- 1CNOOC China Limited Zhanjiang Branch, Guangdong, China
- 2Chongqing University of Science and Technology, Chongqing, China
- 3CNOOC China Limited Hainan Branch, Hainan, China
In the development of multi-layer co-production heterogeneous reservoirs, problems such as serious inter-layer heterogeneity and interference always exist, resulting in an unclear understanding of inter-layer production. A clear understanding of the interference mechanism and influence of main controlling factors of multi-layer heterogeneous reservoirs on the production of small layers is the key to the effective development of the reservoirs. On the basis of clarifying the main controlling factors affecting the production of multi-layer heterogeneous reservoirs, this paper developed a multi-pipe parallel displacement experiment system to carry out indoor heterogeneous reservoir multi-layer water injection flooding experiments. Combined with dynamic and static parameters, the experiments simulated and evaluated the effects of factors such as permeability ratio, water cut, shutting down high permeability layers, production pressure difference, and change in crude oil viscosity in high permeability layers. The primary objective of this work is to reveal the mechanism of small-layer interference under different conditions, and clarify the influence of main control factors on the production of small-layer. The results show that the smaller the permeability ratio is, the weaker the difference in physical properties among layers along the vertical direction is. The reduction in the difference in seepage resistance decreases the dynamic interference among layers. The reduction in the water ratio among layers and shutting down high permeability layers can reduce the interlayer interference effectively. Increasing production pressure difference effectively improves the oil displacement efficiency of reservoirs with poor physical properties. A lower fluidity in the high permeability layers can effectively improve the oil displacement efficiency of other layers.
1 Introduction
In a heterogeneous reservoir with multiple layers producing oil, due to the vertical heterogeneity among layers, the interlayer and intralayer contradictions in the production process cannot be ignored. The interference phenomenon is serious, which leads to high production in the high-permeability layer(s), early water breakthrough time and high oil recovery (Huang et al., 2015; Liu et al., 2017; Xu et al., 2018; Renan et al., 2019). The low-permeability layers are inhibited by the high-permeability layers, leading to low oil production, late water breakthrough, which ultimately affects the total oil recovery (Cui and Zhao, 2010; Liu et al., 2019).
The research on the influence law of production of small-layers in multi-layer producing reservoirs mainly focuses on the interlayer interference mechanism and the establishment of small-layer production splitting models (Liu et al., 2012; Jiang et al., 2016; Zhao et al., 2016). The known interlayer interference mechanism is that, the greater the permeability ratio is, the greater the mutual influence among various flow units is, and the greater the oil displacement efficiency difference (Xu et al., 2019; Xu et al., 2020) is. When the permeability ratio exceeds a certain limit, production of the low-permeability layers is extensively reduced (Rahman and Mattar, 2007; Shen et al., 2018). Increasing the production pressure difference can enhance the production capacity of the low-permeability layer, and also make the small layer with higher starting pressure be used, thereby reducing the impact of interlayer interference (Larsen, 1981; Fetkovich et al., 1990). However, the existing research on the interlayer interference mechanism considers limited factors, the change of the minimum permeability and the water content of different layers are not considered. In terms of small-layer production splitting models: at present, the small-layer production splitting models mainly include the KH splitting model, KHK splitting model, KNK splitting model, and dynamic splitting model, etc. (Kuppe et al., 2000; Hu et al., 2018; Mi et al., 2019). However, the existing splitting models mainly focus on the study of the basic physical properties of the reservoir, do not consider the impact of dynamic factors on the production law of the small layer. It is difficult to accurately describe the impact of dynamic factors such as the different water content of each small layer on their productions.
Generally speaking, the research on the production influence law of small layers in a multi-layer production reservoir is not comprehensive. This article focuses on the development of a multi-layer production reservoir. For the problem of unclear understanding of the production law, through the development of a multi-tube displacement system, an indoor multi-layer water injection displacement experiment was carried out. The experiment simulated and evaluated the impacts of different factors on interlayer production, such as permeability ratios, different water cuts, shutting down high permeability layers, and changing production pressure and viscosity, etc. The experiment is designed to reveal the interference mechanism of the small layer under different conditions and clarify the law of influence of main control factors on the production of the small layer. The research results show that the degree of dynamic interference among layers decreases as the decreasing permeability ratio decreases, and increases as the increasing water cut. Shutting down the high-permeability layer, increasing the production pressure difference, and increasing the oil viscosity of the high-permeability layer can reduce vertical production differences and inter-layer interference. The research results can provide a more comprehensive understanding of mechanisms for the effective development of multi-layer heterogeneous reservoirs.
