- 1School of Smart City, Chongqing Jiaotong University, Chongqing, China
- 2Chongqing Geomatics and Remote Sensing Center, Chongqing, China
- 3School of Civil Engineering, Chongqing Jiaotong University, Chongqing, China
Defining a reasonable and feasible watershed ecological compensation threshold is the key to protecting watershed ecological functions and maintaining the sustainable utilization of watershed ecosystems. However, many studies have obtained compensation values that are too high to promote the implementation of ecological compensation policies. This study chose the upper reaches of the Yangtze River as the research area, taking water resources closer to people’s daily needs as the evaluation object. Based on the InVEST (Integrated Valuation of Ecosystem Services and Trade-offs) model and grey water footprint method, the ecological compensation threshold model for water resources was established. Combined with the eco-compensation priority sequence coefficient identification of protected areas and beneficiary areas and allowed for the measurement of the watershed ecological compensation value in 2015 and 2020. Finally, compare the advantages and disadvantages of different ecological compensation calculation methods, compare the gap between different watershed ecological compensation standards and the theoretical threshold globally. The results showed that from 2015 to 2020, the value of the water content in the upper reaches of the Yangtze River increased, while the value of the grey water footprint decreased. The classified watershed ecological compensation beneficiary areas were mainly concentrated in the central-eastern and southern parts of the upper Yangtze River, while the ecological compensation protected areas were concentrated in the western and northwestern parts. The mean absolute values of the watershed ecological compensation thresholds for each prefecture-level city and state ranged from 0.43 to 24.63 billion CNY in 2015 and from 0.67 to 41.60 billion CNY in 2020, which were close to the actual values. Among the different land-use types, the water conservation service value per unit area of shrubs was the highest. The lower limit value of watershed ecological compensation calculated using the grey water footprint method was closer to the amount of compensation in practice than was the commonly used opportunity cost method. The findings of the study can help improve the watershed ecological compensation mechanism in the upper Yangtze River region, promote win–win cooperation among transboundary watershed areas, and form a harmonious and stable green development model.
1 Introduction
With accelerated urbanization worldwide, economic development brings rapid population growth (He et al., 2021), and the demand for industrial and domestic water increases (Florke et al., 2018; Garrick et al., 2019). Additionally, climate change in nature brings extreme weather effects that exacerbate water scarcity by causing water resources to be unevenly distributed in time and space (Greve et al., 2018; Schilling et al., 2020; Schilling et al., 2020). In many developing countries, due to technological constraints, the long-term economic development model based on high energy consumption and pollution has caused damage to the regional ecological environment, resulting in the decline of ecosystem functions and frequent problems such as water and environmental pollution (Bekturganov et al., 2016; Best, 2019; Wang et al., 2021). And a healthy watershed ecosystem is the basis for abundant natural resources and social wealth for human beings (Grill et al., 2019). China has attached great importance to the protection of water ecology and the environment in recent years and has actively adopted environmental protection measures and implemented economic transformation and industrial restructuring to gradually change the situation of water pollution and water shortages (Chen and Qian, 2020; Wan et al., 2022). According to Tang et al. (2022), China’s wastewater treatment rate has exceeded 90% in the past 20 years, and significant results have been achieved in surface water environmental pollution management. Although there is still a trend of deteriorating water quality in some watersheds, the direction of water pollution management is reasonable. The Yangtze River, the longest river in Asia and one of the most famous rivers in China, is rich in water resources and plays an important role in China’s history, culture and economy, especially in the upper reaches of the Yangtze, where it has extremely important ecological and economic and social values (E et al., 2018). For thousands of years, the Yangtze River has spanned the east and west of China, serving multiple functions such as transportation, water supply and ecological safety barrier, making it China’s “Golden Waterway” and an important link in the “Silk Road” (Xu et al., 2021; Yang et al., 2021). However, as in many developing countries, the erosion and water pollution problems caused by the crude economy in the past have greatly hindered the path of green development (Xu et al., 2022). For the sustainable development of water resources, the Chinese government has introduced a series of related policies such as the Outline for the Development of Yangtze River Economic Belt and the Yangtze River Protection Law, making the restoration of the Yangtze River ecological environment a top priority (Liu and Yuan, 2022). The upper Yangtze River is the most fragile area of the basin’s ecological environment, and its water ecological environment security is the basis of the ecological environment quality of the whole basin and the key to ensure the smooth implementation of the relevant national strategic plans (Wang et al., 2022). How to reconcile economic development and the sustainable use of ecosystem services is a major long-term challenge. The ecological protection of water resources in the upper reaches of the Yangtze River can be strengthened through the establishment of an ecological compensation system, which can help alleviate the contradiction between rapid local economic development and excessive resource use, and can narrow the large gap between the economic development level of the eastern and western parts of China. (Jiang et al., 2022).
Ecological compensation, also known as payment for environmental services, has become a hot topic in the 21st century as a mechanism for transforming environmental externalities and nonmarket values into internal real financial resources for environmental stakeholders, and its theoretical and practical research has been widely used in countries around the world (Engel et al., 2008; Farley and Costanza, 2010; Gastineau et al., 2021). The application of ecological compensation in different contexts is not entirely consistent and is generally agreed upon as a voluntary and quantifiable transaction between the compensating party and the compensated party, an institutional arrangement that regulates stakeholder interests primarily through economic means (Wen et al., 2011). Its purpose is to protect the sustainability and stability of ecosystem services and to promote the harmonious development of humans and nature. Funding sources currently include government financial compensation supplemented by market compensation, with an increasing number of nongovernmental organizations (NGOs) participating as market-based mechanisms are improved (Kinzig et al., 2011). Ecological compensation in China is in a preliminary stage and is piloted in several regions, such as transboundary compensation involving the Xin’an River (Sheng and Han, 2022) and Chishui River (Qiu and Zhai, 2014) and market trading involving Zhejiang Province (Wang H. et al., 2010). Among them, watershed ecological compensation has been gradually promoted in China to protect the ecological benefits of shared water resources and mitigate transboundary water pollution conditions (Gao et al., 2019). Ecological compensation for highly spatially integrated and connected watersheds requires upstream and downstream consultation for joint protection, and how to formulate ecological compensation scientifically and rationally for watersheds is the key to making sound ecological management decisions within watersheds (Gao et al., 2020). Academic research on ecological compensation has mainly focused on the determination of ecological compensation areas and the calculation of ecological compensation criteria.
How to identify protected areas and beneficiary areas is the primary issue in establishing ecological compensation mechanisms, and this information directly determines the science and effectiveness of compensation. Related studies, e.g., Gastineau et al. (2021), discussed the mechanism of the spatial distribution, environment and land cost factors affecting the location of ecological compensation. Hu et al. (2022) used a mathematical model to determine the changes in spatial and temporal patterns of wind erosion prevention services provided by desert ecosystems from recharge areas to benefit areas from the perspective of ecosystem service flows. Villarreal-Rosas et al. (2022) also considered the impact of land-use change on spatial and temporal changes in the supply, demand, and flow of ecosystem services in different beneficiary sectors from the perspective of ecosystem service flows. Schirpke et al. (2014) applied a spatially explicit modeling approach to determine the specific location distribution of beneficiary areas and protected areas for ecosystem services. Garau et al. (2021) focused on the spatial distributions of service supply and demand characteristics among water ecosystems to help identify sources of conflict and improve stakeholder group coordination. Yu and Xu. (2016) helped identify the supply and demand of services by constructing a complete framework of ecological compensation mechanisms to identify recipients and payers. Burdon et al. (2022) constructed a new stakeholder-driven participatory mapping approach to analyze the logical relationship between stakeholders and natural capital from multiple perspectives. Xi and Jing (2021) used the ecological footprint method to distinguish ecological deficit areas from ecological surplus areas and found that eastern China was mostly classified as an ecological deficit area, while central and western China were mostly classified as an ecological surplus area. However, identifying the location of protected areas and beneficiary areas for ecosystem services, as well as identifying those who use and protect them, remain key challenges in the field (Bagstad et al., 2013). Many studies have used a separate modeling framework to distinguish ecological compensation areas, identifying ecological compensation protected areas and beneficiary areas primarily through stakeholder and natural resource participation.
The measurement of ecological compensation standards is the core issue, and reasonable ecological compensation standards are directly related to compensation effects and policy feasibility. Many studies have carried out large-scale, high-specification calculations of ecological compensation standards to maintain the use of their natural resources (Hou et al., 2021). For example, under the full consideration of the service functions of each ecosystem, the ecosystem service value before and after the change in regional land use/cover has been evaluated, and the influencing factors and temporal and spatial changes have been analyzed to provide optimized information on ecosystem services for land-use planning (Aziz, 2020; Ma et al., 2020; Wu et al., 2020; Anley et al., 2022). There are many other methods used to measure ecological compensation for various ecosystems. Gu (2017) established an ecological compensation standard evaluation model for the Zhoushan Islands New Area using the theory related to ecological compensation for tourism and the ecological footprint composition method. Fu et al. (2017) established an ecological compensation standard calculation system for the sustainable development of watershed agriculture based on the convertibility of energy and price. Rao et al. (2014) considered the spatial variation in ecological services by establishing the criteria of marine ecological damage compensation to suppress unsustainable development that causes ecological damage. One of the most frequently used models to calculate ecosystem services is the InVEST (Integrated Valuation of Ecosystem Services and Trade-offs) model, which has had good applications in hydrological ecosystem services (e.g., Cong et al., 2020; Benra et al., 2021; Zhang et al., 2021; McMahon et al., 2022). There are also many studies on watershed ecosystems that use water quality and quantity as the basis for compensation measurements, and in the context of rapid global economic development, which has resulted in a large increase in urban water demand and a large amount of unreasonable sewage discharge, it is urgent to consider the water environment as a compensation target; furthermore, ecological compensation based on pollution damage can be used to better control and monitor the quality of the water environment (Liu et al., 2016; Guan et al., 2021). Related research also includes the water footprint theory that can be used to quantify the supply and demand of water ecosystem services, which can be divided into green water (rainwater stored in the soil), blue water (surface and groundwater) and grey water (polluted water in production) (Chapagain and Hoekstra, 2011; Pacetti et al., 2021). Applying the Total Pollutant Control-Water Resource Value model, the concept of diluted water was introduced to quantify the basin ecological compensation criteria by combining the total pollutant and water resource value (Guan et al., 2018). In addition, the integrated pollution index method was used to calculate the transboundary water quality compensation standard (Hao et al., 2021), calculate the compensation index of pollution loss in a watershed based on the pollution loss rate and energy value method (Guan et al., 2021), and establish a pollutant abatement differential game model for transboundary watersheds to obtain the optimal compensation strategy (Jiang et al., 2019a). The study of ecological compensation in watersheds is dedicated to proposing a reasonable ecological compensation standard measurement method based on water allocation and water quality control, and the grey water footprint approach can adequately integrate water quality and quantity (Chai and Chen, 2022). The application of the grey water footprint to ecological compensation in watersheds is still very limited. There are several methods for measuring ecological compensation standards, but the results of these methods often correspond to a fixed ecological compensation standard value, and the results often differ significantly from the actual watershed compensation values, making it difficult to provide direct guidance for actual compensation cases. The ecological compensation threshold can be used to calculate the ecological compensation standards of different time periods and different judgment standards in the same area, and a range interval can be obtained to represent the fluctuating interval of ecosystem service value within the ecosystem carrying capacity of the study area, which can be judged by integrating more factors.
