreted microfibrils are confirmed, a more thorough assessment of the homology of the filamentous arches needs to be implemented. The interpreted dorsal and ventral horizontal rods in Tian et al. (2022) have been neutrally described as linear structures running along the anterior dorsal and ventral sclerotized rods (differ from ‘rods’ in Tian et al., 2022) showing a degree of stiffness (Figures 3B, D–G) (Cong et al., 2015). These linear structures are in pairs in both dorsal and ventral sclerotized rods, but there is no evidence to show that the dorsal and ventral linear structures are connected at the rear end of the feeding region. In lateral view, the width of these linear structures can vary, indicating that they might be a dorsal-ventrally extended structure. The depression of the space between the paired linear structures indicates a trench exists between them, further corroborating their dorsal-ventrally extended nature. The attaching points of the filamentous arches located in this trench are normally preserved as concave or convex small circular structures. It is these three-dimensional small circular structures exerting the linear structures a pattern of undulation.
The biggest challenge in homologizing the filamentous arches with the gill arches of chordates is the presence of dorsal and ventral sclerotized rods, which functionally not only support the filamentous arches but also probably drive their movement. Comparison across specimens indicates that the dorsal and ventral rods can move relative to each other, making the whole feeding complex function like a ‘jawed’ mouth (Figures 3D–G). Another challenge in this homologizing practice is the sac-shaped membrane coverings that are exterior to the filamentous arches. They are very easy to detach from the sclerotized rods (Figures 3F, G). With current evidence, the claimed finding of the bundled microfibrils cannot overcome all these barriers, thus even do not meet the criterium of similarity (ontogeny, topography and composition), one of the three testable rules in assessing homology (Patterson, 1982).
6 Remarks
Although several ideas proposed by Stephen J. Gould, such as ‘weird wonders’ and the decreasing disparity through Phanerozoic, have been losing their standing, his metaphor about early animal evolution (and life evolution in general) remains telling (Gould, 1989). If we take the animals’ origin and their early radiation as one of the ‘speeches’ of life evolution, this “speech” (and all others) is only recorded on a ruined tape with some fragments (strata and fossils). Fossils represent the remains of the basic parts (words as components) of each sentence (evolutionary scenario) of the “speech,” which are the only components we can use for grammar parsing. We will never be able to rewind, erase and replay the tape again. But we can manage to recover what has been spoken in the tape fragments we are left with. In this sense, interpreting the Ediacaran-Cambrian fossils is more like trying to repeat (retell indeed, in almost all cases) the sentence they constituted in the tape in the most feasible way by drawing the parse tree of that sentence. The problematic fossils might be the most difficult part during paring each sentence of the “speech” by the Cambrian Explosion. However, the unremitting work in fossil discoveries, comparative studies of genic and genomic data, and molecular phylogenies together are bringing forth a more accurate framework to do this parsing. A repeatable and testable method for interpreting problematic fossils has been under development. The application of this method in a wide variety of problematic fossils promises that most interpretations can be fairly evaluated. With this developing method, we are parsing each sentence spoken by the Cambrian animals during their earliest radiation event.
Author contributions
PC conceived and conducted the study, prepared all figures, and wrote the manuscript. The author agrees to be accountable for the content of the work. The author confirms being the sole contributor of this work and has approved it for publication.
Funding
This work is supported by the National Natural Science Foundation of China (42072019), the Yunling Scholar Project, the Joint Project of the YUN-Local Government (202201BF070001-016) and the Strategic Priority Research Program of CAS (XDB26000000).
Acknowledgments
I thank Bruce S. Lieberman for the invitation to submit this review, and two reviewers for their insightful comments. The idea of this work emerged when I presented a talk on a similar topic at the inaugural meeting (2018) of the MEC International Joint Laboratory for Palaeobiology and Palaeoenvironment, Yunnan University. Thanks are given to all my international colleagues and to my students who have been dedicating their talent to unravel the mystery of the Chengjiang problematic fossils. This work benefits from discussions and collaborations with them.
Conflict of interest
The author declares that the research was conducted in the absence of any commercial or financial relationships that could be construed as a potential conflict of interest.
The handling editor BL declared a past co-authorship with the author PC.
Publisher’s note
All claims expressed in this article are solely those of the authors and do not necessarily represent those of their affiliated organizations, or those of the publisher, the editors and the reviewers. Any product that may be evaluated in this article, or claim that may be made by its manufacturer, is not guaranteed or endorsed by the publisher.