2 Multi-tube parallel displacement experiment
Aiming at solving the problems of strong inter-layer heterogeneity, serious interference, and unclear understanding in the development of heterogeneous reservoirs, we carried out multi-layer water flooding experiments to simulate and evaluate the impact factors on the inter-layer production, such as permeability ratio, water content, shutting down high-permeability layers, changing production pressure differences and fluid viscosity. The experiment was carried out to reveal the mechanism of small-layer interference under different conditions, and clarify the law of influence of main control factors on the production of small-layer.
2.1 Experimental equipment and procedures
The multi-pipe parallel displacement experiment equipment included a high temperature and high-pressure displacement device, which was mainly composed of a set of parallel sand filling pipes, a displacement pump, a constant temperature device, and an intermediate container. The main technical indicators included a temperature range of 20–200°C, and a pressure range of 0.1–50 MPa. The experimental process is shown in Figure 1. The specific experimental process steps are described as follows:
① Sand filling. According to the experimental requirements, select quartz sand of different meshes and fill the sand-filled tube for use.
② Cleaning. Clean the filled sand pipe to remove impurities.
③ Drying. Use nitrogen to dry the cleaned sand-filled pipe for later use.
④ Vacuuming. Use a vacuum pump to vacuum the blow-dried sand-packed pipe to saturate the fluid in the next step.
⑤ Saturating water and measuring porosity. Saturate the evacuated sand pipe with water, record the volume, pressure, rate of flow and other data, and calculate the porosity and permeability of the sand pipe.
⑥ Saturating oil. Use oil to displace water and inject oil into the sand-packed pipe to establish oil saturation.
⑦ Waterflooding. Displacing the sand-packing pipe with water.
⑧ Layer measurement. Simulate the production situation of different layers and split the production.
2.2 Experimental materials
To ensure the comparability in the experimental research, the experiment used 200–600 mesh quartz sand to fill eight sand-filling pipes, and then tested them in sequence. The basic parameters of each sand-filling pipe are shown in Table 1. In the experiment, the crude oil used 2# white oil (viscosity 2 mPa·s, density 800 kg/m3), 5# white oil (viscosity 5 mPa·s, density 820 kg/m3), 10# white oil (viscosity 10 mPa·s, density kg/m3); the injected water is pure water with a density of 1,000 kg/m3. After each experiment, the sand-filled tube was cleaned, and the procedures were repeated for the next experiment.
2.3 Experimental plan
In general, a parallel displacement experiment was designed to analyze the inter-layer interference phenomenon and reveal the interference mechanism. In the multi-pipe parallel flooding experiment, four sand-packed pipes were selected each time in different combinations. The permeability gradually decreased from the first layer to the fourth layer. The experiment separately considered the influence of factors such as permeability ratio, different water-bearing stages, production pressure difference, shutting down layers and crude oil viscosity on the interlayer production. The design of experiments was divided into five categories and 19 groups of experiments (Table 2).
2.4 Evaluation parameters
2.4.1 Production splitting coefficient
The ratio of the production of each layer to the sum of the total production of each layer, which can be shown as:
2.4.2 Cumulative oil production ratio
The ratio between the displacement amount of each sublayer to the total displacement amount of all production layers, which can be shown as:
Where α—Production splitting coefficient, dimensionless; Qi—The production of the i-th layer, m3; ΣQi—The total production of all layers, m3. Voi—The amount of oil displaced by the i-th layer, cm3; fi—Portion of cumulative oil production in i-th layer, %; n—Total floors.
3 Results and discussion
3.1 The influence of permeability ratio
3.1.1 Experimental plan
According to the actual loading situation of a single sand-filling pipe, and the designing requirements of the overall experimental plans, pipes with permeability from 48 mD to 2,412 mD were selected, and five groups of test plans with permeability ratios of 5.5, 8, 15, 25, and 50 were designed (Table 3). In these tests, the formation pressure was 14 MPa. The formation temperature was 90°C, and the experimental pressure difference was 1 MPa. When the water production rate at the outlet end of the low permeability layer reached 98%, the experiment stopped.