How to combine the upper and lower limits of ecological compensation to calculate the ecological compensation threshold is a difficult problem. However, the ecological compensation threshold is closely related to ecological benefits, and both ecological benefits and ecological impacts must be considered when setting ecological compensation threshold. If the ecological compensation threshold is set too high, ecological policies cannot be achieved, and if the ecological compensation threshold is set too low, ecological environmental protection effects cannot be achieved. However, ecological compensation actions in the past have often been criticized for low levels of achievement, where planned conservation actions were only partially achieved or not achieved at all, while development activities were clearly still underway. Brown et al. (2013) investigated the compliance of 245 conditions related to ecological compensation in 81 case studies in New Zealand according to the Resource Management Act of 1991, and the results showed that 35.2% of the requirements were not met. Therefore, determining a reasonable ecological compensation threshold has become a pressing issue for the integrity of ecological compensation mechanisms. For example, Simmonds et al. (2020) categorized the pathways to achieve specific biodiversity characterization targets as net gain, no net loss, or (rarely) managed net loss by specifying different types and amounts of ecological compensation to ensure the achievement of different targets. De Mello et al. (2021) developed a novel offsetting methodological approach for the compensation of legal reserves. If landowners fail to meet the legal requirements on their land, they may compensation in other equivalent properties. Hou et al. (2021) found that grassland ecological compensation policy improved grassland quality to a small extent and had a positive impact on income, but it exacerbated existing income inequalities among local pastoralists. Jiang et al. (2019b) constructed a stochastic differential game model to analyze transboundary pollution control options between ecological compensation beneficiary areas and protected areas and found that the ecological compensation mechanism provided long-term, effective incentives only when the marginal losses of environmental damage in the compensating region were more than twice those of the compensated region. Qin and Wang (2022) constructed an evolutionary game model to define the ecological compensation threshold, which showed that from the government’s perspective, the social benefits must exceed $10.69 million per year; however, from the perspective of enterprises, government subsidies should be less than $21.38 million per year. Therefore, using a combination of ecological benefits and ecological impacts to measure the ecological compensation threshold is a valid and reasonable approach.
Although the determination of ecological compensation areas and the calculation of ecological compensation criteria have been richly discussed, the following two fundamental aspects have long been left to be enriched and are also the main research questions of this study. 1) How can ecological compensation protected areas and beneficiary areas be reasonably determined? 2) How can the ecological compensation threshold model be constructed and how can the upper and lower thresholds of ecological compensation be accurately grasped? To solve these two problems, this study adopts quantitative methods. 1) The concept of eco-compensation priority sequence is invoked, and the InVEST model is applied to obtain the non-market value of ecosystem services, which is divided by the local GDP to obtain the ratio. Revised it in combination with the income of farmers of major related interest groups, to judge the ecological compensation protected areas and benefit areas according to the priority. 2) According to water conservation and grey water footprint value, a watershed ecological compensation threshold model is constructed. From the perspectives of watershed ecological resource value and water quality and water quantity damage value. It indicates that the ability of the region to supply the value of the water conservation service is directly taken as the upper limit of ecological compensation, and the grey water footprint compensation result of the “zero-sum model” is introduced as the lower limit of ecological compensation. Thus, the spatial differences and evolutionary patterns of this ecological compensation threshold are clarified. The conclusion can assist the establishment of the ecological compensation system in the upper Yangtze River, provide a reference for the management of regional ecological land types, and contribute to the conservation and sustainable use of water resources.
The rest of this study is structured as follows. In Section 2, the natural conditions of the upper Yangtze River and the policy background of ecological compensation are introduced, and the method of constructing the ecological compensation threshold model is explained. In Section 3, the specific results generated by each phase of the method are analyzed. In Section 4 discusses the comparative analysis of compensation cases and results of different ecosystems, different calculation methods and different river basins. The last part of section 5 is the conclusion and prospect.
2 Data and methods
2.1 Study area and data sources
The upper reaches of the Yangtze River refer to the reach from the source of the Yangtze River to the main stream of the Yichang, with a total length of approximately 4,511 km, accounting for approximately 70% of the total length of the Yangtze River. The main tributaries include the Yalong River, Minjiang River, Jialing River and Wujiang River, covering nine provinces (municipalities and autonomous regions), including Qinghai, Tibet, Sichuan, Gansu, Shaanxi, Yunnan, Guizhou, Chongqing and Hubei, accounting for approximately 58.9% of the total area of the Yangtze River basin (Hong et al., 2019), as shown in Figure 1. This plays an important role in the safety of water resources and the water environment as well as the sustainable development of the economy and society in the Yangtze River basin. However, rivers in the basin are prone to natural disasters due to their large gap, changeable climate and landforms. For example, global climate change causes flooding in the lower reaches of the river when rainfall is abundant in summer, and the melting of glaciers and the decline of the snow line make it a fragile ecological environment and sensitive area with global strategic significance. In addition, long-term human disturbance has reduced the ecosystem function of the river basin, which has seriously weakened the water conservation function of the system. It is imperative to establish and improve an ecological compensation mechanism for water conservation in the upper Yangtze River basin. By 2016, the “Yangtze River Economic Belt Development Outline” aimed to guide the coordinated development of ecological environmental protection and the green economy in the Yangtze River Economic Belt. Following the promulgation of the “Guiding Opinions on Establishing and Improving the Long-term Mechanism of Ecological Compensation and Protection in the Yangtze River Economic Belt” in 2018, a special fund was set up to subsidize the ecological protection of the Yangtze River Economic Belt to ensure that the ecological compensation mechanism of the Yangtze River Economic Belt could be effectively realized for a long time. In December 2020, the National People’s Congress meeting passed the “Protection Law of the People’s Republic of China”, which continuously strengthens the protection of the ecological environment in the Yangtze River basin (Qiao et al., 2021). The data from 2015 to 2020 were selected for analysis, and the 5 years centered on the construction of an ecological civilization and the improvement of environmental quality were regarded as the new stage of environmental protection implementation. Analyzing and calculating the changes in ecological compensation in river basins, evaluating the effects of environmental protection implementation, and promoting the sustainable and healthy development of environmental protection were objectives of this research.
The data sources used in the study are as follows: 1) Land use remote sensing monitoring data: The land use remote sensing monitoring data were selected from the Resource and Environment Science and Data Center of the Chinese Academy of Sciences (https://www.resdc.cn/) with a spatial resolution of 1 km. The downloaded raw data were spliced and cropped as required for the study area, and the blank values were interpolated. 2) Soil data: Soil data in which soil hydraulic conductivity saturation rates were calculated from sand content, clay content, chalk content soil organic matter and soil salinity by soil-plant-air-water (SPAW) model. And the rest of the data were obtained from the Cold and Arid Regions Science Data Centre (http://www.ncdc.ac.cn/auth/register) 1:1 million data of China’s soil in 2017. 3) Meteorological data: The meteorological data mainly include annual average precipitation, sunshine hours and monthly average temperature data for each prefecture-level city, and are obtained from the daily dataset of Chinese meteorological element station observations provided by the Resource Science and Data Centre of the Chinese Academy of Sciences (https://www.resdc.cn/), and collated according to the stations included in the upper Yangtze River study area.4) DEM data: The DEA data is mainly used to calculate the number of catchment areas and percentage of slope in the study area through ArcGIS hydrological analysis to obtain topographic index data. The data were obtained from the topographic and geomorphological data from the Resource Science and Data Centre of the Chinese Academy of Sciences (https://www.resdc.cn/) with a spatial resolution of 90 m. 5) Socio-economic data: Socio-economic data include data on population, GDP, grain output, crop sown area, economic forestry output, afforestation area and fishery output of each prefecture-level city (autonomous prefecture) in 2015 and 2020, as well as data on nitrogen fertilizer application, livestock breeding, industrial and domestic wastewater emissions of various pollutants, total water resources, etc. From the regional 2015 and 2020 Statistical Yearbook, National Economic and Social Development Statistical Bulletin, Environmental Statistics Bulletin and Water Resources Bulletin, as well as from applications to relevant authorities for public access.6) Vector data: All administrative boundary data, vector data of ecological function reserves and water systems were obtained from the Resource Science and Data Centre of the Chinese Academy of Sciences (https://www.resdc.cn/), and the vector data within the upper reaches of the Yangtze River were obtained by intersecting and cropping with ArcGIS software.
2.2 Methodology
2.2.1 Model of water content in the upper Yangtze River
In this study, the InVEST model was used to assess the water content in the upper reaches of the Yangtze River. The input data required for calculating water yield in the water supply module of the InVEST model include the maximum soil root depth data, average annual precipitation data, plant available water data, average annual potential evapotranspiration data, land use/cover data, watershed vector data, different surface cover types corresponding to the biophysical coefficient table and Zhang coefficient, which has a value of 4.433 (Liu J. et al., 2019).
where WR denotes the water content (mm); TI denotes the topographic index;
2.2.2 Calculation of grey water footprint in the upper Yangtze River
Based on the ecological and environmental loss value of water resources in the basin, an ecological compensation calculation approach based on water quality and quantity was considered to quantify the relationship between compensation beneficiary areas and compensation protected areas in the basin. The grey water footprint focuses on water quality and pollution discharge and represents the volume of fresh water needed to dilute pollutants to meet water quality standards (De Girolamo et al., 2019). It can be regarded as the cost of water quality improvement and effectively combines water quality and quantity to be considered in the calculation of the loss value of the environment. Referring to the formula of the grey water footprint described in the “Water Footprint Evaluation Handbook” (Hoekstra and Chapagain, 2007), which is determined by the pollutant that produces the largest grey water footprint in agricultural, industrial, and domestic uses. The three grey water footprints are added together to obtain the total regional grey water footprint value.
