- 1Department of Earth Sciences, Indian Institute of Technology, Kanpur, India
- 2Department of Civil Engineering, L. D. College of Engineering, Ahmedabad, India
- 3National Center for Seismology, Ministry of Earth Sciences, New Delhi, India
In recent years, the National Capital Region (NCR) of Delhi has experienced several earthquakes ranging in magnitude from 1.0 to 6.7. According to the last 50 years of earthquake data, the majority of earthquakes in the NCR have occurred near the Mahendragarh Dehradun Fault (MDF) and the Sohna Fault (SF). The region is bounded by a number of subsurface Ridges, Faults, and Lineaments, which are also influenced by the active plate boundary of the Indian and Eurasian plates. Active fault mapping is critical for the precise identification and marking of active fault traces in the NCR area for a precise seismic hazard assessment. We used high resolution Cartosat-1 stereopair data obtained from NRSC, Hyderabad, and Anaglyph (A 3D representation of the surface) and DEM prepared with ENVI software to map the active faults. We identified 12 sites in the NCR region based on satellite data interpretation, primarily along the MDF and Sohna Fault and their extensions. The presence of tectono-geomorphic markers along the MDF and Sohna Fault, such as warped surfaces indicative of fault scarps, stream offsets, gully erosion, and sag ponds, suggests active tectonic movement along these faults, most likely in the recent geological past. We believe the MDF is a right-lateral strike-slip fault with a compressional component on the western side and an extensional component on the eastern side. It acts as a segment boundary between compressional and extensional boundaries. We also identified the right lateral Nuh-Jhirka fault (NJF), which can be the Sohna Fault’s southern extension from Nuh to Jhirka. The western limb of the Delhi Mega fold has also seen a few right-lateral strike-slip movements that have extended up to the eastern bank of the Yamuna River, where the river reflects the base-level change and tight meandering on its upward side and a straight pattern on its downward side. This fault is known as the Delhi Fault (DF). The findings are preliminary, and further research would be required to create a detailed active fault map of the Delhi-NCR region to conduct a precise Seismic Hazard Assessment (SHA) of the region.
1 Introduction
Large magnitude earthquakes are thought to be caused by active faults. As a result, identifying, characterising, and deciphering their seismic potential is critical for a precise Seismic Hazard Assessment (SHA) of any region. The seismic vulnerability of India is well known as more than 60% of its area lies in high hazard zones due to the presence of major active faults in its plate boundaries and continental interiors, which have produced significant earthquakes in the past and have the potential to generate major earthquakes in future. Previous studies in the Indian peninsula beneath the Ganga plains have identified NE-SW trending basement ridges (Sastri et al., 1971). These ridges are known as the Delhi-Haridwar Ridge (DHR), the Faizabad Ridge (FR), and the Munger-Saharsa Ridge (MSR) from west to east (Figure 1).
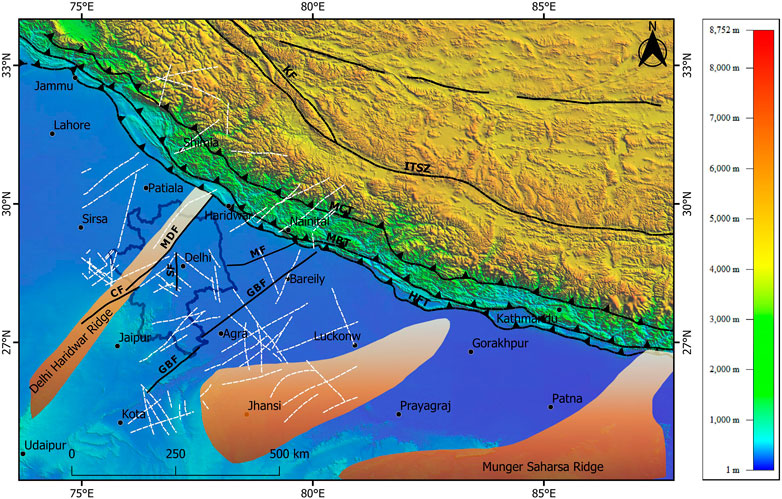
FIGURE 1. The map showing the location of Delhi-NCR on a regional scale in context with the Himalayan Orogeny Belt along with the Indo-Gangetic Plain in the north. NNE-SSW trending major subsurface ridges, i.e., Delhi-Haridwar Ridge, Faizabad Ridge, and Munger Saharsa Ridge, in the Indo-Gangetic Plains, are marked as orange shades. All faults and lineaments are marked with black and white lines, respectively. Major Himalayan thrust and faults are marked as solid black color lines with triangle symbols and dashed black color lines, respectively. Delhi-NCR outer boundary is marked with solid blue line. GBF- Great Boundary Fault; MDF - Mahendragarh Dehradun Fault; SF- Sohna Fault; CF- Chahpoli Fault; MF- Moradabad Fault; HFT - Himalayan Frontal Thrust; MBT - Main Boundary Thrust; MCT - Main Central Thrust; KF- Karakoram Fault; ITSZ - Indo Tsangpo Suture Zone.
The National Capital Territory (NCT) is being developed, and it consists of a few districts from Rajasthan, Uttar Pradesh, and Haryana, covering an area of 55,083 square kilometres, with Delhi and the National Capital Region (Delhi-NCR) at its heart. In the past, Delhi-NCR has been subjected to several moderate-magnitude earthquakes, as well as a few minor earthquakes. The Delhi-NCR region is classified as Zone IV on the Indian Seismic Zoning Map (Bureau of Indian Standards, 2016). The region is ∼260 km from the Himalayan Belt, which is one of the world’s most seismically active zones (Figure 1). Geologically, the Indo-Gangetic Plain and Himalayan Belt border it to the north, the Bundelkhand Complex to the east, and the NNE-SSW striking Aravalli Fold Belt to the west (Figure 2). A few historical earthquakes have been reported from the Delhi-NCR region (Table 1) (Figure 2), including (a) 15 July 1720, with Mw 6.5 and seismic intensity reaching X; and (b) 27 August 1960, with Mw 6.0. (Chandra, 1978). However, the occurrence of small magnitude earthquakes in the last 2 years (2020–2022) in the Delhi-NCR area has caused concern and raised many questions, including (a) Are these earthquakes indicative of a large magnitude earthquake in the near future? (b) Are these tremors? (c) Or is this just a normal phenomenon caused by ongoing deformation between the Indian and Eurasian plates in the region? In particular, the Indo-Gangetic Plain and the Delhi-NCR region have been affected by Himalayan earthquakes; therefore, if another moderated-large magnitude earthquake occurs in the Himalaya, the Indo-Gangetic Plain and the Delhi-NCR regions will be severely affected by strong seismic shaking.
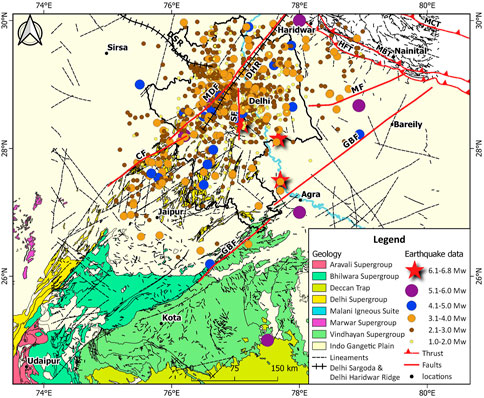
FIGURE 2. The simplified geological map along with various faults, lineaments, and extensional and compressional features in Delhi-NCR. Along with seismicity of the area in and around the Delhi-NCR. The magnitude of earthquakes ranges from Mw 1.0 to Mw 6.8. Black dashed lines are the lineaments marked in the region. Delhi-NCR outer boundary is marked with a solid white polygon. Yamuna river is marked with blue solid line. The geological and tectonic features are from BHUKOSH, Geological Survey of India (http://bhukosh.gsi.gov.in/Bhukosh/MapViewer.aspx). Earthquake epicenter data is from 1720 to 2020, downloaded from NCS, New Delhi (https://riseq.seismo.gov.in/riseq/earthquake/archive). DSR-Delhi Sargoda Ridge; DHR- Delhi-Haridwar Ridge; MDF- Mahendragarh Dehradun Fault; GBF- Great Boundary Fault; MF- Moradabad Fault; CF- Chahpoli Fault; SF- Sohna Fault; HFT- Himalayan Frontal Thrust; MBT- Main Boundary Thrust; MCT- Main Central Thrust.
The seismicity of the Delhi Aravalli Fold Belt (DAFB) is still debatable. However, the relative movement of the Marwar Craton to the west and the Buldenkhand Craton to the east of the ADFB is caused by the seismicity of the region (Yadav et al., 2022). The intraplate seismicity caused by strain in the Indian lithosphere due to its northward convergence with Eurasia may be attributed to such small earthquakes that frequently occur in this region. Delhi and its surrounding regions have prominent NNE-SSW oriented subsurface Delhi-Haridwar Ridge (DHR), and NNW-SSE oriented Delhi Sargoda Ridge (DSR). Alternatively, these earthquakes appear to be along these subsurface ridges, which are also subducting (part of the Indian Plate) beneath the Eurasian Plate along the active plate boundary. DHR and DSR are seismically active and believed to have generated seismicity in the past (Bansal and Verma, 2012; Bansal et al., 2021a). Furthermore, some prominent geological faults and lineaments also pass through the region, such as the Mahendragarh Dehradun Fault (MDF) and Sohna Fault (SF) (Figure 2). These faults represent the basement fault formed before the indo-Eurasia collision (Gahalaut and Kundu, 2012). The seismicity in the Delhi-NCR is scattered, and it is difficult to correlate the seismicity to a specific fault or lineament. There may be a possibility that several lineaments are seismically active (Verma et al., 1995). However, the occurrence of earthquakes indicates the reactivations of these faults in recent times. Tremendous efforts have been made to understand the kinematics of the faults and associated earthquakes based on fault plane solutions in Delhi and its surrounding regions. However, there is a lack of information about the surface morphology of the faults in Delhi-NCR. Understanding the seismic pattern and distribution of probable active faults that could generate future earthquakes is crucial for the Delhi-NCR as the population growth and infrastructure development in the region are at their peak. In this study, we have visited the epicentral region of some of the prominent earthquakes of Delhi-NCR and mapped the faults based on the satellite data interpretation and morphological behavior of the faults. Tectono-Geomorphic markers such as warped surfaces indicative of fault scarps, stream offsets, gully erosion, and sag ponds observed across the faults are also correlated with fault plane solutions by previous studies and proposed a tectonic model for the Delhi-NCR.