3.1.2 Experimental results and analysis
3.1.2.1 The impact of permeability ratio on the production of small layers when the minimum value of permeability is the same
The minimum permeability is 48 mD, and the permeability ratio is 5.5, 8, 15, 25, 50 respectively. The experiment results show that:
① From the perspective of fluid production, the fluid production of the high-permeability layer is absolutely dominant, reflecting the high-permeability layer’s contribution to fluid production (Table 4; Figure 2). When the permeability ratio is 5.5, the high-permeability layer accounts for more than 48% of the liquid production, and the low-permeability layer accounts for less than 7%. As the permeability ratio increases, the proportion of liquid production in the high-permeability layer increases, and the proportion of liquid production in the low-permeability layer gradually decreases. When the permeability ratio is 50, the proportion of liquid production in the high-permeability layer exceeds 74.32%, and the proportion of liquid production in the low-permeability layer is 1.15%.
② From the perspective of cumulative oil production, the cumulative oil production of the high-permeability layer has an absolute advantage (Table 4; Figure 3). When the permeability ratio is 5.5, the cumulative oil production of the high-permeability layer accounts for more than 42%, and the cumulative oil production of the low permeable layer accounts for less than 11%. With the increase of the permeability ratio, the cumulative oil production of the high-permeability layer also gradually increases, and the percentage of fluid production in the low-permeability layer also gradually decreases. When the permeability ratio is 50, the cumulative oil production of the high-permeability layer accounts for over 80.93%, and the cumulative oil production of the low-permeability layer accounts for 2.47%.
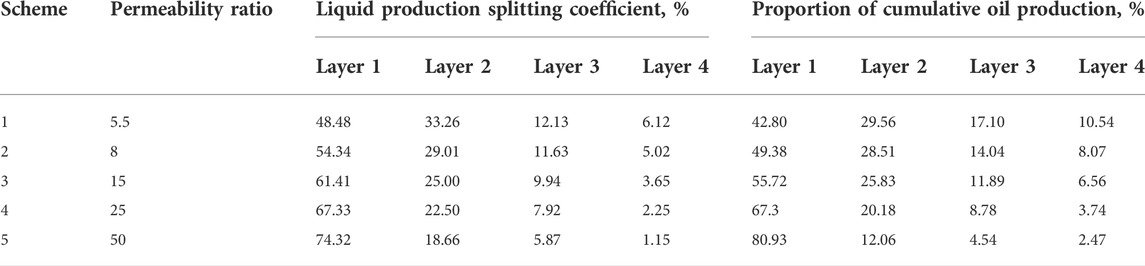
TABLE 4. Summary of the liquid production splitting coefficient and the cumulative oil production proportion of different permeability ratio.
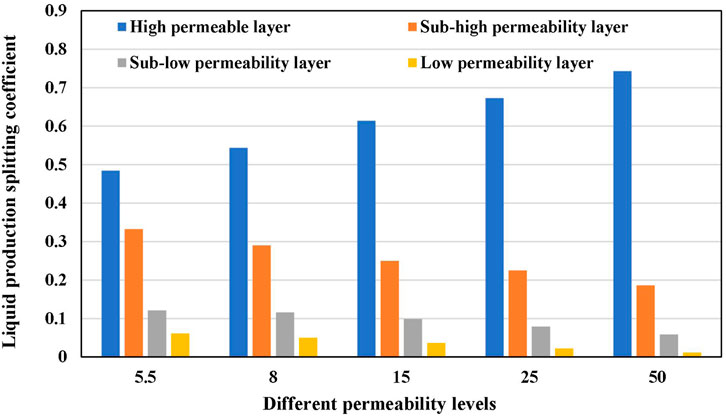
FIGURE 2. Comparison chart of splitting coefficients of produced liquid with different permeability ratios.