2.2.2.1 Agriculture grey water footprint
The agricultural grey water footprint includes planting and breeding. Nitrogen pollution is the main problem faced by rivers in China (Rong et al., 2016), so nitrogen fertilizer application (e.g., total nitrogen, TN) was chosen as the main pollutant in the plantation industry. The large amount of chemical oxygen demand (COD) and TN contained in the excrement of representative livestock (cattle, sheep, pig and poultry) was chosen as the main source of pollution in the breeding industry. Finally, the grey water footprint produced by different pollutants was compared, and the maximum was taken as the agricultural grey water footprint. The calculation formulas are as follows:
where
2.2.2.2 Industrial grey water footprint
Industrial grey water footprint two major pollutants, COD and ammonia nitrogen, were selected as representatives, and the maximum values were taken after separate calculations using the following equations:
where
2.2.2.3 Domestic grey water footprint
The domestic grey water footprint was also calculated using two types of pollutants, COD and ammonia nitrogen, as indicators, and the formulas are as follows:
where
2.2.2.4 Regional grey water footprint
The regional grey water footprint is the sum of the industrial, agricultural and domestic grey water footprints of the entire region.
where
According to the primary emission standard in the Comprehensive Emission Standard for Pollutants (GB8978-1996),
2.2.3 Eco-compensation priority sequence calculation in the upper Yangtze River
The eco-compensation priority sequence (ECPS) refers to the ratio of the regional nonmarket value of ecosystem services per unit area to GDP per unit area, which indicates the demand intensity of ecological compensation in different regions (Wang N. J. et al., 2010). The value of water conservation services is mainly reflected in the value generated by water storage and water conservation. The value of water content services for each grid was simulated using the shadow engineering method to build water facilities with a storage capacity comparable to the ecosystem water content, and the unit cost required to build such water facilities was used to estimate the unit value of water conservation services. With reference to the results of Gao et al. (2020), the price index discount showed that the value was 7.29 CNY/m3 in 2015 and 10.92 CNY/m3 in 2020. Taking the main stakeholders in the compensation area into account in ecological protection, the participation of ecological stakeholders can make the compensation priority more reasonable (Sterling et al., 2017). The farmers’ collective is the most closely related to the natural ecosystem and is the main compensation object in ecological compensation. To distinguish the main compensation protection areas and beneficiary areas in the upper reaches of the Yangtze River, the per capita disposable income of rural residents was substituted into the ECPS for correction. According to the ECPS, the compensation carrier was divided, and the area higher than the average value was defined as the protected area, while the area lower than the average value was defined as the beneficiary area. The specific expressions are as follows:
where
2.2.4 Model of the ecological compensation threshold in the upper Yangtze River
Using the InVEST model to evaluate the water content of the study area in 2015 and 2020, the upper limit of compensation in the study area was based on the ecological benefits and used to reflect the water storage and preservation value of the water ecosystem in the upper reaches of the Yangtze River; specifically, the water conservation value was chosen to measure it. The lower limit of compensation in the study area was based on the loss value of the ecological environment, the cost of maintaining and improving water quality and quantity was the basis for evaluating the loss of water resource value in the basin, and the grey water footprint method was chosen to measure it. The market value of freshwater resources in each region was chosen, and the zero-sum model (Liu H. G. et al., 2019) was used to set all ecological compensation in the basin to be self-consistent; that is, all administrative districts in the basin compensated each other, and the ecological compensation demand intensity coefficients of each prefecture-level city were combined to make the lower limit value closer to the actual situation. The ecological compensation demand intensity coefficient ti was then obtained by ECPS calculation.
The specific expression for the upper limit of the threshold is as follows:
The per capita ecological surplus (deficit) of water resources in the whole basin is as follows:
The per capita ecological surplus (deficit) of water resources in the region is as follows:
The specific expressions for the lower limit of the threshold are as follows:
where population is
3 Results
3.1 Results of the upper and lower limits of ecological compensation in the upper Yangtze River
3.1.1 The upper Yangtze River water containment
The water yield module of the InVEST model was applied to obtain the water yield of the upper Yangtze River ecosystem, as shown in Figure 2. In 2015, the unit water yield of the upper Yangtze River ranged from 0 to 1,667.13 mm, and in 2020, the unit water yield of the upper Yangtze River ranged from 0 to 1840.22 mm, with the range interval increasing by 173.09 mm. The water yield of a watershed was mainly influenced by precipitation and evapotranspiration. The higher the precipitation, the higher the water yield, and the higher the evapotranspiration, the lower the water yield in the area. The spatial distribution characteristics of the results show a gradual increase in water yield in the upper Yangtze River from northwest to southeast. The high value of water yield was concentrated in the southeast of the upper Yangtze River and near the Chengdu Plain, where precipitation was abundant, while the low value is mainly distributed in Qinghai, Tibet and Gansu, where evapotranspiration was high and precipitation was low. Land use/cover type, soil properties, elevation and slope also directly or indirectly influence water yield.
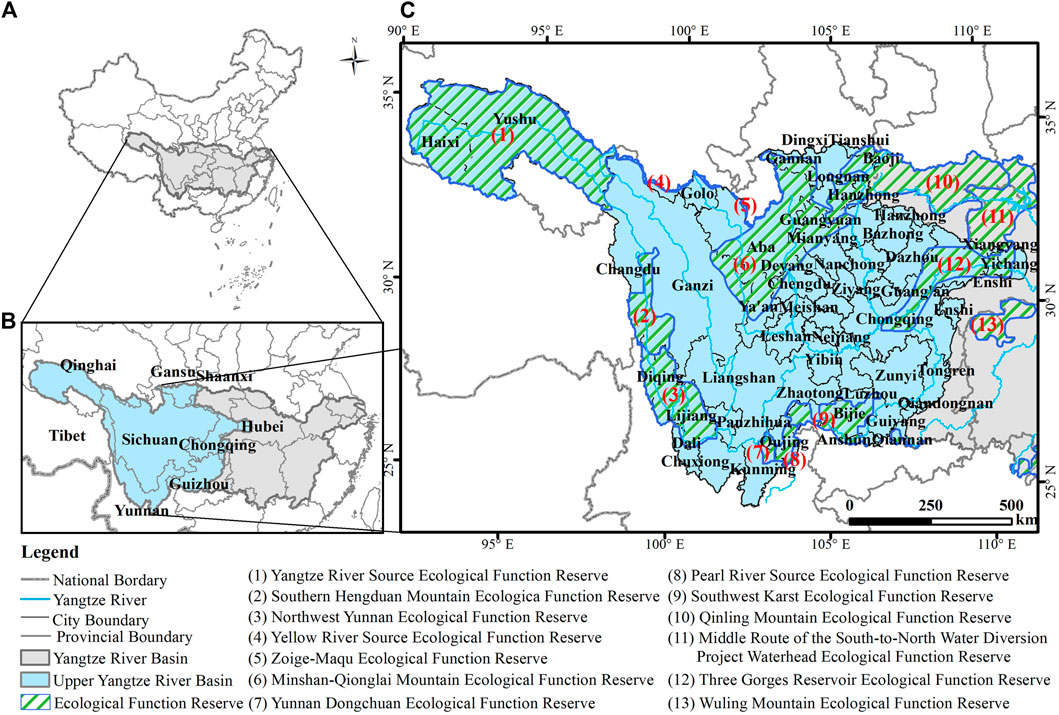
FIGURE 1. Location map of the upper Yangtze River. (A) Geographical location of the Yangtze River Basin; (B) Geographical location of the study area; (C) Water resources and ecological function protection areas in the study area.
The water content was further obtained from the water yield, as shown in Figure 3. The water content per square kilometer of the upper Yangtze River ranged from 0 to 1,359.74 mm in 2015 and from 0 to 1,427.64 mm in 2020, an increase of 67.9 mm. The water content calculated by the InVEST model was the regulated amount of water in the soil layer obtained by combining surface runoff based on the water yield. It indicates that in addition to rainfall and evapotranspiration, the infiltration and water-holding capacity of the soil also determines the amount of water content. The figure shows a spatial distribution of water content gradually increasing from northwest to southeast, with obvious spatial variability. The regions with high water content were distributed in central Sichuan and northern Guizhou and southern Chongqing, which have high water yield, are dominated by hilly and mountainous areas with dense vegetation and have better water holding capacity. The areas with low water content are mainly located in the north and west, where the water yield is low and the main vegetation is grassland, making the soil layer weak in its ability to retain precipitation. The land use/cover type was very important to the precipitation retention and buffering, and thus determines the size of water content.
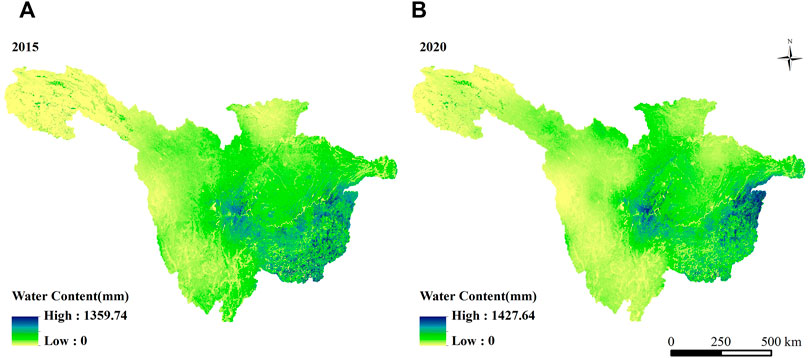
FIGURE 3. The upper Yangtze River water content. (A) water content in 2015; (B) water content in 2020.
3.1.2 The upper Yangtze River grey water footprint
According to the calculation formula of the grey water footprint, the average annual grey water footprint of different sources was obtained. Because the numerical values of different sources were quite different, the method of arc tangent function normalization was used to map the original data to the interval of [0,1], and the formula was x'=ATAN(x)×(2/π); this approach benefits the comparative analysis of grey water footprint structures in different areas, as shown in Figure 4. The comparison between different sources clearly showed that the agricultural grey water footprint accounted for the largest share, followed by the domestic grey water footprint, and the industrial grey water footprint was the smallest. The difference between the agricultural grey water footprint and the other two was large, indicating that nonpoint source pollution caused by agriculture is the main problem affecting water pollution in the Yangtze River basin.