2 Geology and tectonics
Delhi NCR is a part of the Delhi Aravalli Fold Belt (DAFB). It is bounded by the Indo- Gangetic alluvial plains in the north and the Delhi Aravalli mountain ranges to the south. The geological history of the DAFB can be categorized into four tectono-magmatic and metamorphic events: Bhilwara Gneissic Complex (BGC), Aravalli Orogeny, Delhi Orogeny, and post-Delhi magmatic events (Heron, 1953). The Aravalli-Delhi sediments were deposited over the oldest Archean basement of the Bhilwara Gneissic Complex (Sinha-Roy et al., 1994). The Delhi-NCR and its surrounding regions are situated on the Proterozoic folded sequence of meta-sedimentary quartzite and metapelite rock of the Delhi Fold Belt (DFB) or Delhi Aravalli Fold Belt (DAFB). DAFB comprises over 10 km thick volcano-sedimentary layers and comprises a series of half grabens and horsts (Sinha - Roy, 1988). The DAFB is the northern extension of the Alwar Series of the Aravalli Range, extending in the NNE direction from Rajasthan (Roy, 1988; Sugden et al., 1990). The Alwar Series is overlain and surrounded by alluvium of the Quaternary age. The Delhi Aravalli Fold Belt is a region where, during the Proterozoic, the eastward underthrusting of an intermediate oceanic crust was caused by the westward motion of an Archaean craton and its collision with another craton to the west (Sinha-Roy, 1988). This movement suggests a two-stage rifting. The Aravalli Supergroup and the Delhi Supergroup were created because the sediments were deformed and transformed during the compressional phase, which sealed the rift. At the end of the Proterozoic (750–850 Ma), the Delhi Supergroup had another episode of tectono-magmatic deformation that produced an igneous suite and intrusive granites (Roy, 1988). The Aravalli Supergroup can be found in the south and overlain by Delhi Supergroup rocks, which run the length of the DAFB from Mount Abu in the south to the Delhi-NCR region in the north (Gupta et al., 1980). Along the length of the DAFB between Delhi and Mount Abu, geological formations on the surface and subsurface have been mapped (Vijaya Rao et al., 2000; Mandal et al., 2018). The rocks in Delhi have undergone numerous folding processes and different stages of metamorphism. The DAFB is bounded to the east by the Great Boundary Fault with a Strike-Slip nature and to the west by Mahendragarh Dehradun Fault (MDF). The region is also surrounded by the prominent NNE-SSW subsurface Delhi-Haridwar Ridge (DHR), NNW-SSE Delhi Sargoda Ridge (DSR) (Figure 2), which is subparallel to the Himalayan thrusts and fold belt and is probably forming a bulge on the Indo-Gangetic Basin. These ridges form a tri-junction around the Delhi region (Bansal et al., 2021a). Most of the part of Delhi-NCR is comprised of quaternary sediments of older and newer alluvium along with the thick sediments deposits of Yamuna plains, making it a potential region for ground motion amplification during earthquakes (Srivastava, 1988; Verma et al., 2014). Apart form the quaternary alluvium quartzite is dominant in delhi-NCR (Gupta and Rao, 2000; Bansal et al., 2009). Delhi-NCR is comprised of ridges and faults aligned in the NNE-SSW direction, with a few faults/lineaments striking in N-S (Figure 2). Some prominent geological faults passing through the region are Mahendragarh Dehradun Fault (MDF), Sohna Fault (SF), Mathura Fault, and Moradabad Fault. Along with these faults, several NW-SE and N-S trending subsurface and basement faults also exist (Figure 2). Drilling, geophysical surveying, and an aeromagnetic survey have all been used by Oil and Natural Gas Corporation (ONGC) and Geological Survey of India (GSI) to map these faults (Sastri et al., 1971; Karunakaran and Ranga Rao, 1976). MDF is a NE-SW trending, 295 km long fault connected to the Peninsular Craton to the south and the Himalayan Frontal Thrust (HFT) to the north (Geological Survey of India, 2000). The Delhi region also has several subsurface faults that have been marked based on data from ground penetrating radar (GPR) and bouger gravity anomaly. Dubey et al. (2012) identified three NW-SE trending reverse faults in the Delhi region. Several shallow steep vertical faults were observed close to the MDF using a GPR survey, however, MDF was not traced (Patel et al., 2020). In contrast to the thoroughly studied Munger-Saharsa Ridge, deformation of the Indo-Gangetic plains caused by the ADFB ridge and its relationship with the Himalayan arc is relatively poorly understood (Duvall et al., 2020).
3 Seismicity
The Himalayan Orogeny to the north and the NNE-SSW striking Delhi Aravalli Fold Belt (DAFB) to the west both had a significant impact on the complex tectonic framework of the Delhi-NCR. In comparison to the Himalayan region, the area around Delhi-NCR and Rajasthan has a poor historical seismicity record. The DAFB is bounded in the west and east by Mahendragarh Dehradun Fault and Great Boundary Fault (GBF). Low seismicity has occurred on the eastern flank of the DAFB, where the faults are highly developed and have distinctive geomorphic expressions (Mandal et al., 2018). Instead, along the western side of the DAFB where seismicity seems to occur on poorly mapped or unmapped faults (Yadav et al., 2022)., A few earthquakes with magnitudes ranging from Mw 5.3 to Mw 6.7 have been reported from Delhi-NCR in the past. (Table 1). The available historical data suggest that on 26 July 1720, Delhi was struck by a deadly and locally devastating earthquake. The 1720 earthquake is documented in KhaB Khan’s Muntakhab-al-lubab (Iyengar, 2000). Though the precise location of the 1720 earthquakes is unknown, it is believed to be in the vicinity of Delhi (Iyengar and Ghosh, 2004) (Figure 2). Another well instrumentally recorded earthquake in 1960, Gurgaon (Now Gurugram), occurred in the Delhi region, although the location and magnitude of the event are debated. Simultaneously, the 1803 Mathura Earthquake is probably the one that occurred in the Himalaya (Ambraseys and Jackson, 2003; Ambraseys and Douglas, 2004; Bilham and Ambraseys, 2005; Rajendran and Rajendran, 2005; Szeliga et al., 2010; Malik et al., 2017; Bilham et al., 2019; Bansal et al., 2021a). However, Oldham (1883); Chandra (1978) suggested the epicenter of the 1803 earthquake in Mathura. Aside from these events, the occurrence of the remaining events is unambiguous and is thought to occur in the vicinity of Delhi-NCR.
Epicenter data from past and recent earthquakes show clustering of events around the Rohtak and Delhi region (Source: National Center for Seismology, New Delhi) (Figure 2). The clustering indicates frequent earthquakes occurred along the Mahendragarh Dehradun Fault (MDF) in the Delhi-Rohtak area (Figure 2). Simultaneously, some seismicity was detected along the western limb of the Delhi Mega Fold, following upto the banks of the Yamuna river near Wazirabad. It is, therefore, essential to understand the pattern and distribution of this seismicity, as well as whether it is following any of the region’s active faults and whether there is any potential for feature earthquakes. To determine the relationship with the faulting pattern, available focal plane mechanisms were considered.
4 Recent seismicity and fault plane solution
Delhi-NCR has been the locus of some small-magnitude earthquakes (up to Mw 4.9) in the last 2 decades (Figure 3). At depths of 15 km and 8 km, respectively, two minor earthquakes occurred in the center of Delhi on 28 April 2001, and 18 March 2004. The strike of one of the nodal planes showed a NE-SW trend, which is consistent with the faults and lineaments mapped in the region. Waveform modeling and initial motion suggest that the fault plane solution for these events is normal faulting with a significant strike-slip component (Bansal et al., 2009) (Figure 3).
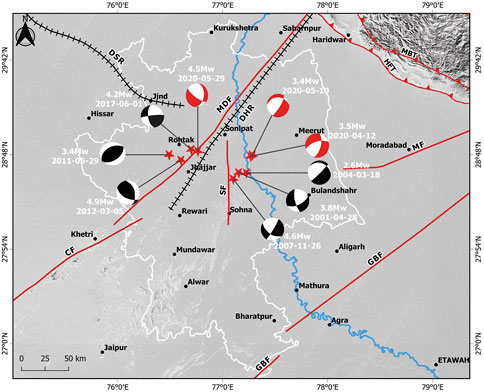
FIGURE 3. The map showing major earthquakes in Delhi-NCR in the last 2 decades. Fault plane solutions (Beach Ball) of the earthquakes is also plotted on the map. The epicenters of most of the earthquakes lay along the MDF and SF. Delhi-NCR region outer boundary is marked with solid white polygon. Yamuna river is marked with solid blue line. Blue triangles are the locations chosen for the field investigations. 1-Ghamroj Earth Dam; 2- Near Sector 56, Gurugram; 3- Asola Wildlife Sanctuary; 4- Yamuna Bank Near Khanpur Japti; 5- Quaternary Linear Ridge Rohtak; 6- Jhirka; 7- Mundawar; 8- Nuh; 9- Khetri; 10- Tirmarpur; 11- Wazirabad; 12- Kamla Nehru Ridge; 13- Baghpat. MDF- Mahendragarh Dehradun Fault; CF- Chahpoli Fault; SF- Sohna Fault; MF- Moradabad Fault; GBF- Great Boundary Fault; HFT- Himalayan Frontal Thrust; MBT- Main Boundary Thrust.
Between 2001 and 2004, approximately 288 earthquakes occurred throughout the NCR region. Visually, it is difficult to correlate these events to known faults. Approximately 19 earthquakes in Delhi and the Delhi Fold Belt area had a magnitude greater than Mw 3.0. These earthquakes’ fault plane solutions point to a dominant thrust mechanism with a strike-slip component (Shukla et al., 2007). The Mw 4.6 earthquake that struck Delhi on 26 November 2007, at a depth of 30 km, is the most notable local occurrence. It involved strike-slip faulting with some normal components, as opposed to thrust faulting with a minor strike-slip component previously recorded for the area (Singh et al., 2010). (Figure 3). The earthquakes on 29 May 2011, and 1 June 2017, had magnitudes of Mw 3.4 and Mw 4.2, respectively, and occurred west of MDF at a distance of about 18 km and 7 km, respectively. Bansal et al. (2021b) found that both earthquakes had a dominant reverse fault mechanism with strike slip components (Figure 3). On 5 March 2012, a Mw 4.9 magnitude earthquake struck northwest of Delhi near the Delhi-Haryana border. This earthquake’s epicentre is 4 km west of MDF. Bansal and Verma (2012) propose a strike-slip motion with a reverse component trending with a strike of N348° and a dip of 48° for this event. Three earthquakes with Mw 3.5, Mw 3.4, and Mw 4.5 shook Delhi and the surrounding areas on 12 April 2020, 10 May 2020, and 29 May 2020, respectively. The April 12 and May 10 quakes occurred on the eastern outskirts of the Delhi NCR region, while the May 29 quake occurred near Rohtak and MDF. The estimated fault plane solutions for the mainshocks of the April 12 and May 10 events indicate normal faulting with a strike-slip component (Pandey et al., 2020; Bansal et al., 2021a). (Figure 3). The focal mechanism is supported by the NE-SW oriented lineaments traced close to the epicentre. The earthquake on May 29 had a nodal plane with a dip between 37° and 75° and was oriented NNE-SSW; it was normal faulting with a strike-slip mechanism (Bansal et al., 2021a) (Figure 3).
