- 1Department of Atmospheric Sciences, Yunnan University, Kunming, China
- 2Center for Monsoon System Research, Institute of Atmospheric Physics, Chinese Academy of Sciences, Beijing, China
This paper reviews recent advances in understanding the interaction between the East Asian winter monsoon (EAWM) and El Niño-Southern Oscillation (ENSO). The achievements are summarized into two aspects: 1) the impacts of ENSO on the EAWM, and 2) effects of the EAWM on ENSO. For the first aspect, the results show that: the current climate model simulations of ENSO impacts on the EAWM have a common weaker bias than in the observations; The influence of central Pacific type ENSO on the EAWM is generally weaker than that of the eastern Pacific type ENSO; The precipitation anomalies in the tropical Indian Ocean tend to contribute to the intra-seasonal transition of ENSO teleconnection over East Asia; The ENSO-EAWM relationship is unstable and subject to non-linear modulation by the state of oceans and extratropical atmospheric phenomena, which include the Pacific Decadal Oscillation and the Arctic Oscillation. Regarding the second aspect, studies have shown that the “pure” EAWM (denoted as EAWMres), which is independent of the ENSO signal, can lead to significant variations in the tropical convection over the western Pacific, the local Hadley circulation over East Asia, and the Walker circulation over the equatorial latitudes; The CMIP6 models can preproduce the above EAWMres effects well, although with some weaker bias. The changes in tropical convection and extratropical zonal flow associated with the EAWMres tend to have a significant modulating effect on the ENSO atmospheric teleconnection over North America. A strong EAWM and a strong Australian summer monsoon coherently provide favorable conditions for the onset of El Niño.
1 Introduction
The East Asian winter monsoon (denoted as EAWM) is one of the essential components of the global climate system during boreal winter (Staff Members of the Academia Sinica, 1957; Ding, 1994; Chen et al., 2000; Wang and Lu, 2017; Chen et al., 2019a). The EAWM serves as a major conduit for cold air from high latitudes moving south to low latitudes, and dominants the weather and climate in East Asia (Chen and Graf, 1998; Iwasaki et al., 2014; Liu et al., 2021). The EAWM is characterized by strong near-surface northerly winds along the coast of East Asia (Figure 1). These northerlies can continue to travel southward into the South China Sea, reach the Maritime Continent, and sometimes cross the equator (Boyle and Chen, 1987; Chen and Graf, 1998). The intrusion of northerlies into the tropics can stir up/enhance tropical convective activity; through this, the EAWM can influence the latent heat released by convection around the Maritime Continent (Lau and Lau, 1984; Wang and Chen, 2010; Gong et al., 2014; Ma et al., 2018b; Jiao and Wu, 2019). In addition, the Maritime Continent heat source is of primary importance for driving the global atmospheric circulation in boreal winter (Ramage, 1971; Nitta, 1987). Through its influence on the tropical convection, the EAWM can further affect the local Hadley circulation over East Asia and the Walker circulation along the equatorial latitudes (Figure 1; Chen and Graf, 1998; Ma et al., 2018a; Ma et al., 2018b; Ma et al., 2020). Therefore, the EAWM serves as a bridge between the high latitudes and the tropics and has a far-reaching impact on global atmospheric circulation.
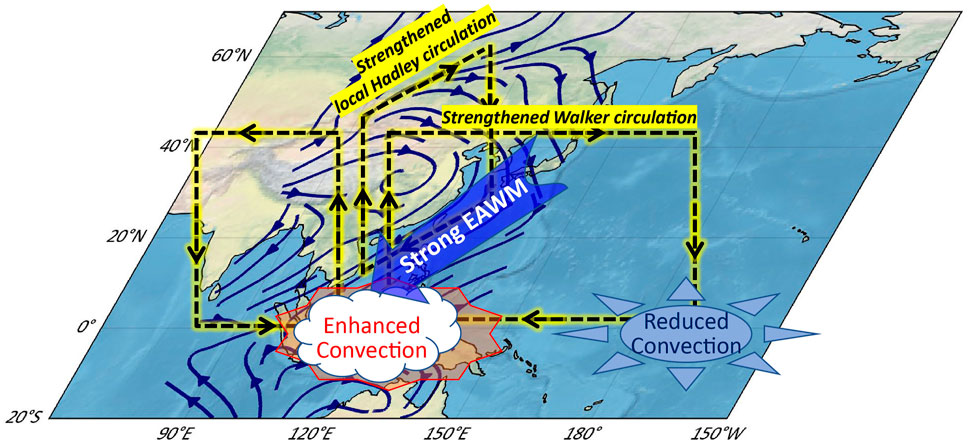
FIGURE 1. Schematic diagram illustrating the effects of EAWM on tropical convection and large-scale atmospheric circulation. A strong EAWM can cause anomalous convergence over the tropical western Pacific region, leading to enhanced convective activities there. The enhanced convection further leads to strengthened local Hadley circulation over East Asia and strengthened Walker circulation along the equatorial latitudes.
The El Niño-Southern Oscillation (ENSO) is the dominate mode of interannual climate variability on Earth. The tropical convection anomalies associated with ENSO can generate Rossby waves that propagate outside the tropics; hence ENSO has profound impacts on extratropical regions (Horel and Wallace, 1981; Trenberth et al., 1998; Yeh et al., 2018; Hu et al., 2021). East Asia is one of the regions that affected by ENSO (Tao and Zhang, 1998; Chen et al., 2000). During El Niño (La Niña) mature phase (winter), the East Asian region usually experiences increased (decreased) precipitation and warmer (colder) temperature (Zhang et al., 1996; Chen et al., 2000; Wu et al., 2003). It is well established that an anomalous anticyclone (cyclone) over the western North Pacific (WNP) play a key role in connecting the El Niño (La Niña) to the East Asian climate (Zhang et al., 1996; Wang et al., 2000; Chen et al., 2013a; Gong et al., 2015; Kim et al., 2017a; Feng et al., 2017).
Considerable progress has been made in recent years in understanding the interaction between ENSO and the EAWM. Previous paper has reviewed recent advances in the ENSO atmospheric teleconnections (abbreviated as ENSO teleconnections hereafter; Yeh et al., 2018). However, there are both progresses and challenges in understanding the ENSO atmospheric teleconnection over the East Asia. For example, to what extent can the state-of-the-art climate models reproduce the ENSO-EAWM relationship; how does the diversity of ENSO affects this teleconnection? What are the roles of atmospheric circulation and ocean outside the tropical Pacific Ocean in modulating the teleconnection? On the other hand, in spite of numerous studies on the impact of ENSO on the EAWM, there are relatively few studies on the effects of EAWM on ENSO. It is crucial to reveal the modulation effect of the EAWM on ENSO atmospheric teleconnections and the influence of East Asian cold surges on the development of El Niño. Progress and debate on these issues motivate us to review on the interaction between ENSO and the EAWM.
The remainder of this paper is organized as follows: Section 2 introduces achievements regarding the impact of ENSO on the EAWM. In this section, we focus on climate models simulations, diversity, non-linearity, and modulation factors of the ENSO-EAWM teleconnection. The progress on effects of the EAWM on ENSO are presented in Section 3. A summary and an outlook for future investigations are provided in Section 4.
2 The impact of ENSO on the EAWM
2.1 In observations
ENSO is one of the most important external forcings influencing the variations of the EAWM (Zhang et al., 1996; Chen et al., 2000; Wang et al., 2000; Wu et al., 2003; Huang et al., 2004; Chen et al., 2019a). El Niño is usually accompanied by a weakening of the EAWM, which advects warm and moist air to East Asia such as southern China, Japan, and Korea; reversed conditions are observed in the La Niña winters, but with smaller amplitudes (e.g., Kug et al., 2012; Son et al., 2014; Ma et al., 2018a). Air pollution over northern China tends to get worse (lighter) during the El Niño (La Niña) winters due to the weakening (strengthening) of EAWM (An et al., 2022a; An et al., 2022b; Zhao et al., 2022).
2.1.1 Possible mechanisms
The mechanisms by which ENSO affects the EAWM have been extensively investigated. Studies consistently showed that an anomalous anticyclone (cyclone) over the WNP played a critical role (e.g., Zhang et al., 1996; Wang et al., 2000). The anomalous anticyclone (cyclone) is a Rossby wave response to the cooling (heating) over the tropical western Pacific, where convection is depressed (increased) during El Niño (La Niña). Southerly (northerly) winds anomalies on the western edge of the anticyclone (cyclone) in turn weaken (enhance) the EAWM (e.g., Zhang et al., 1996; Wang et al., 2000).
In addition to the anomalous WNP anticyclone (cyclone) mechanism, some recent studies have shown that ENSO can also affect the EAWM through other pathways, such as exciting Rossby wave trains over Eurasia. During boreal winter, there are two dominant modes of the wave train over Eurasia, the northern wave train along the polar jet and the southern wave train along the subtropical jet (Hu et al., 2018; Jiao et al., 2019). Studies suggested that El Niño tends to force a northern wave train that propagates into East Asia (Tian and Fan, 2020; Yu and Sun, 2020; An et al., 2022a; Zhao et al., 2022). This northern wave train is reinforced by the positive feedback effect of sea-air coupling over the Mediterranean (An et al., 2022a). It has also been argued that the southern wave train, especially the parts over South and East Asia, can be forced by ENSO (Ma et al., 2022; Zhao et al., 2022). They suggested that precipitation anomalies in the tropical Indian Ocean that associated with ENSO played a crucial role.
2.1.2 Asymmetric impact of El Niño and La niña
It has been well documented that El Niño tends to have a stronger impact on the East Asian winter climate than La Niña (e.g., Zhang et al., 1996; Chen, 2002; Wu et al., 2010; Feng et al., 2017; Zhang et al., 2017). Different perspectives are proposed to understand this asymmetric effect. For example, Wu et al. (2010) showed that, compared to the anomalous WNP anticyclone during El Niño, the anomalous WNP cyclone during La Niña is weaker and shifted more westward, resulting in a weaker climate impact on East Asia. They argued that the asymmetries in the amplitude and longitude of SST anomaly in the WNP are the most important factors. However, Zhang et al. (2015), Zhang et al., 2017 showed that the amplitude of SST anomalies in the WNP was similar during the El Niño and La Niña winters. They suggested that the difference in intraseasonal oscillation between El Niño and La Niña, i.e., strong (weak) intraseasonal oscillation but weak (strong) interannual oscillation during La Niña (El Niño), is responsible for the asymmetric effects. Moreover, it has also been suggested that the asymmetry in background state may plays a vital role in the asymmetry of ENSO atmospheric teleconnections (Feng et al., 2017).