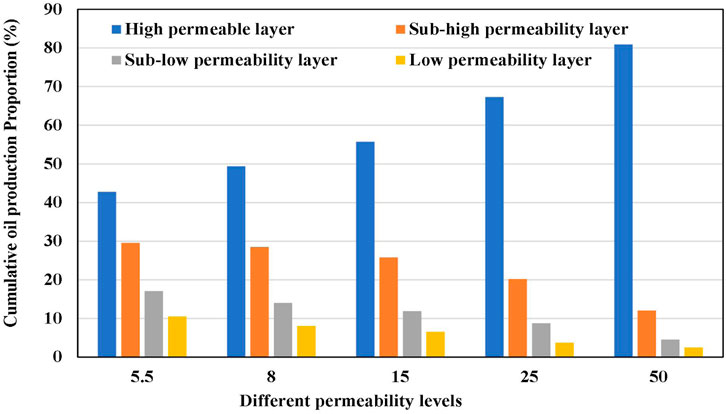
FIGURE 3. Comparison of the proportion of cumulative oil production in each layer with different permeability ratios.
The main reason is that the greater the permeability ratio in multi-layer production is, the greater the mutual influence among the flow in each layer is, and the higher the seepage resistance of the low-permeability layer is, and the lower the oil production. When the permeability ratio is small, the difference of physical properties among vertical layers in the flow resistance is reduced, and the difference in seepage resistance is reduced. The degree of utilization is more uniform, and the degree of dynamic interference among layers is reduced.
3.1.2.2 The influence of different minimum permeability on the production of small layers
The minimum permeability is 48 mD and 146 mD (Table 3), and the permeability of the other three layers keeps the same (Schemes 2, 4). The experiment results show that increasing the minimum permeability means the permeability ratio difference between the high-permeability layer and the low-permeability layer is reduced, which leads to the reduction of interlayer interference. When the minimum permeability changes from 48 mD to 146 mD, and the difference in liquid production ratio between the high-permeability layer and the low-permeability layer changes from 65.08% to 49.32%, with a decrease of 15.76%; the cumulative oil production ratio difference dropped from 63.56% to 41.31%, with a decrease of 22.25%.
3.2 The influence of different water content
3.2.1 Experimental test plan
The permeability ratio of 5.5 with relatively small interference is selected as the basis of the experimental scheme. The experiments consider the low water cut stage, the medium and low water cut stage, the medium-high water cut stage, the high water cut stage and the mixed water cut stage. The five groups of different water content combination schemes are shown in Table 5. In these tests, the formation pressure is designed to be 14 MPa, the formation temperature is designed to be 90°C, the experimental pressure difference is 1 MPa. When the water production rate at the outlet end of the low permeability layer reaches 98%, the experiment stops.
3.2.2 Experimental results and analysis
The experimental results show that the fluid production of the high permeability layer always has an absolute advantage (Table 5; Figure 4), which is mainly indicated in the following aspects:
① The difference between high and low permeability layers increases with the increase of water content. As the water content increases, the liquid production ratio of the high permeability layer increases from 56.92% in the low water cut stage to 75.54% in the high water cut stage. The low permeability layer is the opposite. It shows that the change in water content has aggravated the level difference among layers. The greater the difference in water content among layers is, the greater the difference in liquid production splitting coefficient is. The main reason is that the seepage resistance of the high-permeability layer is small. The water injection breaks through and the seepage resistance decreases early, which leads to an increase in liquid production and an increase in water content, and causes an increase in the water content difference of each layer in the longitudinal direction.
② In the mixed water-bearing stage, the fluid production of the high-permeability layer reaches the maximum value of 86.52%, mainly because the mixed water-bearing and high-water-bearing layer is a high-permeability layer, which leads to higher liquid production in the high-permeability layer. Therefore, reducing the difference in water content of each vertical layer is an important factor to effectively reduce the interference for the layers with more serious interference.
3.3 The effect of shutting down high permeability layer
3.3.1 Experimental test plan
The permeability ratio of 5.5 with relatively small interference was selected as the basis of the experimental plan to simulate the impact of closing the high permeability layer on the overall development of the reservoir. The experiments compared the effects of shutting down 1 layer, shutting down 1 and 2 layers, shutting down 1, 2, and 3 layers (Table 6). The layers were shutting down when the water content reaches 90. In these tests, the formation pressure was 14 MPa, the formation temperature was 90°C, the experimental pressure difference was 1 MPa. When the water production rate at the outlet end of the low permeability layer reached 98%, the experiment stopped.