5 Methodology
This study consists of a preliminary survey with the help of high-resolution 2.5 m CARTOSAT-1 Stereo-pair satellite data followed by field surveys in the region to identify the geomorphic markers of active faults. Accordingly, 149 scenes of CARTOSAT-1 data covering the Delhi-NCR were procured from National Remote Sensing Center (NRSC) Hyderabad (Supplementary Figure S1). The CARTOSAT-1 satellite is positioned in the polar Sun Synchronous Orbit, which has a radius of 618 km (616 km at perigee and 621 km at apogee) (Supplementary Table S1). It offers stereo images with a 27 km swath and 2.5 m nadir resolution. Two payloads, PAN-fore and PAN-aft, are created to deliver high-resolution images (Supplementary Table S2) (Srivastava et al., 2006; Nandakumar et al., 2008). These payloads are mounted with a fixed tilt of 26° (Fore) and 5° (Aft) to capture the along-track stereo image. The spatial resolution of CARTOSAT-1 is 2.5 m. Two types of images are produced by CARTOSAT-1. The first category is radiometrically corrected and georeferenced and is the Standard product. The second category, the orthorectified images, is called the precision product (NRSC).
The satellite data is processed using GIS software (ENVI), and anaglyphs (a 3D representation of the area) of different scenes of the data are generated. The satellite data processed in such a manner will help generate Digital Elevation Models (DEM) of the study area and 3D analysis of the region. Comprehensive analysis of such data can help identify active tectonic geomorphic features in the region. The stereo pair forward (BAND F) and afterward (BAND A) image of Cartosat-1 data is opened in ENVI with the Dem extraction module given in ENVI software. Rational Polynomial Coefficient (RPC) values (Metadata) help to orthorectify the images. The features that are identical on the afterward and forward stereo images are chosen to create the DEM. These points are known as tie points. The tie points can be manually or automatically added to increase the DEM resolution; the higher the number, the better will the resolution. The Y-parallax must be less than or close to 1.0 in order to generate the DEM and Anaglyph. Y-Parallax should be verified for each tie point. If Y-parallax is more than 1, the point should be eliminated, and a new tie point should be marked that closely resembles the same object in both images. We have marked almost 300 to 400 tie points for each cartosat scene to generate the Anaglyph.
6 Field investigation
Satellite data interpretations helped us identify active tectonic features like linear valleys, sag-ponds, offset streams, steeply dipping meta-sedimentary beds, elongated ridges, and fault scarps. Field excursions were organized after identifying a few field sites along the surface traces of the Mahendragarh Dehradun Fault (MDF) and Sohna Fault (SF) based on our early interpretations of the satellite data. In conjunction with published literature and observed seismicity from the region, our observations from satellite data helped us develop a basic understanding of the tectonic setup around Delhi-NCR. We conducted the field survey on 12 locations along the MDF, Sohna Fault, and Delhi Mega Fold (DMF) (Figure 4).
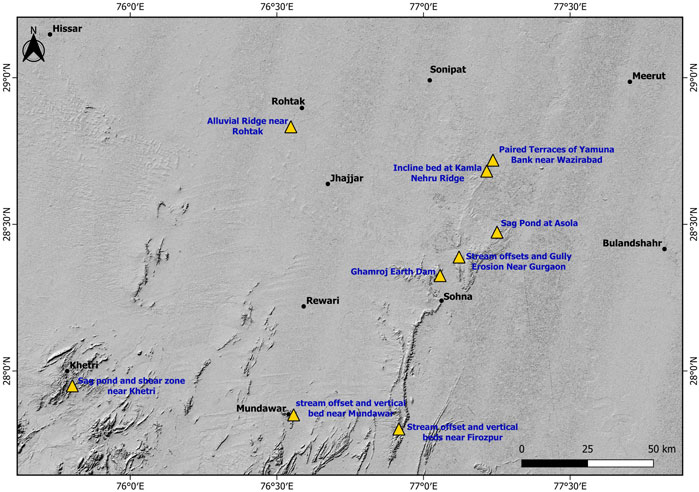
FIGURE 4. The map show the locations we visited during the field investigations, along with the significant observations of the area.
6.1 Ghamroj Earth dam
Ghamroj Dam is located 33 km southwest of Delhi. At this site, NE-SW and NW-SE striking conjugate fractures displaced a severely folded or deformed structure. At the location of the Ghamroj Earth Dam, intercalated strata of brittle (quartzite) and ductile (phyllite) rocks of Delhi Supergroup (Proterozoic) were seen forming limbs of a significant anticlinal fold across a 200 m wide valley (Figure 5A). The North-Western limb of the fold dips gently, whereas the South-Eastern limb dips steeply. The ductile layers of the meta-sedimentary rock series displayed deformational characteristics such as drag-folding and embedded/rotated fragments of underlying/supervening brittle layers. We have not identified any evidence of surface exposure of active fault signature in this location. The bedding-parallel slip between the ductile/brittle layers during tectonic activity in the geological past may have resulted in the formation of a drag fold. However, it is also possible that the deformation in the DAFB, either due to the Himalayan strain (Bansal et al., 2021a) or relative motion between Marwar and Buldenkhand craton (Yadav et al., 2022), is contributing to the bedding parallel slip in these drag folds. Further study is required to validate the phenomena. (Figures 5B,C).
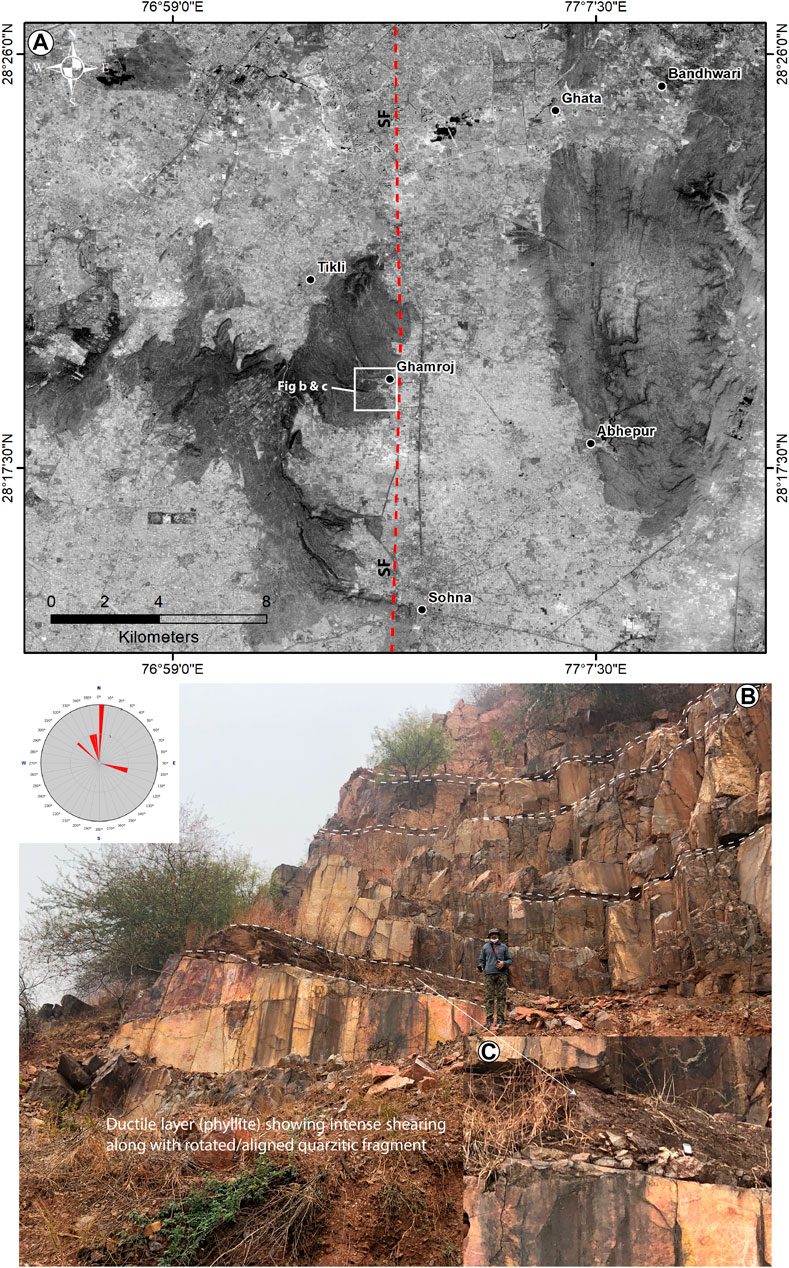
FIGURE 5. (A) Ghamroj Earth Dam 200 m wide valley is depicted on the Cartosat-1 data nearby Delhi Mega Fold. In figures (B) and (C), Intercalated brittle (Quartzite) and ductile (Phyllite) layers can be seen in the meta-sedimentary rock sequence. The attitude of the folded bed is shown by the rose diagram. SF- Sohna Fault.
6.2 Kotla, Nuh and Firozpur Jhirka
Interpretations of satellite data pointed to notable streams offset along a right-lateral fault system (Figure 6A). It also indicates that the area is characterized by several fault lines. Our preliminary findings indicate that the southern extension of the Sohna fault is located west of the Delhi mega fold. The terrain from Sohna and its south is marked by a narrow linear ridge striking in the N-S direction comprised of steeply dipping meta-sedimentary succession (Figure 6B). A few prominent streams offset with a right-lateral sense of movement were observed on the eastern flank of the fold. These linear ridges are typically steeply dipping beds of metasedimentary rocks (Figure 6C).