References
Aguinaldo, A. M. A., Turbeville, J. M., Linford, L. S., Rivera, M. C., Garey, J. R., Raff, R. A., et al. (1997). Evidence for a clade of nematodes, arthropods and other moulting animals. Nature 387, 489–493. doi:10.1038/387489a0
Aldridge, R. J., Briggs, D. E. G., Sansom, I. J., and Smith, M. P. (1994). The latest vertebrates are the earliest. Geol. Today 10, 141–145. doi:10.1111/j.1365-2451.1994.tb00891.x
Aldridge, R. J., Hou, X., Siveter, D. J., Siveter, D. J., and Gabbott, S. E. (2007). The systematics and phylogenetic relationships of vetulicolians. Palaeontology 50, 131–168. doi:10.1111/j.1475-4983.2006.00606.x
Allison, P. A. (1986). Soft-bodied animals in the fossil record: The role of decay in fragmentation during transport. Geology 14, 979–981. doi:10.1130/0091-7613(1986)14<979:saitfr>2.0.co;2
Andrade, M. P., Santos, D., Bueno, G. M., and Santos, C. M. D. (2021). What if sponges originated 890 million years ago? On the emergence of some precursors of animal sentience. Evol. Biol. 48, 404–406. doi:10.1007/s11692-021-09551-z
Arendt, D., and Wittbrodt, J. (2001). Reconstructing the eyes of urbilateria. Philos. Trans. R. Soc. Lond Ser. B Biol. Sci. 356, 1545–1563. doi:10.1098/rstb.2001.0971
Beall, B. S. (1991). “The Tully Monster and a new approach to analyzing problematica,” in The early evolution of Metazoa and the significance of problematic taxa. Editors A. M. Simonetta, and S. Conway Morris (Cambridge, UK: Cambridge University Press).
Bengtson, S., and Collins, D. (2015). Chancelloriids of the cambrian burgess shale. Palaeontol. Electron 18, 1–67. doi:10.26879/498
Bengtson, S., Collins, D. H., and Runnegar, B. (1996). Chancelloriid sclerite formation – turning the problem inside-out. Paleontol. Soc. Spec. Publ. 8, 29. doi:10.1017/s2475262200000319
Bengtson, S., and Hou, X. (2001). The integument of Cambrian chancelloriids. Acta Palaeontol. Pol. 46, 1–22.
Bergström, J. (2010). “The earliest arthropods and other animals,” in Darwin’s heritage today: Proceedings of the Darwin 200 beijing international conference. Editors M. Long, H. Gu, and Z. Zhou (Beijing, China: Higher Education Press), 28–43.
Botting, J. P., and Muri, L. A. (2018). Early sponge evolution: A review and phylogenetic framework. Palaeoworld 27, 1–29. doi:10.1016/j.palwor.2017.07.001
Briggs, D. E. G., and Fortey, R. A. (2005). Wonderful strife: Systematics, stem groups, and the phylogenetic signal of the cambrian radiation. Paleobiology 31, 94–112. doi:10.1666/0094-8373(2005)031[0094:wsssga]2.0.co;2
Briggs, D. E. G., Lieberman, B. S., Halgedahl, S. L., and Jarrard, R. D. (2005). A new metazoan from the Middle Cambrian of Utah and the nature of the Vetulicolia. Palaeontology 48, 681–686. doi:10.1111/j.1475-4983.2005.00489.x
Briggs, D. E. G. (2015). The Cambrian explosion. Curr. Biol. 25, R864–R868. doi:10.1016/j.cub.2015.04.047
Briggs, D. E. G. (2003). The role of decay and mineralization in the preservation of soft-bodied fossils. Annu. Rev. Earth Pl. S. C. 31, 275–301. doi:10.1146/annurev.earth.31.100901.144746
Briggs, D. E. G., and Williams, S. H. (1981). The restoration of flattened fossils. Lethaia 14, 157–164. doi:10.1111/j.1502-3931.1981.tb01918.x
Brunet, T., and King, N. (2017). The Origin of animal multicellularity and cell differentiation. Dev. Cell 43, 124–140. doi:10.1016/j.devcel.2017.09.016
Budd, G. E. (2013). At the origin of animals: The revolutionary Cambrian fossil record. Curr. Genomics 14, 344–354. doi:10.2174/13892029113149990011
Budd, G. E., and Jensen, S. (2000). A critical reappraisal of the fossil record of the bilaterian phyla. Biol. Rev. 75, 253–295. doi:10.1111/j.1469-185x.1999.tb00046.x
Budd, G. E., and Telford, M. J. (2009). The origin and evolution of arthropods. Nature 457, 812–817. doi:10.1038/nature07890
Butterfield, N. J. (2003). Exceptional fossil preservation and the Cambrian explosion. Integr. Comp. Biol. 43, 166–177. doi:10.1093/icb/43.1.166
Caron, J.-B. (2005). Banffia constricta, a putative vetulicolid from the middle cambrian burgess shale. Trans. R. Soc. Edinb Earth Sci. 96, 95–111. doi:10.1017/s0263593300001255
Caron, J.-B., Morris, S. C., and Shu, D. (2010). Tentaculate fossils from the cambrian of Canada (British columbia) and China (yunnan) interpreted as primitive deuterostomes. Plos One 5, e9586. doi:10.1371/journal.pone.0009586
Chen, J., Huang, D.-Y., and Bottjer, D. J. (2005). An Early Cambrian problematic fossil: Vetustovermis and its possible affinities. Proc. Biol. Sci. 272, 2003–2007. doi:10.1098/rspb.2005.3159
Chen, J., Huang, D., and Li, C.-W. (1999). An early Cambrian craniate-like chordate. Nature 402, 518–522. doi:10.1038/990080
Chen, J. Y., Dzik, J., Edgecombe, G. D., Ramsköld, L., and Zhou, G. Q. (1995). A possible Early Cambrian chordate. Nature 377, 720–722. doi:10.1038/377720a0
Chen, Z., Zhou, C., Yuan, X., and Xiao, S. (2019). Death march of a segmented and trilobate bilaterian elucidates early animal evolution. Nature 573, 412–415. doi:10.1038/s41586-019-1522-7
Chipman, A. D. (2010). Parallel evolution of segmentation by co-option of ancestral gene regulatory networks. Bioessays 32, 60–70. doi:10.1002/bies.200900130
Chipman, A. D. (2018). “The evolution and development of segmented body plans,” in Evolutionary developmental biology. Editors L. Nuno de la Rosa, and G. Müller (Berlin, Germany: Springer).