2.1.3 Different impact on the EAWM by ENSO diversity
There is a growing interest in the ENSO atmospheric teleconnections considering the diversity in ENSO spatial patterns (Weng et al., 2009; Feng et al., 2010; Jia et al., 2016; Ding et al., 2017b; Feng et al., 2017; Yeh et al., 2018). Chen et al. (2018) reviewed the progresses of the impacts of two types of ENSO, namely the Central Pacific (CP) and the Eastern Pacific (EP) types, on the East Asian monsoons in the past decade. They concluded that the CP type El Niño generally has a weaker impact on the EAWM than the EP type. And the weaker impact is attributed to the smaller amplitude and westward shift of the anomalous anticyclone associated with the CP El Niño compared to that associated with the EP El Niño (e.g., Weng et al., 2009; Feng et al., 2010). In addition, Huang et al. (2021) suggested that the winter climate over southern China is significantly affected by the poleward edge of the regional Hadley cell over the western Pacific, which in turn is influenced by the CP type of ENSO.
Studies on the El Niño diversity often classify the El Niño events into the EP and CP types. The EP and CP El Niños are characterized by SST anomalies located in the eastern and central tropical Pacific, respectively. However, recent studies argued that the CP El Niño needs to be further separated into the CP-I and CP-II types because different spatial patterns and evolutions of SST anomalies (Wang and Wang 2013; Chen M. et al., 2019). The CP-I El Niño SST anomalies originate at the equator and are symmetric around the equator. The CP-II El Niño SST anomalies are asymmetric about the equator, with additional warm SST anomalies extending from the central Pacific towards North America (Wang and Wang 2013; Chen et al., 2019b). Chen et al. (2019b) further suggested that the three different types of El Niño, namely the EP, CP-I and CP-II types, can lead to distinct responses of the western Pacific subtropical high. Kim et al. (2021) investigated the varying response of EAWM to the above three types of El Niño in both observations and model hindcasts. They reported that the EP El Niño has the strongest weakening effect on the EAWM, followed by the CP-II and then the CP-I types.
2.1.4 Sub-seasonal changes in ENSO’s signal over East Asia
Accumulating evidence has shown that the impacts of ENSO on the EAWM have clear sub-seasonal changes, with stronger impacts during early winter (Nov-Dec) and weaker impacts during late winter (Jan-Feb) (Son et al., 2014; Kim et al., 2018; Tian and Fan, 2020; Li et al., 2021; Ma et al., 2022; Zhao et al., 2022). For example, Son et al. (2014) reported that the impacts of ENSO on precipitation in Korea is strong in early winter and weak in late winter. Zhao et al. (2022) showed that the impact of ENSO on haze pollution over the Beijing-Tianjin-Hebei region is strong in early winter while weak in late winter. Ma et al. (2022) further showed that the ENSO-induced anomalous southerly winds along the East Asian coast can extent to mid-and-high latitudes in early winter, whereas those anomalous winds are confined to the subtropics in late winter. Figure 2 shows the 500-hPa geopotential height anomalies over East Asia are distinct different in the early and late winter of the ENSO events. In the El Niño (La Niña) early winters, a weakened (strengthened) East Asian Trough is observed; however, in the late winters, anomalies in the East Asian Trough are weak and insignificant.
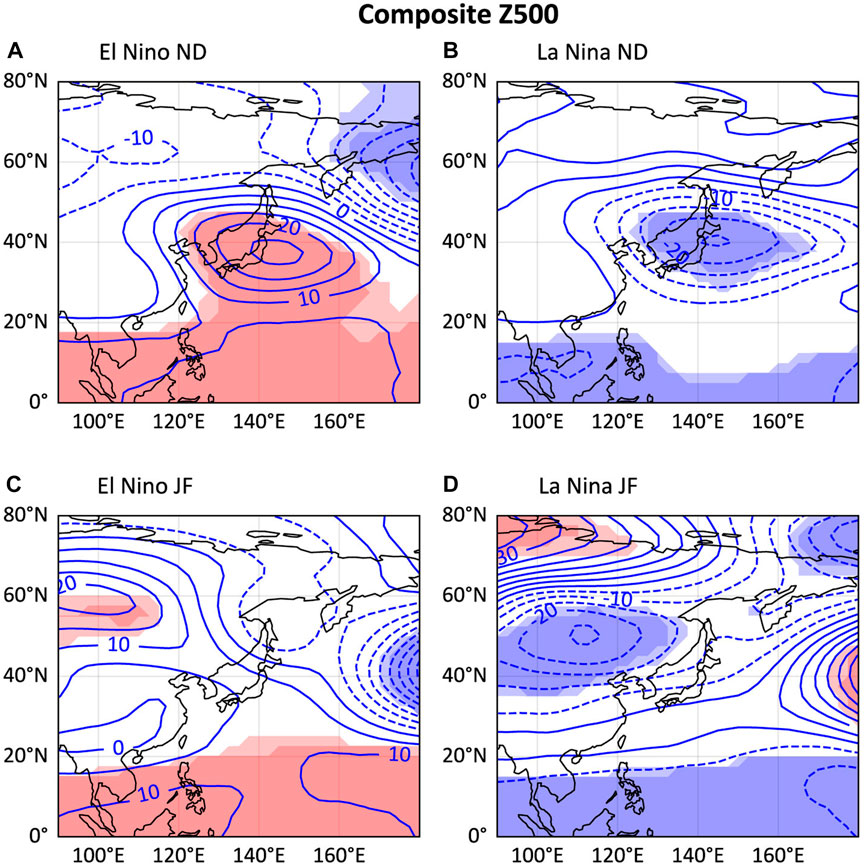
FIGURE 2. Composite patterns of (A, B) Nov-Dec-averaged 500 hPa anomalous geopotential height (Z500, unit = gpm, contour interval = 5) in El Niño and La Niña winters during 1979–2021, respectively. (C, D) Are the same as in (A, B), but for Jan–Feb-averaged Z500 anomalies. Red (Blue) shading indicates positive (negative) anomalies that exceed the 95% confidence level. The list of El Niño and La Niña events can be found in NOAA’s Climate Prediction Center https://origin.cpc.ncep.noaa.gov/products/analysis_monitoring/ensostuff/ONI_v5.php.
Studies on the mechanisms of the sub-seasonal variation of the ENSO-East Asia teleconnection have shown progresses with some controversies. Kim et al. (2018) suggested that the weakened precipitation anomaly in the WNP and the enhanced precipitation anomaly in the tropical CP from December to January lead to changes in the ENSO-East Asia teleconnection. In addition, the precipitation in the tropical Indian Ocean (TIO) may also play an important role. Several studies have shown that ENSO can induce a Rossby wave train emitting from the TIO to East Asia during early winter (Figure 3), yet this wave train is very weak and without an extratropical node during late winter (Tian and Fan, 2020; Ma et al., 2022; Zhao et al., 2022). Ma et al. (2022) identified that the formation of this wave train is closely related to the precipitation anomalies in the TIO, especially in the eastern TIO (Figure 3). During El Niño early winter, positive and negative precipitation anomalies occur in the western and eastern TIO, respectively. The diabatic heating associated with the precipitation anomalies, particularly the eastern part, stimulates a Rossby wave train emitting from the TIO towards East Asia. This TIO-East Asia wave train further leads to a weakened East Asian trough, and thereby a weakened EAWM. The situations are reversed in La Niña. Because the climatological mean SST is warmer and the precipitation in the TIO is stronger in early than late winter, the ENSO-induced TIO precipitation anomalies and the associated atmospheric response are stronger in early winter than in late winter (Ma et al., 2022).
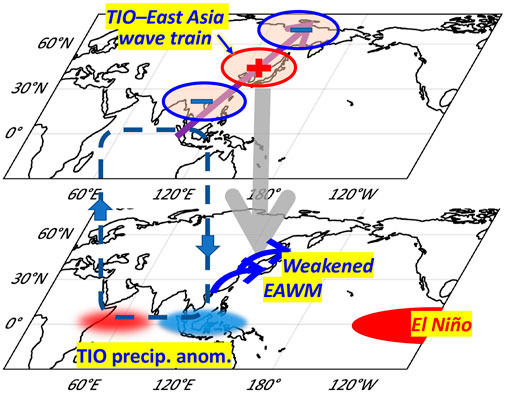
FIGURE 3. Schematic diagram illustrating possible roles of the precipitation anomalies in the tropical Indian Ocean (TIO) in the teleconnection of ENSO over East Asia. During El Niño early winter, positive and negative precipitation anomalies occur in the western and eastern TIO, respectively. The diabatic heating associated with the precipitation anomalies, particularly the eastern part, stimulates a Rossby wave train emitting from the TIO towards East Asia. This TIO-East Asia wave train further leads to a weakened East Asian trough, and thereby a weakened EAWM. The situations are reversed in La Niña.
The North Atlantic Ocean may also play a role in the sub-seasonal variations of ENSO-East Asia teleconnection (Feng and Chen, 2022). The variation of EAWM has shown to be closely related to two Rossby wave trains propagating from the North Atlantic to East Asia along the polar and the subtropical jets (Jiao et al., 2019; Wei et al., 2020; An et al., 2022a). During the ENSO early and late winters, both two wave trains exhibit significant differences (An et al., 2022a; Ma et al., 2022). However, the mechanism of the sub-seasonal variation of these two wave trains remains to be investigated.
2.1.5 Modulation factors on the ENSO-EAWM teleconnection
The ENSO-EAWM teleconnection can be affected by the state of the oceans, such as the North Pacific, the Indian Ocean, and the Atlantic Ocean.
The Pacific Decadal Oscillation (PDO) has been documented to modulate the effects of ENSO on the EAWM (Wang et al., 2008; Feng et al., 2014; Ding et al., 2017a; Jia and Ge, 2017a). The PDO is often described as decades-long El Niño-like pattern of Pacific climate variability (Newman et al., 2016). The positive (negative) PDO phase is characterized by warm (cold) SST anomalies along the tropical Pacific and cool (warm) SST anomalies in the North Pacific. In an in-phase combination of ENSO and the PDO (i.e., El Niño/positive PDO and La Niña/negative PDO), the ENSO-EAWM relationship tends to be strong and significant. In contrast, in an out-of-phase combination, the ENSO-EAWM correlation becomes weak and insignificant. The PDO can also modulate the asymmetry impacts of El Niño and La Niña on East Asian winter climate (Gao et al., 2019). They showed that the asymmetric impact was significant during the positive phase of PDO and disappeared during the negative phase of PDO. The warm (cold) background of the tropical Pacific in positive (negative) PDO played a key role in amplifying (reducing) the asymmetric responses of convection anomaly over the WNP to ENSO, resulting in large (small) asymmetric impacts of ENSO over East Asia (Gao et al., 2019).