3.3.2 Experimental results and analysis
The experiments were carried out by closing one, two and three high permeability layers respectively. The experimental results show that:
① From the perspective of liquid production, after closing the high permeability layer, the production splitting coefficient in the low permeability layer has increased significantly (Figures 5–7), and the permeability ratio has also decreased from 5.5 to 3, 2, and 1. After shutting down the high permeability layer, the difference in permeability among the layers is substantially reduced, thereby reducing the interference among the layers. As the permeability ratio decreases, the difference in physical properties among vertical layers decreases, the difference in seepage resistance decreases, the degree of production becomes more uniform, and the dynamic interference among layers decreases. Therefore, for the production layer with more serious interference, closing the high permeability layer to reduce the permeability ratio can effectively reduce the interference. It can be seen that closing the high permeability layer is an effective method to reduce interlayer interference.
② From the perspective of cumulative oil production, after shutting down the high-permeability layer, the cumulative oil production ratio of the minimum permeability layer increases from 10.54% to 25.25% (Figures 8–10). The crude oil in the low-permeability layer has been effectively produced, and the degree of production has been significantly improved. Therefore, by shutting down the high permeability layer, the oil displacement efficiency of the reservoir with poor physical properties can be effectively improved.
3.4 The influence of different pressure differences
3.4.1 Experimental test plan
The permeability ratio of 5.5 with relatively small interference was selected as the basis of the experimental program, and five sets of tests with different pressure differences of 1, 2, 3, 4, and 5 MPa were designed. In these tests, the formation pressure was designed to be 14 MPa, the formation temperature was designed to be 90°C, the experimental pressure difference was 1 MPa. When the water production rate at the outlet end of the low permeability layer reached 98%, the experiment stopped.
3.4.2 Experimental results and analysis
The experimental results (Table 7) showed:
① From the perspective of liquid production, as the production pressure difference increased from 1 to 5 MPa, the production splitting coefficient in the high permeability layer gradually decreases from 48.48% to 38.61% (Figure 11). And production splitting coefficient in the low permeability layer gradually increases, from 6.13% to 12.18%. It can be concluded that when the physical properties of each layer do not change, as the production pressure difference increases, the low-permeability layer in the vertical direction is used, and the difference in the degree of production among layers is reduced, showing that the dynamic interference among layers decreases with the increase in the production pressure difference. Therefore, for the production layer with more serious inter-layer interference, increasing the production pressure difference can effectively reduce the interference degree.
② From the perspective of cumulative oil production, as the production pressure difference increased from 1 to 5 MPa, and the proportion of cumulative oil production from high-permeability layers gradually decreased (Figure 12) from 42.8% to 37.25%, and the proportion of cumulative oil production from low-permeability layers gradually increased from 10.54% to 15.78%. It shows that increasing the production pressure difference enables the effective development of crude oil in the low-permeability layer, and the degree of production is significantly improved. By increasing the pressure difference, the oil displacement efficiency of reservoirs with poor physical properties can be effectively improved.
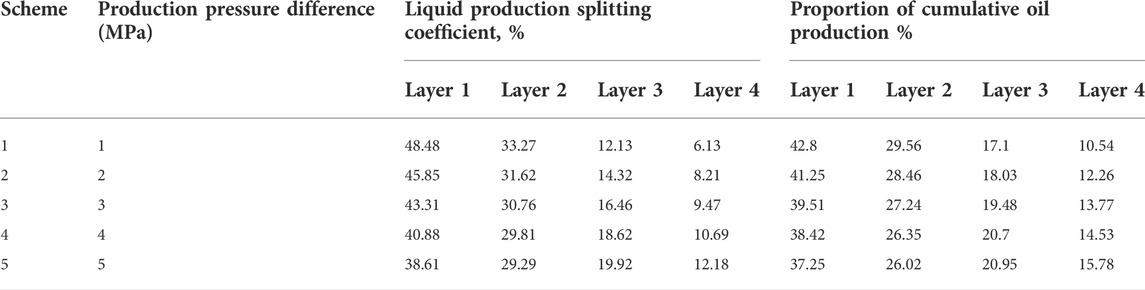
TABLE 7. Different pressure difference test liquid production splitting coefficient and accumulative oil production ratio table.