Cong, P.-Y., Harvey, T. H. P., Williams, M., Siveter, D. J., Siveter, D. J., Gabbott, S. E., et al. (2018). Naked chancelloriids from the lower Cambrian of China show evidence for sponge-type growth. Proc. R. Soc. B 285, 20180296. doi:10.1098/rspb.2018.0296
Cong, P.-Y., Hou, X.-G., Aldridge, R. J., Purnell, M. A., and Li, Y.-Z. (2015). New data on the palaeobiology of the enigmatic yunnanozoans from the Chengjiang biota, lower cambrian, China. Palaeontology 58, 45–70. doi:10.1111/pala.12117
Conway Morris, S. (1977). A new entoproct-like organism from the Burgess Shale of British Columbia. Palaeontology 20, 833–845.
Costa-Paiva, E. M., Mello, B., Bezerra, B. S., Coates, C. J., Halanych, K. M., Brown, F., et al. (2022). Molecular dating of the blood pigment hemocyanin provides new insight into the origin of animals. Geobiology 20, 333–345. doi:10.1111/gbi.12481
Daley, A. C., Budd, G. E., Caron, J.-B., Edgecombe, G. D., and Collins, D. (2009). The Burgess Shale anomalocaridid Hurdia and its significance for early euarthropod evolution. Science 323, 1597–1600. doi:10.1126/science.1169514
Darroch, S. A. F., Smith, E. F., Laflamme, M., and Erwin, D. H. (2018). Ediacaran extinction and cambrian explosion. Trends Ecol. Evol. 33, 653–663. doi:10.1016/j.tree.2018.06.003
Davidson, E. H., and Erwin, D. H. (2006). Gene Regulatory Networks and the evolution of animal body plans. Science 311, 796–800. doi:10.1126/science.1113832
Davidson, E. H., Peterson, K. J., and Cameron, R. A. (1995). Origin of bilaterian body plans: Evolution of developmental regulatory mechanisms. Science 270, 1319–1325. doi:10.1126/science.270.5240.1319
Dewel, R. A. (2000). Colonial origin for eumetazoa: Major morphological transitions and the origin of bilaterian complexity. J. Morphol. 243, 35–74. doi:10.1002/(sici)1097-4687(200001)243:1<35::aid-jmor3>3.0.co;2-#
Donoghue, P. C. J., Forey, P. L., and Aldridge, R. J. (2000). Conodont affinity and chordate phylogeny. Biol. Rev. 75, 191–251. doi:10.1017/s0006323199005472
Donoghue, P. C. J., and Purnell, M. A. (2009). Distinguishing heat from light in debate over controversial fossils. Bioessays 31, 178–189. doi:10.1002/bies.200800128
Donoghue, P. C. J. (2005). Saving the stem group—A contradiction in terms? Paleobiology 31, 553–558. doi:10.1666/0094-8373(2005)031[0553:stsgci]2.0.co;2
Droser, M. L., and Gehling, J. G. (2015). The advent of animals: The view from the Ediacaran. Proc. Natl. Acad. Sci. 112, 4865–4870. doi:10.1073/pnas.1403669112
Dunn, C. W., Giribet, G., Edgecombe, G. D., and Hejnol, A. (2014). Animal phylogeny and its evolutionary implications. Annu. Rev. Ecol. Evol. Syst. 45, 371–395. doi:10.1146/annurev-ecolsys-120213-091627
Dunn, C. W., Leys, S. P., and Haddock, S. H. D. (2015). The hidden biology of sponges and ctenophores. Trends Ecol. Evol. 30, 282–291. doi:10.1016/j.tree.2015.03.003
Dunn, F. S., Kenchington, C. G., Parry, L. A., Clark, J. W., Kendall, R. S., and Wilby, P. R. (2022). A crown-group cnidarian from the ediacaran of charnwood forest, UK. Nat. Ecol. Evol. 6, 1095–1104. doi:10.1038/s41559-022-01807-x
Dunn, F. S., Liu, A. G., and Donoghue, P. C. J. (2017). Ediacaran developmental biology. Biol. Rev. 93, 914–932. doi:10.1111/brv.12379
Dunn, F. S., and Liu, A. G. (2019). Viewing the Ediacaran biota as a failed experiment is unhelpful. Nat. Ecol. Evol. 3, 512–514. doi:10.1038/s41559-019-0815-4
Edgecombe, G. D. (2020). Arthropod origins: Integrating paleontological and molecular evidence. Annu. Rev. Ecol. Evol. Syst. 51, 1–25. doi:10.1146/annurev-ecolsys-011720-124437
Edgecombe, G. D., and Legg, D. A. (2014). Origins and early evolution of arthropods. Palaeontology 57, 457–468. doi:10.1111/pala.12105