The Atlantic Multidecadal Oscillation (AMO) has also been found to play a modulation role. The AMO is known as a coherent mode of natural variability in the North Atlantic Ocean on the timescale of several decades (60–80 years). The phase of AMO is based on the average SST anomalies in the North Atlantic basin (0°–80°N). A positive (negative) phase of AMO may drive a westward (eastward) shifted anomalous WNP anticyclone, contributing to a stronger (weaker) impact of El Niño on the EAWM (Kim et al., 2017a).
In the tropical Indian Ocean, the Indian Ocean dipole (IOD) is recognized to be an important component of the SST variability especially during boreal autumn. A positive IOD is featured with warmer SST anomalies in the western Indian Ocean relative to the east, while the opposite is true for a negative IOD. The IOD is considered to be weakly correlated to ENSO, though the strongest and most extreme events do show better teleconnections. For example, Zhang X. D. et al. (2022) reported that the combined effect of ENSO and the IOD contributed to the extreme wetness in Eastern China during summer 2020. In addition, the state of the tropical Indian Ocean has been suggested to play an important role in the subseasonal transition of ENSO-EAWM relationship (Ma et al., 2022). The climatological mean SST and precipitation in are warmer and stronger in early winter than in late winter. According to the Clausius-Clapeyron relationship, the early winter warmer and wetter basic-state in the tropical northern Indian Ocean can provide favorable conditions for a stronger atmospheric response to SST anomaly (Hu et al., 2021; Ma et al., 2022). Thus, the stronger atmospheric response to ENSO SST in early winter leads to a closer ENSO-EAWM relationship.
Extratropical atmospheric circulations have also been found to be potential factors modulating the ENSO-EAWM relationship (Yu and Sun, 2018; Chen et al., 2020; Yu and Sun, 2020; An et al., 2022). Chen et al. (2013b) found that when the ENSO and Arctic Oscillation (AO) are in in-phase combinations (El Niño/negative AO and La Niña/positive AO), the climate in northern and southern China are mainly influenced by the AO and ENSO, respectively. However, when the ENSO and AO are in out-of-phase combinations, the climate anomalies in China, especially in southern China, exhibit a very different pattern. Their results suggested that ENSO and the AO have a non-linear superposition effect on the EAWM. Chen et al. (2020) reported that after removing the AO signal, the ENSO-EAWM relationship shows a stable and significant negative correlation over the past 140 years. Luo et al. (2021) found that ENSO affects the winter cold anomalies over central Eurasia through modulating the strengthen and location of the Ural blocking. Song and Wu (2022) further found that the cold events during El Niño winters are closely related to negative AO, while the cold events during La Niña winters are mainly associated with the Ural Blocking. Dai and Tan (2019) showed that the eastern Pacific atmospheric teleconnection pattern plays an important role in the impact of ENSO on East Asian winter climate. Particularly, the eastern Pacific teleconnection pattern can induce significant East Asian temperature anomalies during its developing and mature phase.
2.2 Performance of the CMIP models
In this subsection, we begin by reviewing the performance of climate models in representing the climatology and interannual variability of the EAWM. Both the multi-model ensemble (MME) of the fifth and sixth phase of the Coupled Model Intercomparison Project (CMIP5/6) (Taylor et al., 2012; Eyring et al., 2016) and the multi-member mean from the Community Earth System Model (CESM) Large Ensemble Project reproduce well the climatology of the EAWM (Figures 4A–C; Gong et al., 2014; Wang et al., 2019a; Jiang et al., 2020). It is suggested that the CMIP5 models have a slightly strong bias in simulating the climatological mean of the EAWM, especially for its southern part (Figure 4F; Gong et al., 2014). Figures 4D–G further show that the biases are different between the northern and southern parts of the EAWM. For the northern part of the EAWM, both CMIP5 and CMIP6 have a common weaker bias. However, for the southern part of the EAWM, the CMIP5 has a stronger bias with a remarkable improvement in the CMIP6. In addition, the interannual variations of the EAWM can be distinguished into two modes, namely the northern and the southern modes (Chen et al., 2014). Studies have also shown that the variations of winter-mean surface air temperature (SAT) in East Asia are dominated by two different principal modes (Wang et al., 2000; Li and Yang, 2017). The first mode is characterized by SAT variations north of 40°N, while the second mode is characterized by SAT variations south of 40°N. More importantly, Li and Yang (2017) analyzed the quantitative contributions of thermodynamic and dynamic processes to both modes. Their results indicated that the northern SAT mode is closely related to AO and the southern SAT mode is tightly connected to ENSO. Gong et al. (2018) showed that the variation of the EAWM northern mode and its relationship with the Siberian high can be well reproduced in the CMIP5 models. Using a recently developed vector field evaluation method, Huang et al. (2019) evaluated the vector winds in the Asian-Australian monsoon region in 37 CMIP5 modes. Their results showed that the MME is the best in reproducing the climatological mean of the vector winds compared to individual models; most models overestimate the strength of low-level winds in tropical areas such as the South China Sea, the Philippine Sea, and the Maritime Continent.
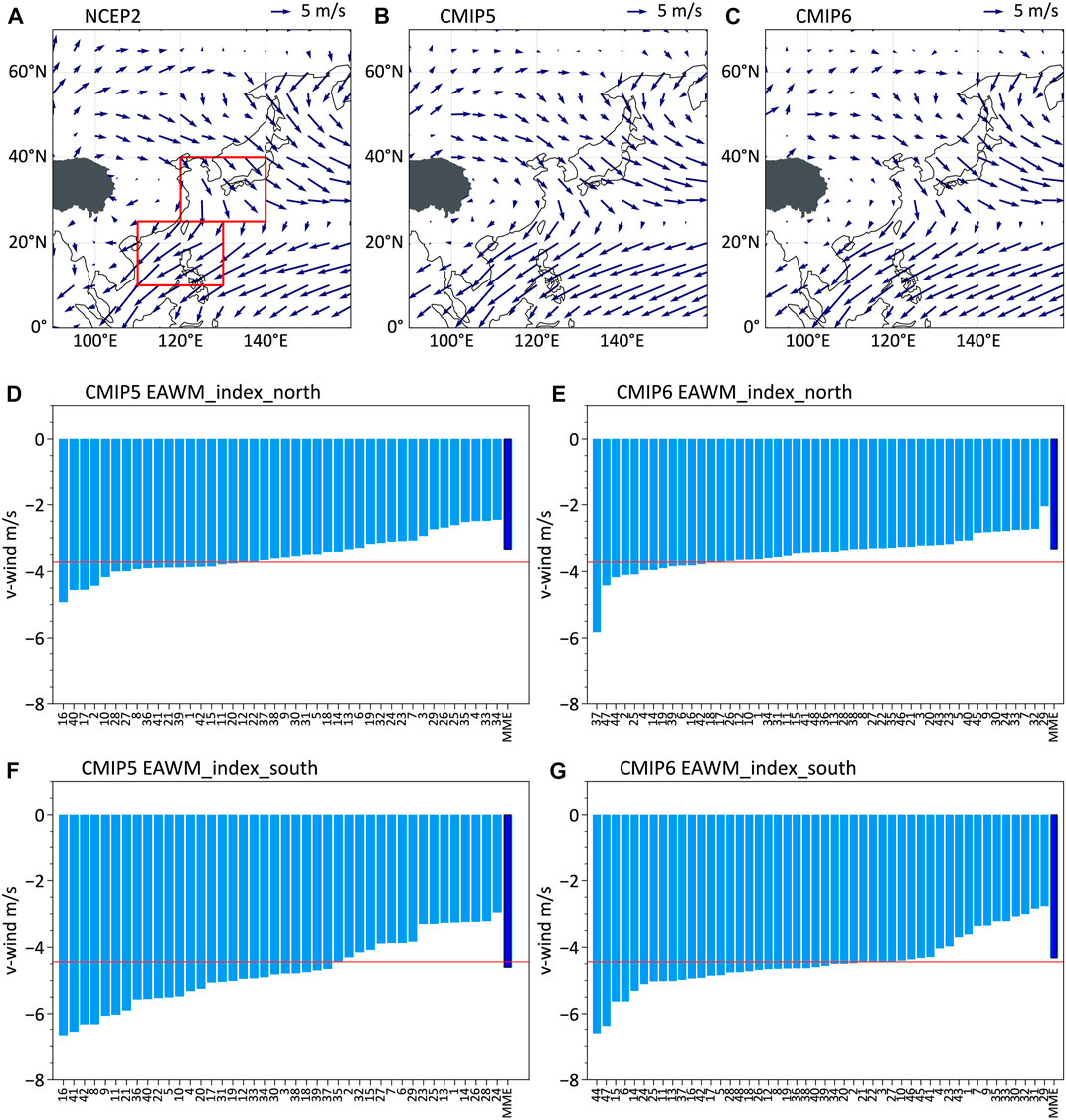
FIGURE 4. Climatological mean of the horizontal winds at 1000 hPa during boreal winter (Dec-Jan-Feb). The data used in each subplot is (A) NCEP/DOE Reanalysis II (denoted as NCEP2) reanalysis during 1979–2014; (B) CMIP5 MME (42 models) during 1979–2005; (C) CMIP6 MME (47 models) during 1979–2014. (D, E) The northern part of the EAWM index simulated by the CMIP5 and CMIP6 models; (F, G) the same as in (D, E), but for the southern part of the EAWM index. The northern and southern parts of the EAWM index (Chen et al., 2000) are defined as the area-mean 1000 hPa meridional wind in (25°–40°N, 120°–140°E; upper red box) and (10°–25°N, 110°–130°E; bottom` red box), respectively. Red lines in (D–G) indicate the corresponding values in the NCEP2 reanalysis. The list of CMIP5/6 models is shown in Table 1.