As shown in Figure 5, spatial distribution of the total grey water footprint values in different regions. To better compare the changes in the greywater footprint, the range of values from 180 million to 50.62 billion m3 for 2015 and from 100 million to 37.10 billion m3 for 2020 were combined to unify the intervals, which were classified into five intervals using natural breakpoints. In terms of time change, the grey water footprint value from 2015 to 2020 decreased by 80 million to 13.52 billion m3. And in terms of spatial change, most areas also show a transition from a dark high numerical range to a light low numerical range. This reflects the effectiveness of the upper Yangtze River in following green development and energy conservation and emission reduction during this period. The spatial distribution of the two results was characterized by high values on both sides and low values in the middle, with the lower grey water footprint interval concentrated around the Chengdu Plain, including Suining, Neijiang, Zigong, Leshan, and Ya’an. In the northwest region is less populated and economically underdeveloped, yet all regions have a high grey water footprint, except for Haixi Prefecture. Combined with Figure 4, the industrial and domestic wastewater emissions in Yushu Prefecture, Guoluo Prefecture, Changdu city, Aba Prefecture, and Ganzi Prefecture were low, but the breeding and raising of more livestock and a large amount of livestock excretion among the sources of pollution required more freshwater resources in nature to dilute, and were thus mainly affected by agricultural pollution. As a result, the grey water footprint was relatively high. These areas belong to the source area of the Yangtze River basin, and the protection of water resources is very important in these regions; thus, the prevention and control of agricultural nonpoint source pollution should be strengthened.
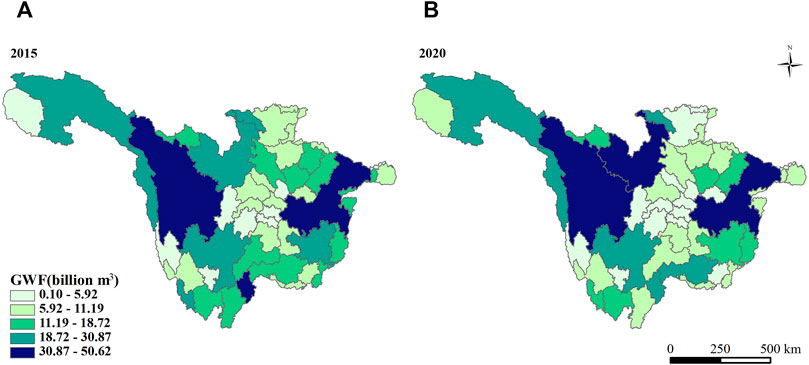
FIGURE 5. Grey water footprint values in the upper Yangtze River. (A) Grey water footprint values of prefecture-level cities in 2015; (B) Grey water footprint values of prefecture-level cities in 2020.
3.2 Upstream of the Yangtze River ecological compensation, protected areas and beneficiary areas were identified
In this study, we considered the relationship between the ratio of water conservation value and the economy and determined the ecological compensation protection cities and beneficiary cities in the upper Yangtze River according to the size of the ECPS (Wang N. J. et al., 2010). The mean ECPS values were calculated to be 0.74 in 2015 and 1.07 in 2020. Areas with ECPS values greater than 0.74 in 2015 were ecological compensation protected areas in the basin, and those below 0.74 were ecological compensation beneficiary areas. The areas with ECPS values greater than 0.74 in 2015 were ecological compensation protected areas, and those with values lower than 0.74 were ecological compensation beneficiary areas. Similarly, those with ECPS values greater than 1.07 in 2020 were protected areas, and those with values less than 1.07 were beneficiary areas. The intervals greater than the mean and those less than the mean was graded using the natural breakpoint method, respectively, and the results were divided into six intervals, as shown in Table 1, and the spatial distribution results are shown in Figure 6. From 2015 to 2020, the number of low beneficiary areas increased, mainly transformed by low protected and medium-benefit areas. In terms of spatial location, most of the beneficiary areas were concentrated in the middle, east and south of the upper reaches of the Yangtze River, and high-benefit areas were mostly concentrated in the Chengdu-Chongqing area. Most of the protected areas were concentrated in the west and northwest of the upstream basin. The classification result was in line with the general socioeconomic development law. The cities in the central-eastern region with better economic development enjoy the water-conserving ecosystem services supplied by the upper reaches and should pay compensation to the western upper reaches to account for some of the economic development benefits sacrificed by the protected areas due to the protection of water resources to ensure the long-term stability of ecosystem services, coordinate and alleviate the development conflicts between regions, and maintain a harmonious win–win situation in the basin.
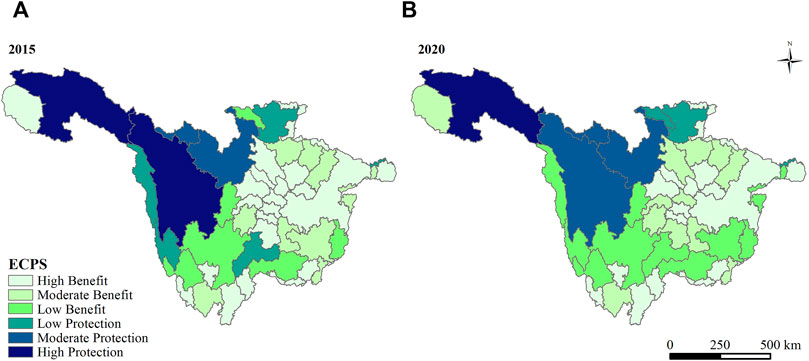
FIGURE 6. The upper Yangtze River eco-compensation priority sequence. (A) ECPS in prefecture-level cities in 2015; (B) ECPS in prefecture-level cities in 2020.
3.3 Results of ecological compensation thresholds in the upper Yangtze River
The threshold model was constructed to obtain the upper and lower threshold limits of ecological compensation for each administrative region in the upper reaches of the Yangtze River in 2015 and 2020 (Figure 7). The lower threshold limits for 2015 and 2020 were based on the grey water footprint and water resources. A value less than 0 indicated that the region had a negative externality, that is, its own freshwater resources were insufficient to solve the pressure of environmental pollution discharge and encroached on the freshwater resources of other regions; as a result, this region must pay ecological compensation to the regions with a water resource surplus. A value greater than 0 indicated a surplus of regional water resources, meaning the region has positive externalities and can supply water to areas with water resource deficits and obtain ecological compensation in return. In terms of spatial distribution, the lower limit of the 2-year ecological compensation threshold tended to be that the compensation benefit areas were clustered in the western region and the compensation payment areas were clustered in the eastern region. Among them, the cities of Yushu, Guoluo and Longnan were transformed from compensation payment areas to compensation benefit areas in 2015; specifically, there were less total water resources in 2015, but the value doubled by 2020, causing the regions to transition from having a water deficit to having a water surplus.
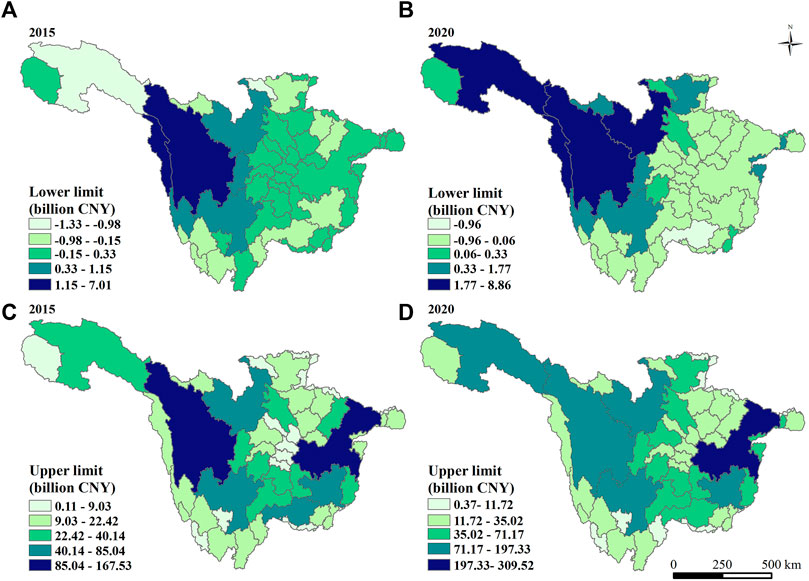
FIGURE 7. Total ecological compensation threshold in the upper Yangtze River. (A) The lower limit of the total threshold of prefecture-level cities in 2015; (B) The lower limit of the total threshold of prefecture-level cities in 2020; (C) The upper limit of the total threshold of prefecture-level cities in 2015; (D) The upper limit of the total threshold of prefecture-level cities in 2020.
The upper limit of the threshold in 2015 and 2020 was obtained based on the water content, which reflects the total value of water conservation ecosystem services in the region. The higher the threshold value is, the stronger the water retention and storage capacity are, and the more important it is to protect the water resources; a lower threshold value indicate the ecological environment needs to be improved to increase the water retention capacity. From 2015 to 2020, the values of the upper threshold levels increased significantly, and the overall water conservation ecosystem service function of the upper Yangtze River improved significantly. Ganzi, Aba, Liangshan, Chongqing, and Zunyi maintained high water conservation ecosystem service values for the 5 years, while the urban clusters around Chengdu and Kunming had low water conservation ecosystem service values that increased. Comparing the upper and lower thresholds in 2015 and 2020, the upper and lower thresholds of Ganzi, Aba, and Liangshan were all in the higher range. This result means that they had high water conservation value, and at the same time, the surplus of water resources was abundant, which enabled these regions to benefit other areas in the basin. The upper threshold and lower thresholds of Chengdu and Kunming were both low and categorized the areas as high beneficiary areas in the basin, which was in line with the eco-compensation priority sequence and the general rule. Among them, Chongqing and Zunyi have good ecological environment quality of water conservation, so the upper threshold was higher. Additionally, due to the high demand for fresh water in economic development, the lower threshold was paid.
The unit compensation threshold of each prefecture-level city in the upper Yangtze River was compared, and since the difference between the upper and lower thresholds was large (note: the upper thresholds were larger than the lower thresholds in each region), the upper and lower thresholds were represented by line graphs, and the maximum and minimum values of each type of threshold were marked, as shown in Figure 8. In 2015, the lower threshold range of the unit area of each prefecture-level city and state in the upper reaches of the Yangtze River was −254.55–637.04 CNY/ha, with an absolute average value of 90.94 CNY/ha, and the upper threshold range was 55.11–27903.05 CNY/ha, with an average value of 10,663.48 CNY/ha. In 2020, each prefecture-level city and state in the upper reaches of the Yangtze River state unit area threshold lower limit ranged from −357.19 to 1,016.45 CNY/ha, the absolute value of the average value was 165.71 CNY/ha, the upper limit ranged from 188.21 to 54,006.75 CNY/ha, and the average value was 17,929.34 CNY/ha. The absolute value of the total compensation threshold in the upper reaches of the Yangtze River ranged from 0.430 to 24.626 billion CNY in 2015 and from 0.671 to 41.602 billion CNY in 2020.