Edgecombe, G., Giribet, G., Dunn, C., Hejnol, A., Kristensen, R., Neves, R., et al. (2011). Higher-level metazoan relationships: Recent progress and remaining questions. Org. Divers Evol. 11, 151–172. doi:10.1007/s13127-011-0044-4
Erwin, D. H. (2021). A conceptual framework of evolutionary novelty and innovation. Biol. Rev. 96, 1–15. doi:10.1111/brv.12643
Erwin, D. H., and Davidson, E. H. (2009). The evolution of hierarchical gene regulatory networks. Nat. Rev. Genet. 10, 141–148. doi:10.1038/nrg2499
Erwin, D. H., and Davidson, E. H. (2002). The last common bilaterian ancestor. Development 129, 3021–3032. doi:10.1242/dev.129.13.3021
Erwin, D. H. (2009). Early origin of the bilaterian developmental toolkit. Philos. Trans. R. Soc. B Biol. Sci. 364, 2253–2261. doi:10.1098/rstb.2009.0038
Erwin, D. H., Laflamme, M., Tweedt, S. M., Sperling, E. A., Pisani, D., and Peterson, K. J. (2011). The cambrian conundrum: Early divergence and later ecological success in the early history of animals. Science 334, 1091–1097. doi:10.1126/science.1206375
Erwin, D. H. (2015). Novelty and innovation in the history of life. Curr. Biol. 25, R930–R940. doi:10.1016/j.cub.2015.08.019
Evans, S. D., Droser, M. L., and Erwin, D. H. (2021). Developmental processes in Ediacara macrofossils. Proc. Biol. Sci. 288, 20203055. doi:10.1098/rspb.2020.3055
Evans, S. D., Hughes, I. V., Gehling, J. G., and Droser, M. L. (2020). Discovery of the oldest bilaterian from the ediacaran of south Australia. P Natl. Acad. Sci. U. S. A. 117, 7845–7850. doi:10.1073/pnas.2001045117
García-Bellido, D. C., Lee, M. S. Y., Edgecombe, G. D., Jago, J. B., Gehling, J. G., and Paterson, J. R. (2014). A new vetulicolian from Australia and its bearing on the chordate affinities of an enigmatic Cambrian group. Bmc Evol. Biol. 14, 214. doi:10.1186/s12862-014-0214-z
Genikhovich, G., and Technau, U. (2017). On the evolution of bilaterality. Development 144, 3392–3404. doi:10.1242/dev.141507
Giribet, G., and Edgecombe, G. D. (2017). Current understanding of Ecdysozoa and its internal phylogenetic relationships. Integr. Comp. Biol. 57, 455–466. doi:10.1093/icb/icx072
Giribet, G. (2016). Genomics and the animal tree of life: Conflicts and future prospects. Zool. Scr. 45, 14–21. doi:10.1111/zsc.12215
Gould, S. J. (1989). Wonderful life, the Burgess Shale and the nature of history. New York: W.W. Norton and Company.
Guo, J., Han, J., Iten, H. V., Song, Z., Qiang, Y., Wang, W., et al. (2021). A ten-faced hexangulaconulariid from cambrian stage 2 of south China. J. Paleontol. 95, 957–964. doi:10.1017/jpa.2021.25
Guo, J., Han, J., Iten, H. V., Wang, X., Qiang, Y., Song, Z., et al. (2019). A fourteen-faced hexangulaconulariid from the early cambrian (stage 2) yanjiahe formation, south China. J. Paleontol. 1–11, 45–55. doi:10.1017/jpa.2019.56
Guo, J., Parry, L. A., Vinther, J., Edgecombe, G. D., Wei, F., Zhao, J., et al. (2022). A Cambrian tommotiid preserving soft tissues reveals the metameric ancestry of lophophorates. Curr. Biol. 32, 4769–4778.e2. doi:10.1016/j.cub.2022.09.011
He, J., and Deem, M. W. (2010). Hierarchical evolution of animal body plans. Dev. Biol. 337, 157–161. doi:10.1016/j.ydbio.2009.09.038
He, S., Viso, F., Chen, C.-Y., Ikmi, A., Kroesen, A. E., and Gibson, M. C. (2018). An axial Hox code controls tissue segmentation and body patterning in Nematostella vectensis. Science 361, 1377–1380. doi:10.1126/science.aar8384
Heger, P., Zheng, W., Rottmann, A., Panfilio, K. A., and Wiehe, T. (2020). The genetic factors of bilaterian evolution. Elife 9, e45530. doi:10.7554/elife.45530
Hoffman, A., and Nitecki, M. H. (1986). Problematic fossil taxa. Oxford, UK: Oxford University Press.
Hou, X.-G., Aldridge, R., Aldridge, R., Aldridge, R. J., Bergstrom, J., Cong, P.-Y., et al. (2017). The cambrian fossils of Chengjiang. China: John Wiley & Sons, Ltd.