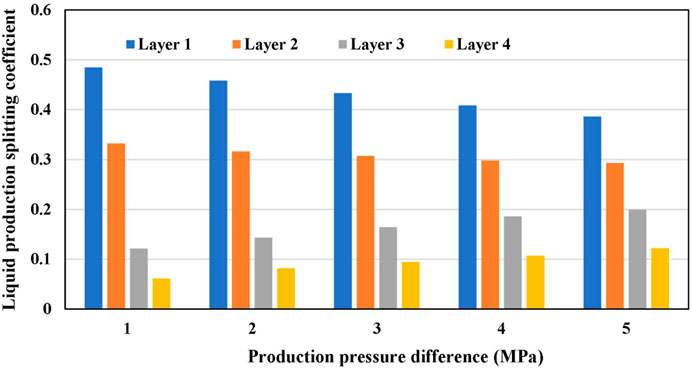
FIGURE 11. Comparison of splitting coefficients of liquid production in each layer with different pressure differences.
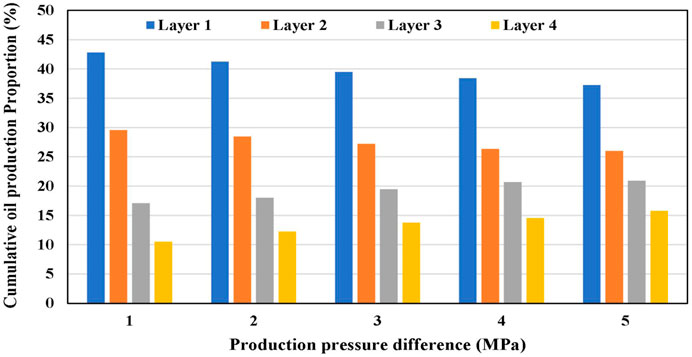
FIGURE 12. Comparison of the proportion of cumulative oil production in each layer with different pressure differences.
3.5 The influence of crude oil viscosity
3.5.1 Experimental test plan
Different viscosities were used to carry out comparative displacement experiments to simulate the interference mechanism of different crude oil viscosities. The permeability ratio of 5.5 with relatively small interference was selected as the basis of the experimental plan. The crude oil viscosity of the high permeability layer was designed to be 2, 5, and 10 mPa·s respectively. In these tests, the formation pressure was designed to be 14 MPa, the formation temperature was designed to be 90°C, the experimental pressure difference was 1 MPa. When the water production rate at the outlet end of the low permeability layer reached 98%, the experiment stopped.
3.5.2 Experimental results and analysis
Experiments were carried out with different crude oil viscosities in the highly permeable layer, and the experimental results (Table 8) showed that:
① From the perspective of fluid production, when the viscosity of crude oil in the high permeability layer increases from 2 mPa·s to 10 mPa·s, the proportion of fluid produced from the sub- high permeability layer rises faster and has an absolute advantage, and the proportion of fluid production increases from 33.27% to 68.98%. The proportion of fluid production in the high permeability, low permeability, and sub-low permeability layers gradually decreased, the proportion of fluid production in the high permeability layer decreased from 48.48% to 19.88%, and the proportion of fluid production in the low permeability and sub-low permeability layers decreases from 18.3% to 11.14%. The main reason is that the viscosity of the high permeability layer increases, and the sub-high permeability layer will be the main channel for liquid flow.
② From the perspective of cumulative oil production, as the viscosity of crude oil in the high-permeability layer gradually increases, the proportion of cumulative oil produced from the high-permeability layer gradually decreases from 42.8% to 26.28%, while the proportion of cumulative oil produced from other layers all rise. The percentage of cumulative oil production in the second-highest permeable layer increased from 29.56% to 31.51%, the proportion of the second-lowest permeability layer increased from 17.1% to 22.89%, and the lowest permeability layer increased from 10.54% to 19.32%. The main reason is that after the oil viscosity of the high-permeability layer increases, the fluidity decreases, the seepage resistance increases, and the production degree of the relatively low-permeability layer increases, which promotes the effective development of the low-permeability layer. The mobility of the permeable layer can effectively improve the oil displacement efficiency of other reservoirs.