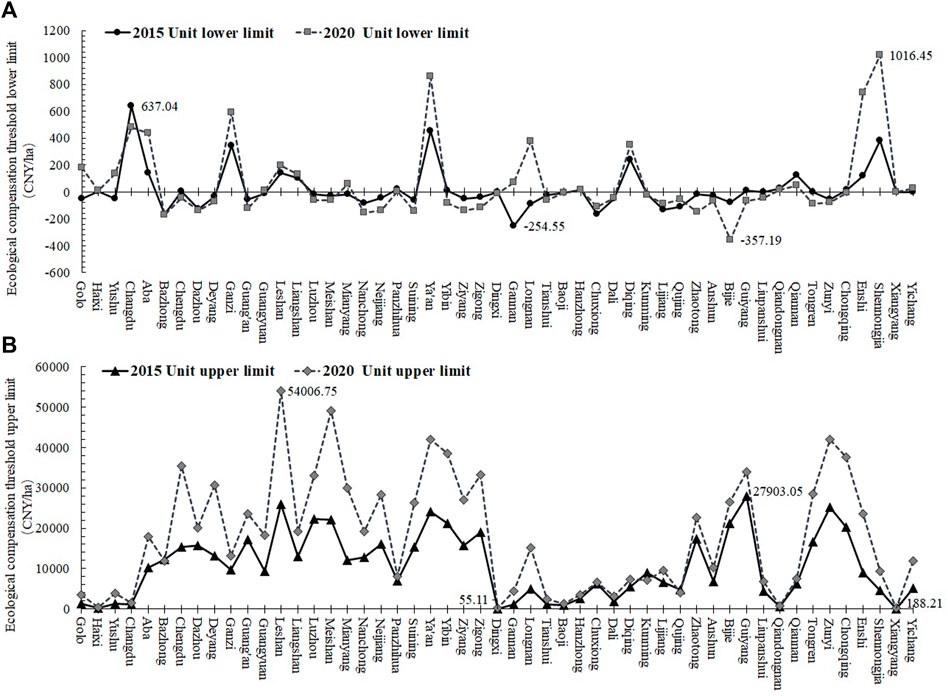
FIGURE 8. Ecological compensation unit threshold in the upper Yangtze River. (A) Lower limit of unit threshold in prefecture-level cities; (B) Upper limit of unit threshold in prefecture-level cities.
Figure 8 shows that the trend of the peak distribution points of the threshold in 2015 and 2020 was generally consistent, with the peak range in 2020 being larger than that in 2015. In the comparison of the threshold lower limit, the number of prefecture-level cities with unit compensation values less than 0 was greater than that of prefecture-level cities with unit compensation values greater than 0; that is, there were more areas paying ecological compensation than receiving ecological compensation.
The highest value of the unit lower limit ecological compensation was in Changdu city in 2015 and in Shennongjia in 2020, while the lowest values of unit lower limit ecological compensation were in Gannan Prefecture and Bijie city. The comparison of the threshold upper limit showed that the overall threshold upper limit of each administrative region in 2020 was significantly higher than that in 2015, where the changes were obvious and the values exceeding 10,000 CNY/ha were in Leshan, Meishan, Chengdu, Deyang, Ya’an, Yibin, Ziyang, Zigong, Zunyi, Chongqing and Enshi, and most of the regions were in the Chengdu city cluster.
4 Discussion
4.1 Water conservation service values in different ecosystems in the upper Yangtze River
Ecosystem services include water conservation, food production, climate regulation, carbon sequestration and oxygen release, biodiversity and other ecosystem service functions (Tian et al., 2019). For watershed ecosystems, the water content service occupies a more important position than other ecosystem services, and the global water scarcity problem causes people to pay close attention to water content services (Liu J. et al., 2019). As the water source area of the Yangtze River, the protection of water conservation in the upper reaches of the Yangtze River is an important guarantee for the safety of water resources in the whole basin and the whole country. In common cases of watershed ecological compensation implementation, water price is often used to assess compensation values, and the value of water conservation services should be close to the actual situation than accounting for all ecosystem service values; thus, inflated compensation standards can be reduced and the process becomes more feasible. Discussing the service value of water conservation in different ecosystems can clarify the relationship between them, and provide reference for the improvement of ecological environment and land space planning.
Referring to the classification method of the China Ecosystem Assessment and Ecosystem Security Database1 and the Resource and Environmental Science and Data Center of the Chinese Academy of Sciences2, the upper reaches of the Yangtze River can be divided into eight ecosystem types: forest ecosystem, shrub ecosystem, grassland ecosystem, desert ecosystem, wetland ecosystem, farmland ecosystem, urban ecosystem and other ecosystems. And count the changes of the area ratio and water conservation service value of each ecosystem, as shown in Figure 9. The results showed that urban ecosystems and forest ecosystems in the upper Yangtze River increased from 2015 to 2020, while the rest of the ecosystems decreased to different degrees, with the scrub ecosystem area decreasing the most. The ecosystem type of the upper Yangtze River is mainly grassland, followed by forest and farmland, with the sum of the three exceeding 80% of the total area of the study area. And influenced by the increase of precipitation, the value of water connotation services per unit in 2020 increased compared to 2015. From the perspective of environmental improvement, just considering the water conservation services value per unit area of only four types of ecosystems, namely wetland, forest, grassland and scrub, the ranking from high to low as scrub > forest > grassland > wetland. Corresponding to the previous results (Figure 2, Figure 3), the water yield and water conservation are increasing from northwest to southeast. The grassland, desert and wetland ecosystems mainly distributed in the northwest area of the upper reaches of the Yangtze River have low water conservation service value per unit, while the southeast area, which is dominated by forest and shrub ecosystems, has dense vegetation, which is not conducive to the formation of surface runoff, and developed roots are conducive to soil and water conservation, so the water conservation service value is high.
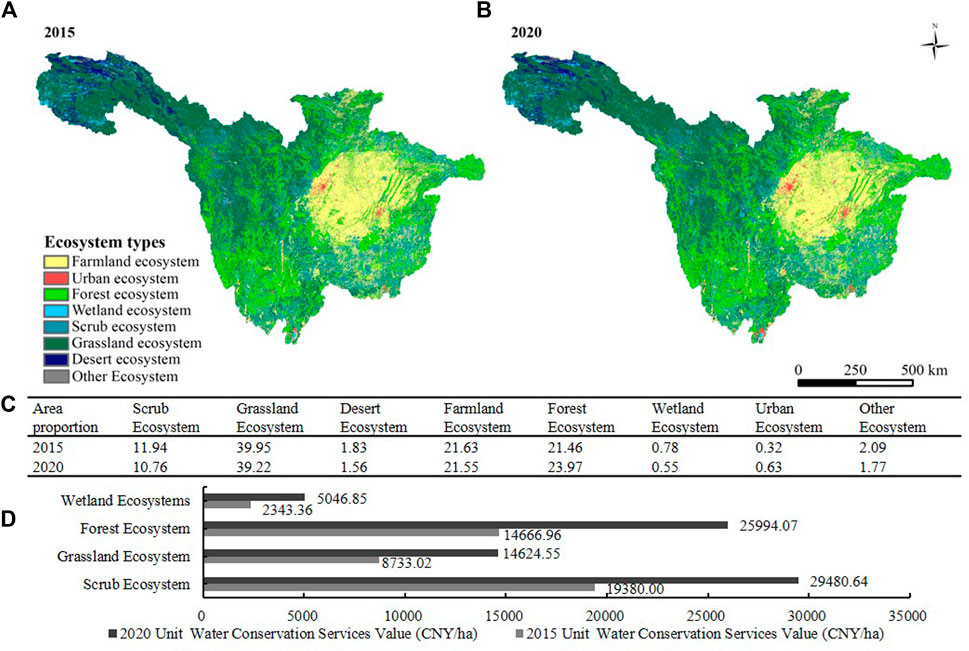
FIGURE 9. Distribution of different ecosystems in the upper Yangtze River and water conservation service value. (A) different ecosystems in 2015; (B) different ecosystems in 2020; (C) Area proportion of different ecosystems; (D) water conservation service value of different ecosystems.
4.2 Comparative analysis of grey water footprint and opportunity cost methods
In determining ecological compensation criteria, many studies have used the opportunity cost approach to determine the economic benefits lost by farmers to provide ecosystem services and used this value as a lower bound for ecological compensation. Economic losses result from the restriction of industrial development in ecological reserves to implement ecological compensation policies, and they include the opportunity costs of participating in reforestation, reforestation, ecological public welfare forests and water source protection. The study assumed that after participating in the ecological compensation policy, farmers would not be able to obtain economic benefits in the short term, so the benefits provided by the land-use type before participating in ecological compensation could be characterized as the opportunity cost of participating in the ecological compensation policy, and again, the results of the opportunity cost were multiplied by the results of the opportunity cost and were multiplied by the demand intensity coefficient ti of ecological compensation. The grey water footprint was analyzed in comparison with the results of the opportunity cost approach, as shown in Figure 10.
In Figure 10, the opportunity cost compensation value and the grey water footprint compensation value corresponded to different axes. The range of unit opportunity cost compensation values for 2015–2020 for each region is 110.39–808510.25 CNY/ha. Since the unit grey water footprint compensation values were calculated only for 2015 and 2020, the average value was multiplied by the number of years to obtain a range of ecological compensation values per unit grey water footprint for 2015–2020 of -1,297.50-4,188.46 CNY/ha. The ecological compensation value of unit opportunity cost to each prefecture-level city (state) is greater than that of grey water footprint, which indicates that the grey water footprint approach adopted in this study was closer to the actual feasible compensation value. The figure reflects that the compensation value curves generated by the two methods fluctuated with similar trends among the prefecture-level cities, such as Ganzi, Ya’an, Longnan, and Diqing, which showed significant peaks in both compensation methods. Most of the regions with negative grey water footprints also corresponded to relatively low opportunity cost values, such as in Xiangyang, Bijie, Chuxiong, Suining, Nanchong, Guang’an, and Bazhong city. These areas with a small loss of opportunity cost have a great demand for economic development, thus generating a greater grey water footprint, resulting in water deficits and the need to pay ecological compensation to balance development within the basin. Both approaches have their advantages, with the opportunity cost being able to compensate as much as possible for the economic loss of farmers who must protect ecological land, and the economic development aspirations of the region being considered more than the grey water footprint. The grey water footprint is more reflective of the “polluter pays” principle, which considers the cost of pollution control more than the opportunity cost. The opportunity cost mainly considers the change of land use type, but less considers the ecological value of water resources in the watershed. The grey water footprint better reflects the degree of pollution caused by different industries. For example, non-point source pollution caused by agriculture has a greater impact on the water environment, taking the stakeholder relationship into account more comprehensively. In addition, the grey water footprint introduces a zero-sum model to calculate ecological compensation, which makes the results have positive and negative values. It can represent the compensation subject-object relationship in the basin, which can reflect more information than the opportunity cost method of calculating ecological compensation.