Recent works have also investigated the performance, systematic biases, and improvements of the climate models in simulating the ENSO-EAWM teleconnection. Figure 5 shows the correlation coefficients between the EAWM index (Chen et al., 2000) and the Niño 3.4 index simulated by the CMIP5/6 models. We can see that most of the models, i.e., 34 of 37 CMIP5 and 39 of 47 CMIP6, reproduced weaker ENSO-EAWM correlations than in the observation. Gong et al. (2014), Gong et al. (2015) showed that about half (16) of the CMIP5 models capture the observed ENSO-EAWM relationship, as well as the key bridge of the WNP anomalous anticyclone/cyclone. They concluded that the amplitude and spatial pattern of the ENSO SST anomalies, especially its longitude location, play a crucial role in simulating the ENSO-EAWM relationship. Wang et al. (2022a) further suggested SST anomalies in the tropical WNP also play an important role. The common bias in the CMIP5/6 models revealed by Jiang et al. (2022) is that the ENSO-EAWM relationship is weaker than in the observations. This bias can be attributed to the excessive extension of cold tongue to the equatorial western Pacific. And the related mechanism has been suggested as follows: a stronger climatological cold tongue causes the ENSO-SST anomaly to extend westward, forcing an anomalous cyclonic circulation over the WNP, offsetting the key bridge of the anomalous WNP anticyclone, and resulting in a weakened ENSO-EAWM relationship. Overall, the ability of CMIP3, CMIP5, and CMIP6 models in simulating the ENSO-EAWM relationship has been gradually improved (Gong et al., 2015; Jiang et al., 2022). However, Kim et al. (2017b) reported that the CMIP5 models fail to capture the sub-seasonal transition of ENSO teleconnection over the East Asia-North Pacific region. This may be attributed to systematic errors in the amplitude of precipitation anomalies in the WNP and tropical central Pacific associated with ENSO.
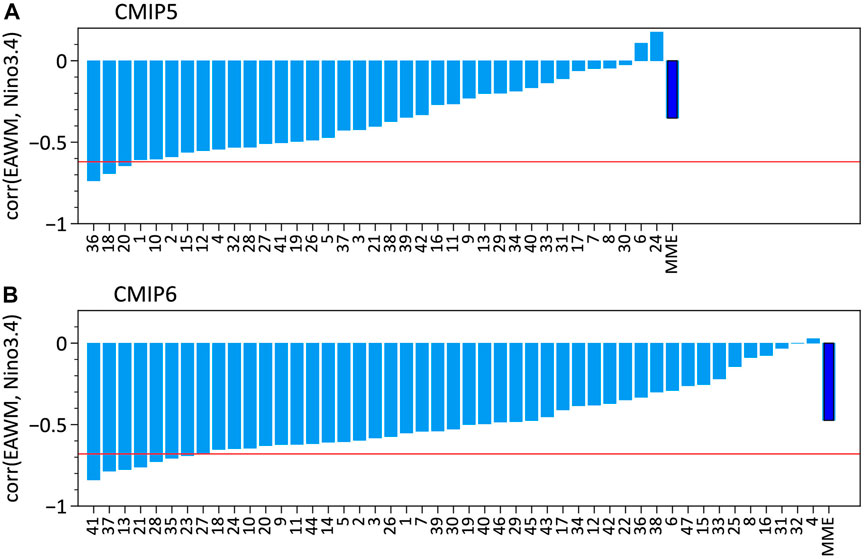
FIGURE 5. Correlation coefficients between the winter (Dec-Jan-Feb-averaged) EAWM index and Niño 3.4 index (A) in 37 CMIP5 models during 1979–2005; (B) in 47 CMIP6 models during 1979–2014. Red lines in (A, B) indicate the corresponding correlation coefficients using NCEP2 reanalysis. The list of CMIP5/6 models is shown in Table 1.
Previous studies have evaluated the performance of climate models in simulating the two types of ENSO (Kim and Yu, 2012; Kug et al., 2012). More recent studies have been extended to investigate the simulation of atmospheric teleconnections associated with the two types of ENSO. The results consistently showed that most CMIP6 models cannot capture well the different responses of EAWM to the two types of ENSO (Wang et al., 2019b; Kim et al., 2021). Wang et al. (2019b) showed that about half of the CMIP5 models cannot reproduce the response of rainfall in Southern China to either type of ENSO. The remaining half models can only simulate the response to one type of ENSO. Only five models produce a reasonable response to both types of ENSO. Kim et al. (2021) reported that the model hindcasts underestimate the effect of EP and CP-II El Niño on the EAWM. And this underestimation is suggested to be induced by the model’s weak bias in simulating the anomalous WNP anticyclone and its complete inability to simulate anomalous anticyclone near the Kuroshio. In general, present climate models face significant challenges in reproducing the teleconnections of different types of El Niño events.
3 Effects of the EAWM on ENSO
3.1 In observations
3.1.1 Modulation of ENSO teleconnections by the EAWM
The first systematic study of EAWM interannual variability and its connection to global circulation was reported by Chen and Graf (1998). They suggested that both the local Hadley circulation over East Asia and the Walker Circulation over equatorial latitudes are more intense in a strong EAWM winter than in a weak EAWM winter (Figure 1). This can be attributed to the impacts of EAWM on tropical convection. The tropical convection tends to be enhanced over the Maritime Continent and suppressed over the tropical EP in a strong EAWM winter, while the opposite is true in a weak EAWM winter. In addition, opposite phases of the Pacific North America (PNA) pattern appear in the strong and weak EAWM winters, respectively. They further suggested that this may be related to the barotropic instability of the extratropical zonal flow and the tropical convection anomalies associated with the interannual variation of the EAWM (Chen and Graf, 1998). This study led us to explore the active role played by the EAWM in its interaction with ENSO.
Progresses have been made in extracting the impacts of “pure” EAWM (denoted as EAWMres), which is a component independent of the ENSO signal (Ma and Chen, 2021). Chen et al. (2013a) defined a EAWMres index from the raw EAWM index using partial regression method. Figures 6A, B show that both indexes can represent the variations of low-level winds along East Asia well. As shown in Chen et al. (2013a), the EAWMres accounts for −65% of the total EAWM variance during the period 1957–2001. This percentage has increased to −80% in recent decades (Figure 6C), since the impacts of ENSO on the EAWM has weakened (Jia and Ge, 2017b). Figures 6D, E indicate that the EAWMres index is linearly independent of the SST anomalies in the tropical central-eastern Pacific. For the winter SST anomalies in the South China Sea (SCS), Wu et al. (2014) showed that the EAWMres contributes largely while the independent ENSO signal (with the EAWM signal removed) contributes less.
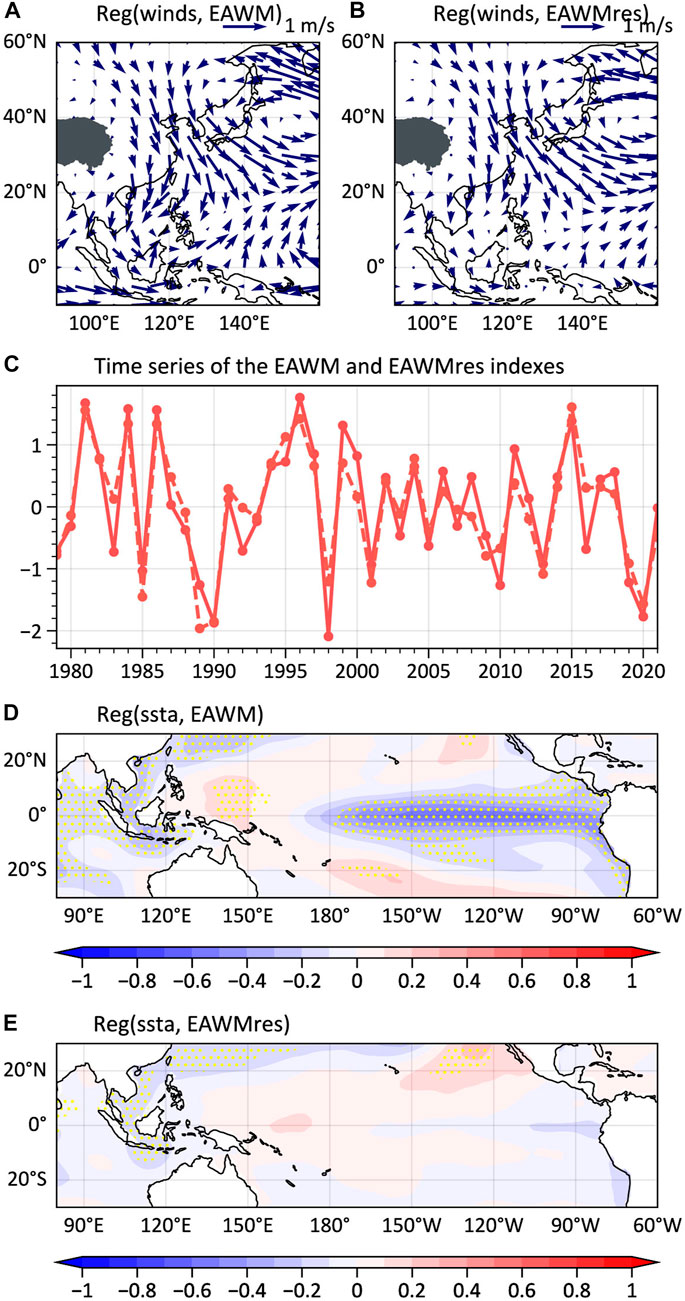
FIGURE 6. Regression patterns of anomalous winds at 850 hPa onto the (A, B) EAWM index and the EAWMres index, respectively. (C)Time series of the EAWM and EAWMres indexes. (D, E) Regression patterns of anomalous SST onto the EAWM and EAWMres indexes, respectively. Dotting in (D, E) indicates anomalies that exceed the 95% confidence level. Details for definitions of the EAWM and the EAWMres indexes can be found in (Chen et al., 2013a; Ma et al., 2018b). The NCEP2 reanalysis and the ERSSTv5 dataset for the period 1979–2021 are used in this figure.
Figure 7 illustrates the effects of EAWMres on tropical convection and large-scale atmospheric circulation. A strong EAWMres can lead to anomalous convergence over the tropical western Pacific (including the southern SCS, Philippine Sea, and Maritime Continent regions), resulting in anomalous ascent and increased precipitation there (Figures 7A, C). In response to the anomalous diabatic heating, the local Hadley circulation over East Asia and the Walker circulation over the northern equatorial Pacific are enhanced (Figures 7E, G). In a weak EAWMres winter, the situations are generally reversed, but the anomalies are smaller. (Figures 7B, D, F, H; Ma et al., 2018a; Ma et al., 2018b; Wang et al., 2022b).