Hou, X. (1987). Early cambrian large bivalved arthropods from Chengjiang, eastern yunnan. Acta Palaeotologica Sin. 26, 286–292.
Hou, X., Ramsköld, L., and Bergström, J. (1991). Composition and preservation of the Chengjiang fauna: A lower cambrian soft-bodied biota. Zool. Scr. 20, 395–411.
Huxley, J. (1959). “Clades and grades,” in Function and taxonomic importance. Editor A. J. Cain (London, UK: Systematics Association).
Janussen, D., Steiner, M., and Zhu, M. (2002). New well-preserved scleritomes of chancelloridae from the early Cambrian Yuanshan Formation (Chengjiang, China) and the middle Cambrian Wheeler shale (Utah, USA) and paleobiological implications. J. Paleontol. 76, 596–606. doi:10.1017/s0022336000041895
Jefferies, R. P. S. (1979). “The origin of chordates: A methodological essay,” in The origin of major invertebrate groups. Editor M. R. House (London, UK: Academic Press), 443–447.
Jenner, R. A., and Littlewood, D. T. J. (2008). Problematica old and new. Philos. Trans. R. Soc. B Biol. Sci. 363, 1503–1512. doi:10.1098/rstb.2007.2240
Jenner, R. A., and Schram, F. R. (1999). The grand game of metazoan phylogeny: Rules and strategies. Biol. Rev. 74, 121–142. doi:10.1111/j.1469-185x.1999.tb00183.x
Kapli, P., Natsidis, P., Leite, D. J., Fursman, M., Jeffrie, N., Rahman, I. A., et al. (2021). Lack of support for Deuterostomia prompts reinterpretation of the first Bilateria. Sci. Adv. 7, eabe2741. doi:10.1126/sciadv.abe2741
Kimmel, C. B. (1996). Was urbilateria segmented? Trends Genet. 12, 329–331. doi:10.1016/s0168-9525(96)80001-1
King, N., and Rokas, A. (2017). Embracing uncertainty in reconstructing early animal evolution. Curr. Biol. 27, R1081–R1088. doi:10.1016/j.cub.2017.08.054
Knoll, A. H. (2003). Biomineralization and evolutionary history. Rev. Mineral. Geochem 54, 329–356. doi:10.2113/0540329
Knoll, A. H., and Carroll, S. B. (1999). Early animal evolution: Emerging views from comparative biology and geology. Science 284, 2129–2137. doi:10.1126/science.284.5423.2129
Kocot, K. M. (2016). On 20 years of lophotrochozoa. Org. Divers Evol. 16, 329–343. doi:10.1007/s13127-015-0261-3
Kraus, Y., Aman, A., Genikhovich, G., and Technau, U. (2016). Pre-bilaterian origin of the blastoporal axial organizer. Nat. Commun. 7, 11694. doi:10.1038/ncomms11694
Lacalli, T. C. (2002). Vetulicolians—are they deuterostomes? Chordates? Bioessays 24, 208–211. doi:10.1002/bies.10064
Laumer, C. E., Fernández, R., Lemer, S., Combosch, D., Kocot, K. M., Riesgo, A., et al. (2019). Revisiting metazoan phylogeny with genomic sampling of all phyla. Proc. Biol. Sci. 286, 20190831. doi:10.1098/rspb.2019.0831
Leininger, S., Adamski, M., Bergum, B., Guder, C., Liu, J., Laplante, M., et al. (2014). Developmental gene expression provides clues to relationships between sponge and eumetazoan body plans. Nat. Commun. 5, 3905. doi:10.1038/ncomms4905
Leys, S. P., Nichols, S. A., and Adams, E. D. M. (2009). Epithelia and integration in sponges. Integr. Comp. Biol. 49, 167–177. doi:10.1093/icb/icp038
Li, Y., Dunn, F. S., Murdock, D. J. E., Guo, J., Rahman, I. A., and Cong, P. (2023). Cambrian stem-group ambulacraraians and the nature of the ancestral duterostome. Curr. Biol. 2023, 1–10.
Lieberman, B. S. (2008). The Cambrian radiation of bilaterians: Evolutionary origins and palaeontological emergence; Earth history change and biotic factors. Palaeogeogr. Palaeoclim. Palaeoecol. 258, 180–188. doi:10.1016/j.palaeo.2007.05.021
Lozano-Fernandez, J., Reis, M., Donoghue, P. C. J., and Pisani, D. (2017). RelTime rates collapse to a strict clock when estimating the timeline of animal diversification. Genome Biol. Evol. 9, 1320–1328. doi:10.1093/gbe/evx079
Luo, H., Hu, S., Chen, L., Zhang, S., and Tao, Y. (1999). Early cambrian Chengjiang fauna from kunming region. China: Yunnan Science and Technology Press, 129.