TABLE 8. Different pressure difference test liquid production splitting coefficient and accumulative oil production percentage table.
4 Conclusion and understanding
Due to the strong inter-layer heterogeneity and serious interference in the development of multi-layer production reservoirs, the interlayer production law is unclear. By carrying out an indoor multi-tube parallel displacement experiment, the influence of different permeability ratios, water content, shutting down layers, different production pressure, and viscosity on the interlayer production was simulated and evaluated. This research is to reveal the mechanism of small-layer interference under different conditions and provide a basis for the effective development of multi-layer production reservoirs. The following understandings have been obtained during the study:
① When the permeability difference is from 5 to 50, the cumulative oil production of high permeability layer increases from 42% to 80.93%.
② During multi-layer production, the crude oil displaced by water injection mainly comes from the high-permeability layer. The high-permeability layer has a higher water injection utilization rate, which makes a large contribution to the overall recovery. The low permeability layer makes a small contribution to the overall recovery.
③ The degree of dynamic interference among layers decreases as the permeability difference decreases. It increases as the water cut increases. Shutting down the production layer, increasing the production pressure difference, and increasing the oil viscosity of the high-permeability layer can reduce the longitudinal production difference and alleviate vertical conflicts and reduce inter-layer interference.
④ According to the results of the production interference mechanism and the law of the small layers of multi-layer production reservoirs, it is suggested that the small layers with similar reservoir physical properties should be firstly combined and developed. Controlling the difference among layers in water content, and shutting down the high water-bearing layers can better reduce the contradictions among the layers. At the same time, for multi-layer producing reservoirs with large differences in physical properties, the production pressure difference can be increased because the utilization degree of low-permeability layers is low.
Data availability statement
The original contributions presented in the study are included in the article/supplementary material, further inquiries can be directed to the corresponding author.
Author contributions
XD, XH, SL, XZ, and QY contributed to conception and design of the study. CY and ZW organized the database. SL performed the statistical analysis. SL wrote the first draft of the manuscript. All authors contributed to manuscript revision, read, and approved the submitted version.
Conflict of interest
XD was employed by CNOOC China Limited Zhanjiang Branch. QY, SL, and CY were employed by CNOOC China Limited Hainan Branch.
The remaining authors declare that the research was conducted in the absence of any commercial or financial relationships that could be construed as a potential conflict of interest.
Publisher’s note
All claims expressed in this article are solely those of the authors and do not necessarily represent those of their affiliated organizations, or those of the publisher, the editors and the reviewers. Any product that may be evaluated in this article, or claim that may be made by its manufacturer, is not guaranteed or endorsed by the publisher.
References
Cui, C., and Zhao, X. (2010). Method for calculating production indices of multilayer water drive reservoirs. J. Pet. Sci. Eng. 75 (1–2), 66–70. doi:10.1016/j.petrol.2010.10.003
Fetkovich, M. J., Bradley, M. D., Works, A. M., and Thrasher, T. S. (1990). Depletion performance of layered reservoirs without crossflow. SPE Form. Eval. 5 (03), 310–318.