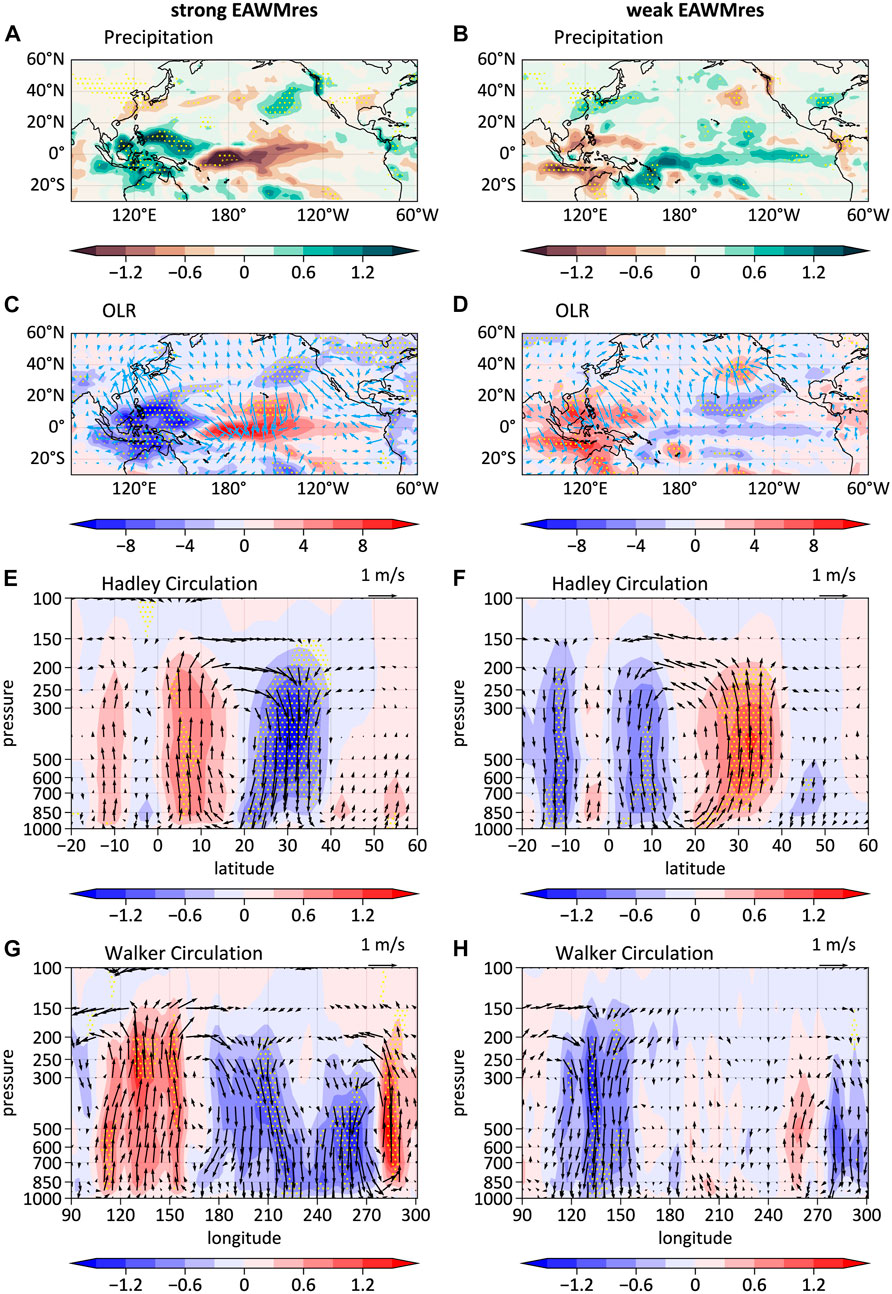
FIGURE 7. Composite anomalous patterns for the strong (left column) and weak (right column) EAWMres winters: (A, B) precipitation (mm day−1), (C, D) outgoing longwave radiation (OLR, color, W m−2) and divergent winds at 200-hPa (vector, m s−1), (E, F) zonal-averaged vertical velocity (shading, −10−2 Pa s−1) and meridional divergent wind (vector, m s−1) along East Asia (100°–140°E), and (G, H) meridional-averaged vertical velocity and zonal divergent wind (vector, m s−1) over (0°–10°N). Dotting indicates the anomalies that exceed the 95% confidence level.
The EAWMres has documented to influence the ENSO teleconnections both over East Asia and North America (Ma et al., 2018a; Ma et al., 2018b). Ma et al. (2018b) showed that the precipitation in southern China-Japan is greatly increased when El Niño coincide with weak EAWMres winters; in contrast, the precipitation anomalies are very weak when El Niño coincide with strong EAWMres winters. This can be attributed to the combined (offsetting) effects of weak (strong) EAWMres and El Niño on tropical convection over the tropical western Pacific, the anomalous WNP anticyclone and the anomalous East Asian local Hadley circulation. The EAWMres can also modulate the surface air temperature (SAT) anomalies in North America associated with ENSO (Ma et al., 2018b; Ma et al., 2020; Ma and Chen, 2021). They showed that the magnitude of SAT differences between strong and weak EAWMres winters in the same phase of ENSO is comparable to the ENSO-related differences. The EAWMres can induce the changes in extratropical zonal flow and tropical convection anomalies, leading to meridional shifts and amplitude changes in the PNA, which in turn affects the SAT anomalies in North America (Ma et al., 2018b; Ma et al., 2020; Ma and Chen, 2021).
3.1.2 Possible role of cold surge activity in triggering an El Niño
Early works by Li (1989, 1990) have reported the link between cold surge activities associated with the EAWM and the occurrence of El Niño. His work showed that the cold surges were stronger and more frequent than normal during the winter preceding El Niño. This is because stronger and more frequent cold surges tend to enhance the tropical convection and weaken the trade winds over the tropical western Pacific, thus providing favorable conditions for El Niño events to occur.
The study by Zheng et al. (2014) have shown that a strong EAWM and a strong Australian summer monsoon usually coexist in the winter preceding an El Niño. Using a simple analytical model, they showed that when anomalous northerlies from the EAWM converge with anomalous southerlies from the Australian summer monsoon, the westerly anomalies over the tropical western Pacific are greatly enhanced. Their study provided a possible explanation for the onset of El Niño concerning the combined effects of the EAWM and the Australian summer monsoon. Recently, Feng et al. (2022) divided the EAWM cold surges into two types according to their pathway. The western type refers to the cold surges passing through the SCS, and the eastern type refers to the cold surge passing through the Philippine Sea. They suggested that only the eastern type of cold surges can efficiently trigger El Niño.
3.2 Model simulations
Based on observational analysis and numerical simulations, Li and Mu (2000) showed that a strong EAWM can cause continuous westerly wind anomalies over the equatorial western Pacific; These anomalous westerly winds can further lead to propagation of the warm subsurface water in the warm pool towards the tropical eastern Pacific, resulting in an El Niño-like SST pattern. The model results showed that a weak EAWM generally has opposite effects. Their work proposed a new perspective that the EAWM can also influence the occurrence of ENSO events in addition to the tropical air-sea interaction processes.
In the review work of Huang et al. (2004), they summarized the thermal and dynamical effects of tropical western Pacific warm pool on the ENSO cycle. Using a simple coupled sea-air model, their theoretical results suggested that the zonal wind anomalies over near surface tropical western Pacific can excite a downwelling oceanic Kelvin wave that propagate eastward, causing warm anomalies in the eastern equatorial Pacific Ocean (Huang et al., 1998). The origins of the zonal wind anomalies over the tropical western Pacific can be categorized to three basic processes: 1) local air-sea interactions; 2) the passage of convective events, such as the Madden-Julian oscillation; and 3) anomalous activities of the EAWM. Overall, they concluded that the EAWM is one of the important mechanisms that trigger the occurrence El Niño.
Figure 8 identifies that the MME of 47 CMIP6 models can reproduce the above-mentioned effects of EAWMres on tropical convection and large-scale atmospheric circulation. The MME shows that a strong EAWMres is connected to positive and negative precipitation anomalies in the tropical western and central Pacific, respectively (Figure 8A), consistent with the observations; however, the simulated precipitation anomalies exhibit a westward shift and have smaller values compared to the observations. The sources of model bias need further investigation. Figures 8C–F further show that more than 80% of the models agree on the effects of EAWMres on the East Asian local Hadley circulation and the Walker circulation, although the MME anomalies are weaker than those in the observations.