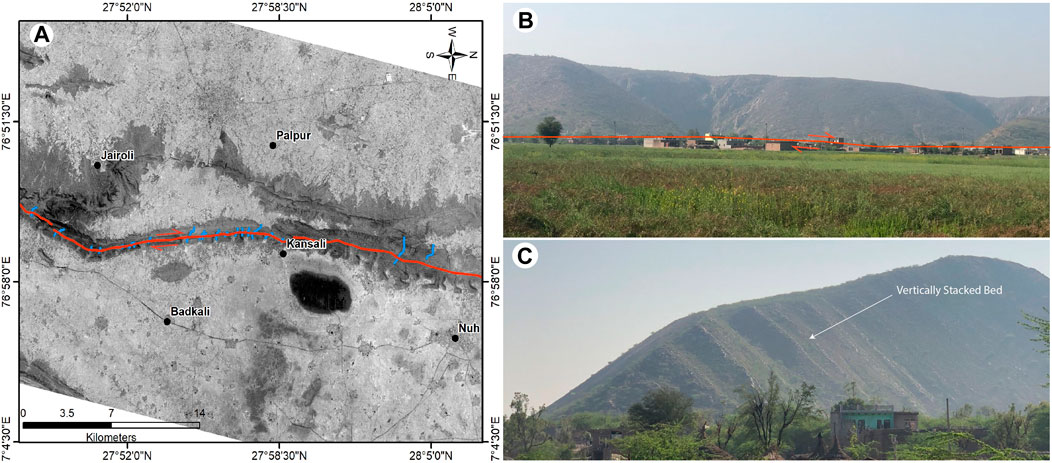
FIGURE 6. (A) South of Sohna, folded terrain can be seen at Kotla, Nuh, and Firozpur Jhirka on a Cartosat-1 image. Along the eastern flank of the linear Ridge, a few streams with a right lateral offset were marked as blue lines. Field photographs of folded and steeply dipping beds (B and C) around Kotla, Nuh, and Firozpur Jhirka south of Sohna.
6.3 Sag-pond near Khetri, Rajasthan
A linear Ridge of Delhi Aravalli Fold Belt is striking NNE-SSW from 6 km southeast of Khetri, Rajasthan (Figure 7). It was observed that the metasedimentary rocks of Delhi Supergroup exhibit vertical beds and shear zone in this region (Figures 7A, C). The deformation observed as a shear zone might have developed over the geological past. However, the vertical beds indicate strike-slip movement along a fault. The origin of the sag-pond is due to the right stepping of the Mahendragarh Dehradun Fault. The right stepping of a right-lateral fault can result in localized extension; hence, features like normal faulting and sag-pond are expected. Vertical beds of metasedimentary rock were observed on either side of the sag-pond, with N45°E and N55°E strikes (Figures 7B, D). Based on the fault topography marked by NW facing fault scarps and distinct sag-pond as well as shear zone, we suggest that this could be a trace of active fault and represents an active portion of the Mahendragarh Dehradun Fault (MDF).
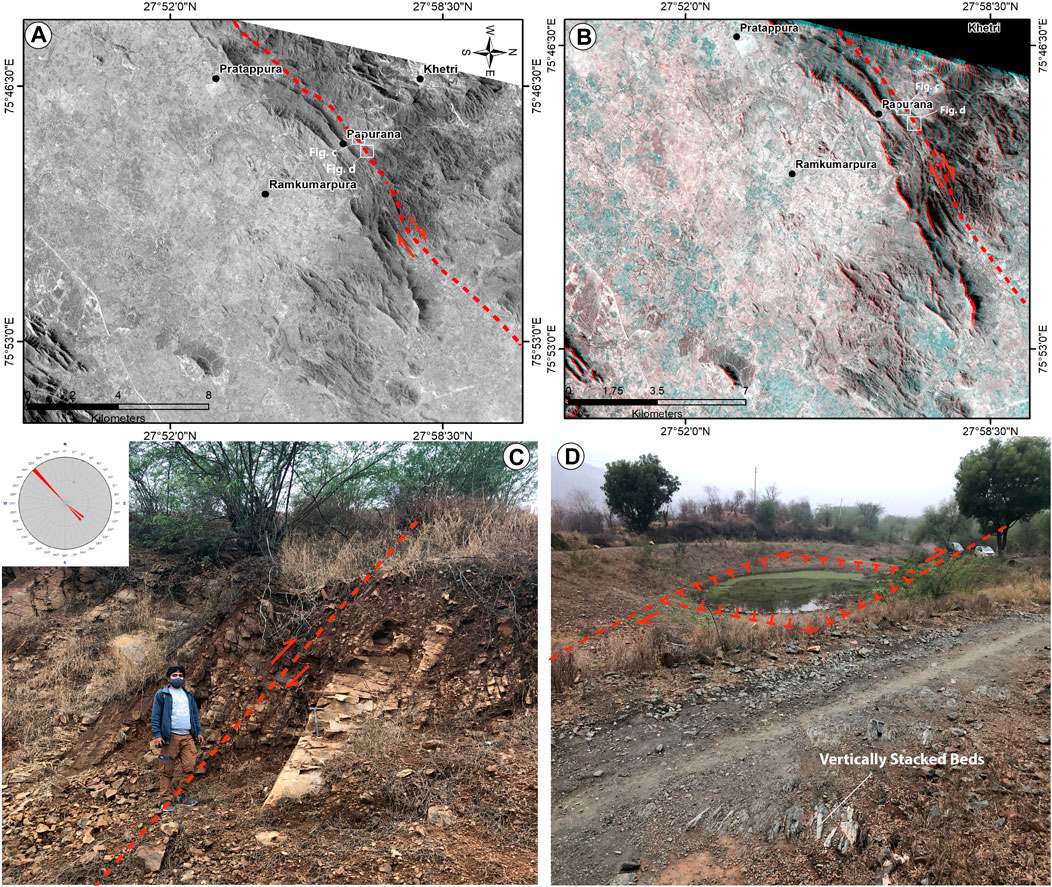
FIGURE 7. (A) CARTOSAT-1 Band F image and (B) Anaglyph of the region depicts the significant shearing of the Aravalli folded bed (C) and a sag pond (D) (A high resolution Anaglyph is given as Supplementary 7B). This could be a remnant of an active fault. Aravalli folded succession striking NNE-SSW has been displaced by it. NW facing Scarp and sag ponds (D) identify the topography of the fault. This fault trace marks the Mahendragarh Dehradun Fault. The orientation of beds is shown by a rose diagram. A red dashed line traces the fault.
6.4 Delhi mega fold
South-East of Gurgaon, a noticeable, N-S trending deeply incised linear valley was observed along the western limb of the Delhi Mega Fold (Figure 8). With a right-lateral sense of movement, the western and eastern flanks of the valley delineate discrete stream offsets (Figures 8A,B). A reconnaissance survey was conducted in the area based on the interpretation of satellite data. It was found that a valley surrounded by two rocky ridges is characterized by thick Quaternary deposits that are susceptible to severe gully erosion (Figure 8C). The linear features identified on satellite data exhibit right-lateral offset of streams (Figure 8B). It was observed that the stream offset is accompanied by gulley erosion of Quaternary deposits, predominantly silty sand with sporadic gravel layers (20–25 m). Based on the subsurface GPR survey through this area, Dubey et al. (2012) reported a subsurface fault trending NW-SE direction named as ‘Inferred Fault sub-parallel to DSR.’ It is important to note here that the epicenter of the historic 1720 earthquake (as suggested by IMD) of magnitude Mw 6.5 is located in close proximity to this region (Figure 8).
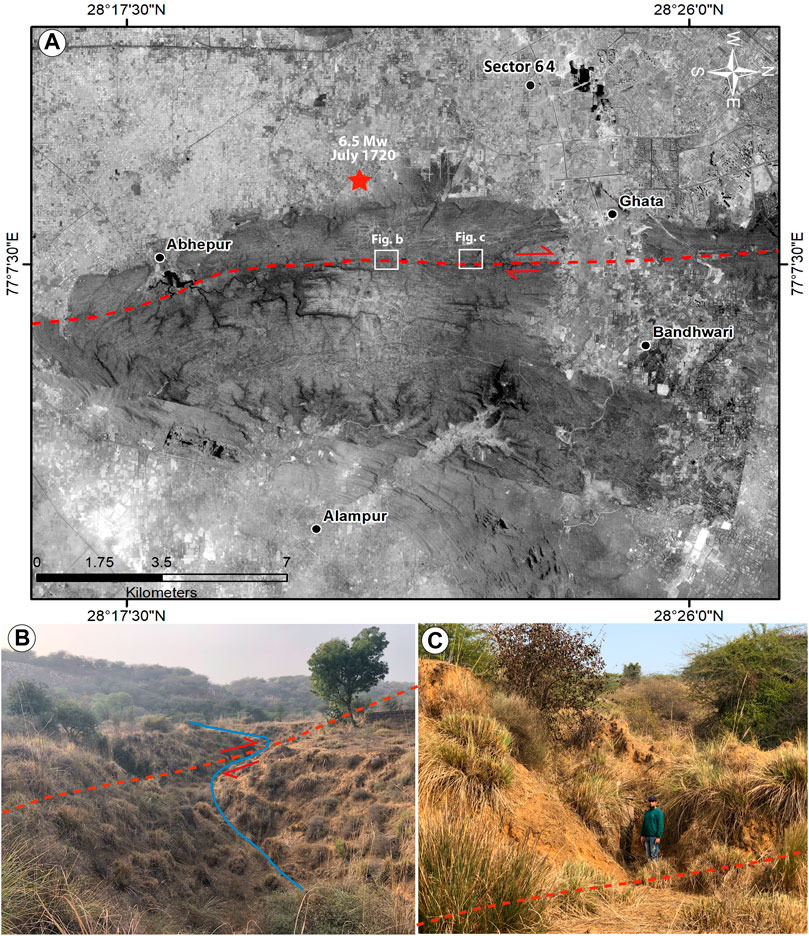
FIGURE 8. (A) Image from CARTOSAT-1 depicting the Delhi Mega-Fold and an inferred active fault trace (marked by a red dashed line). (B) Streams with pronounced right-lateral offset were seen along N-S oriented linear valley. (C) The area exhibits severe gulley erosion in the Quaternary succession. The location of the 1720 AD earthquake is also marked on the image (Source: NCS).
In the epicentral region of the 1720 AD earthquake, a brief field experiment was undertaken along the Kamla Nehru Ridge and the west bank of the Yamuna River near Wazirabad (as per the location marked by Chandra, 1978). The folded Kamla Nehru Ridge is the northern extension of the Delhi Mega Fold (Figure 9A). The north-Western limb of the Kamla Nehru Ridge dip gently, and the South-Eastern limb dip steeply (Figure 9B). The northernmost point of the Kamla Nehru Ridge, which continues to cut through the Timarpur outer ring road, was explored for active tectonic features.