Hu, Q., Wang, X., Tan, Y., Yang, H., Zhang, F., and Lu, M. (2018). The production split method in multilayer reservoir based on grey relational analysis. IOP Conf. Ser. Earth Environ. Sci. 113 (1), 012018. doi:10.1088/1755-1315/113/1/012018
Huang, S., Kang, B., Cheng, L., Zhou, W., and Chang, S. (2015). Quantitative characterization of interlayer interference and productivity prediction of directional wells in the multilayer commingled production of ordinary offshore heavy oil reservoirs. Pet. Explor. Dev. 42 (4), 533–540. doi:10.1016/s1876-3804(15)30046-x
Jiang, W., Wu, C., Wang, Q., Xiao, Z., and Liu, Y. (2016). Interlayer interference mechanism of multi-seam drainage in a CBM well: an example from Zhucang syncline. Int. J. Min. Sci. Technol. 26 (6), 1101–1108. doi:10.1016/j.ijmst.2016.09.020
Kuppe, F., Chugh, S., and Connell, P. (2000). “Material balance for multi-layered, commingled, tight gas reservoirs,” in SPE/CERI gas technology symposium. Society of petroleum engineers. doi:10.2118/59760-MS
Larsen, L. (1981). “Wells producing commingled zones with unequal initial pressures and reservoir properties,” in SPE annual technical conference and exhibition. Society of petroleum engineers. doi:10.2118/10325-MS
Liu, G., Meng, Z., Luo, D., Wang, J., Gu, D., and Yang, D. (2019). Experimental evaluation of interlayer interference during commingled production in a tight sandstone gas reservoir with multi-pressure systems. Fuel 262, 116557. doi:10.1016/j.fuel.2019.116557
Liu, H., Pang, J., Wang, X., Xinan, Y., and Qing, L. (2012). Analysis of interlayer interference and research of development strategy of multilayer commingled production gas reservoir. Energy Procedia 16, 1341–1347. doi:10.1016/j.egypro.2012.01.214
Liu, Y., Luo, X., Kang, K., Li, T., Jiang, S., Zhang, J., et al. (2017). Permeability characterization and directional wells initial productivity prediction in the continental multilayer sandstone reservoirs: a case from penglai 19-3 oil field, bohai bay basin. Pet. Explor. Dev. 44 (1), 97–104. doi:10.1016/s1876-3804(17)30012-5
Mi, L., Hu, X., Jia, Y., and Liu, Q. (2019). “A novel dynamic production splitting method based on the catastrophe theory,” in SPE middle east oil and gas show and conference. Society of petroleum engineers. doi:10.2118/194968-MS
Rahman, N. M. A., and Mattar, L. (2007). New analytical solution to pressure transient problems in commingled, layered zones with unequal initial pressures subject to step changes in production rates. J. Pet. Sci. Eng. 56 (4), 283–295. doi:10.1016/j.petrol.2006.10.002
Renan, V., Sinesio, P., and Barreto, A. (2019). Modeling falloff tests in multilayer reservoirs. J. Pet. Sci. Eng. 174, 161–168. doi:10.1016/j.petrol.2018.10.101
Shen, F., Cheng, L., Sun, Q., and Huang, S. (2018). Evaluation of the vertical producing degree of commingled production via waterflooding for multilayer offshore heavy oil reservoirs. Energies 11 (9), 2428. doi:10.3390/en11092428
Xu, J., Chen, Z., and Li, R. (2020). Impacts of pore size distribution on gas injection in intraformational water zones in oil sands reservoirs. Oil Gas. Sci. Technol. –. Rev. IFP. Energies Nouv. 75, 75. doi:10.2516/ogst/2020047
Xu, J., Wu, K., Li, R., Li, Z., Li, J., Xu, Q., et al. (2018). Real gas transport in shale matrix with fractal structures. Fuel 219, 353–363. doi:10.1016/j.fuel.2018.01.114
Xu, J., Wu, K., Li, R., Li, Z., Li, J., Xu, Q., et al. (2019). Nanoscale pore size distribution effects on gas production from fractal shale rocks. Fractals 27 (08), 1950142. doi:10.1142/s0218348x19501421
Keywords: multi-layer co-production heterogeneous reservoirs, interference mechanism, multi-pipe parallel displacement experiment system, water injection flooding experiments, oil displacement efficiency
Citation: Deng X, Huang X, Ye Q, Li S, Yu C, Zhang X and Wang Z (2022) Experimental research on production law of multilayer heterogeneous reservoirs. Front. Earth Sci. 10:990554. doi: 10.3389/feart.2022.990554
Received: 10 July 2022; Accepted: 21 July 2022;
Published: 19 August 2022.
Edited by:
Jinze Xu, University of Calgary, CanadaReviewed by:
Wenlian Xiao, Southwest Petroleum University, ChinaBo Yang, Chengdu University of Technology, China
Copyright © 2022 Deng, Huang, Ye, Li, Yu, Zhang and Wang. This is an open-access article distributed under the terms of the Creative Commons Attribution License (CC BY). The use, distribution or reproduction in other forums is permitted, provided the original author(s) and the copyright owner(s) are credited and that the original publication in this journal is cited, in accordance with accepted academic practice. No use, distribution or reproduction is permitted which does not comply with these terms.
*Correspondence: Xiaoliang Huang, MTIxMzQ3MDc0QHFxLmNvbQ==