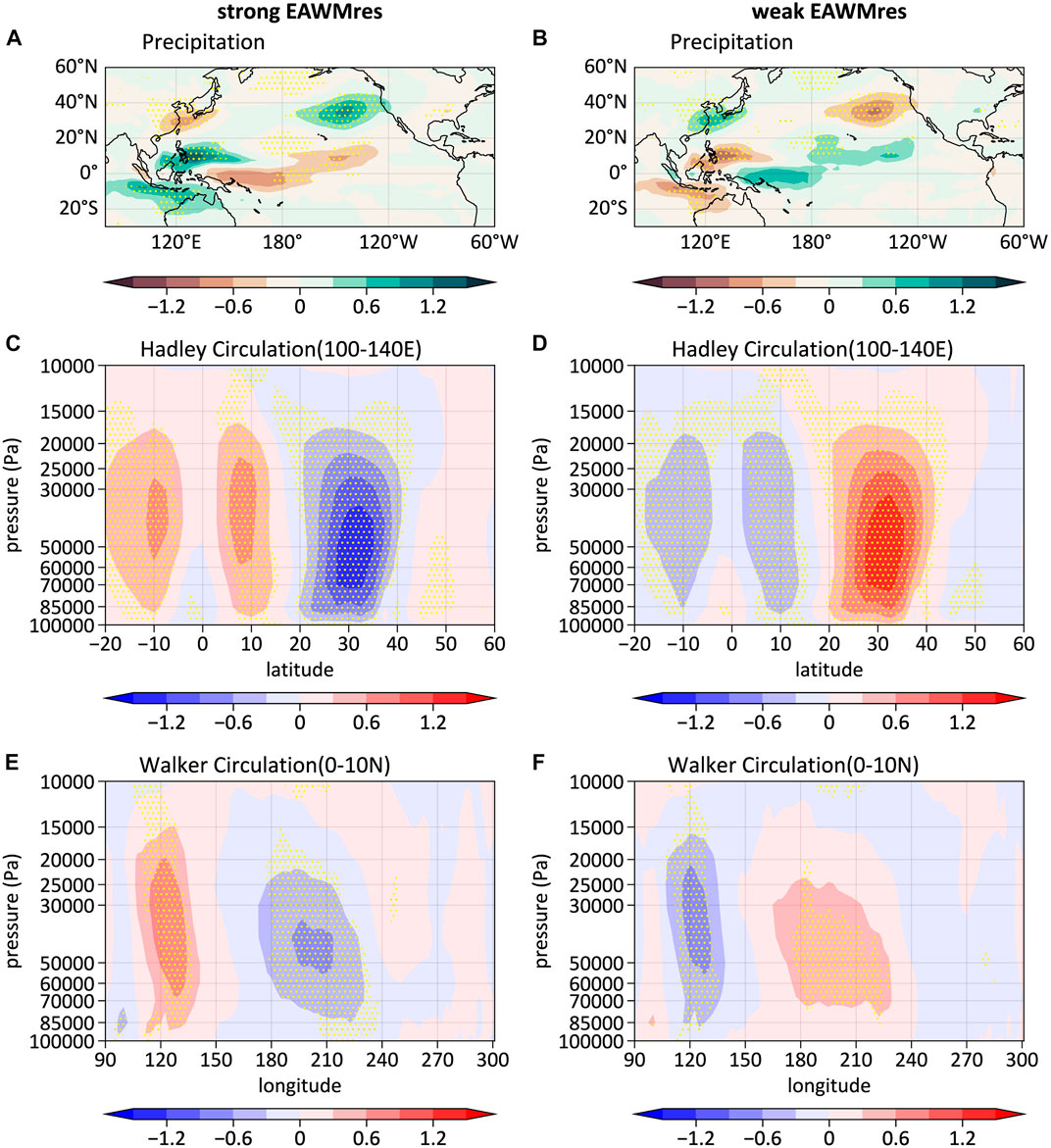
FIGURE 8. The MME of 47 CMIP6 models. Composite anomalous patterns of (A, B) precipitation, (C, D) zonal averaged vertical velocity along (100°–140°E), and (E, F) meridional averaged vertical velocity along (0°–10°N). Left and right columns are for the strong and weak EAWMres winters during 1979–2014, respectively. Dotting indicates more than 80% of the models have consistent signs of the anomalies. The models are shown in Table 1.
4 Summary and outlook
This paper reviews recent achievements in understanding the interactions between ENSO and the EAWM. We mainly focused on two aspects, i.e., the impacts of ENSO on the EAWM and the effects of EAWM on ENSO. For the first aspect, it is suggested that the simulation of ENSO impacts on the EAWM has improved from CMIP5 to CMIP6 models, though both generations of the models have a common weak bias; The impact of CP-type ENSO on the EAWM is generally weaker than the EP-type, and it is difficult for current climate models to reproduce the different impacts of the two types of ENSO on EAWM; The precipitation anomalies in the tropical Indian Ocean can play an important role in the influence of ENSO on the EAWM; The ENSO-EAWM teleconnection is modulated by the state of oceans, such as the PDO and AMO, and has non-linearly interactions with extratropical atmospheric phenomena, such as the AO. Regarding the second aspect, although less attention has been paid on, the important role of EAWMres on modulating the ENSO atmospheric teleconnections is revealed; The combined effect of strong EAWM and strong Australian summer monsoon on the westerly wind anomalies over the tropical western Pacific provides a plausible explanation for the occurrence of El Niño events.
It is noteworthy that the ENSO-EAWM relationship is unstable and modulated by many factors. We still face great challenges in understanding the interaction between these two phenomena. For example, under global warming, the atmospheric circulation response to ENSO will be strengthened, and the frequency of extreme ENSO events will be increased (Cai et al., 2014; Zhou et al., 2014; Yeh et al., 2018; Hu et al., 2021), how will this affect the winter climate in East Asia? During the mid-winter of 2020/21, extreme cold events occurred across East Asia and North America. This can be related to a major sudden stratospheric warming event in a La Niña background (Zhang X. D. et al., 2022; Yu et al., 2022; Zhang Y. et al., 2022). However, the relationship between occurrence/frequency of extreme events and ENSO has not been revealed; the modulating effects of stratospheric atmospheric circulations such as the Poler Vortex and the Quasi-Biennial Oscillation (QBO), have not been identified. In addition, it is crucial to realistically simulate the effects of EAWM on ENSO atmospheric teleconnections.
Author contributions
WC designed the research. TM performed the analysis. TM and WC wrote the paper. Both authors approved the final manuscript.
Funding
This study was supported jointly by the National Natural Science Foundation of China (Grants 41975051 and 42005032), and the China Postdoctoral Science Foundation (Grant 2020M670417).
Conflict of interest
The authors declare that the research was conducted in the absence of any commercial or financial relationships that could be construed as a potential conflict of interest.
Publisher’s note
All claims expressed in this article are solely those of the authors and do not necessarily represent those of their affiliated organizations, or those of the publisher, the editors and the reviewers. Any product that may be evaluated in this article, or claim that may be made by its manufacturer, is not guaranteed or endorsed by the publisher.
References
An, X., Chen, W., Fu, S., Hu, P., Li, C., and Sheng, L. (2022a). Possible dynamic mechanisms of high- and low-latitude wave trains over Eurasia and their impacts on air pollution over the NorthNorth China plain in early winter. J. Geophys. Res. Atmos. 127 (13). doi:10.1029/2022JD036732
An, X., Chen, W., Hu, P., Chen, S., and Sheng, L. (2022b). Intraseasonal variation of the northeast Asian anomalous anticyclone and its impacts on PM<sub>2.5</sub> pollution in the North China Plain in early winter.
Boyle, J. S., and Chen, T. J. (1987). “Synoptic aspects of the wintertime East Asian monsoon,” in Monsoon meteorology. Editors C. P. Chang,, and T. N. Krishnamurti (New York: Oxford Univ. Press), 125–160.
Cai, W., Borlace, S., Lengaigne, M., van Rensch, P., Collins, M., Vecchi, G., et al. (2014). Increasing frequency of extreme El Niño events due to greenhouse warming. Nat. Clim. Change 4 (2), 111–116. doi:10.1038/nclimate2100
Chen, D., Sun, J., and Gao, Y. (2020). Effects of AO on the interdecadal oscillating relationship between the ENSO and East Asian winter monsoon. Int. J. Climatol. 40 (10), 4374–4383. doi:10.1002/joc.6459
Chen, M., Yu, J.-Y., Wang, X., and Jiang, W. (2019b). The changing impact mechanisms of a diverse El Niño on the western pacific subtropical high. Geophys. Res. Lett. 46 (2), 953–962. doi:10.1029/2018GL081131
Chen, W., and Graf, H.-F. (1998). The interannual variability of east asian winter monsoon and its relation to global circulation. Hamburg: Report/Max-Planck-Institut für Meteorologie, 250.
Chen, W., Hans-F, G., and Huang, R. (2000). The interannual variability of EastEast Asian winter monsoon and its relation to the summer monsoon. Adv. Atmos. Sci. 17 (1), 48–60. doi:10.1007/s00376-000-0042-5
Chen, W. (2002). Impacts of El Niño and La Niña on the cycle of the East asian winter and summer monsoon. Chin. J. Atmos. Sci. 26, 595–610. (in Chinese). doi:10.3878/j.issn.1006-9895.2002.05.02
Chen, W., Feng, J., and Wu, R. (2013a). Roles of ENSO and PDO in the link of the east Asian winter monsoon to the following summer monsoon. J. Clim. 26 (2), 622–635. doi:10.1175/JCLI-D-12-00021.1
Chen, W., Lan, X. Q., Wang, L., and Ma, Y. (2013b). The combined effects of the ENSO and the Arctic Oscillation on the winter climate anomalies in East Asia. Chin. Sci. Bull. 58 (12), 1355–1362. doi:10.1007/s11434-012-5654-5
Chen, W., Ding, S., Feng, J., Chen, S., Xue, X., and Zhou, Q. (2018). Progress in the study of impacts of different types of ENSO on the East asian monsoon and their mechanisms. Chin. J. Atmos. Sci. 42 (3), 640–655. doi:10.3878/j.issn.1006-9895.1801.17248
Chen, W., Wang, L., Feng, J., Wen, Z., Ma, T., Yang, X., et al. (2019a). Recent progress in studies of the variabilities and mechanisms of the East asian monsoon in a changing climate. Adv. Atmos. Sci. 36 (9), 887–901. doi:10.1007/s00376-019-8230-y
Chen, Z., Wu, R., and Chen, W. (2014). Distinguishing interannual variations of the northern and southern modes of the East asian winter monsoon. J. Clim. 27 (2), 835–851. doi:10.1175/jcli-d-13-00314.1
Dai, Y., and Tan, B. (2019). On the role of the eastern Pacific teleconnection in ENSO impacts on wintertime weather over East Asia and North America. J. Clim. 32 (4), 1217–1234. doi:10.1175/JCLI-D-17-0789.1
Ding, S., Chen, W., Feng, J., and Graf, H.-F. (2017a). Combined impacts of PDO and two types of La Niña on climate anomalies in europe. J. Clim. 30 (9), 3253–3278. doi:10.1175/jcli-d-16-0376.1
Ding, S., Chen, W., Graf, H.-F., Guo, Y., and Nath, D. (2017b). Distinct winter patterns of tropical Pacific convection anomaly and the associated extratropical wave trains in the Northern Hemisphere. Clim. Dyn. 51 (5-6), 2003–2022. doi:10.1007/s00382-017-3995-0
Ding, Y. (1994). “The winter monsoon in East Asia,” in Monsoons over China. Editor D. Yihui (Dordrecht: Springer Netherlands), 91–173.