4.3 Comparative analysis of theoretical ecological compensation thresholds in the upper reaches of the Yangtze River and ecological compensation in different river basins
In global watershed ecological compensation, many countries are early to address the issue of inter-basin benefit distribution and ecological compensation. There have been more cases of successful improvement of the watershed ecological environment by means of ecological compensation (Table 2). These experiences can be used as a reference for establishing ecological compensation in watersheds in China. Table 2 shows that the compensation effects of various watershed ecological compensation cases are mainly centered on water quality improvement, which is the main purpose of watershed ecological compensation. And due to the nature of rivers, the relationship between ecological compensation recipients and payers in a watershed is often upstream and downstream. Comparing the existing watershed ecological compensation standards with the theoretical threshold in this study, the overall theoretical value of the upper threshold was higher, while the theoretical value of the lower threshold was close to the actual watershed compensation amount because the upper threshold represented the value of water conservation services in the region. In practice, the ecological value of the area is not used as the evaluation criterion, but the compensation is based on the degree of loss. The actual compensation standard is often not a unique price but rather a range of compensation values based on a combination of multiple instruments, as different watersheds have different social environments and varying degrees of pollution.
In recent years, the practice of ecological compensation in China’s watersheds has developed rapidly, and funding has increased significantly, but the effects of compensation are not yet obvious. The domestic compensation cases in Table 2 also show that the compensation funds are more dependent on government finance, and the compensation funds come from a single source. The survey shows that the proportion of funds invested from social parties has not yet reached 1% of the total ecological protection compensation funds in China (Wu et al., 2019). Although local governments at all levels have been actively experimenting with market-based and diversified ecological compensation mechanisms in recent years. For example, The Xin’an River (Ren et al., 2021) and Jinhua River (Zhang, 2011) are the first examples of cross-provincial watershed ecological compensation and water rights trading in China, respectively, and both have achieved good results. However, to further promote it, it is necessary to provide corresponding legal system protection and policy support, standardize the methods of market compensation and compensation fund accounting, and promote the transition of watershed ecological compensation work from basic research to technical guidance and policy (Feng et al., 2018). International watershed ecological compensation has been developed earlier than in China, and it is easy to see that governments have a major role in funding ecological compensation for larger projects. Examples include the treatment of eutrophication in Lake Biwa waters in Japan, the New York City plan in the United States of America and the construction of sewage treatment plants in the Elbe River basin in Germany and the Czech Republic (Smith and Porter, 2010; Sauer et al., 2015; Yu 2016). This type of ecological compensation for larger watersheds requires the coercive power of government to coordinate between people, sectors and enterprises. When ecological compensation is initiated by the market, it is mostly motivated by a conflict between economic interests and environmental protection, as in the case of mineral water companies in France, NGOs in Costa Rica, local funds in Ecuador (Jiang and Chen, 2016). A common feature of these types of compensation is that they occur in small watersheds, where the amount of compensation is small, and where government subsidies make it possible to fund the compensation more adequately. Combined with the international experience of watershed ecological compensation, the upper Yangtze River basin involves several administrative regions, and the implementation of watershed ecological compensation should establish a horizontal compensation mechanism, which makes local governments compensate each other. The compensation can be combined with market mechanisms, NGOs can be encouraged to jointly initiate compensation projects, and the sources of compensation can be broadened to ensure sufficient funds. In addition, we should improve the ecological compensation system and form a legal basis to promote the participation of various stakeholders to promote the harmonious coexistence between humans and nature and to ensure the sustainable development of water resources in the upper reaches of the Yangtze River.
5 Conclusion
The water yield per unit in the upper reaches of the Yangtze River was 0–1,667.13 mm and 0–1840.22 mm in 2015 and 2020, respectively, with the range interval increasing by 173.09 mm. The water content per unit area was 0–1,359.74 mm and 0–1,427.64 mm, with the range interval increasing by 67.9 mm from 2015 to 2020. The source structure of the grey water footprint indicates that the agricultural grey water footprint accounts for the largest proportion, followed by the domestic grey water footprint, and the smallest is the industrial grey water footprint. Nonpoint source pollution is the main problem facing water pollution in the Yangtze River basin. The highest value of the grey water footprint from 2015 to 2020 was reduced by 13.52 billion m3, the total amount of the grey water footprint was reduced, and environmental protection initiatives became more effective.
The eco-compensation priority sequence was divided into ecological compensation protected areas and ecological compensation beneficiary areas according to the relationship with the mean. Most of the beneficiary areas were concentrated in the central-eastern and southern parts of the upper reaches of the Yangtze River, especially in the Chengdu-Chongqing urban agglomeration. Most of the protected areas were concentrated in the western and northwestern parts of the upper watershed. The classification results conformed to the general economic development law. Finally, the lower limit of the threshold for each prefecture-level city and state in 2015 ranged from −254.55 to 637.04 CNY/ha, and the upper limit ranged from 55.11 to 27,903.05 CNY/ha. The lower limit of the threshold for each prefecture-level city and state in the upper Yangtze River in 2020 ranged from −357.19 to 1,016.45 CNY/ha, and the upper range was 188.21–54,006.75 CNY/ha. The absolute mean range of the total compensation threshold was 0.43–24.63 billion CNY in 2015 and 0.67 to 41.60 billion CNY in 2020. From 2015 to 2020, the ecological compensation threshold of the Yangtze River basin improved overall.
The value of water conservation services per unit area in the different ecosystems in descending order was scrub > forest > grassland > wetland. The grey water footprint and opportunity cost compensation value curves fluctuated similarly at the prefecture level, but the compensation value of opportunity cost was almost always much larger than the compensation value of the grey water footprint. Compared with the actual ecological compensation standards adopted in different river basins, the overall theoretical threshold lower limit was closer to the actual river basin compensation amount than was the upper limit, indicating that the compensation accounting based on the ecological damage degree was more in line with the actual situation.
In summary, the threshold model of ecological compensation in the upper reaches of the Yangtze River was constructed by using the InVEST model and greywater footprint. The results proved that this method has certain applicability and reference value. There are also some shortcomings. First, this study calculates the non-market value and market value of watershed ecological compensation as upper and lower limits separately, but the actual ecological compensation is often a combination of market value and non-market value. Second, the ecological compensation of the grey water footprint regards the upper Yangtze River as a complete basin, lacking consideration of the impact on the middle and lower reaches of the basin. Thirdly, due to the limited data acquisition, the lack of the supplement of actual measurement data and the survey of people’s willingness to compensate, resulting in the gap between the research results and the reality. In future studies, more influence factors can be added to optimize the parameter selection of the ecological compensation threshold model. And considering the correlation of the whole basin, we can try to calculate the ecological compensation threshold for the whole Yangtze River and discuss the benefit relationship between upstream and downstream. Supplementing the fieldwork data and questionnaires in the study area makes the parameters more localized and enhances the feasibility of the results.
Data availability statement
The original contributions presented in the study are included in the article/supplementary material, further inquiries can be directed to the corresponding author.
Author contributions
DG: Conceptualization; Methodology; Funding acquisition; Investigation; Project administration; Supervision; Validation; Writing--original draft, review, and editing. LW: Conceptualization; Methodology; Data curation; Investigation; Formal analysis; Software; Validation; Visualization; Writing--original draft, review, and editing. LC: Conceptualization; Methodology; Investigation; Formal analysis; Writing--review and editing. YZ: Data curation; Investigation; Writing--review and editing. LZ: Data curation; Project administration; Supervision; Validation; Writing--review and editing.
Funding
This work is partially supported by National Natural Science Foundation of China (42171298), Late Project of National Social Science Foundation in China (20FJYB035), the Ministry of education of Humanities and Social Science project (20YJA790016) and Natural Science Foundation of Chongqing in China (cstc2020jcyj-jqX0004).
Conflict of interest
The authors declare that the research was conducted in the absence of any commercial or financial relationships that could be construed as a potential conflict of interest.
Publisher’s note
All claims expressed in this article are solely those of the authors and do not necessarily represent those of their affiliated organizations, or those of the publisher, the editors and the reviewers. Any product that may be evaluated in this article, or claim that may be made by its manufacturer, is not guaranteed or endorsed by the publisher.
Footnotes
1The Chinese terrestrial ecosystem classification system in the China Ecosystem Assessment and Ecosystem Security Database (https://www.ecosystem.csdb.cn/ecosys/eco_classes.jsp).
2The 2015 data on the spatial distribution of terrestrial ecosystem types in China from the Resource and Environmental Science and Data Center of the Chinese Academy of Sciences (https://www.resdc.cn/data.aspx?DATAID=198).