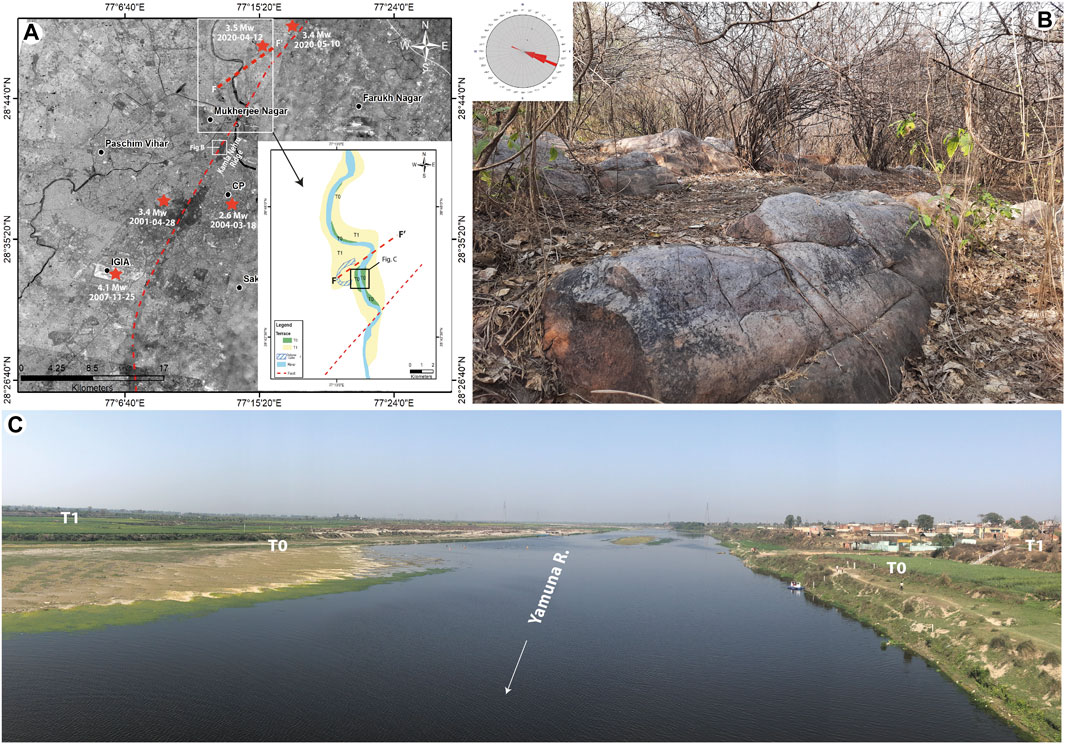
FIGURE 9. (A) A typical hairpin curve associated with the Yamuna River has been located on Cartosat data. The change in the Yamuna River channel pattern can be seen in the satellite data, along with the creation of minor meanders, the expansion of straight channels, and a rapid pattern shift. The red dashed line showed the NE extension of the Delhi fault. The inset geomorphic map shows the Oxbow Lake, terraces, and Yamuna River fault line. Major earthquakes that occurred in this region are also marked on the map. F-F′ fault is Khanpur Japti Fault marked by Bansal et al. (2021b). (B) Field Photograph of Kamla Nehru Ridge. The orientation of beds is shown by a rose diagram. (C) Yamuna river bank near Wazirabad, Delhi. Two levels of Yamuna river terraces can be seen here.
Indicators of active tectonic deformation in alluvial terrain include the unusual channel pattern, such as a sudden change of course, the appearance of minor meanders, the unusually straight channel course, and a rapid shift in pattern. Changes in water discharge, sediment load, sediment type, and gradient can all affect the shape of the alluvial channel. The gradient of a river and its valley may change due to tectonic activity. When there is a sudden change in the river base level, it will start to incise the river bed and try to achieve equilibrium. Because there are not any prominent outcrops of folded ridges on the Indo-Gangetic Plain, we focused on the channel patterns in alluvium. The Yamuna River in Wazirabad has an oxbow lake and two levels of paired river terraces that indicate that the river has been changing its base level, possibly due to tectonic activity (Figures 9A, C). There are also hairpin twists along the Yamuna channel close to Wazirabad and Khanpur Japti. A NE-SW fault (F-F′) is marked near the hairpin band of Khanpur Japti based on the seismological and morphometric analysis by Bansal et al. (2021b). An increase and decrease in the valley slope due to uplift or subsidence may result in tight meandering to the upstream and a straight channel pattern to the downstream in an alluvial river channel. Increasing the slope of the channel bed will cause a straight to meandering pattern, and decreasing the slope will result in a meander to a straight channel pattern (Schumm et al., 1984). The ongoing dynamic tectonic deformation that produced the mix of the straight channel and hairpin meanders along with the terraces may have been influenced by a fault running parallel to the axis of Kamla Nehru Ridge starting from the southern tip of the Delhi Mega Fold to Khanpur Japti (Figure 9A). The region includes the epicenter of the earthquakes that occurred on 28 April 2001, 12 April 2020, and 10 May 2020.
Asola Wildlife Sanctuary is located northwest of Faridabad, and a NNW-SSE striking fault passes across it. A deeply incised linear valley along it has been observed. Based on GPR data, Dubey et al. (2012) identified a fault about 2 km south of this deeply incised linear valley that runs parallel to its strike named as ‘Inferred Faridabad-Mehrauli-Rohtak Fault’ (Figure 10D). However, the interpretation of the satellite data and field observations during this study suggests that an active fault is passing through Asola also. The eastern part of the Delhi Mega Fold appears to have been moved or bent by this fault. The fault is distinguished by a deep, narrow linear valley that stretches 6–10 km in the NNW–SSE direction (Figure 10B). The fault topography is characterized by fault scarps generated in the Quartzitic succession, together with deep, narrow gorges (Figure 10C) and sag-ponds (Figure 10A) created as a result of lateral fault movement.
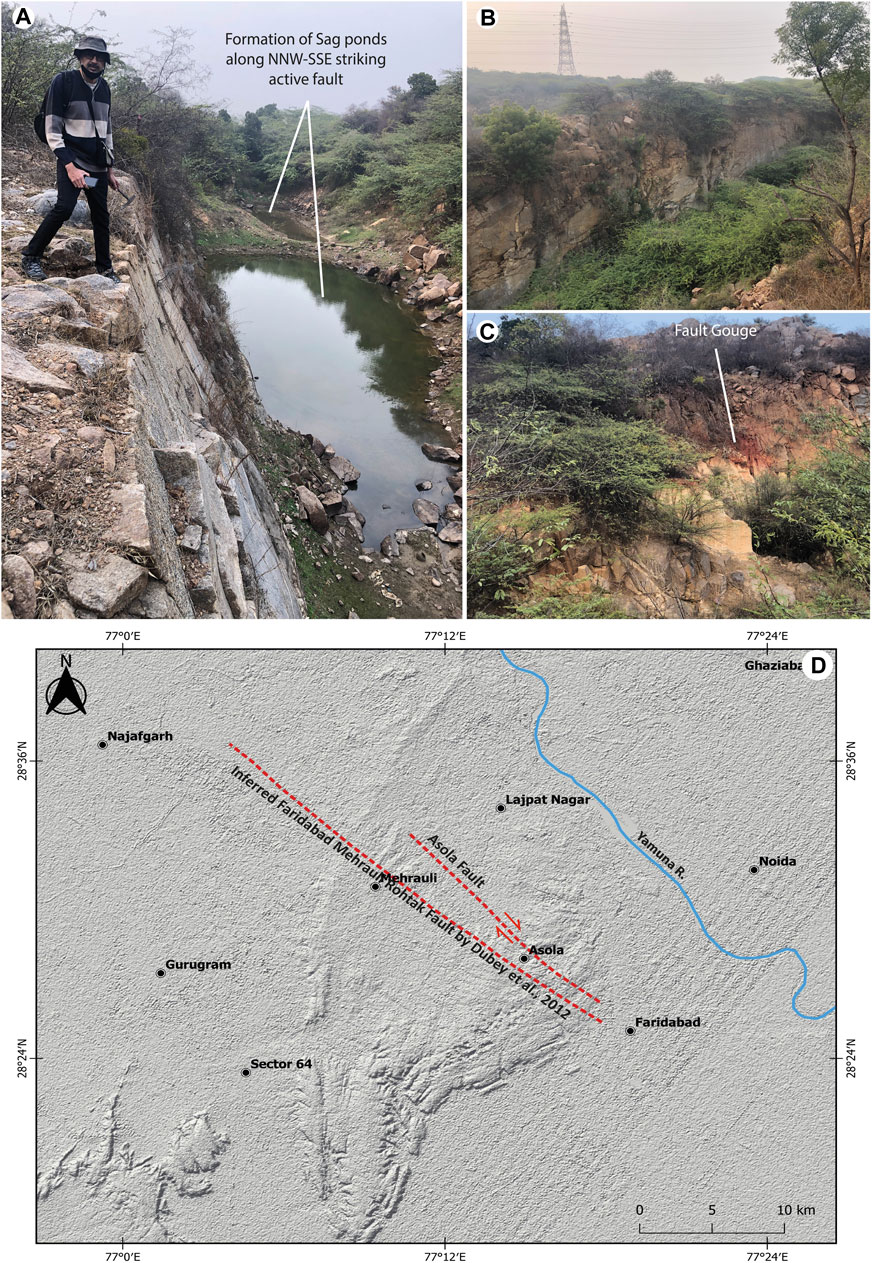
FIGURE 10. Field photos of the Asola, Linear valleys, and formation of sag ponds (A and B) and the fault gauge (C) along the fault passing through the region. (D) Two faults are marked on the Suttle Radar Topographic Mission (SRTM) data of the Delhi Mega Fold. Asola Fault (AF) identified in this study, and Inferred Faridabad Mehrauli Rohtak Fault identified by Dubey et al. (2012), are marked on the map. Yamuna River is marked with solid blue line.