Eyring, V., Bony, S., Meehl, G. A., Senior, C. A., Stevens, B., Stouffer, R. J., et al. (2016). Overview of the coupled model Intercomparison Project phase 6 (CMIP6) experimental design and organization. Geosci. Model Dev. 9 (5), 1937–1958. doi:10.5194/gmd-9-1937-2016
Feng, J., Chen, W., and Li, Y. (2017). Asymmetry of the winter extra-tropical teleconnections in the Northern Hemisphere associated with two types of ENSO. Clim. Dyn. 48 (7), 2135–2151. doi:10.1007/s00382-016-3196-2
Feng, J., and Chen, W. (2022). Respective and combined impacts of North Indian ocean and tropical North Atlantic SST anomalies on the subseasonal evolution of anomalous western North Pacific anticyclones. J. Clim. 35 (17), 5623–5636. doi:10.1175/JCLI-D-21-0799.1
Feng, J., Lian, T., Ding, Y., Li, X., Sun, C., and Chen, D. (2022). Two types of the East asian cold surge and their impacts on El Niño. Geophys. Res. Lett. 49 (3). doi:10.1029/2021GL096108
Feng, J., Wang, L., Chen, W., Fong, S. K., and Leong, K. C. (2010). Different impacts of two types of Pacific Ocean warming on Southeast Asian rainfall during boreal winter. J. Geophys. Research-Atmospheres 115. doi:10.1029/2010jd014761
Feng, J., Wang, L., and Chen, W. (2014). How does the East asian summer monsoon behave in the decaying phase of El nino during different PDO phases? J. Clim. 27 (7), 2682–2698. doi:10.1175/jcli-d-13-00015.1
Gao, R., Zhang, R., Wen, M., and Li, T. (2019). Interdecadal changes in the asymmetric impacts of ENSO on wintertime rainfall over China and atmospheric circulations over Western North Pacific. Clim. Dyn. 52 (12), 7525–7536. doi:10.1007/s00382-018-4282-4
Gong, H., Wang, L., Chen, W., Nath, D., Huang, G., and Tao, W. (2015). Diverse influences of ENSO on the East asian-western pacific winter climate tied to different ENSO properties in CMIP5 models. J. Clim. 28 (6), 2187–2202. doi:10.1175/jcli-d-14-00405.1
Gong, H., Wang, L., Chen, W., Wu, R., Wei, K., and Cui, X. (2014). The climatology and interannual variability of the East Asian winter Monsoon in CMIP5 models. J. Clim. 27 (4), 1659–1678. doi:10.1175/JCLI-D-13-00039.1
Gong, H., Wang, L., Zhou, W., Chen, W., Wu, R., Liu, L., et al. (2018). Revisiting the northern mode of East Asian Winter Monsoon variation and its response to global warming. J. Clim. 31 (21), 9001–9014. doi:10.1175/JCLI-D-18-0136.1
Horel, J. D., and Wallace, J. M. (1981). Planetary-scale atmospheric phenomena associated with the southern oscillation. Mon. Weather Rev. 109 (4), 813–829. doi:10.1175/1520-0493(1981)109<0813:psapaw>2.0.co;2
Hu, K., Huang, G., Huang, P., Kosaka, Y., and Xie, S. P. (2021). Intensification of El Niño-induced atmospheric anomalies under greenhouse warming. Nat. Geosci. 14 (6), 377–382. doi:10.1038/s41561-021-00730-3
Hu, K. M., Huang, G., Wu, R. G., and Wang, L. (2018). Structure and dynamics of a wave train along the wintertime Asian jet and its impact on East Asian climate. Clim. Dyn. 51 (11-12), 4123–4137. doi:10.1007/s00382-017-3674-1
Huang, F., Xu, Z., and Guo, W. (2019). Evaluating vector winds in the Asian-Australian monsoon region simulated by 37 CMIP5 models. Clim. Dyn. 53 (1-2), 491–507. doi:10.1007/s00382-018-4599-z
Huang, R., Chen, S., Chen, W., Yu, B., Hu, P., Ying, J., et al. (2021). Northern poleward edge of regional Hadley cell over Western pacific during boreal winter: Year-to-year variability, influence factors and associated winter climate anomalies. Clim. Dyn. 56 (11-12), 3643–3664. doi:10.1007/s00382-021-05660-9
Huang, R. H., Chen, W., Yang, B. L., and Zhang, R. H. (2004). Recent advances in studies of the interaction between the east Asian winter and summer monsoons and ENSO cycle. Adv. Atmos. Sci. 21 (3), 407–424. doi:10.1007/bf02915568
Huang, R., Zang, X., Zhang, R., and Chen, J. (1998). The westerly anomalies over the tropical pacific and their dynamical effect on the ENSO cycles during 1980-1994. Adv. Atmos. Sci. 15 (2), 135–151. doi:10.1007/s00376-998-0035-3
Iwasaki, T., Shoji, T., Kanno, Y., Sawada, M., Ujiie, M., and Takaya, K. (2014). Isentropic analysis of polar cold airmass streams in the northern hemispheric winter. J. Atmos. Sci. 71 (6), 2230–2243. doi:10.1175/jas-d-13-058.1
Jia, X., and Ge, J. (2017a). Modulation of the PDO to the relationship between moderate ENSO events and the winter climate over North America. Int. J. Climatol. 37 (12), 4275–4287. doi:10.1002/joc.5083
Jia, X., Ge, J., and Wang, S. (2016). Diverse impacts of ENSO on wintertime rainfall over the Maritime Continent. Int. J. Climatol. 36 (9), 3384–3397. doi:10.1002/joc.4562
Jia, X. J., and Ge, J. W. (2017b). Interdecadal changes in the relationship between ENSO, EAWM, and the wintertime precipitation over China at the end of the twentieth century. J. Clim. 30 (6), 1923–1937. doi:10.1175/jcli-d-16-0422.1
Jiang, D., Hu, D., Tian, Z., and Lang, X. (2020). Differences between CMIP6 and CMIP5 models in simulating climate over China and the East asian monsoon. Adv. Atmos. Sci. 37 (10), 1102–1118. doi:10.1007/s00376-020-2034-y
Jiang, W., Gong, H., Huang, P., Wang, L., Huang, G., and Hu, L. (2022). Biases and improvements of the ENSO-East Asian winter monsoon teleconnection in CMIP5 and CMIP6 models. Clim. Dyn. 59 (7-8), 2467–2480. doi:10.1007/s00382-022-06220-5
Jiao, Y., and Wu, R. (2019). Propagation and influence on tropical precipitation of intraseasonal variation over mid-latitude East Asia in boreal winter. Atmos. Ocean. Sci. Lett. 12, 155–161. doi:10.1080/16742834.2019.1586518
Jiao, Y., Wu, R., and Song, L. (2019). Individual and combined impacts of two eurasian wave trains on intraseasonal East Asian winter monsoon variability. J. Geophys. Res. Atmos. 124 (8), 4530–4548. doi:10.1029/2018JD029953
Kim, J. W., An, S. I., Jun, S. Y., Park, H. J., and Yeh, S. W. (2017a). ENSO and East Asian winter monsoon relationship modulation associated with the anomalous northwest Pacific anticyclone. Clim. Dyn. 49 (4), 1157–1179. doi:10.1007/s00382-016-3371-5
Kim, J. W., Chang, T. H., Lee, C. T., and Yu, J. Y. (2021). On the varying responses of east Asian winter monsoon to three types of El Niño: Observations and model hindcasts. J. Clim. 34 (10), 4089–4101. doi:10.1175/JCLI-D-20-0784.1
Kim, S., Son, H. Y., and Kug, J. S. (2017b). How well do climate models simulate atmospheric teleconnctions over the North Pacific and East Asia associated with ENSO? Clim. Dyn. 48 (3-4), 971–985. doi:10.1007/s00382-016-3121-8
Kim, S., Son, H. Y., and Kug, J. S. (2018). Relative roles of equatorial central Pacific and Western North Pacific precipitation anomalies in ENSO teleconnection over the North Pacific. Clim. Dyn. 51 (11-12), 4345–4355. doi:10.1007/s00382-017-3779-6
Kim, S. T., and Yu, J. Y. (2012). The two types of ENSO in CMIP5 models. Geophys. Res. Lett. 39 (11). doi:10.1029/2012GL052006
Kug, J. S., Ham, Y. G., Lee, J. Y., and Jin, F. F. (2012). Improved simulation of two types of El Niño in CMIP5 models. Environ. Res. Lett. 7 (3), 034002. doi:10.1088/1748-9326/7/3/034002
Lau, N. C., and Lau, K. M. (1984). The structure and energetics of midlatitude disturbances accompanying cold-air outbreaks over East Asia. Mon. Weather Rev. 1122 (7), 1309–1327. doi:10.1175/1520-0493(1984)112<1309:tsaeom>2.0.co;2
Li, C. (1989). Frequent activities of stronger areotroughs in East Asia in wintertime and the occurrence of the El Nino event. Sci. China Ser. B-Chemistry 32 (8), 976–985.
Li, C. (1990). Interaction between anomalous winter monsoon in East Asia and El nino events. Adv. Atmos. Sci. 7 (1), 36–46. doi:10.1007/BF02919166
Li, C., and Mu, M. (2000). Relationship between East Asian winter monsoon, warm pool situation and ENSO cycle. Chin. Sci. Bull. 45 (16), 1448–1455. doi:10.1007/BF02898885
Li, H., Fan, K., He, S., Liu, Y., Yuan, X., and Wang, H. (2021). Intensified impacts of central pacific ENSO on the reversal of december and january surface air temperature anomaly over China since 1997. J. Clim. 34 (5), 1601–1618. doi:10.1175/jcli-d-20-0048.1
Li, Y., and Yang, S. (2017). Feedback attributions to the dominant modes of EastEast Asian winter monsoon variations. J. Clim. 30 (3), 905–920. doi:10.1175/jcli-d-16-0275.1
Liu, Q., Chen, G., Wang, L., Kanno, Y., and Iwasaki, T. (2021). Southward cold airmass flux associated with the East asian winter monsoon: Diversity and impacts. J. Clim. 34 (8), 1–37. doi:10.1175/jcli-d-20-0319.1
Luo, B., Luo, D., Dai, A., Simmonds, I., and Wu, L. (2021). A connection of winter eurasian cold anomaly to the modulation of ural blocking by ENSO. Geophys. Res. Lett. 48 (17). doi:10.1029/2021GL094304
Ma, T., Chen, W., Chen, S., Garfinkel, C. I., Ding, S., Song, L., et al. (2022). Different ENSO teleconnections over East Asia in early and late winter: Role of precipitation anomalies in the tropical Indian ocean and far western pacific. J. Clim. 35, 4319–4335. doi:10.1175/JCLI-D-21-0805.1
Ma, T., and Chen, W. (2021). Climate variability of the East asian winter monsoon and associated extratropical–tropical interaction: A review. Ann. N. Y. Acad. Sci. 1504, 44–62. doi:10.1111/nyas.14620
Ma, T., Chen, W., Feng, J., and Wu, R. (2018a). Modulation effects of the East Asian winter monsoon on El Nino-related rainfall anomalies in southeastern China. Sci. Rep. 8 (1), 14107. doi:10.1038/s41598-018-32492-1
Ma, T., Chen, W., Graf, H.-F., Ding, S., Xu, P., Song, L., et al. (2020). Different impacts of the East asian winter monsoon on the surface air temperature in NorthNorth America during ENSO and neutral ENSO years. J. Clim. 33 (24), 10671–10690. doi:10.1175/jcli-d-18-0760.1
Ma, T., Chen, W., Nath, D., Graf, H. F., Wang, L., and Huangfu, J. (2018b). East Asian winter monsoon impacts the ENSO-related teleconnections and NorthNorth American seasonal air temperature prediction. Sci. Rep. 8 (1), 6547. doi:10.1038/s41598-018-24552-3
Newman, M., Alexander, M. A., Ault, T. R., Cobb, K. M., Deser, C., Di Lorenzo, E., et al. (2016). The pacific decadal oscillation, revisited. J. Clim. 29 (12), 4399–4427. doi:10.1175/Jcli-D-15-0508.1
Nitta, T. (1987). Convective activities in the tropical Western Pacific and their impact on the northern hemisphere summer circulation. J. Meteorological Soc. Jpn. 65 (3), 373–390. doi:10.2151/jmsj1965.65.3_373
Son, H. Y., Park, J. Y., Kug, J. S., Yoo, J., and Kim, C. H. (2014). Winter precipitation variability over Korean Peninsula associated with ENSO. Clim. Dyn. 42 (11-12), 3171–3186. doi:10.1007/s00382-013-2008-1
Song, L., and Wu, R. (2022). Different processes of occurrence of cold events over East Asia in El Niño and La Niña winters. Clim. Dyn. 58 (11-12), 3139–3154. doi:10.1007/s00382-021-06082-3
Staff Members of the Academia Sinica (1957). On the general circulation over eastern Asia (I). Tellus 9 (4), 432–446. doi:10.1111/j.2153-3490.1957.tb01903.x
Tao, S., and Zhang, Q. (1998). Response of the asian winter and summer monsoon to ENSO events. Chin. J. Atmos. Sci. 22 (4), 399–407.