Mallatt, J., Chen, J., and Holland, N. D. (2003). Comment on “A new species of yunnanozoan with implications for deuterostome evolution”. Science 300, 1372c. doi:10.1126/science.1085064
Marlétaz, F., Peijnenburg, K. T. C. A., Goto, T., Satoh, N., and Rokhsar, D. S. (2019). A new spiralian phylogeny places the enigmatic arrow worms among Gnathiferans. Curr. Biol. 29, 312–318.e3. doi:10.1016/j.cub.2018.11.042
Marshall, C. R. (2006). Explaining the Cambrian “explosion” of animals. Annu. Rev. Earth Pl. S. C. 34, 355–384. doi:10.1146/annurev.earth.33.031504.103001
Marshall, C. R., and Valentine, J. W. (2010). The importance of preadapted genomes in the origin of the animal bodyplans and the Cambrian explosion. Evolution 64, 1189–1201. doi:10.1111/j.1558-5646.2009.00908.x
Martindale, M. Q., and Hejnol, A. (2009). A developmental perspective: Changes in the position of the blastopore during bilaterian evolution. Dev. Cell 17, 162–174. doi:10.1016/j.devcel.2009.07.024
Martindale, M. Q. (2005). The evolution of metazoan axial properties. Nat. Rev. Genet. 6, 917–927. doi:10.1038/nrg1725
Mazurek, D., and ZatoŃ, M. (2011). Is Nectocaris pteryx a cephalopod? Lethaia 44, 2–4. doi:10.1111/j.1502-3931.2010.00253.x
Mills, D. B., Francis, W. R., and Canfield, D. E. (2018). Animal origins and the tonian earth system. Emerg. Top. Life Sci. 2, 289–298. doi:10.1042/etls20170160
Murdock, D. J. E. (2020). The ‘biomineralization toolkit’ and the origin of animal skeletons. Biol. Rev. 95, 1372–1392. doi:10.1111/brv.12614
Nanglu, K., Cole, S. R., Wright, D. F., and Souto, C. (2023). Worms and gills, plates and spines: The evolutionary origins and incredible disparity of deuterostomes revealed by fossils, genes, and development. Biol. Rev. 98, 316–351. doi:10.1111/brv.12908
Nielsen, C. (2019). Early animal evolution: A morphologist’s view. Roy. Soc. Open Sci. 6, 190638. doi:10.1098/rsos.190638
Nielsen, C., and Parker, A. (2010). Morphological novelties detonated the Ediacaran-Cambrian “explosion”. Evol. Dev. 12, 345–346. doi:10.1111/j.1525-142x.2010.00420.x
Nielsen, C. (2008). Six major steps in animal evolution: Are we derived sponge larvae? Evol. Dev. 10, 241–257. doi:10.1111/j.1525-142x.2008.00231.x
Oakley, T. H. (2003). The eye as a replicating and diverging, modular developmental unit. Trends Ecol. Evol. 18, 623–627. doi:10.1016/j.tree.2003.09.005
Ou, Q., Han, J., Zhang, Z., Shu, D., Sun, G., and Mayer, G. (2017). Three Cambrian fossils assembled into an extinct body plan of cnidarian affinity. Proc. Natl. Acad. Sci. 114, 8835–8840. doi:10.1073/pnas.1701650114
Ou, Q., Morris, S. C., Han, J., Zhang, Z., Liu, J., Chen, A., et al. (2012). Evidence for gill slits and a pharynx in Cambrian vetulicolians: Implications for the early evolution of deuterostomes. Bmc Biol. 10, 81. doi:10.1186/1741-7007-10-81
Patterson, C. (1982). “Morphological characters and homology,” in Problems of phylogenetic reconstruction, the Systematic Association special volume No 21. Editors K. A. Joysey, and A. E. Friday (London UK: Academic Press).
Pérez-Huerta, A., Coronado, I., and Hegna, T. A. (2018). Understanding biomineralization in the fossil record. Earth-sci Rev. 179, 95–122. doi:10.1016/j.earscirev.2018.02.015
Peterson, K. J., Cotton, J. A., Gehling, J. G., and Pisani, D. (2008). The ediacaran emergence of bilaterians: Congruence between the genetic and the geological fossil records. Philos. Trans. R. Soc. B Biol. Sci. 363, 1435–1443. doi:10.1098/rstb.2007.2233
Peterson, K. J., and Eernisse, D. J. (2016). The phylogeny, evolutionary developmental biology, and paleobiology of the deuterostomia: 25 years of new techniques, new discoveries, and new ideas. Org. Divers Evol. 16, 401–418. doi:10.1007/s13127-016-0270-x
Philippe, H., Poustka, A. J., Chiodin, M., Hoff, K. J., Dessimoz, C., Tomiczek, B., et al. (2019). Mitigating anticipated effects of systematic errors supports sister-group relationship between Xenacoelomorpha and Ambulacraria. Curr. Biol. 29, 1818–1826.e6. doi:10.1016/j.cub.2019.04.009
Pontefract, A., and Stone, J. (2007). “Gradual origin for the metazoans,” in Planetary systems and the origins of life. Editors R. Pudritz, P. Higgs, and J. Stone (Cambridge, UK: Cambridge University Press), 210–222.