6.5 Quaternary alluvial ridge near rohtak, Haryana
On satellite data, a topographic mound with an NNW-SSE trend was noticed close to Rohtak town (Figures 11A, B). This quaternary ridge is located 8 km SW of Rohtak with a dimension of 1.2 × 2 km at the height of almost 10–12 m. The observed feature is located west of the Mahendragarh Dehradun Fault (MDF). Given the locations of previous and recent seismic activity, it is evident that the clustering of the earthquake was in this area. (Figure 2). The region is also affected by swarm activity during 1963–65 (Kamble and Chaudhary, 1979). It was observed during the field trip that the feature noted on the satellite data close to Rohtak has no hard rock exposure. The quaternary ridge makes up medium to fine silty sand (Figure 11C). The silty sand-containing quaternary sediments have seen significant gully erosion. Several subsurface NW-SE oriented ridges are identified on the Anaglyph to the western side of MDF. This can result from the region’s tectonic bulging because of compression on the western side of MDF. However, this region is forming a trijunction from Delhi-Haridwar Ridge (DHR)/Mahendragarh Dehradun Fault (MDF) and Delhi Sargoda Ridge (DSR) (Bansal et al., 2021a) that might play an essential role in the region’s seismicity and compression. Patel et al. (2020) performed the GPR survey at several places across the MDF near Rohtak, a close proximity to 2 June 2017 earthquake, and identified shallow subsurface normal faults parallel to the MDF. However, MDF was not traced during this study.
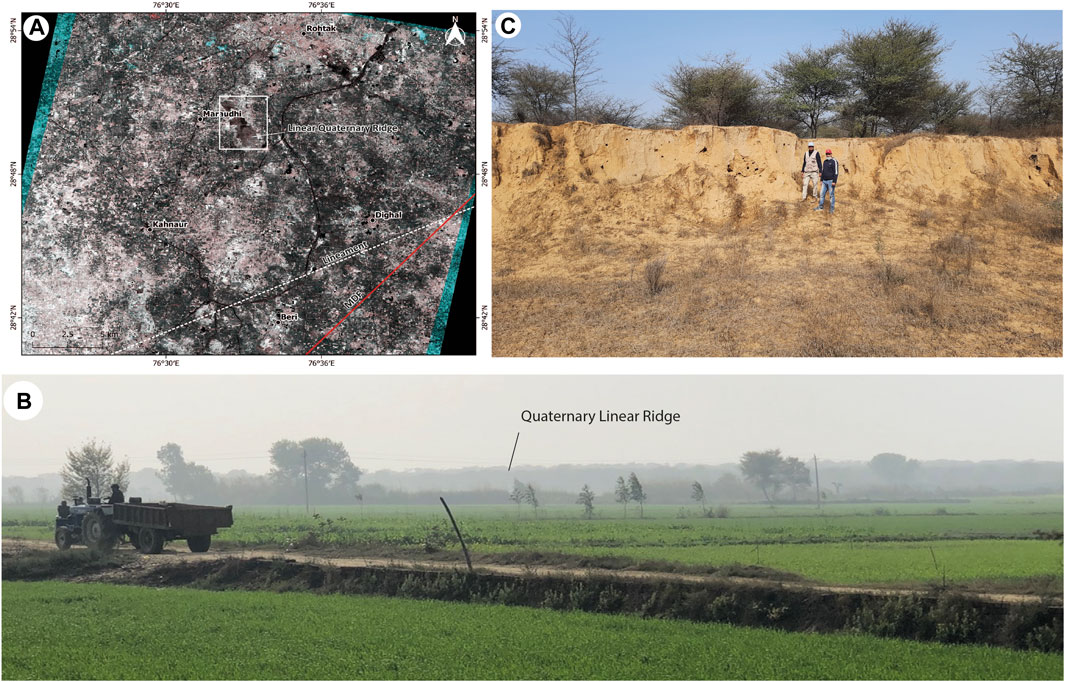
FIGURE 11. (A) Near Rohtak town, a notable NW-SE linear quaternary Ridge extends for 2–4 km. The axis of the linear mound is oriented in the NW-SE direction, marked with a white square box. (B) A field photograph was taken of the linear quaternary Ridge near Rohtak. (C) A closeup view of Alluvial Quaternary. The Ridge is made of medium to fine silty sand. MDF- Mahendragarh Dehradun Fault.
6.6 Mundawar
A geological investigation was carried out in the epicentral area of 3 July 2020 (Mw 4.7) earthquake near Mundawar. We have not observed any surface rupture or deformation related to 3 July 2020 earthquake in the epicentral area. However, we have observed shearing in SE dipping (near to vertical ∼65°SE) quartzite beds along Delhi Aravalli Fold Belt on the way to Mundawar, probably responsible for the slip during tectonic activity in the geological past. (Figure 12A, B). Further, near Mundawar North-West facing fault scarp along the NNE-SSW striking fault was observed on satellite data (Figure 12C). The fault scarp has developed within partially metamorphosed sandstone (Figure 12D). Right-lateral stream offsets were also identified on satellite data and confirmed in the field along this fault (Figure 12E).
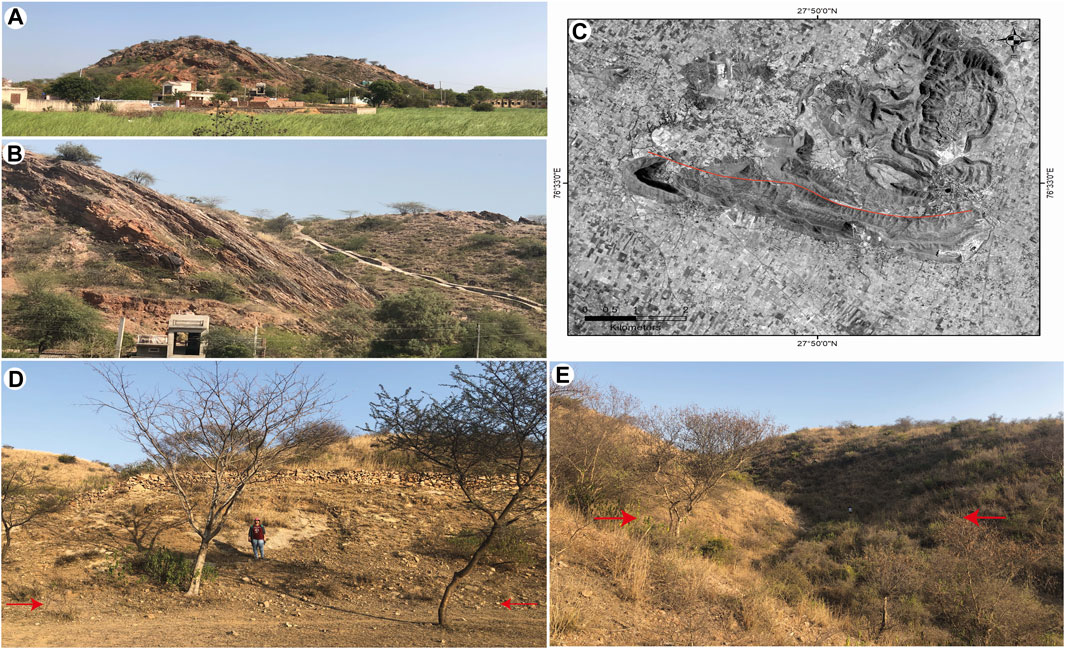
FIGURE 12. (A) A shear zone was observed on the way to Mundawar. The shearing has occurred along the SE dipping (near to vertical ∼65°SE) beds. (B) Close-up view of the shear zone in a nearly vertical bed. (C) The terrain around Mundawar is shown in the Cartosat-1 data. Solid red lines have demarcated the trace of the North-West facing fault scarp. (D) Field photo of North-West facing fault scarp along NE-SW trending fault. (E) Right-lateral stream offset of about 10–12 m along NE-SW striking fault. The red arrow marks the fault trace.
7 Discussion
Given the past seismic activity in and around Delhi-NCR, which ranged from Mw 1.0 to Mw 6.7, we cannot rule out the possibility of similar events occurring in the future. The clustering of earthquakes along the northern edge of the Delhi Aravalli fold belt (DAFB) indicates the probable reactivation of several DAFB faults and lineaments. Therefore, a detailed active fault mapping is required to precisely identify and mark the active fault traces in the area. The western and eastern boundaries of the Aravali Delhi Fold Belt (ADFB), which is tectonically active and has higher seismicity in the vicinity of Delhi, are defined by the Mahendragarh Dehradun Fault (MDF) and the Great Boundary Fault (GBF) (Shukla et al., 2007; Yadav et al., 2022). The lineaments are significant structures in the region, including the N-S oriented Sohna Fault (SF) (Geological Survey of India, 2000) (Figure 2). The Himalayan zone, Delhi-Haridwar Ridge zone (DHR), Moradabad Fault (MF), Great Boundary Fault (GBF), Mathura Fault, and Sohna Fault (SF) have all been identified as seismic sources of the area (Sharma et al., 2003). The Delhi NCR is one of the most densely populated region in the world. Due to the excessive withdrawal of groundwater for irrigation and urban requirements, the region is experiencing serious groundwater depletion issues. One of the causes of small magnitude earthquakes in Delhi and the National Capital Region could be groundwater extraction. Bansal et al. (2021a) reviewed the geophysical and geological investigations in the area and discovered three mechanisms for the pattern and occurrence of earthquakes: (a) episodic reactivation of the DHR, (b) Himalayan crustal loading on the Delhi Sargoda Ridge (DSR), and (c) interaction of the Aravalli Delhi Mobile belt with the DSR. It is believed that relative motion between the Buldenkhand and Marwar cratons to the east and west respectively causes seismicity in the DAFB (Yadav et al., 2022). The earthquakes associated with the MDF show that it is an active fault, which has significance for earthquake hazard assessments (Patel et al., 2020). We focused on the epicentral region of earthquakes that occurred in the last 2 decades in this study and attempted to understand surface morphology changes using geomorphic markers. We visited a few sites along the MDF, Sohna Fault, and Delhi Mega Fold and were able to identify the extension of the MDF to its southern end up to Khetri (Figures 7, 14) and the extension of the Sohna Fault as Nuh Jhirka Fault (Figures 6, 14). Based on satellite data and field investigations, we believe these faults have distinct right-lateral strike-slip components. We found NW-SE oriented Quaternary Ridges to the west of the MDF as well as NW-SE and NE-SW oriented extensional fractures to its east, indicating a compressional regime toward the west of the MDF and an extensional regime to the eastern part of the MDF (Figure 13). The fault plane solution of the last 2 decades is also suggesting that the earthquakes that occurred towards the western side of MDF show strike slip with reverse component (Compression), and the eastern side shows strike slip with normal component (Extensional) (Bansal et al., 2009; Singh et al., 2010; Bansal and Verma, 2012; Pandey et al., 2020; Bansal et al., 2021a) (Figure 3). Shukla et al. (2007) suggested that the DAFB is bounded towards the west by a prominent strike-slip faults, i.e., Mahendragarh Dehradun Fault (MDF). Srinivasa and Khar (1995) also suggested that MDF is geologically inferred as a strike slip fault. On the basis of geological and geomorphological data, it can be inferred that the MDF behaves as a dextral strike-slip fault separating the compressional and extensional regimes of northern DAFB.