References
Anley, M. A., Minale, A. S., Haregewoyn, N., and Gashaw, T. (2022). Assessing the impacts of land use/cover changes on ecosystem service values in Rib watershed, Upper Blue Nile Basin, Ethiopia. Trees For. People 7, 100212. doi:10.1016/j.tfp.2022.100212
Aziz, T. (2020). Changes in land use and ecosystem services values in Pakistan, 1950-2050. Environ. Dev. 37, 100576. doi:10.1016/j.envdev.2020.100576
Bagstad, K. J., Johnson, G. W., Voigt, B., and Villa, F. (2013). Spatial dynamics of ecosystem service flows: A comprehensive approach to quantifying actual services. Ecosyst. Serv. 4, 117–125. doi:10.1016/j.ecoser.2012.07.012
Bai, T. J., and Sun, C. Z. (2018). Regional inequality and factor decomposition of the per capita grey water footprint in China. Acta Ecol. Sin. 38 (17), 6314–6325. doi:10.5846/stxb201709291764
Bekturganov, Z., Tussupova, K., Berndtsson, R., Sharapatova, N., Aryngazin, K., and Zhanasova, M. (2016). Water related health problems in central asia—a review. Water 8 (6), 219. doi:10.3390/w8060219
Benra, F., De Frutos, A., Gaglio, M., Alvarez-Garreton, C., Felipe-Lucia, M., and Bonn, A. (2021). Mapping water ecosystem services: Evaluating InVEST model predictions in data scarce regions. Environ. Model. Softw. 138, 104982. doi:10.1016/j.envsoft.2021.104982
Best, J. (2019). Anthropogenic stresses on the world’s big rivers. Nat. Geosci. 12 (1), 7–21. doi:10.1038/s41561-018-0262-x
Brown, M. A., Clarkson, B. D., Barton, B. J., and Joshi, C. (2013). Ecological compensation: An evaluation of regulatory compliance in New Zealand. Impact Assess. Proj. Apprais. 31 (1), 34–44. doi:10.1080/14615517.2012.762168
Burdon, D., Potts, T., Barnard, S., Boyes, S. J., and Lannin, A. (2022). Linking natural capital, benefits and beneficiaries: The role of participatory mapping and logic chains for community engagement. Environ. Sci. Policy 134, 85–99. doi:10.1016/j.envsci.2022.04.003
Chai, M., and Chen, Y. R. (2022). Spatio-temporal variations and driving factors of greywater footprint in the Y angtze River economic Belt, China. Pol. J. Environ. Stud. 31 (2), 1577–1586. doi:10.15244/pjoes/143247
Chapagain, A. K., and Hoekstra, A. Y. (2011). The blue, green and grey water footprint of rice from production and consumption perspectives. Ecol. Econ. 70 (4), 749–758. doi:10.1016/j.ecolecon.2010.11.012
Chen, X., and Qian, W. W. (2020). Effect of marine environmental regulation on the industrial structure adjustment of manufacturing industry: An empirical analysis of China’s eleven coastal provinces. Mar. Policy 113, 103797. doi:10.1016/j.marpol.2019.103797
Cong, W. C., Sun, X. Y., Guo, H., and Shan, R. F. (2020). Comparison of the SWAT and InVEST models to determine hydrological ecosystem service spatial patterns, priorities and trade-offs in a complex basin. Ecol. Indic. 112, 106089. doi:10.1016/j.ecolind.2020.106089
De Girolamo, A. M., Miscioscia, P., Politi, T., and Barca, E. (2019). Improving grey water footprint assessment: Accounting for uncertainty. Ecol. Indic. 102, 822–833. doi:10.1016/j.ecolind.2019.03.040
De Mello, K., Fendrich, A. N., Borges-Matos, C., Brites, A. D., Tavares, P. A., da Rocha, G. C., et al. (2021). Integrating ecological equivalence for native vegetation compensation: A methodological approach. Land Use Policy 108, 105568. doi:10.1016/j.landusepol.2021.105568
E, G. X., Zhao, Y. J., Chen, L. P., Ma, Y. H., Chu, M. X., Li, X. L., et al. (2018). Genetic diversity of the Chinese goat in the littoral zone of the Yangtze River as assessed by microsatellite and mtDNA. Ecol. Evol. 8 (10), 5111–5123. doi:10.1002/ece3.4100
Engel, S., Pagiola, S., and Wunder, S. (2008). Designing payments for environmental services in theory and practice: An overview of the issues. Ecol. Econ. 65 (4), 663–674. doi:10.1016/j.ecolecon.2008.03.011
Farley, J., and Costanza, R. (2010). Payments for ecosystem services: From local to global. Ecol. Econ. 69 (11), 2060–2068. doi:10.1016/j.ecolecon.2010.06.010
Feng, D. Y., Wu, W. L., Liang, L., Li, Li., and Zhao, G. S. (2018). Payments for watershed ecosystem services: Mechanism, progress and challenges. Ecosyst. Health Sustain. 4 (1), 13–28. doi:10.1080/20964129.2018.1434318
Florke, M., Schneider, C., and McDonald, R. I. (2018). Water competition between cities and agriculture driven by climate change and urban growth. Nat. Sustain. 1 (1), 51–58. doi:10.1038/s41893-017-0006-8
Fu, Y. C., Du, X., Ruan, B. Q., Liu, L. S., and Zhang, J. (2017). Agro-ecological compensation of watershed based on emergy. Water Sci. Technol. 76 (10), 2830–2841. doi:10.2166/wst.2017.455
Gao, X. L., Huang, B. B., Hou, Y., Xu, W. H., Zheng, H., Ma, D. C., et al. (2020). Using ecosystem service flows to inform ecological compensation: Theory & application. Int. J. Environ. Res. Public Health 17 (9), 3340. doi:10.3390/ijerph17093340
Gao, X., Shen, J. Q., He, W. J., Sun, F. H., Zhang, Z. F., Guo, W. J., et al. (2019). An evolutionary game analysis of governments’ decision-making behaviors and factors influencing watershed ecological compensation in China. J. Environ. Manag. 251, 109592. doi:10.1016/j.jenvman.2019.109592
Garau, E., Pueyo-Ros, J., Palom, A. R., and Vila-Subiros, J. (2021). Follow the flow: Analysis of relationships between water ecosystem service supply units and beneficiaries. Appl. Geogr. 133, 102491. doi:10.1016/j.apgeog.2021.102491
Garrick, D., De Stefano, L., Yu, W., Jorgensen, I., O'Donnell, E., TurleyL Aguilar-Barajas, I., et al. (2019). Rural water for thirsty cities: A systematic review of water reallocation from rural to urban regions. Environ. Res. Lett. 14 (4), 043003. doi:10.1088/1748-9326/ab0db7
Gastineau, P., Mossay, P., and Taugourdeau, E. (2021). Ecological compensation: How much and where? Ecol. Econ. 190, 107191. doi:10.1016/j.ecolecon.2021.107191
Greve, P., Kahil, T., Mochizuki, J., Schinko, T., Satoh, Y., Burek, P., et al. (2018). Global assessment of water challenges under uncertainty in water scarcity projections. Nat. Sustain. 1 (9), 486–494. doi:10.1038/s41893-018-0134-9
Grill, G., Lehner, B., Thieme, M., Geenen, B., Tickner, D., Antonelli, F., et al. (2019). Mapping the world’s free-flowing rivers. Nature 569 (7755), 215–221. doi:10.1038/s41586-019-1111-9
Gu, Y. Q. (2017). Research on ecological compensation in island tourism destination based on pes-the zhoushan archipelago new area as an example. J. Environ. Prot. Ecol. 18 (4), 1765–1771.
Guan, X. J., Liu, M., and Meng, Y. (2021). A comprehensive ecological compensation indicator based on pollution damage – protection bidirectional model for river basin. Ecol. Indic. 126, 107708. doi:10.1016/j.ecolind.2021.107708
Guan, X. J., Liu, W. K., and Wang, H. L. (2018). Study on the ecological compensation standard for river basin based on a coupling model of TPC-WRV. Water Sci. Technol. Water Supply 18 (4), 1196–1205. doi:10.2166/ws.2017.183
Hao, C. L., Yan, D. H., Gedefaw, M., Qin, T. L., Wang, H., and Yu, Z. L. (2021). Accounting of transboundary ecocompensation standards based on water quantity allocation and water quality control targets. Water Resour. manage. 35 (6), 1731–1756. doi:10.1007/s11269-021-02807-5
He, C. Y., Wu, J. G., Pan, X. H., Fang, Z. H., Li, J. W., Bryan, B. A., et al. (2021). Future global urban water scarcity and potential solutions. Nat. Commun. 12, 4667. doi:10.1038/s41467-021-25026-3
Hoekstra, A. Y., and Chapagain, A. K. (2007). Water footprints of nations: Water use by people as a function of their consumption pattern. Water Resour. manage. 21 (1), 35–48. doi:10.1007/s11269-006-9039-x
Hong, B. T., Ren, P., Yuan, Q. Z., and Wang, L. (2019). Ecological function regionalization in the upper Yangtze River. J. Ecol. Rural Environ. 35 (08), 1009–1019. doi:10.19741/j.issn.1673-4831.2018.0459
Hou, L. L., Xia, F., Chen, Q. H., Huang, J. K., He, Y., Rose, N., et al. (2021). Grassland ecological compensation policy in China improves grassland quality and increases herders' income. Nat. Commun. 12 (1), 4683. doi:10.1038/s41467-021-24942-8
Hu, W. J., Wu, X. Q., and Zhang, K. B. (2022). Spatiotemporal change of beneficiary area from wind erosion prevention service in the Ulan Buh Desert in 2008 and 2018. Geogr. Sustain. 3 (2), 119–128. doi:10.1016/j.geosus.2022.04.002
Jiang, K., Merrill, R., You, D. M., Pan, P., and Li, Z. D. (2019a). Optimal control for transboundary pollution under ecological compensation: A stochastic differential game approach. J. Clean. Prod. 241, 118391. doi:10.1016/j.jclepro.2019.118391
Jiang, K., You, D. M., Li, Z. D., and Shi, S. S. (2019b). A differential game approach to dynamic optimal control strategies for watershed pollution across regional boundaries under eco-compensation criterion. Ecol. Indic. 105, 229–241. doi:10.1016/j.ecolind.2019.05.065
Jiang, Y. N., Guan, D. J., He, X. J., Yin, B. L., Zhou, L. L., Sun, L. L., et al. (2022). Quantification of the coupling relationship between ecological compensation and ecosystem services in the Yangtze River Economic Belt, China. Land Use Policy 114, 105995. doi:10.1016/j.landusepol.2022.105995
Jiang, Y. Q., and Chen, K. (2016). A review of researches on payment for watershed ecosystem services. Ecol. Econ. 32 (04), 175–180.