Pridmore, P. A., Barwick, R. E., and Nicoll, R. S. (1996). Soft anatomy and the affinities of conodonts. Lethaia 29, 317–328. doi:10.1111/j.1502-3931.1996.tb01667.x
Reis, M., Thawornwattana, Y., Angelis, K., Telford, M. J., Donoghue, P. C. J., and Yang, Z. (2015). Uncertainty in the timing of origin of animals and the limits of precision in molecular timescales. Curr. Biol. 25, 2939–2950. doi:10.1016/j.cub.2015.09.066
Richter, D. J., and King, N. (2013). The genomic and cellular foundations of animal origins. Annu. Rev. Genet. 47, 509–537. doi:10.1146/annurev-genet-111212-133456
Rokas, A. (2008). The origins of multicellularity and the early history of the genetic Toolkit for animal development. Annu. Rev. Genet. 42, 235–251. doi:10.1146/annurev.genet.42.110807.091513
Sansom, I. J., Smith, M. P., Armstrong, H. A., and Smith, M. M. (1992). Presence of the earliest vertebrate hard tissue in conodonts. Science 256, 1308–1311. doi:10.1126/science.1598573
Schiffbauer, J. D., Selly, T., Jacquet, S. M., Merz, R. A., Nelson, L. L., Strange, M. A., et al. (2020). Discovery of bilaterian-type through-guts in cloudinomorphs from the terminal Ediacaran Period. Nat. Commun. 11, 205. doi:10.1038/s41467-019-13882-z
Sebé-Pedrós, A., Degnan, B. M., and Ruiz-Trillo, I. (2017). The origin of metazoa: A unicellular perspective. Nat. Rev. Genet. 18, 498–512. doi:10.1038/nrg.2017.21
Seilacher, A. (2007). “Evolutionary innovation versus ecological incumbency,” in Planetary systems and the origins of life. Editors R. Pudritz, P. Higgs, and J. Stone (Cambridge, UK: Cambridge University Press), 193–209.
Seilacher, A., Grazhdankin, D., and Legouta, A. (2003). Ediacaran biota: The dawn of animal life in the shadow of giant protists. Paleontol. Res. 7, 43–54. doi:10.2517/prpsj.7.43
Shu, D. (2003). A paleontological perspective of vertebrate origin. Chin. Sci. Bull. 48, 725–735. doi:10.1007/bf03187041
Shu, D. G., Morris, S. C., Han, J., Chen, L., Zhang, X. L., Zhang, Z. F., et al. (2001). Primitive deuterostomes from the Chengjiang lagerstätte (lower cambrian, China). Nature 414, 419–424. doi:10.1038/35106514
Shu, D. G., Morris, S. C., Zhang, Z. F., and Han, J. (2010). The earliest history of the deuterostomes: The importance of the Chengjiang fossil-lagerstätte. Proc. R. Soc. B Biol. Sci. 277, 165–174. doi:10.1098/rspb.2009.0646
Shu, D., and Morris, S. C. (2003). Response to Comment on “A new species of yunnanozoan with implications for deuterostome evolution. Science 300, 1372. doi:10.1126/science.1085573
Shu, D., Morris, S. C., Zhang, X. L., Chen, L., Li, Y., and Han, J. (1999). A pipiscid-like fossil from the Lower Cambrian of south China. Nature 400, 746–749. doi:10.1038/23445
Shu, D., Morris, S. C., Zhang, Z. F., Liu, J. N., Han, J., Chen, L., et al. (2003). A new species of yunnanozoan with implications for deuterostome evolution. Science 299, 1380–1384. doi:10.1126/science.1079846
Shu, D., Zhang, X., and Chen, L. (1996). Reinterpretation of Yunnanozoonas the earliest known hemichordate. Nature 380, 428–430. doi:10.1038/380428a0
Simonetta, A. M., and Conway Morris, S. (1991). The early evolution of Metazoa and the significance of problematic taxa. Cambridge, UK: Cambridge University Press.
Simonetta, A. M. (1988). Is Nectocaris pteryx a chordate? Boll. Zool. 55, 63–68. doi:10.1080/11250008809386601
Smith, M. R., and Caron, J.-B. (2011). Nectocaris and early cephalopod evolution: Reply to Mazurek & zatoń. Lethaia 44, 369–372. doi:10.1111/j.1502-3931.2011.00295.x
Smith, M. R., and Caron, J.-B. (2010). Primitive soft-bodied cephalopods from the Cambrian. Nature 465, 469–472. doi:10.1038/nature09068
Smith, M. R. (2013). Nectocaridid ecology, diversity, and affinity: Early origin of a cephalopod-like body plan. Paleobiology 39, 297–321. doi:10.1666/12029
Sperling, E. A., and Stockey, R. G. (2018). The temporal and environmental context of early animal evolution: Considering all the ingredients of an “explosion. Integr. Comp. Biol. 58, 605–622. doi:10.1093/icb/icy088
Steinmetz, P. R. H., Kraus, J. E. M., Larroux, C., Hammel, J. U., Amon-Hassenzahl, A., Houliston, E., et al. (2012). Independent evolution of striated muscles in cnidarians and bilaterians. Nature 487, 231–234. doi:10.1038/nature11180
Swalla, B. J., and Smith, A. B. (2008). Deciphering deuterostome phylogeny: Molecular, morphological and palaeontological perspectives. Philos. Trans. R. Soc. B Biol. Sci. 363, 1557–1568. doi:10.1098/rstb.2007.2246