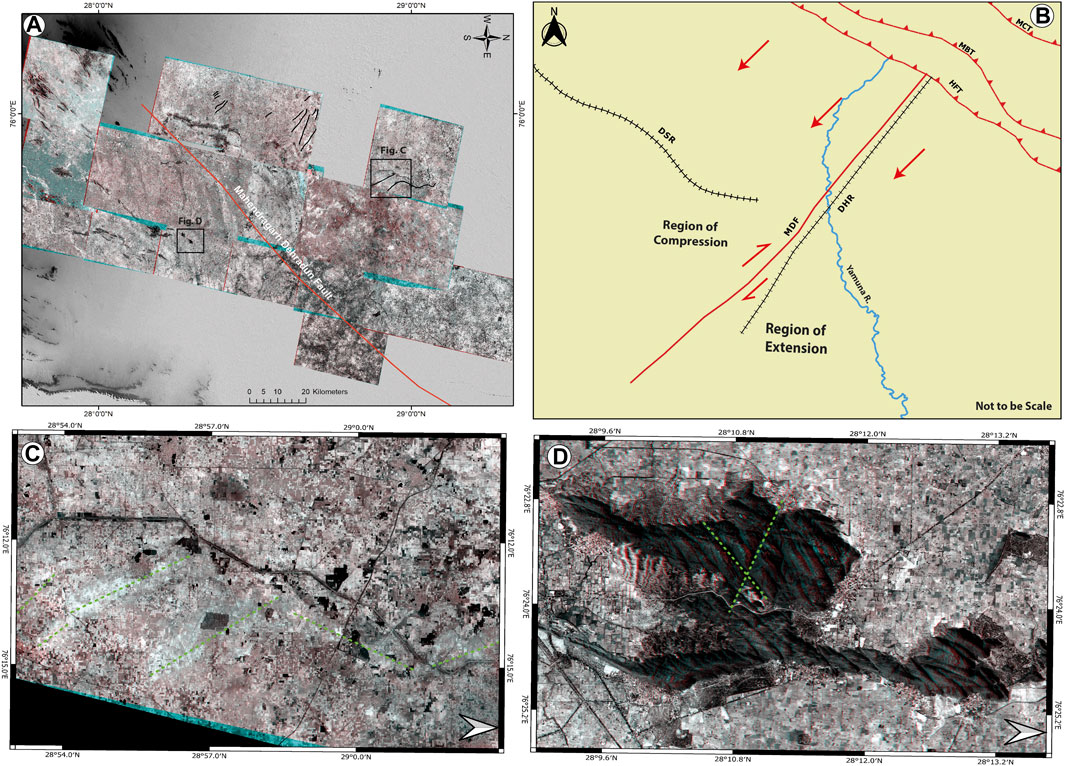
FIGURE 13. (A) The Anaglyphs show the NNE-SSW oriented Mahendragarh Dehradun Fault with compression on the western side and extensional on the eastern side. (B) A systematic cartoon shows the mechanism of tectonics in Delhi-NCR. The NNE-SSW oriented Mahendragarh Dehradun Fault with compression on the western side and extensional on the eastern side. (C) and (D) are the closeup of areas showing extensional features with linear mounds and compressional features with fractures. Broken green lines shows the axis of linear mounds in (C) and extensional fractures in (D). MDF- Mahendragarh Dehradun Fault; DHR- Dehradun-Haridwar Ridge; DSR- Delhi Sargoda Ridge; HFT- Himalayan Frontal Thrust; MBT- Main Boundary Thrust; MCT- Main Central Thrust.
The Earthquake occurrence in Delhi and its surrounding region is diverse, and it is very difficult to correlate the seismicity to any particular faults. There are possibilities that a number of faults of lineament within the DAFB are reactivated. The Geological Survey of India (GSI) has delineated the N-S oriented Sohna Fault (SF), which extends from Sohna to the west of Delhi. Our investigation along the Ridge from Nuh to Firozpur Jhirka has also identified multiple active tectonic features. This fault is named as Nuh-Jhirka Fault and is present south of the Sohna Fault. It is a right-lateral strike-slip fault, most likely the Sohna Fault’s southern extension (Figure 6). This N-S oriented fault system is active and most probably one of the causes of the Delhi earthquake clustering. In addition, a fault that runs across the western limb of the Delhi Mega Fold and up to the Khanpur Japti is identified during this study using geomorphic markers on satellite data and field investigations (Figures 8, 9A). Despite this, no previous studies have identified this flaw. Two levels of terraces are observed along the Yamuna River, indicating a change in the river’s base level. The river also has a sharp meandering pattern on the upstream side of the identified fault, whereas the downstream side of the fault has a straight course. There are two hairpin bands near Wazirabad and Khanpur Japti. Bansal et al. (2021b) identified a NE-SW oriented fault and named it Khanpur Japti Fault. Some historical and recent earthquakes have occurred in this area (Figure 9A). These earthquakes could have been caused by the Khanpur Japti and Delhi Fault (DF), which has the characteristics of an active fault. The findings of this study, which are based on the interpretation of satellite data and field observation, are preliminary. We have marked the probable active faults of the Delhi-NCR region (Figure 14). In order to produce a comprehensive active fault map of the Delhi-NCR for proper Seismic Hazard Assessment (SHA), more detailed investigations should be carried out.
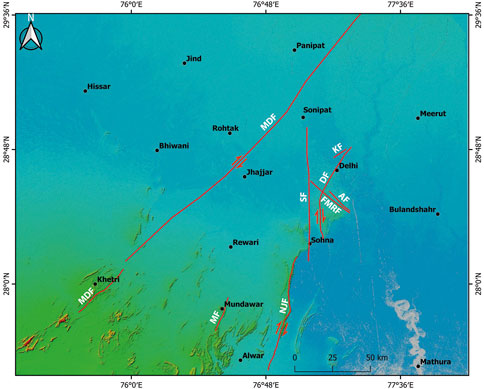
FIGURE 14. Location of probable active fault map around Delhi-NCR. Active faults are marked with solid red color. MDF- Mahendragarh Dehradun Fault; SF- Sohna Fault; DF- Delhi Fault; NJF- Nuh-Jhirka Fault; AF- Asola Fault; KF- Khanpur Japti Fault; FMRF- Faridabad Mehrauli Rohtak Fault.
8 Conclusion
The following conclusions are drawn from the present study.
1. The presence of a shear zone in the meta-sedimentary succession shows that ductile layer and steeply dipping bedding caused bedding parallel slip during local tectonic activity in Delhi-NCR in the geological past and possibilities of cause of recent seismicity in Delhi NCR.
2. Several geomorphic features have been identified in the NCR, including offset streams, sag ponds, linear ridges and valleys, fault scarps, and shear zones. All these geomorphic markers are indicators of active faults in Delhi-NCR.
3. The location of the historic Mw 6.5 earthquake of 1720 AD is close to active tectonic geomorphic features found along the Delhi Fault (DF). The NNE-SSW Mahendragarh Dehradun Fault, N-S oriented Sohna, Nuh-Jhirka Fault, and NE-SW oriented Delhi Fault contribute to the region’s seismicity.
4. Based on the available focal plane mechanisms and geomorphic evidence from this study, it is possible to hypothesize that the western side of the Mahendragarh Dehradun Fault (MDF) represents compression, whereas the eastern side represents an extensional environment with prominent right lateral strike slip movement. On the basis of geological and geomorphological data, it could also be inferred that the MDF behaves as a dextral strike-slip fault separating the reverse component (Compression) to the west of MDF and normal component (Extension) to its eastern part of northern DAFB.
Data availability statement
The original contributions presented in the study are included in the article/Supplementary Material, further inquiries can be directed to the corresponding author.
Author contributions
JM, MG, MD, and PN contributed towards identifying preliminary active fault topography based on satellite data interpretation and field survey. KM contributed to the seismicity data of the region. KM also accompanied in the field. All authors contributed to the article and approved the submitted version.
Funding
Financial support was received from the National Centre for Seismology (NCS), MoES, New Delhi.
Acknowledgments
We are thankful to National Centre for Seismology (NCS), Ministry of Earth Sciences, New Delhi for providing funds to undertake this study. Suggestions provided by Mishra is duly acknowledged. Mr. Harendra and Mr. Bhanu from NCS helped during the field survey. We are also thankful to both the reviewers (Mandal and Maurya) for providing constructive suggestions/comments.
Conflict of interest
The authors declare that the research was conducted in the absence of any commercial or financial relationships that could be construed as a potential conflict of interest.
The handling editor declared a past co-authorship with the author KM.
Publisher’s note
All claims expressed in this article are solely those of the authors and do not necessarily represent those of their affiliated organizations, or those of the publisher, the editors and the reviewers. Any product that may be evaluated in this article, or claim that may be made by its manufacturer, is not guaranteed or endorsed by the publisher.
Supplementary material
The Supplementary Material for this article can be found online at: https://www.frontiersin.org/articles/10.3389/feart.2023.1092927/full#supplementary-material
References
Ambraseys, N., and Douglas, J. (2004). Magnitude calibration of north Indian earthquakes. Geophys. J. Int. 159, 165–206.
Ambraseys, N., and Jackson, D. (2003). A note on early earthquakes in northern India and southern Tibet. Curr. Sci. 84, 570–582.
Bansal, B. K., Mohan, K., Ul Haq, A., Verma, M., Prajapati, S. K., and Bhat, G. M. (2021b). Delineation of the causative fault of recent earthquakes (April–May 2020) in Delhi from seismological and morphometric analysis. J. Geol. Soc. India 97 (5), 451–456.
Bansal, B. K., Mohan, K., Verma, M., and Sutar, A. K. (2021a). A holistic seismotectonic model of Delhi region. Sci. Rep. 11 (1), 13818–13911. doi:10.1038/s41598-021-93291-9
Bansal, B. K., Singh, S. K., Dharmaraju, R., Pacheco, J. F., Ordaz, M., Dattatrayam, R. S., et al. (2009). Source study of two small earthquakes of Delhi, India, and estimation of ground motion from future moderate, local events. J. Seismol. 13 (1), 89–105. doi:10.1007/s10950-008-9118-y
Bansal, B. K., and Verma, M. (2012). The M 4.9 Delhi earthquake of March 5 2012. Curr. Sci. 102 (12), 1704–1708.
Bilham, R., and Ambraseys, N. (2005). Apparent Himalayan slip deficit from summation of seismic moments for Himalayan earthquakes 1500- 2000. Curr. Sci. 88 (10), 1658–1663.