Taylor, K. E., Stouffer, R. J., and Meehl, G. A. (2012). An overview of CMIP5 and the experiment design. Bull. Am. Meteorological Soc. 93 (4), 485–498. doi:10.1175/bams-d-11-00094.1
Tian, B. Q., and Fan, K. (2020). Different prediction skill for the East Asian winter monsoon in the early and late winter season. Clim. Dyn. 54 (3-4), 1523–1538. doi:10.1007/s00382-019-05068-6
Trenberth, K. E., Branstator, G. W., Karoly, D., Kumar, A., Lau, N. C., and Ropelewski, C. (1998). Progress during TOGA in understanding and modeling global teleconnections associated with tropical sea surface temperatures. J. Geophys. Research-Oceans 103 (C7), 14291–14324. doi:10.1029/97jc01444
Wang, B., Wu, R. G., and Fu, X. H. (2000). Pacific-East asian teleconnection: How does ENSO affect East Asian climate? J. Clim. 13 (9), 1517–1536. doi:10.1175/1520-0442(2000)013<1517:peathd>2.0.co;2
Wang, C., and Wang, X. (2013). Classifying El Niño modoki I and II by different impacts on rainfall in southern China and typhoon tracks. J. Clim. 26 (4), 1322–1338. doi:10.1175/jcli-d-12-00107.1
Wang, L., and Chen, W. (2010). How well do existing indices measure the strength of the East Asian winter monsoon? Adv. Atmos. Sci. 27 (4), 855–870. doi:10.1007/s00376-009-9094-3
Wang, L., Chen, W., and Huang, R. (2008). Interdecadal modulation of PDO on the impact of ENSO on the east Asian winter monsoon. Geophys. Res. Lett. 35 (20), L20702. doi:10.1029/2008GL035287
Wang, L., Deng, A., and Huang, R. (2019a). Wintertime internal climate variability over Eurasia in the CESM large ensemble. Clim. Dyn. 52 (11), 6735–6748. doi:10.1007/s00382-018-4542-3
Wang, L., and Lu, M. M. (2017). “The East asian winter monsoon,” in World scientific series on asia-pacific weather and climate.
Wang, Q., Cai, W., Zhong, W., Zeng, L., Wu, L., and Wang, D. (2019b). Response of southern China winter rainfall to El Niño diversity and its relevance to projected southern China rainfall change. J. Clim. 32 (11), 3343–3356. doi:10.1175/JCLI-D-18-0571.1
Wang, Z., Wu, R., Gong, H., Jia, X., and Dai, P. (2022a). What determine the performance of the ENSO-East Asian winter monsoon relationship in CMIP6 models? J. Geophys. Res. Atmos. 127 (6). doi:10.1029/2021JD036227
Wang, Z., Wu, R., and Wang, Y. (2022b). Impacts of the East asian winter monsoon on winter precipitation variability over East asia-Western North Pacific. Clim. Dyn. 58 (11-12), 3041–3055. doi:10.1007/s00382-021-06086-z
Wei, W., Wang, L., Chen, Q., Liu, Y., and Li, Z. (2020). Definition of early and late winter and associated interannual variations of surface air temperature in China. Chin. J. Atmos. Sci. 44 (1), 122–137. doi:10.3878/j.issn.1006-9895.1904.18238
Weng, H., Behera, S. K., and Yamagata, T. (2009). Anomalous winter climate conditions in the pacific rim during recent El Niño modoki and El Niño events. Clim. Dyn. 32 (5), 663–674. doi:10.1007/s00382-008-0394-6
Wu, B., Li, T., and Zhou, T. (2010). Asymmetry of atmospheric circulation anomalies over the western North Pacific between El Niño and La Niña. J. Clim. 23 (18), 4807–4822. doi:10.1175/2010jcli3222.1
Wu, R., Chen, W., Wang, G., and Hu, K. (2014). Relative contribution of ENSO and East Asian winter monsoon to the South China Sea SST anomalies during ENSO decaying years. J. Geophys. Res. Atmos. 119 (9), 5046–5064. doi:10.1002/2013JD021095
Wu, R., Hu, Z. Z., and Kirtman, B. P. (2003). Evolution of ENSO-related rainfall anomalies in East Asia. J. Clim. 16 (22), 3742–3758. doi:10.1175/1520-0442(2003)016<3742:EOERAI>2.0.CO;2
Yeh, S. W., Cai, W., Min, S. K., McPhaden, M. J., Dommenget, D., Dewitte, B., et al. (2018). ENSO atmospheric teleconnections and their response to greenhouse gas forcing. Rev. Geophys. 56 (1), 185–206. doi:10.1002/2017RG000568
Yu, S., and Sun, J. (2020). Potential factors modulating ENSO's influences on the East Asian trough in boreal winter. Int. J. Climatol. 40 (12), 5066–5083. doi:10.1002/joc.6505
Yu, S., and Sun, J. (2018). Revisiting the relationship between El niño-southern oscillation and the East asian winter monsoon. Int. J. Climatol. 38 (13), 4846–4859. doi:10.1002/joc.5702
Yu, Y., Li, Y., Ren, R., Cai, M., Guan, Z., and Huang, W. (2022). An isentropic mass circulation view on the extreme cold events in the 2020/21 winter. Adv. Atmos. Sci. 39 (4), 643–657. doi:10.1007/s00376-021-1289-2
Zhang, R. H., Li, T. R., Wen, M., and Liu, L. K. (2015). Role of intraseasonal oscillation in asymmetric impacts of El Nino and La Nina on the rainfall over southern China in boreal winter. Clim. Dyn. 45 (3-4), 559–567. doi:10.1007/s00382-014-2207-4
Zhang, R., Min, Q., and Su, J. (2017). Impact of El nino on atmospheric circulations over East Asia and rainfall in China: Role of the anomalous Western North Pacific anticyclone. Sci. China-Earth Sci. 60 (6), 1124–1132. doi:10.1007/s11430-016-9026-x
Zhang, R., Sumi, A., and Kimoto, M. (1996). Impact of El Niño on the East asian monsoon: A diagnostic study of the '86/87 and '91/92 events. J. Meteorological Soc. Jpn. 74 (1), 49–62. doi:10.2151/jmsj1965.74.1_49
Zhang X. D., X. D., Fu, Y. F., Han, Z., Overland, J. E., Rinke, A., Tang, H., et al. (2022). Extreme cold events from East Asia to North America in winter 2020/21: Comparisons, causes, and future implications. Adv. Atmos. Sci. 39 (4), 553–565. doi:10.1007/s00376-021-1229-1
Zhang Y., Y., Zhou, W., Wang, X., Chen, S., Chen, J., and Li, S. (2022). Indian Ocean Dipole and ENSO’s mechanistic importance in modulating the ensuing-summer precipitation over Eastern China. npj Clim. Atmos. Sci. 5 (1), 48. doi:10.1038/s41612-022-00271-5
Zhao, W., Chen, S., Zhang, H., Wang, J., Chen, W., Wu, R., et al. (2022). Distinct impacts of ENSO on haze pollution in the beijing–tianjin–hebei region between early and late winters. J. Clim. 35 (2), 687–704. doi:10.1175/JCLI-D-21-0459.1
Zheng, Y., Zhang, R., and Bourassa, M. A. (2014). Impact of east Asian winter and Australian summer monsoons on the enhanced surface westerlies over the Western tropical Pacific ocean preceding the El Niño onset. J. Clim. 27 (5), 1928–1944. doi:10.1175/JCLI-D-13-00369.1
Keywords: East Asian winter monsoon, El Niño–Southern Oscillation (ENSO), CMIP5, CMIP6, extratropical-tropical interactions
Citation: Ma T and Chen W (2023) Recent progress in understanding the interaction between ENSO and the East Asian winter monsoon: A review. Front. Earth Sci. 11:1098517. doi: 10.3389/feart.2023.1098517
Received: 15 November 2022; Accepted: 10 January 2023;
Published: 20 January 2023.
Edited by:
Steven L. Forman, Baylor University, United StatesCopyright © 2023 Ma and Chen. This is an open-access article distributed under the terms of the Creative Commons Attribution License (CC BY). The use, distribution or reproduction in other forums is permitted, provided the original author(s) and the copyright owner(s) are credited and that the original publication in this journal is cited, in accordance with accepted academic practice. No use, distribution or reproduction is permitted which does not comply with these terms.
*Correspondence: Wen Chen, chenwen-dq@ynu.edu.cn