Technau, U., and Genikhovich, G. (2018). Evolution: Directives from sea anemone Hox genes. Curr. Biol. 28, R1303–R1305. doi:10.1016/j.cub.2018.09.040
Telford, M. J., Budd, G. E., and Philippe, H. (2015). Phylogenomic insights into animal evolution. Curr. Biol. 25, R876–R887. doi:10.1016/j.cub.2015.07.060
Tian, Q., Zhao, F., Zeng, H., Zhu, M., and Jiang, B. (2022). Ultrastructure reveals ancestral vertebrate pharyngeal skeleton in yunnanozoans. Science 377, 218–222. doi:10.1126/science.abm2708
Turner, E. C. (2021). Possible poriferan body fossils in early Neoproterozoic microbial reefs. Nature 596, 87–91. doi:10.1038/s41586-021-03773-z
Valentine, J. W. (2002). Prelude to the cambrian explosion. Annu. Rev. Earth Pl. S. C. 30, 285–306. doi:10.1146/annurev.earth.30.082901.092917
Vernale, A., Prünster, M. M., Marchianò, F., Debost, H., Brouilly, N., Rocher, C., et al. (2021). Evolution of mechanisms controlling epithelial morphogenesis across animals: New insights from dissociation-reaggregation experiments in the sponge Oscarella lobularis. Bmc Ecol. Evol. 21, 160. doi:10.1186/s12862-021-01866-x
Vinther, J., Parry, L., Briggs, D. E. G., and Roy, P. V. (2017). Ancestral morphology of crown-group molluscs revealed by a new Ordovician stem aculiferan. Nature 542, 471–474. doi:10.1038/nature21055
Voigt, O., Adamski, M., Sluzek, K., and Adamska, M. (2014). Calcareous sponge genomes reveal complex evolution of α-carbonic anhydrases and two key biomineralization enzymes. Bmc Evol. Biol. 14, 230. doi:10.1186/s12862-014-0230-z
Wagner, G. P., and Lynch, V. J. (2010). Evolutionary novelties. Curr. Biol. 20, R48–R52. doi:10.1016/j.cub.2009.11.010
Walcott, C. D. (1911). Cambrian geology and paleontology II: No. 3–Middle cambrian holothurians and medusae. Smithson. Misc. Collect. 57, 41–68.
Wood, R., Liu, A. G., Bowyer, F., Wilby, P. R., Dunn, F. S., Kenchington, C. G., et al. (2019). Integrated records of environmental change and evolution challenge the Cambrian Explosion. Nat. Ecol. Evol. 3, 528–538. doi:10.1038/s41559-019-0821-6
Yang, Q., Ma, J., Sun, X., and Cong, P. (2007). Phylochronology of early metazoans: Combined evidence from molecular and fossil data. Geol. J. 42, 281–295. doi:10.1002/gj.1074
Yin, Z., Zhu, M., Davidson, E. H., Bottjer, D. J., Zhao, F., and Tafforeau, P. (2015). Sponge grade body fossil with cellular resolution dating 60 Myr before the Cambrian. Proc. Natl. Acad. Sci. 112, E1453–E1460. doi:10.1073/pnas.1414577112
Yun, H., Zhang, X., Brock, G. A., Li, L., and Li, G. (2021). Biomineralization of the cambrian chancelloriids. Geology 49, 623–628. doi:10.1130/g48428.1
Zhao, Y., Parry, L. A., Vinther, J., Dunn, F. S., Li, Y., Wei, F., et al. (2023). An early Cambrian poly reveals a potential anemone-like ancestor for medusozoans cnidarians. Palaeontology 66, e12637.
Zhao, Y., Vinther, J., Li, Y., Wei, F., Hou, X., and Cong, P. (2022). An early Cambrian mackenziid reveals links to modular Ediacaran macro-organisms. Pap. Palaeontol. 8, e1412. doi:10.1002/spp2.1412
Zhao, Y., Vinther, J., Parry, L. A., Wei, F., Green, E., Pisani, D., et al. (2019). Cambrian sessile, suspension feeding stem-Group ctenophores and evolution of the comb jelly body plan. Curr. Biol. 29, 1112–1125.e2. doi:10.1016/j.cub.2019.02.036
Keywords: metazoan, evolutionary grade, Cambrian, problematic fossils, homology, taphonomy
Citation: Cong P (2023) The early animal radiation: insights from interpreting the Cambrian problematic fossils. Front. Earth Sci. 11:1120118. doi: 10.3389/feart.2023.1120118
Received: 09 December 2022; Accepted: 02 May 2023;
Published: 10 May 2023.
Edited by:
Bruce S. Lieberman, University of Kansas, United StatesReviewed by:
Julien Kimmig, State Museum of Natural History Karlsruhe, GermanyDongjing Fu, Northwest University, China
Copyright © 2023 Cong. This is an open-access article distributed under the terms of the Creative Commons Attribution License (CC BY). The use, distribution or reproduction in other forums is permitted, provided the original author(s) and the copyright owner(s) are credited and that the original publication in this journal is cited, in accordance with accepted academic practice. No use, distribution or reproduction is permitted which does not comply with these terms.
*Correspondence: Peiyun Cong, Y29uZ0B5bnUuZWR1LmNu