Bilham, R. (2019). Himalayan earthquakes: A review of historical seismicity and early 21st century slip potential. Geol. Soc. Lond. Spec. Publ. 483, 423–482. doi:10.1144/SP483.16
Bureau of Indian Standards (2016). “IS 1893:2016 - Indian standard criteria for earthquake resistant design of structures,” in Part 1 - general provisions and buildings (Manak Bhawan, Old Delhi: Bureau of Indian Standards).
Chandra, U. (1978). Seismicity, earthquake mechanisms and tectonics along the Himalayan mountain range and vicinity. Phys. Earth Planet. Inter. 16 (2), 109–131. doi:10.1016/0031-9201(78)90083-3
Chouhan, R. K. S. (1975). Seismotectonics of Delhi region in proc. Indian Nat. Sci. Acad. 41, 429–447.
Dubey, C. S., Shukla, D. P., Singh, R. P., Sharma, M., Ningthoujam, P. S., and Bhola, A. M. (2012). Present activity and seismogenic potential of himalayan sub-parallel thrust faults in Delhi: Inferences from remote sensing, GPR, gravity data and seismicity. Near Surf. Geophys. 10 (5), 369–380. doi:10.3997/1873-0604.2012006
Duvall, M. J., Waldron, J. W., Godin, L., and Najman, Y. (2020). Active strike-slip faults and an outer frontal thrust in the Himalayan foreland basin. PNAS 17, 17615–17621. doi:10.1073/pnas.2001979117
Gahalaut, V. K., and Kundu, B. (2012). Possible influence of subducting ridges on the Himalayan arc and on the ruptures of great and major Himalayan earthquakes. Gondwana Res. 21, 1080–1088. doi:10.1016/j.gr.2011.07.021
Geological Survey of India (2000). GSI (2000) Seismotectonic atlas of India and its environs. India: Geological Survey of India.
Gupta, A. S., and Rao, K. S. (2000). Weathering effects on the strength and deformational behaviour of crystalline rocks under uniaxial compression state. Eng. Geol. 56, 257–274. doi:10.1016/S0013-7952(99)00090-3
Gupta, S. N., Arora, Y. K., Mathur, R. K., Iqbaluddin, B. P., Sahai, T. N., Sharma, S. B., et al. (1980). Lithostratigraphic map of Aravalli region (1:1000,000). Calcutta: Geol. Surv. India.
Iyengar, R. N., and Ghosh, S. (2004). Microzonation of earthquake hazard in Greater Delhi area. Curr. Sci. 87 (9), 1193–1202.
Kamble, V. P., and Chaudhary, H. M. (1979). Recent seismic activity in Delhi and neighbourhood. Mausam 30, 305–312. doi:10.54302/mausam.v30i2.3046
Karunakaran, C., and Ranga Rao, A. (1976). Status of exploration for hydrocarbons in the Himalayan region-contribution to stratigraphy and structure. Geol. Surv. India. Misc. Publ. 41, 1–66.
Malik, J. N., Naik, S. P., Sahoo, S., Okumura, K., and Mohanty, A. (2017). Paleoseismic evidence of the CE 1505 (?) and CE 1803 earthquakes from the foothill zone of the kumaon Himalaya along the himalayan frontal thrust (HFT), India. Tectonophysics 714–715, 133–145. doi:10.1016/j.tecto.2016.07.026
Mandal, B., Vaidya, V. R., Sen, M. K., Periyasamy, K., and Sarkar, D. (2018). Common reflection surface stack imaging of the Proterozoic Chambal Valley Vindhyan basin and its boundary fault in the northwest India: Constraints on crustal evolution and basin formation. Tectonics 37, 1393–1410. doi:10.1029/2017TC004895
Nandakumar, R., Prakash, B., KartikeyanChamy, M. P. T., Singh, S., and Srinivasan, T. P. (2008). Improvements in cartosat-1 stereo orthokit data products since launching isprs-isro cartosat-1 scientific assessment programme. Beijing: The international archives of the photogrammetry, remote sensing and spatial information sciences. Vol. Part B1, 1357–1362. Archives.
Oldham, T. A. (1883). Catalogue of Indian earthquake from the earliest time to the end of A.D. 1869. Mem. Geol. Surv. India 19, 169–215.
Pandey, A. P., Suresh, G., Singh, A. P., Sutar, A. K., and Bansal, B. K. (2020). A widely felt tremor (ML3.5) of 12 April 2020 in and around NCT Delhi in the backdrop of prevailing COVID-19 pandemic lockdown: Analysis and observations. Geomat. Nat. Hazards Risk 11 (1), 1638–1652. doi:10.1080/19475705.2020.1810785
Patel, N. K., Pati, P., Verma, A. K., Dash, C., Gupta, A., and Sharma, V. (2020). Seismicity around the mahendragarh–dehradun basement fault in the Western Ganga plain, India: A neotectonic perspective. Int. J. Earth Sci. 109 (2), 689–706. doi:10.1007/s00531-020-01826-8
Rajendran, C. P., and Rajendran, K. (2005). The status of central seismic gap: A perspective based on the spatial and temporal aspects of the large himalayan earthquakes. Tectonophysics 395, 19–39. doi:10.1016/j.tecto.2004.09.009
Roy, A. B. (1988). Stratigraphic and tectonic framework of the Aravalli mountain range. India, Rajasthan: Precambrian of the Aravalli Mountain Rajasthan, 3–31.
Sastri, V. V., Bhandari, L. L., Raju, A. T. R., and Datta, A. K. (1971). Tectonic framework and subsurface stratigraphy of the Ganga basin. J. Geol. Soc. India 12 (3), 222–233.
Schumm, S. A., Gregory, D. I., Lattman, L. H., and Watson, C. C. (1984). River response to active tectonics: Final report to national science faoundation. Fort Collins, CO: Water Engineering and Technology, Inc., 125.
Sharma, M. L., Wason, H. R., and Dimri, R. (2003). Seismic zonation of the Delhi region for bedrock ground motion. Pure Appl. Geophys. 160 (12), 2381–2398. doi:10.1007/s00024-003-2400-6
Shukla, A. K., Prakash, R., Singh, R. K., Mishra, P. S., and Bhatnagar, A. K. (2007). Seismotectonic implications of Delhi region through fault plane solutions of some recent earthquakes. Curr. Sci. 93, 1848–1853.
Singh, S. K., Kumar, A., Suresh, G., Ordaz, M., Pacheco, J. F., Sharma, M. L., et al. (2010). Delhi earthquake of november 25 2007 (M w 4.1): Implications for seismic hazard. Curr. Sci. 99, 939–947.
Sinha-Roy, S., Malhotra, G., and Guha, D. B. (1994). A transect across Rajasthan Precambrian terrain in relation to geology, tectonics and crustal evolution of south-central Rajasthan. J. Geol. Soc. 31, 63–90.
Srinivasa, S., and Khar, B. M. (1995). “Status of hydrocarbon exploration in NW Himalaya and foredeep contributions to stratigraphy and structure,” in Symposium on Recent Advances in Geological Studies of Northwest Himalaya and the Foredeep (Lucknow: GSI), 1–20.
Srivastava, L. S., and Somayajulu, J. G. (1966). “The seismicity of area around Delhi,” in Proc. III symposiam on earthquake engg (Roorkee, Uttarakhand: Univ. of Roorkee), 417–422.
Srivastava, A. K. (1988). “Geoscientific appraisal of Najafgarh depression and related environmental problems of Delhi,” in Geomorphology and environment. 631–642. Editors S. Singh, and R. C. Tewari (Allahabad, India: The Allahabad Geographical Society).
Srivastava, P. K., Gopala, K., Srinivasan, B., Amitabh, T. P., Trivedi, S., and Nandakumar, R. (2006). Cartosat-1 data products for topographic mapping. ISPRS Commission-IV Int. Symposium Geospatial Databases Sustain. Dev. 37, 1357–1362.
Sugden, T. J., Deb, M., and Windley, B. F. (1990). “The tectonic setting of mineralisation in the proterozoic Aravalli Delhi orogenic belt, nw India,” in Precambrian continental crust and its economic resources. Editors S. M. B. T.-D. Naqvi (Amsterdam, Netherlands: Elsevier), 367–390. doi:10.1016/S0166-2635(08)70175-0in
Szeliga, W., Hough, S., Martin, S., and Bilham, R. (2010). Intensity, magnitude, location, and attenuation in India for felt earthquakes since 1762. Bull. Seismol. Soc. Am. 100 (2), 570–584. doi:10.1785/0120080329
Verma, M., Singh, R. J., and Bansal, B. K. (2014). Soft sediments and damage pattern: A few case studies from large Indian earthquakes vis-a-vis seismic risk evaluation. Nat. Hazards 74, 1829–1851. doi:10.1007/s11069-014-1283-4
Verma, R. K., Roonwal, G. S., Kamble, V. P., Mohanty, W. K., Dutta, U., Gupta, Y., et al. (1995). Seismicity of Delhi and its surrounding region. J. Himal. Geol. 6, 75–82.
Vijaya Rao, V., Prasad, B. R., Reddy, P. R., and Tewari, H. C. (2000). Evolution of Proterozoic Aravalli Delhi fold belt in the northwestern Indian shield from seismic studies. Tectonophys 327, 109–130. doi:10.1016/S0040-1951(00)00156-6
Keywords: active faults, Delhi NCR, mahendragarh dehradun fault, geomorphic markers, seismic hazard
Citation: Dhali M, Gadhavi MS, Mohan K, Narayana PP and Malik JN (2023) Active faults studies in Delhi and national capital region (NCR): Inferences from satellite data and field investigations. Front. Earth Sci. 11:1092927. doi: 10.3389/feart.2023.1092927
Received: 08 November 2022; Accepted: 20 February 2023;
Published: 22 May 2023.
Edited by:
Brijesh K. Bansal, Indian Institute of Technology Delhi, IndiaReviewed by:
Prantik Mandal, National Geophysical Research Institute (CSIR), IndiaDee Pak M. Maurya, Maharaja Sayajirao University of Baroda, India
Copyright © 2023 Dhali, Gadhavi, Mohan, Narayana and Malik. This is an open-access article distributed under the terms of the Creative Commons Attribution License (CC BY). The use, distribution or reproduction in other forums is permitted, provided the original author(s) and the copyright owner(s) are credited and that the original publication in this journal is cited, in accordance with accepted academic practice. No use, distribution or reproduction is permitted which does not comply with these terms.
*Correspondence: Javed N. Malik, amF2ZWRAaWl0ay5hYy5pbg==