Kinzig, A. P., Perrings, C., Chapin, F. S., Polasky, S., Smith, V. K., Tilman, D., et al. (2011). Paying for ecosystem services-promise and peril. Science 334 (6056), 603–604. doi:10.1126/science.1210297
Liu, H. G., Chen, M., and Tang, Z. P. (2019). Study on ecological compensation standards of water resources based on grey water footprint: A case of the Yangtze River economic Belt. Resour. Environ. Yangtze Basin 28 (11), 2553–2563. doi:10.11870/cjlyzyyhj201911002
Liu, J., Fu, B., Zhang, C. H., Hu, Z. P., and Wang, Y. K. (2019). Assessment of ecosystem water retention and its value in the upper reaches of Minjiang River based on InVEST model. Resour. Environ. Yangtze Basin 28 (03), 577–585. doi:10.11870/cjlyzyyhj201903008
Liu, J. G., Liu, Q. Y., and Yang, H. (2016). Assessing water scarcity by simultaneously considering environmental flow requirements, water quantity, and water quality. Ecol. Indic. 60, 434–441. doi:10.1016/j.ecolind.2015.07.019
Liu, Y. W., and Yuan, L. (2022). Evolution of water-use efficiency in the Yangtze River Economic Belt based on national strategies and water environment treatment. Ecol. Inf. 69, 101642. doi:10.1016/j.ecoinf.2022.101642
Ma, X. F., Zhu, J. T., Zhang, H. B., Yan, W., and Zhao, C. Y. (2020). Trade-offs and synergies in ecosystem service values of inland lake wetlands in central Asia under land use/cover change: A case study on ebinur lake, China. Glob. Ecol. Conservation 24, e01253. doi:10.1016/j.gecco.2020.e01253
McMahon, J. M., Hasan, S., Brooks, A., Curwen, G., Dyke, J., Saint Ange, C., et al. (2022). Challenges in modelling the sediment retention ecosystem service to inform an ecosystem account-Examples from the Mitchell catchment in northern Australia. J. Environ. Manag. 314, 115102. doi:10.1016/j.jenvman.2022.115102
Pacetti, T., Castelli, G., Schröder, B., Bresci, E., and Caporali, E. (2021). Water ecosystem services footprint of agricultural production in central Italy. Sci. Total Environ. 797, 149095. doi:10.1016/j.scitotenv.2021.149095
Pang, A. P., Li, C. H., and Yi, Y. J. (2021). Spatial-temporal patterns and driving forces of nitrogen flows for agricultural plantation-consumption system in Yangtze River Economic Belt. J. Agro-Environment Sci. 40 (02), 408–420. doi:10.11654/jaes.2020-1130
Qiao, X., Schmidt, A. H., Xu, Y., Zhang, H. M., Chen, X. L., Xiang, R. T., et al. (2021). Surface water quality in the upstream-most megacity of the Yangtze River basin (Chengdu): 2000-2019 trends, the COVID-19 lockdown effects, and water governance implications. Environ. Sustain. Indic. 10, 100118. doi:10.1016/j.indic.2021.100118
Qin, Y. B., and Wang, W. P. (2022). Research on ecological compensation mechanism for energy economy sustainable based on evolutionary game model. Energies 15 (8), 2895. doi:10.3390/en15082895
Qiu, L., and Zhai, H. J. (2014). An ecological compensation mechanism of Chishui River water resources protection and research. Appl. Mech. Mater. 685, 463–467. doi:10.4028/www.scientific.net/amm.685.463
Rao, H. H., Lin, C. C., Kong, H., Jin, D., and Peng, B. R. (2014). Ecological damage compensation for coastal sea area uses. Ecol. Indic. 38, 149–158. doi:10.1016/j.ecolind.2013.11.001
Ren, Y. S., Lu, L., Yu, H., and Zhu, D. C. (2021). Game strategies in government-led eco-compensation in the Xin’an River Basin from the perspective of the politics of scale. J. Geogr. Sci. 31, 1205–1221. doi:10.1007/s11442-021-1893-1
Rong, N., Shan, B. Q., Lin, C., Guo, Y., Zhao, Y., and Zhu, X. L. (2016). Evolution of the nitrogen pollution in the hai river basin. Acta Sci. Circumstantiae 36 (02), 420–427. doi:10.13671/j.hjkxxb.2015.0414
Sauer, P., Fiala, P., Dvorak, A., and Kolinsky, O. (2015). Coalition projects in wastewater treatment: the case of a drinking water reservoir in the Czech Republic. J. Environ. Protect. Ecol. 16 (4), 1492–1501.
Schilling, J., Hertig, E., Tramblay, Y., and Scheffran, J. (2020). Climate change vulnerability, water resources and social implications in North Africa. Reg. Environ. Change 20 (1), 15. doi:10.1007/s10113-020-01597-7
Schirpke, U., Scolozzi, R., De Marco, C., and Tappeiner, U. (2014). Mapping beneficiaries of ecosystem services flows from Natura 2000 sites. Ecosyst. Serv. 9, 170–179. doi:10.1016/j.ecoser.2014.06.003
Smith, L. E. D., and Porter, K. S. (2010). Management of catchments for the protection of water resources: drawing on the New York City watershed experience. Reg. Environ. Change 10, 311–326. doi:10.1007/s10113-009-0102-z
Sheng, J. C., and Han, X. (2022). Practicing policy mobility of payment for ecosystem services through assemblage and performativity: Lessons from China's Xin'an River Basin Eco-compensation Pilot. Ecol. Econ. 191, 107234. doi:10.1016/j.ecolecon.2021.107234
Simmonds, J. S., Sonter, L. J., Watson, J. E. M., Bennun, L., Costa, H. M., Dutson, G., et al. (2020). Moving from biodiversity offsets to a target-based approach for ecological compensation. Conserv. Lett. 13 (2), 12695. doi:10.1111/conl.12695
Sterling, E. J., Betley, E., Sigouin, A., Gomez, A., Toomey, A., Cullman, G., et al. (2017). Assessing the evidence for stakeholder engagement in biodiversity conservation. Biol. Conserv. 209, 159–171. doi:10.1016/j.biocon.2017.02.008\
Tang, W. Z., Pei, Y. S., Zheng, H., Zhao, Y., Shu, L. M., and Zhang, H. (2022). Twenty years of China's water pollution control: Experiences and challenges. Chemosphere 295, 133875. doi:10.1016/j.chemosphere.2022.133875
Tian, Y. C., Bai, X. Y., Huang, Y. L., Zhang, Q., Tao, J., and Zhang, Y. L. (2019). Ecological compensation standard accounting of Chishui River basin based on ecosystem service value. Trans. Chin. Soc. Agric. Mach. 50 (11), 312–322. doi:10.6041/j.issn.1000-1298.2019.11.035
Villarreal-Rosas, J., Wells, J. A., Sonter, L. J., Possingham, H. P., and Rhodes, J. R. (2022). The impacts of land use change on flood protection services among multiple beneficiaries. Sci. Total Environ. 806 (2), 150577. doi:10.1016/j.scitotenv.2021.150577
Wan, L., Zheng, Q. Q., Wu, J., Wei, Z. Y., and Wang, S. Y. (2022). How does the ecological compensation mechanism adjust the industrial structure? Evidence from China. J. Environ. Manag. 301, 113839. doi:10.1016/j.jenvman.2021.113839
Wang, H., Tao, R., Wang, L. L., and Su, F. B. (2010). Farmland preservation and land development rights trading in Zhejiang, China. Habitat Int. 34 (4), 454–463. doi:10.1016/j.habitatint.2009.12.004
Wang, N. J., Liu, J., Wu, D. Q., Gao, S., and Wang, R. Q. (2010). Regional eco-compensation based on ecosystem service assessment: A case study of Shandong province. Acta Ecol. Sin. 30 (23), 6646–6653.
Wang, S. H., He, Y. Q., and Song, M. L. (2021). Global value chains, technological progress, and environmental pollution: Inequality towards developing countries. J. Environ. Manag. 277, 110999. doi:10.1016/j.jenvman.2020.110999
Wang, W., Wu, F. P., and Yu, H. (2022). Optimal design of the ecological compensation mechanism in transboundary river basins under the Belt and Road Initiative. Sustain. Prod. Consum. 32, 173–183. doi:10.1016/j.spc.2022.04.018
Wen, W. J., Xu, G., and Wang, X. J. (2011). Spatial transferring of ecosystem services and property rights allocation of ecological compensation. Front. Earth Sci. 5 (3), 280–287. doi:10.1007/s11707-011-0186-x
Wu, C. Y., Chen, B. W., Huang, X. J., and Dennis Wei, Y. H. (2020). Effect of land-use change and optimization on the ecosystem service values of Jiangsu province, China. Ecol. Indic. 117, 106507. doi:10.1016/j.ecolind.2020.106507
Wu, L., Kong, D. S., and Jin, L. S. (2019). Research on the progress of the eco-compensation mechanism in China. Acta Ecol. Sin. 39 (01), 1–8. doi:10.5846/stxb201808281842
Xi, B., and Jing, H. M. (2021). Research on horizontal compensation of ecological economic benefits under differential responsibility. Environ. Sci. Pollut. Res. Int. 28 (23), 29875–29889. doi:10.1007/s11356-021-12835-8
Xu, C. X., Liu, Y., and Fu, T. B. (2022). Spatial-temporal evolution and driving factors of grey water footprint efficiency in the Yangtze River Economic Belt. Sci. Total Environ. 844, 156930. doi:10.1016/j.scitotenv.2022.156930
Xu, Y. X., Sun, H. L., Ma, L. K., and Yang, J. J. (2021). Promoting the protection and restoration of the ecological environment of the Yangtze River based on the "Yangtze River protection law. Environ. Prot. 49 (19), 56–59. doi:10.14026/j.cnki.0253-9705.2021.11
Yang, X. Y., Meng, F., Fu, P. J., Zhang, Y. X., and Liu, Y. H. (2021). Spatiotemporal change and driving factors of the eco-environment quality in the Yangtze River basin from 2001 to 2019. Ecol. Indic. 131, 108214. doi:10.1016/j.ecolind.2021.108214
Yu, B., and Xu, L. Y. (2016). Review of ecological compensation in hydropower development. Renew. Sustain. Energy Rev. 55, 729–738. doi:10.1016/j.rser.2015.10.038
Yu, H. (2016). Ecosystem restoration and regeneration of Lake Biwa basin, Japan. Res. Environ. Sci. 29 (01), 36–43. doi:10.13198/j.issn.1001-6929.2016.01.05
Zhang, X., Zhang, G. S., Long, X., Zhang, Q., Liu, D. S., Wu, H. J., et al. (2021). Identifying the drivers of water yield ecosystem service: A case study in the Yangtze River basin, China. Ecol. Indic. 132, 108304. doi:10.1016/j.ecolind.2021.108304
Keywords: InVEST model, water conservation services, grey water footprint, ecocompensation priority sequence, ecological compensation threshold
Citation: Guan D, Wu L, Cheng L, Zhang Y and Zhou L (2022) How to measure the ecological compensation threshold in the upper Yangtze River basin, China? An approach for coupling InVEST and grey water footprint. Front. Earth Sci. 10:988291. doi: 10.3389/feart.2022.988291
Received: 08 July 2022; Accepted: 23 August 2022;
Published: 12 September 2022.
Edited by:
Ke Zhang, Hohai University, ChinaReviewed by:
Ehsan Elahi, Shandong University of Technology, ChinaYuechen Li, Southwest University, China
Chuanhao Wen, Yunnan University, China
Copyright © 2022 Guan, Wu, Cheng, Zhang and Zhou. This is an open-access article distributed under the terms of the Creative Commons Attribution License (CC BY). The use, distribution or reproduction in other forums is permitted, provided the original author(s) and the copyright owner(s) are credited and that the original publication in this journal is cited, in accordance with accepted academic practice. No use, distribution or reproduction is permitted which does not comply with these terms.
*Correspondence: Lidan Cheng, Y2xkaGVpcGluZ2d1b0AxNjMuY29t